Abstract
De novo assembled alphoidtetO-type human artificial chromosomes (HACs) represent a novel promising generation of high capacity episomal vectors. Their function and persistence, and any adverse effects, in various cell types in live animals, have not, however, been explored. In this study we transferred the alphoidtetO-HAC into mouse ES cells and assessed whether the presence of this extra chromosome affects their pluripotent properties. AlphoidtetO-HAC-bearing ES cells were indistinguishable from their wild-type counterparts: they retained self-renewal potential and full capacity for multilineage differentiation during mouse development, whereas the HAC itself was mitotically and transcriptionally stable during this process. Our data provide the first example of fully synthetic DNA behaving like a normal chromosome in cells of living animals. It also opens a new perspective into functional genetic studies in laboratory animals as well as stem cell-based regenerative medicine.
Abbreviations
Bsd | = | blasticidin |
DAPI | = | 4′, 6-Diamidino-2-Phenylindole |
dpc | = | days post coitum |
EGFP | = | enhanced green fluorescent protein |
ES cells | = | embryonic stem cells |
FISH | = | fluorescent in situ hybridization |
HAC | = | human artificial chromosome |
HAT | = | hypoxanthine-aminopterin-thymidine |
iPS cells | = | induced pluripotent stem cells |
MMCT | = | microcell mediated chromosome transfer |
PBS | = | phosphate buffered saline |
PFA | = | paraformaldehyde |
Introduction
Gene therapy remains a challenging approach for the treatment of various disorders, including monogenic and polygenic diseases. Gene therapy requires either the correction of a defective gene or provision of a wild-type copy of a damaged gene. The former can be addressed using recently developed gene editing tools: Zinc Finger Nucleases (ZFNs), Transcription Activator-Like Effector Nucleases (TALENs), and, most potently, the Clustered Regularly Interspaced Short Palindromic Repeat (CRISPR)/CAS9.Citation1 For the latter approach, virus-based delivery systems are usually used, but there is no available system that simultaneously meets all the criteria of an ideal gene therapy vector: high cloning capacity, episomal maintenance, stable long-term expression of a gene of interest in quiescent as well as dividing cells, and lack of toxicity or immunogenicity. Typically, some of these criteria are achieved with a trade-off of others. All of them, however, are readily met by one class of DNA vectors, the Human Artificial Chromosomes (HACs).
Most characterized HACs have been generated by a top-down approach involving truncation of various human chromosomes,Citation2,3 which, strictly speaking, makes them modified natural chromosomes (mini-chromosomes) rather than artificial ones. All these HACs are maintained as episomes and are mitotically stable. The most advanced HAC of this type (21HAC) was generated by truncation of human chromosome 21.Citation4 The 21HAC, presumably free of any known genes, has been further modified to contain multiple recombination sites to facilitate loading of sequences of interest.Citation5 Importantly, this HAC has demonstrated its utility as a high capacity gene therapy vector in mouse models of muscular dystrophies.Citation3,Citation6-8 This HAC has also been successfully used to deliver reprogramming factors, but its subsequent removal from de novo generated iPS cells is quite problematic and relies on spontaneous loss during mitotic divisions, which is extremely rare.Citation9
A novel, truly artificial HAC has recently come to the fore as a highly promising vector system. This HAC has been assembled de novo from a synthetic alphoid DNA array with embedded tetracycline operator (tetO) that binds tet-repressor fusion proteins, providing the option to incorporate conditional inhibition of kinetochore assembly, resulting in subsequent loss of the HAC from populations of dividing cells.Citation10-13 The bottom-up assembled alphoidtetO-HAC vector therefore has a significant advantage over top-down constructed HACs because it can be deployed in a hit-and-run fashion, which is the preferred option for several applications. The megabase-size synthetic alphoid DNA array of the alphoidtetO-HAC is fully defined,Citation14 ruling out any encoding of undesired cryptic transcripts. In addition, structural integrity of the HAC during gene loading and transfer into different host cells has been demonstrated, along with the high mitotic and transcriptional stability of embedded genes over multiple rounds of cell division in culture.Citation15,16 Although the alphoidtetO-HAC vector seems to satisfy many features required for a gene delivery vector, data have been lacking regarding its behavior in vivo, i.e. in the context of tissues and organs in the developing and adult organism. Contrary to the described above top-down constructed mini-chromosomes, the behavior of the de novo synthesized alphoidtetO-HACs in living organism is highly unpredictable due their synthetic nature. To address this issue, we have generated mouse ES cells bearing an alphoidtetO type HAC and then demonstrated its tolerance in vitro by the pluripotent cells and differentiated cells derived thereof, as well as its robust maintenance and expression throughout mouse ontogeny.
Materials and Methods
Ethics statement
All animal procedures were performed according to the guidelines for the humane use of laboratory animals, with standards corresponding to those prescribed by the American Physiological Society. Mouse work was performed strictly in agreement with the animal protection legislation acts of the Russian Federation, and was approved as humane use of laboratory animals by the Institute's Ethical Board.
Cell culture
All media and components were from Life Technologies and Sigma, unless indicated. CHO (Chinese Hamster Ovary) cells were routinely maintained in 5% CO2 atmosphere in DMEM/F12 medium supplemented with 10% Fetal Bovine Serum, 100 U/ml penicillin, 100 mg/ml streptomycin, 2 mM L-glutamine. Mouse ES cells (E14 Tg2a, BayGenomics) were cultured on gelatin-coated dishes in Knockout-DMEM supplemented with 15% ES cell-qualified fetal bovine serum, 100 U/ml penicillin, 100 mg/ml streptomycin, 2 mM L-glutamine, non-essential amino acids, 50 μM β-mercaptoethanol, 1000 U/ml LIF (PAA). For routine passaging, cells were rinsed in PBS, treated with TrypLE and split 1:4. Mouse tail-tip fibroblasts were grown in DMEM containing 1 g/l glucose (Gibco, Germany), supplemented with 10% Fetal Bovine Serum, 100 U/ml penicillin, 100 mg/ml streptomycin, and 2 mM L-glutamine.
Microcell-mediated chromosome transfer (ММСТ)
This procedure was performed as described elsewhere.Citation15,17 Microcells were collected from 1 × 10Citation8 CHO cells containing the alphoidTetO-HAC carrying GFP gene. HAC was transferred into E14 mouse ES cells (3 × 106) via fusion of microcells with target cells. For fusion we used Neo EX HVJ Envelope Transfection Kit (Cosmo Bio, Japan). Bsd selection (4 μg/ml) was applied 48 hrs later and ES cell clones were picked after 2 weeks of growth in the selective conditions.
Immunocytochemistry
ES cells were fixed in 4% paraformaldehyde (Sigma)-PBS, permeabilised in 0.1% Triton X-100 (Sigma)-PBS, incubated with blocking buffer (3% BSA-PBS) for 30 minutes. Samples were then incubated overnight at 4°C with primary antibodies to Oct4 (sc-5279, Santa Cruz Biotechnology Inc.), Nanog (REC-RCAB0002P-F, COSMO BIO CO., Tokyo, Japan), SSEA-1 (MC-480, Developmental Studies Iowa Hybridoma Bank), all diluted 1:100 in the blocking buffer supplemented with 0.1% Tween20. Then samples were rinsed 5 times in washing buffer (0.1% Tween in PBS) and stained with goat anti-mouse-Cy3 (Jackson Immunoresearch 115-165-146) or donkey anti-Rabbit-Cy3 (Jackson Immunoresearch 711-165-152) for 1 hour. Subsequently, samples were washed 5 times in washing buffer and counterstained with 10 mg/ml Hoechst 33342 (Sigma). Stained cells were examined on a Keyence BZ-9000 fluorescent microscope with 40x-air and 63x-oil immersion objectives or on a confocal Leica TCS SP5 microscope with 206air and 636oil immersion objectives. UV (405 nm), Argon (488 nm) and HeNe (633 nm) lasers were used to excite the fluorophores.
Teratoma formation test
Mouse ES cell carrying HAC (clones A1, B1, C1, D1, F1) exponentially growing on gelatin coated dishes in ES medium, were harvested with TrypLE express (Gibco), resuspended in PBS and injected subcutaneously (1 × 106 cells) into athymic CD-1 NUDE mice. After 4–6 weeks teratomas were removed from euthanized animals and processed for histological and immunohistochemical analysis.
Immunohistochemistry
Teratomas were excised and fixed in 4% PFA prepared on PBS at 4°C overnight. After washing in PBS (2 times, each for 2 hrs) they were dehydrated in an ethanol series (70–100%) and isobutanol : paraffin series (2:1-1:1-1:2) and were embedded in paraffin (Sigma). 7 μm sections were prepared using Leitz 1208 microtome (Germany). Paraffin sections were washed in xylene, rehydrated through an ethanol series (100–70%) and washed in 0.1% Tween20-PBS. After blocking in 1% BSA-0.1% Tween20-PBS for 30 minutes sections were incubated overnight with primary antibodies against GFP (Life Technologies A-11120) at 4°C. Then, sections were washed several times in 0.1% Tween20-PBS and incubated with secondary antibodies conjugated with Alexa488 (Abcam ab150113) for 3 hrs at room temperature (RT). After that, sections were washed in 0.1% Tween20-PBS, counterstained with DAPI and embedded into an anti-fading media under coverslips. Primary and secondary antibodies were diluted in 1% BSA-0.1% Tween20-PBS, respectively, 1:200 and 1:500. The samples were examined on BZ-9000 (Keyence) or Axiovert 40 (Zeiss) fluorescent microscopes.
Preparation of metaphase spreads
Metaphase spreads were prepared as previously described in refs. 18 and 19 with modifications. Exponentially growing (80% confluent) cells were treated for 2–4 hrs at 37°C with 0,1 μg/ml colcemid (KaryoMAX, Life Technologies) in 5% CO2 atmosphere. Cells were harvested by trypsinization and incubated in hypotonic (0.56% KCl) solution for 20 min. After that cells were fixed in a fixative solution (Methanol/Acetic acid 3:1, v/v) washed 3 times in the fixative solution and stored, if necessary, in fixative solution at −20°C. For metaphase spreads the cells suspension was placed dropwise on pre-cleaned microscope glass slides (Superfrost, Thermo Scientific, Germany), air-dried and aged at least 3 days or longer at RT in dust-free place. For preparation of metaphases from tail-tip fibroblasts, the cells were cultured overnight in 0.05 μg/ml colcemid at 37°C in 5% CO2 atmosphere and processed as described above.
Fish
Fluorescent in situ hybridization analysis was performed as described elsewhere with modifications. The construct containing the alphoid-tetO array p3.5Citation20 was labeled with Alexa546, using the ULYSIS® Nucleic Acid Labeling Kit (Life Technologies) as per manufacturer recommendations. This labeled probe was denatured at 75°C for 5 min, then incubated in hybridization solution containing 50% formamide at 37°C for 30–60 min, and finally incubated overnight with denatured and dehydrated metaphase spreads under the cover slip in a humidified chamber. The slides were washed once in 50% formamide / 2x SSC at 42°C, twice in 2x SSC at 42°C, once in 1x SSC at 42°C, briefly rinsed with ddH2O, air-dried in the dark, and mounted into VECTASHIELD medium containing DAPI (Vector Laboratories). Slides were examined on a BZ-9000 (Keyence) fluorescent microscope with 40x-air and 63x-oil immersion objectives.
Blastocyst injection
Mouse ES cell carrying HAC (clones B1, C1) were trypsinized, resuspended in a small amount of ES-medium and kept on ice until the injection. Embryos were recovered by flushing the uterine horns with M2 medium (Sigma) from superovulated 23–25 days old C57Bl/6 inbred female mice at 3.5 dpc, as previously described.Citation21 15–17 ES-HAC cells were injected per blastocyst and embryos were transferred into uterine horns of 2.5 dpc or to the oviducts of 0.5 dpc anesthetized pseudopregnant CB6F1 females (10–12 embryos per recipient).
Isolation of mouse tail-tip fibroblasts
Tail tip (approximately 1 cm) biopsies were minced by scalpel to small pieces, incubated with 1 mg/ml collagenase IV (Sigma) for 15 min at 37°C, washed with PBS, additionally treated with TrypLE express (Life Technologies) for 20 min at 37°C, resuspended in fibroblast culture medium, collected by centrifugation, resuspended in the same medium and plated in a well of 6-well plate. Culture media was changed every second day.
Results
The alphoidtetO-HAC, featuring a CAG-EGFP-polyA cassette flanked by the cHS4 insulators (), was assembled in CHO cells ( and S1) and then delivered into mouse ES cells. For over 30 years, the only known route to intercellular chromosome transmission remains MMCT. This approach is efficient (10−5) for alphoidtetO-HAC delivery into various cultured cancer cell types,Citation15,16,22 but has repeatedly failed for ES cell recipients. After an assessment of a series of modifications of the original microcell mediated chromosome transfer (MMCT) method, however, we have developed a protocol for EGFP-HAC delivery into mouse ES cells, albeit with limited efficiency (10−6). The modifications included the pre-mixing of HAC-containing microcells and recipient ES cells with the Sendai virus envelope prior to their fusion (see Materials and Methods).
Figure 1. Derivation and characterization of EGFP-HAC. (A) Schematic representation of the 1.1-Mb EGFP-HAC constructed via Cre/loxP-mediated uploading of the CAG-EGFP-polyA cassette with cHS4 insulators onto de novo synthesized alphoidtetO-HAC (see Figure S1 for details and abbreviations) (B) Fluorescent bioimaging of EGFP in living donor CHO cells carrying the assembled EGFP-HAC. (C) Live EGFP imaging in mouse ES cells harboring the EGFP-HAC transferred therein from the CHO cells via the MMCT procedure. Shown are combined transmission light and fluorescent images (B, C). (D) FISH analysis of a metaphase plate of the EGFP-HAC ES cells (clone C1), using a Cy3-labeled DNA probe specific to the alphoid repeat. The DNA was stained with DAPI (blue). The EGFP-HAC is visible as a pink dot (white arrow). (E) Fluorescent immunostaining of the EGFP-HAC ES cells (clone C1) with indicated primary antibodies.
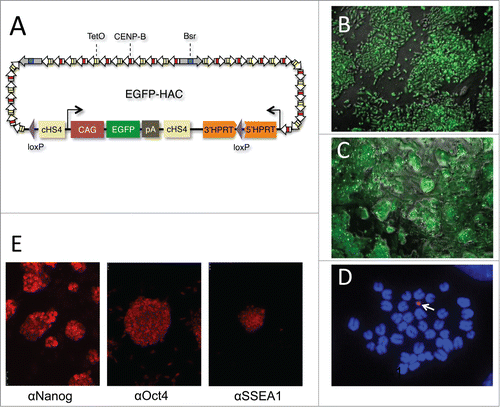
Five morphologically undifferentiated EGFP-HAC-bearing ES cell colonies ( and S2) selected in blasticidin (Bsd)-containing medium were picked, expanded, and analyzed by FISH (). Two EGFP-HAC ES cell clones (B1 and C1) that showed mostly normal male karyotype cells (40XY) and autonomic EGFP-HAC () were chosen for further analysis. The other 3 clones showed either abnormal karyotypes or HAC integration into host chromosomes, and were discarded. ES cell clones B1 and C1 were morphologically indistinguishable from their wild-type counterpart, stably expressed EGFP for at least 15 passages (Fig. S3), and expressed the pluripotency markers Oct4, Nanog, and SSEA-1 (). When injected into NUDE mice, these cells formed teratomas with clearly visible differentiated cell types belonging to all 3 germ layers. Immunostaining revealed widespread expression of EGFP in the germ layers throughout the tumor (). These data taken together suggest that the presence of the alphoidtetO-HAC in mouse ES cells does not affect pluripotent properties such as self-renewal and multilineage differentiation capacity. In addition, this alphoidtetO-HAC is mitotically stable and maintains the expression of the constituent EGFP marker gene throughout multiple cell divisions and processes of differentiation into virtually all cellular types present in teratomas.
Figure 2. Multilineage differentiation capacity of the EGFP-HAC ES cells within teratomas, exemplified by the formation of ciliated epithelium (ectoderm germ layer), skeletal muscles (mesoderm germ layer), and gut epithelium (endoderm germ layer), all showing EGFP expression following immunofluorescent staining (lower row). H&E – hematoxylin-eosin staining (upper row).
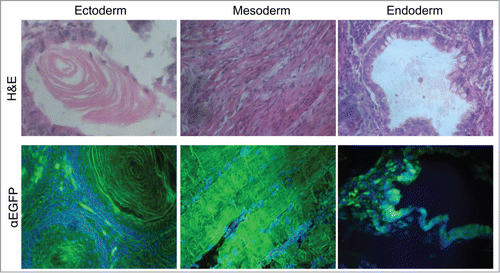
Our next goal was to assess the ability of the EGFP-HAC-bearing ES cells to contribute to embryonic development following their injection into mouse blastocysts (Fig. S4), and to evaluate the mitotic and transcriptional robustness of this HAC during this process. First, we analyzed 10.5 dpc chimeric embryos and found widespread EGFP-HAC-bearing ES cell descendants (). We next examined live born chimeric mice (). EGFP-HAC was found to be maintained as an autonomous chromosome unit, assessed by FISH analysis of primary fibroblast cultures derived from the tail tips of chimeric mice (). We detected significant contribution of EGFP-HAC ES cell clones (B1 and C1) to cell types of all 3 germ layers (). The data support the conclusions of the previous teratoma test results − namely, that alphoidtetO-HAC is highly mitotically stable and effectively maintains transcriptionally active genes through differentiation from the pluripotent epiblast to terminally differentiated cells of adult mice. The data further suggest that the alphoidtetO-HAC is well tolerated by these somatic cell types.
Figure 3. Contribution of EGFP-HAC ES cells to mouse development following blastocyst injection. (A) A representative 10.5 dpc chimeric embryo. (B) A representative 4-week old chimeric mouse. (C) Tail tip of a chimera, which was used to establish primary culture of fibroblasts. (D) FISH analysis of the established fibroblasts, using a Cy3-labeled DNA probe specific to the alphoid repeat. (E–J) EGFP visualization in paraffin sections of representative organs of the chimeric adult mice. Shown are sections of brain (E) and retina (F), both of ectoderm germ layer, heart (G) and skeletal muscles (H) of mesoderm origin, and bronchial epithelial cells (I) and liver hepatocytes (J), both belonging to the endoderm germ layer.
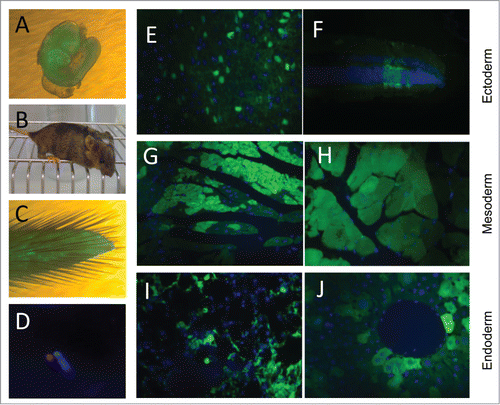
Discussion
HACs provide powerful systems with great potential to study kinetochore and centromere functions, chromosome instability and chromosome mis-segregation in cancer cells, screening of anticancer drugs and environmental mutagens, production of biopharmaceutical proteins and human antibodies, etc. (reviewed in ref. 2 and references therein). Certain functional features, such as virtually unlimited cloning capacity, lack of host genome perturbations, high mitotic and transcriptional stability, and, as incorporated uniquely into alphoidtetO HACs, the option of conditional removal, make HACs a highly versatile gene delivery tool, overcoming most of the problems of viral and non-viral vector systems. A major caveat of this vector system, however, has been that HACs cannot be efficiently delivered to target cells or tissues of the body but require carrier cells that can be manipulated ex vivo and re-introduced into embryos or adult organisms. The carrier cells must meet key criteria: clonogenicity, substantial self-renewal capacity, genetic and epigenetic stability, and differentiation capacity. Obviously, pluripotent stem cells, such as embryo-derived ES cells Citation23 and soma-derived iPS cells Citation24 are ideal HAC carriers that fulfill all above criteria. Some adult stem cell types such as endodermal Lgr5+ stem cells and mesoangioblasts have comparable advantages, but their differentiation potential is limited only to respective adult cell types.Citation7,Citation25-27 This study shows, for the first time, successful delivery of the alphoidtetO-HAC into mouse ES cells and its stable maintenance throughout differentiation of these cells into somatic cell types of adult mice.
AlphoidtetO-HACs could be valuable tools to investigate rodent models of human diseases. Introducing human full-size genes into mice offers the opportunity to analyze in vivo function or screen for therapeutic molecules. This paper shows that this can be done via ES cells, which opens an opportunity to develop specific human disease models and is a first step toward future application of HACs in tackling recessive hereditary human diseases (Fig. S5). Clearly, there are many critical issues that must be further addressed: whether and how alphoidtetO-HACs are transmitted into the mouse germline; whether these HACs can be delivered into human ES, iPS and adult stem cells and how they are maintained in these cells and differentiated cells derived thereof; and whether alphoidtetO-HAC carrying reprogramming factors can be used for iPS cell derivation. Novel HAC transfer methods could also overcome the limitations of MMCT. Nevertheless, based on the data thus far, ES cells containing HACs provide a route to develop specific disease models in mice as a first step to conceivable applications in alleviating recessive hereditary human diseases.
Disclosure of Potential Conflicts of Interest
No potential conflicts of interest were disclosed.
Author Contributions
Conceived and designed the experiments: ML, VL, AT. Performed the experiments: ML, EP, SP. Analyzed the data: ML, SP, AT. Contributed reagents/materials/ analysis tools: VL, NK. Wrote the paper: ML, AT. Final approval of the manuscript: ML, NA, MB, NK, VL, AT.
Supplemental_Material.pdf
Download PDF (9.6 MB)Acknowledgments
We are grateful to David Schlessinger for valuable comments and proofreading of the manuscript.
Funding
This work was supported by International Academy of Life Science (IALS) grant, Boehringer Ingelheim Fonds (BIF) grant, President's stipend for young scientists (3805.2013.4), DAAD (A/12/87445) to ML and NA, German Ministry for Education and Research (BMBF) grant to MB (01DJ13012), Russian Foundation for Basic Research grant to ML (14-04-31556) and to AT (13-02-00923). The part of the study dedicated to chromosome analysis was supported by the Russian Science Foundation (project No 14-50-00068) and the Federal Agency of Scientific Organizations (Russia).
Supplemental Material
Supplemental data for this article can be accessed on the publisher's website.
References
- Hsu PD, Lander ES, Zhang F. Development and applications of CRISPR-Cas9 for genome engineering. Cell 2014; 157:1262-78; PMID:24906146; http://dx.doi.org/10.1016/j.cell.2014.05.010
- Kouprina N, Tomilin AN, Masumoto H, Earnshaw WC, Larionov V. Human artificial chromosome-based gene delivery vectors for biomedicine and biotechnology. Expert Opin Drug Deliv 2014; 11:517-35; PMID:24479793; http://dx.doi.org/10.1517/17425247.2014.882314
- Kazuki Y, Hiratsuka M, Takiguchi M, Osaki M, Kajitani N, Hoshiya H, Hiramatsu K, Yoshino T, Kazuki K, Ishihara C, et al. Complete genetic correction of ips cells from Duchenne muscular dystrophy. Mol Ther 2010; 18:386-93; PMID:19997091; http://dx.doi.org/10.1038/mt.2009.274
- Kazuki Y, Hoshiya H, Takiguchi M, Abe S, Iida Y, Osaki M, Katoh M, Hiratsuka M, Shirayoshi Y, Hiramatsu K, et al. Refined human artificial chromosome vectors for gene therapy and animal transgenesis. Gene Ther 2011; 18:384-93; PMID:21085194; http://dx.doi.org/10.1038/gt.2010.147
- Yamaguchi S, Kazuki Y, Nakayama Y, Nanba E, Oshimura M, Ohbayashi T. A method for producing transgenic cells using a multi-integrase system on a human artificial chromosome vector. PLoS One 2011; 6:e17267; PMID:21390305; http://dx.doi.org/10.1371/journal.pone.0017267
- Hoshiya H, Kazuki Y, Abe S, Takiguchi M, Kajitani N, Watanabe Y, Yoshino T, Shirayoshi Y, Higaki K, Messina G, et al. A highly stable and nonintegrated human artificial chromosome (HAC) containing the 2.4 Mb entire human dystrophin gene. Mol Ther 2009; 17:309-17; PMID:19034264; http://dx.doi.org/10.1038/mt.2008.253
- Tedesco FS, Hoshiya H, D'Antona G, Gerli MF, Messina G, Antonini S, Tonlorenzi R, Benedetti S, Berghella L, Torrente Y, et al. Stem cell-mediated transfer of a human artificial chromosome ameliorates muscular dystrophy. Sci Transl Med 2011; 3:96ra78; PMID:21849666; http://dx.doi.org/10.1126/scitranslmed.3002342
- Tedesco FS, Gerli MF, Perani L, Benedetti S, Ungaro F, Cassano M, Antonini S, Tagliafico E, Artusi V, Longa E, et al. Transplantation of genetically corrected human iPSC-derived progenitors in mice with limb-girdle muscular dystrophy. Sci Transl Med 2012; 4:140ra89; PMID:22745439; http://dx.doi.org/10.1126/scitranslmed.3003541
- Hiratsuka M, Uno N, Ueda K, Kurosaki H, Imaoka N, Kazuki K, Ueno E, Akakura Y, Katoh M, Osaki M, et al. Integration-free iPS cells engineered using human artificial chromosome vectors. PLoS One 2011; 6:e25961; PMID:21998730; http://dx.doi.org/10.1371/journal.pone.0025961
- Ebersole T, Okamoto Y, Noskov VN, Kouprina N, Kim JH, Leem SH, Barrett JC, Masumoto H, Larionov V. Rapid generation of long synthetic tandem repeats and its application for analysis in human artificial chromosome formation. Nucleic Acids Res 2005; 33:e130; PMID:16141190; http://dx.doi.org/10.1093/nar/gni129
- Iida Y, Kim JH, Kazuki Y, Hoshiya H, Takiguchi M, Hayashi M, Erliandri I, Lee HS, Samoshkin A, Masumoto H, et al. Human artificial chromosome with a conditional centromere for gene delivery and gene expression. DNA Res 2010; 17:293-301; PMID:20798231; http://dx.doi.org/10.1093/dnares/dsq020
- Kim JH, Kononenko A, Erliandri I, Kim TA, Nakano M, Iida Y, Barrett JC, Oshimura M, Masumoto H, Earnshaw WC, et al. Human artificial chromosome (HAC) vector with a conditional centromere for correction of genetic deficiencies in human cells. Proceedings of the National Academy of Sciences of the United States of America 2011; 108:20048-53.
- Nakano M, Cardinale S, Noskov VN, Gassmann R, Vagnarelli P, Kandels-Lewis S, Larionov V, Earnshaw WC, Masumoto H. Inactivation of a human kinetochore by specific targeting of chromatin modifiers. Dev Cell 2008; 14:507-22; PMID:18410728; http://dx.doi.org/10.1016/j.devcel.2008.02.001
- Kouprina N, Samoshkin A, Erliandri I, Nakano M, Lee HS, Fu H, Iida Y, Aladjem M, Oshimura H, Masumoto H, et al. Organization of synthetic alphoid DNA array in human artificial chromosome (HAC) with a conditional centromere. ASC Synthetin Biology 2012; 1:590-601; PMID:23411994; http://dx.doi.org/10.1021/sb3000436
- Lee NC, Kononenko AV, Lee HS, Tolkunova EN, Liskovykh MA, Masumoto H, Earnshaw WC, Tomilin AN, Larionov V, Kouprina N. Protecting a transgene expression from the HAC-based vector by different chromatin insulators. Cell Mol Life Sci 2013; 70:3723-37; PMID:23677492; http://dx.doi.org/10.1007/s00018-013-1362-9
- Kononenko AV, Bansal R, Lee NC, Grimes BR, Masumoto H, Earnshaw WC, Larionov V, Kouprina N. A portable BRCA1-HAC (human artificial chromosome) module for analysis of BRCA1 tumor suppressor function. Nucleic Acids Res 2014; 42; PMID: 25260588; http://dx.doi.org/10.1093/nar/gku870
- Tomizuka K, Yoshida H, Uejima H, Kugoh H, Sato K, Ohguma A, Hayasaka M, Hanaoka K, Oshimura M, Ishida I. Functional expression and germline transmission of a human chromosome fragment in chimaeric mice. Nat Genet 1997; 16:133-43; PMID:9171824; http://dx.doi.org/10.1038/ng0697-133
- Campos PB, Sartore RC, Abdalla SN, Rehen SK. Chromosomal spread preparation of human embryonic stem cells for karyotyping. J Vis Exp 2009; PMID:19734841
- Liskovykh M, Chuykin I, Ranjan A, Safina D, Popova E, Tolkunova E, Mosienko V, Minina JM, Zhdanova NS, Mullins JJ, et al. Derivation, characterization, and stable transfection of induced pluripotent stem cells from Fischer344 rats. PLoS One 2011; 6:e27345; PMID:22076153; http://dx.doi.org/10.1371/journal.pone.0027345
- Kononenko AV, Lee NC, Liskovykh M, Masumoto H, Earnshaw WC, Larionov V, Kouprina N. Generation of a conditionally self-eliminating HAC gene delivery vector through incorporation of a tTAVP64 expression cassette. Nucleic Acids Res 2015; PMID: 25712097; http://dx.doi.org/10.1093/nar/gkv124
- Popova E, Rentzsch B, Bader M, Krivokharchenko A. Generation and characterization of a GFP transgenic rat line for embryological research. Transgenic Res 2008; 17:955-63; PMID:18523856; http://dx.doi.org/10.1007/s11248-008-9189-0
- Ebersole T, Kim JH, Samoshkin A, Kouprina N, Pavlicek A, White RJ, Larionov V. tRNA genes protect a reporter gene from epigenetic silencing in mouse cells. Cell Cycle 2011; 10:2779-91; PMID:21822054; http://dx.doi.org/10.4161/cc.10.16.17092
- Evans MJ, Kaufman MH. Establishment in culture of pluripotential cells from mouse embryos. Nature 1981; 292:154-6; PMID:7242681; http://dx.doi.org/10.1038/292154a0
- Takahashi K, Yamanaka S. Induction of pluripotent stem cells from mouse embryonic and adult fibroblast cultures by defined factors. Cell 2006; 126:663-76; PMID:16904174; http://dx.doi.org/10.1016/j.cell.2006.07.024
- Huch M, Boj SF, Clevers H. Lgr5(+) liver stem cells, hepatic organoids and regenerative medicine. Regenerative medicine 2013; 8:385-7; PMID:23826690; http://dx.doi.org/10.2217/rme.13.39
- Sato T, Clevers H. Growing self-organizing mini-guts from a single intestinal stem cell: mechanism and applications. Science 2013; 340:1190-4; PMID:23744940; http://dx.doi.org/10.1126/science.1234852
- Barker N, Rookmaaker MB, Kujala P, Ng A, Leushacke M, Snippert H, van de Wetering M, Tan S, Van Es JH, Huch M, et al. Lgr5(+ve) stem/progenitor cells contribute to nephron formation during kidney development. Cell Rep 2012; 2:540-52; PMID:22999937; http://dx.doi.org/10.1016/j.celrep.2012.08.018