Abstract
The vascular basement membrane (BM) is a thin and dense cross-linked extracellular matrix layer that covers and protects blood vessels. Understanding how cells cross the physical barrier of the vascular BM will provide greater insight into the potentially critical role of vascular BM breaching in cancer extravasation, leukocyte trafficking and angiogenic sprouting. In the last year, new evidence has mechanistically linked the breaching of vascular BM with the formation of specific cellular micro-domains known as podosomes and invadopodia. These structures are specialized cell-matrix contacts with an inherent ability to degrade the extracellular matrix. Specifically, the formation of podosomes or invadopodia was shown as an important step in vascular sprouting and tumor cell extravasation, respectively. Here, we review and comment on these recent findings and explore the functions of podosomes and invadopodia within the context of pathological processes such as tumor dissemination and tumor angiogenesis.
Introduction
A large proportion of cancer deaths are due to distant metastatic occurrences – a complex multistep process of cancer cells spreading from the primary tumor to distant parts of the body. Tumor cells that successfully disseminate from the primary tumor and are able to survive in the vascular system eventually extravasate across endothelium to colonize secondary sites. Extravasation requires cancer cells to arrest and adhere to the vascular endothelium and then cross the endothelial cell (EC) barrier. Once cells have crossed the endothelial cell layer, they must drill the vascular BM. This process is similar to breaching of epithelial BMs, where integrins and proteases have been extensively described as crucial.Citation1,2 All these steps are extremely inefficient. This is demonstrated by the high frequency of circulating cancer cells in the bloodstream compared to the relatively lower occurrence of metastases. Nonetheless, these physical barriers can be overcome, and the immune system accomplishes these steps routinely. During the course of immune surveillance, immune cells can penetrate the BM and cross a range of peripheral and vascular tissues.Citation3 To cross a densely cross-linked matrix barrier such as the vascular BM, cancer cells need to acquire specific features, such as those seen in leukocytes. This peculiar style of migration is caused by physical features of the vascular BM. Indeed, in other less-dense matrices, both leukocytes and cancer cells can rapidly move by squeezing through available pores without the need for integrins or proteases.Citation4
The vascular BM also represents a physical barrier for angiogenic ECs. In stable vessels, ECs typically form a cobblestone-like monolayer of polarized cells. This quiescent phenotype is maintained until ECs detect pro-angiogenic signals that induce alterations in their behavior. In response to angiogenic cues – especially vascular endothelial growth factor (VEGF) – ECs lose their tight cell-to-cell contacts, activate proteases that degrade the surrounding BM, and acquire invasive and motile behavior to initiate new blood vessel sprouting.
BMs are dense cross-linked matrices that have a wide range of functions in many tissues. Mainly, they function as thin boundaries between different tissue compartments. Type-IV collagen and laminins are the 2 major components of BMs. They self-assemble to form networks conferring structural stability and biological activity Citation5 with pores around 10 nm in diameter.Citation6 The vascular BM expresses only 2 laminin isoforms: (i) laminin 411 (formerly called laminin 8), composed of laminin α4, β1, and γ1 chains, and (ii) laminin 511 (formerly 10), composed of laminin α5, β1, and γ1 chains. There are several other components within the vascular BM that play important roles, such as heparan sulfate proteoglycans, nidogens, SPARC, fibulins, collagen types VIII, XV, and XVIII, and thrombospondins.Citation5
Understanding how cells pass through the physical barrier of the vascular BM is of great interest because crucial for cancer extravasation, leukocyte trafficking and angiogenic sprouting.Citation7,8 However, the in vivo mechanism of breaching and invasion of the vascular BM was mostly unknown for years.
Recently, in vivo and ex vivo observations of vascular BM invasion have been reported in 2 works published in Cell Reports Citation9 and Nature Cell Biology Citation10 (). In these articles the authors showed that the process of vascular BM invasion during tumor cell extravasation or endothelial sprouting is dependent on the formation of invadopodia or podosomes, respectively. Podosomes and invadopodia, collectively known as invadosomes, are specialized cell-matrix contacts with an inherent ability to degrade extracellular matrix (ECM). These micro-domains of plasma membrane were discovered in the 1980s in Rous Sarcoma virus (RSV)-transformed fibroblasts and malignant B cells.Citation11,12 Structurally, all types of invadosomes are visualized as punctate dynamic microdomains formed at the ventral membrane. The structures are highly enriched in filamentous actin (F-actin) that polymerises perpendicularly to the substratum.Citation13 Podosomes clearly appear as bipartite entities where the actin core is surrounded by a ring structure containing integrins and other focal adhesion (FA) proteins such as actin binders, signaling molecules and scaffold proteins. As podosomes, mature invadopodia also contain FA proteins.Citation14 Besides these common traits, podosomes and invadopodia differ in their architecture. Podosomes extend upwards from the ventral cell surface into the cytoplasm while invadopodia appear as long membrane extensions that penetrate into the ECM.Citation15 How this difference could reflect a distinct function is unclear. However, these recent findings suggest that both podosomes and invadopodia are structures perfectly suitable for BM breaching.
Figure 1. The process of vascular BM invasion during tumor cell extravasation or endothelial sprouting is dependent on the formation of invadopodia or podosomes, respectively. Podosomes and invadopodia, collectively known as invadosomes, are specialized cell-matrix contacts with an inherent ability to degrade ECM. Molecular players involved in invadopodia formation are cortactin, Tks4/5 and MT1-MMP. VEGF and α6 integrin are crucial for endothelial podosome rosette formation while MT1-MMP is enriched in podosome rosettes.
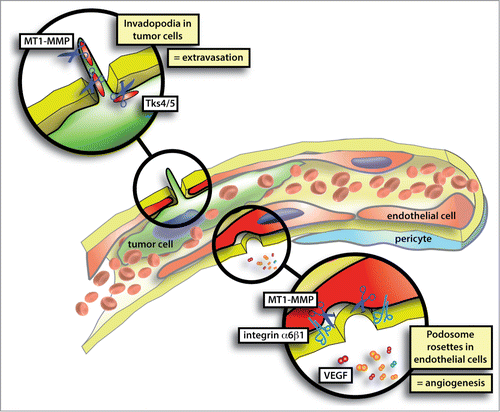
Podosome rosettes and sprouting angiogenesis
Individual podosomes and podosome clusters were described in cultured arterial, venous and microvascular ECs.Citation16-19 Structurally, endothelial podosomes resemble podosomes found in monocytic cells Citation20 and transformed fibroblasts.Citation11 They consist of a ring of adhesive and proteolytic molecules encircling an F-actin column Citation16 which stands perpendicular to the plane of the ECM substrate and plasma membrane. Proteins involved in actin nucleation and architectural organization, such as N-WASP, VASP, Arp2/3, and cortactin, co-localize with the F-actin at the core.Citation16,17 Adhesion is performed by integrin receptors and integrin-associated proteins, such as talin, vinculin and paxillin localized to the adhesive ring.Citation16,17 ECM degradation is carried out by matrix metalloproteases (MMPs), most notably MT1-MMP. This transmembrane enzyme activates MMP2 and MMP9.Citation21,22
The arrangement of podosomes in circular arrays – so-called “rosettes” – is the most frequent presentation in ECs. This particular structural organization has generated some confusion due to nomenclature. In many reports, podosome rosettes are simply referred to as podosomes, which gives the idea that the molecular mechanisms involved in the formation and function of individual podosomes versus podosome rosettes were the same, but this is not true.
Podosome rosettes are typically larger than 3–4 μm and specifically localized at the endothelial basal side; this allows for in vivo visualization by confocal or multiphoton microscopy. Using these techniques, podosome rosettes were indeed visualised in TGFβ-stimulated aortic explants.Citation23 Immunofluorescence staining of aortic endothelium showed endothelial podosome rosettes in ex vivo tissues. More recently, we also noticed the existence of endothelial rosettes in VEGF-stimulated aortic explants. This prompted us to study other VEGF-stimulated vessels,Citation10 and we were able to detect podosome rosettes in sprouting vessels in adult angiogenic tissues in vivo. This included 2 VEGF-dependent mouse models of tumor angiogenesis, a mouse model of hind-limb ischemia, and clinical biopsies from human lung tumors.Citation10
Based on these findings, we proposed the hypothesis that podosome rosettes were specifically expressed by angiogenic endothelium, and we found this to be true in a mouse model of pancreatic insulinoma. The appearance of podosome rosettes increased during tumor progression from the hyperplastic to the malignant stage, and the peak coincided with the VEGF-driven angiogenic switch. The mechanism leading to the formation of podosome rosettes was the expression of α6 integrin (), which was induced by long stimulations with VEGF-A.Citation24 The functional significance of this finding remained elusive until we observed podosome rosette dynamics in an ex vivo sprouting angiogenesis model. Using the mouse aortic ring assay, we dynamically followed podosome rosette formation by using aortas from LifeAct-EGFP mice. First, we observed that podosome rosettes localized distally from the tip cell of growing angiogenic vessels. We also noticed that an endothelial podosome rosette preceded every new lateral branch, and modulating these sub-cellular structures changed the branching density of the angiogenic outgrowths. Moreover, we took advantage of in vivo α6 integrin targeting to confirm these mechanistic findings in tumor angiogenesis, thus demonstrating that podosome rosette blockade impairs vascular branching.
Endothelial podosome rosettes are able to enrich MMPs, such as MT1-MMP, in a specific area while avoiding the generalized release of proteolytic enzymes (). This allows the emergence of a new tip cell that crosses the vascular BM in a specific region, and does not expose intact or degraded BM to vessel lumen. Curiously, this process is analogous to the natural process of sprouting from tree branches (). In specific circumstances, quiescent adventitious buds breach the cortex and emerge to the surface and form new sprouts on the mature trunk or branch (). Similarly, the formation of localized breaches in the dense layer of the vascular BM is necessary for ECs to start the process of sprouting angiogenesis.
Figure 2. (A) Blood vessels are covered by a dense layer of ECM, the vascular BM. In tumors, ischemia or wound healing, quiescent vasculature is actually stimulated to form new vessels. Long time stimulation with pro-angiogenic factors, such as VEGF, induces angiogenic vessels to first overcome the physical barrier of vascular BM and generate a new tip cell and then to sprout by forming a new vessel. (B) Similarly, trees are covered by cortex that is a thin and dense layer protecting them. In the natural process of sprouting of a plant, adventitious buds breach the cortex in specific regions. Only where the cortex is breached the formation of new sprouts and branches is possible.
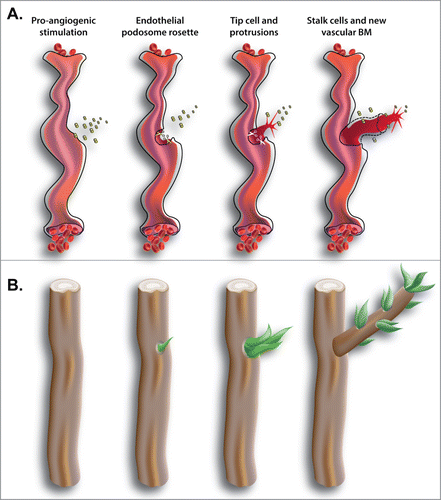
Invadopodia and tumor dissemination
The visualization of cancer cell invadopodia in living organisms has long been elusive due to the challenges associated with distinguishing them from other invasive structures such as lamellipodia. Extensive efforts to characterize invadopodia reveal that they are composed of structural proteins, such as cortactin, N-WASP, Tks4, and Tks5, as well as an enrichment of proteases, such as MT1-MMP, MMP9, and MMP2 for local release to drill the ECM.
Despite increasing evidence that invadopodia are prevalent in metastatic tumor cells and play a key role in the invasion and metastasis of cancer, direct evidence for their contribution in vivo was still lacking. While the formation of invadopodia to initiate intravasation into blood vessels was reported in animal models,Citation25,26 a link to extravasation process had been suggested only by ex vivo models data.
Now, Leong et al. provide direct evidence of the role of invadopodia during cancer cell extravasation.Citation9 Using the ex ovo chicken embryo model and an intravital microscopy platform, they visualized invadopodia formation in cells crossing the vascular wall barrier. Cancer cells injected in the chorioallantoic membrane (CAM) of the chicken embryo were restrained in its highly organized capillary bed network during the first 24 hours in circulation. During this process, dynamic cytoplasmic protrusions were observed at the leading edge of cells. A proportion of these protrusions extended into the adjacent stroma through endothelial junctions that were slightly detached. The enrichment of cortactin, Tks4, Tks5, and MT1-MMP in these invasive protrusions indicated that these structures were indeed invadopodia (). Furthermore, by in vivo loss-of-function experiments the authors elegantly demonstrated a requirement for cortactin, Tks4, and Tks5 in the formation of invadopodia as well as the direct consequences on cancer cell extravasation. Leong et al. showed that CAM and mouse lung extravasation rates were significantly decreased when any of these contributors to invadopodia were genetically depleted in cancer cells.
The depletion of Tks4 or Tks5 in cancer cells also led to a decrease in micro-metastases compared to control cancer cells. This suggests that the inhibition of invadopodia leads to a further decrease in metastatic efficiency. Their findings provide evidence for a functional role for invadopodia during cancer cell extravasation and distant metastasis, and this work reveals an opportunity for therapeutic intervention in a clinically relevant process. Although no direct evidences of vascular BM degradation were provided in this paper, it is likely that MT1-MMP in invadopodia contributes to degradation of the vascular BM, which facilitates cancer cells getting through the vascular wall barrier.
Conclusions and future perspectives
Podosomes and invadopodia were first discovered in the 1980s,Citation11 but their actual biological relevance was doubted for years. However, more recent studies have opened novel intriguing avenues in the investigation of invadosomes. New remarkable microscopic techniques provide the opportunity to study cellular processes and structures directly in situ by framing them in an in vivo context, and allow for the investigation of their biological relevance and correlation with clinically relevant pathological events.
Invadosomes are emerging as specialized cellular structures that degrade and invade BM while maintaining tissue integrity and cell-to-cell contacts. This is particularly relevant in vascular BM invasion where exposure of BM components, such as type-IV collagen, could trigger platelet activation and potential blood clot formation. Taking this into consideration, podosomes and invadopodia are perfect structures to avoid releasing vascular BM components during EC sprouting for new vessels and extravasation by circulating cells.
Interestingly, insights supporting the role of invadosomes in BM invasion – other than vascular BM – were recently provided by Sherwood and colleagues in the model of C. elegans larval development.Citation27 A specialized uterine cell, the anchor cell, initiates uterine-vulval attachment by invading BM. Before invasion, the anchor cell forms multiple invadopodia. A single invadopodium then breaches BM and directs the formation of a single large invasive protrusion that crosses BM. Although it was generally assumed that invading cells dissolve BM, this model shows that proteases might be necessary only for the initial breaching, while the BM widens by sliding over the invagination. Whether a similar mechanism was conserved in sprouting of EC and extravasation of cancer cells remains unclear.
The identification of the molecular regulators of invadosomes in cultured cells could now be used to investigate the functions of these cellular structures in the broader context of tumor dissemination and tumor angiogenesis. Although recent discoveries are encouraging, the field of invadosomes in tumor biology is relatively new and many studies are still necessary for a comprehensive understanding of the role of these structures in tumor progression and metastasis. First evidence for invadosomes in human tissues are emerging, e.g. podosome rosettes in lung tumor vasculature,Citation10 but we need a systematic study of these sub-cellular structures in more tumor types, animal models and patient biopsies.
Lack of univocal and specific markers in invadosome identification is the main challenge for detecting the presence of invadosomes in tissues. Only a very accurate and solid imaging strategy can currently be used to identify these very small sub-cellular structures. Nevertheless, only studies on patient biopsies will finally cement the clinical and pathological relevance of invadosomes in tumor biology.
Disclosure of Potential Conflicts of Interest
No potential conflicts of interest were disclosed.
Acknowledgments
We thank Divya Bezwada and Jonas Kloepper for critical reading of the manuscript.
Funding
This work was supported by Associazione Italiana per la Ricerca sul Cancro (IG 14635) and MIUR-Fondo Investimenti per la Ricerca di Base (RBAP11BYNP Newton) to LP, and The Susan G. Komen Breast Cancer Foundation (grant PDF14301739) to GS.
References
- Beaty BT, Sharma VP, Bravo-Cordero JJ, Simpson MA, Eddy RJ, Koleske AJ, Condeelis J. beta1 integrin regulates Arg to promote invadopodial maturation and matrix degradation. Mol Biol Cell 2013; 24:1661–75, S1-11; http://dx.doi.org/10.1091/mbc.E12-12-0908
- Murphy DA, Courtneidge SA. The 'ins' and 'outs' of podosomes and invadopodia: characteristics, formation and function. Nat Rev Mol Cell Biol 2011; 12:413–26; PMID:21697900; http://dx.doi.org/10.1038/nrm3141
- Carman CV, Sage PT, Sciuto TE, de la Fuente MA, Geha RS, Ochs HD, Dvorak HF, Dvorak AM, Springer TA. Transcellular diapedesis is initiated by invasive podosomes. Immunity 2007; 26:784–97; PMID:17570692; http://dx.doi.org/10.1016/j.immuni.2007.04.015
- Friedl P, Alexander S. Cancer invasion and the microenvironment: plasticity and reciprocity. Cell 2011; 147:992–1009; PMID:22118458; http://dx.doi.org/10.1016/j.cell.2011.11.016
- Hallmann R, Horn N, Selg M, Wendler O, Pausch F, Sorokin LM. Expression and function of laminins in the embryonic and mature vasculature. Physiol Rev 2005; 85:979–1000; PMID:15987800; http://dx.doi.org/10.1152/physrev.00014.2004
- Yurchenco PD, Ruben GC. Basement membrane structure in situ: evidence for lateral associations in the type IV collagen network. JCell Biol 1987; 105:2559–68; PMID:3693393; http://dx.doi.org/10.1083/jcb.105.6.2559
- Glentis A, Gurchenkov V, Matic Vignjevic D. Assembly, heterogeneity, and breaching of the basement membranes. Cell Adh Migr 2014; 8:236–45; PMID:24727304; http://dx.doi.org/10.4161/cam.28733
- Kelley LC, Lohmer LL, Hagedorn EJ, Sherwood DR. Traversing the basement membrane in vivo: a diversity of strategies. J Cell Biol 2014; 204:291–302; PMID:24493586; http://dx.doi.org/10.1083/jcb.201311112
- Leong HS, Robertson AE, Stoletov K, Leith SJ, Chin CA, Chien AE, Hague MN, Ablack A, Carmine-Simmen K, McPherson VA, et al. Invadopodia are required for cancer cell extravasation and are a therapeutic target for metastasis. Cell Rep 2014; 8:1558–70; PMID:25176655; http://dx.doi.org/10.1016/j.celrep.2014.07.050
- Seano G, Chiaverina G, Gagliardi PA, di Blasio L, Puliafito A, Bouvard C, Sessa R, Tarone G, Sorokin L, Helley D, et al. Endothelial podosome rosettes regulate vascular branching in tumour angiogenesis. Nat Cell Biol 2014; 16:931–41, 1–8; http://dx.doi.org/10.1038/ncb3036
- Tarone G, Cirillo D, Giancotti FG, Comoglio PM, Marchisio PC. Rous sarcoma virus-transformed fibroblasts adhere primarily at discrete protrusions of the ventral membrane called podosomes. Exp Cell Res 1985; 159:141–57; PMID:2411576; http://dx.doi.org/10.1016/S0014-4827(85)80044-6
- Caligaris-Cappio F, Bergui L, Tesio L, Corbascio G, Tousco F, Marchisio PC. Cytoskeleton organization is aberrantly rearranged in the cells of B chronic lymphocytic leukemia and hairy cell leukemia. Blood 1986; 67:233–9; PMID:3484425
- Block MR, Badowski C, Millon-Fremillon A, Bouvard D, Bouin AP, Faurobert E, Gerber-Scokaert D, Planus E, Albiges-Rizo C. Podosome-type adhesions and focal adhesions, so alike yet so different. Eur J Cell Biol 2008; 87:491–506; PMID:18417250; http://dx.doi.org/10.1016/j.ejcb.2008.02.012
- Branch KM, Hoshino D, Weaver AM. Adhesion rings surround invadopodia and promote maturation. Biol Open 2012; 1:711–22; PMID:23213464; http://dx.doi.org/10.1242/bio.20121867
- Buccione R, Orth JD, McNiven MA. Foot and mouth: podosomes, invadopodia and circular dorsal ruffles. Nat Rev Mol Cell Biol 2004; 5:647–57; PMID:15366708; http://dx.doi.org/10.1038/nrm1436
- Moreau V, Tatin F, Varon C, Genot E. Actin can reorganize into podosomes in aortic endothelial cells, a process controlled by Cdc42 and RhoA. Mol Cell Biol 2003; 23:6809–22; PMID:12972601; http://dx.doi.org/10.1128/MCB.23.19.6809-6822.2003
- Osiak AE, Zenner G, Linder S. Subconfluent endothelial cells form podosomes downstream of cytokine and RhoGTPase signaling. Exp Cell Res 2005; 307:342–53; PMID:15894313; http://dx.doi.org/10.1016/j.yexcr.2005.03.035
- Juin A, Planus E, Guillemot F, Horakova P, Albiges-Rizo C, Genot E, Rosenbaum J, Moreau V, Saltel F. Extracellular matrix rigidity controls podosome induction in microvascular endothelial cells. Biol Cell 2013; 105:46–57; PMID:23106484; http://dx.doi.org/10.1111/boc.201200037
- Varon C, Tatin F, Moreau V, Van Obberghen-Schilling E, Fernandez-Sauze S, Reuzeau E, Kramer I, Genot E. Transforming growth factor beta induces rosettes of podosomes in primary aortic endothelial cells. Mol Cel Biol 2006; 26:3582–94; PMID:16611998; http://dx.doi.org/10.1128/MCB.26.9.3582-3594.2006
- Linder S, Nelson D, Weiss M, Aepfelbacher M. Wiskott-Aldrich syndrome protein regulates podosomes in primary human macrophages. Proc Natl Acad Sci U S A 1999; 96:9648–53; PMID:10449748; http://dx.doi.org/10.1073/pnas.96.17.9648
- Guegan F, Tatin F, Leste-Lasserre T, Drutel G, Genot E, Moreau V. p190B RhoGAP regulates endothelial-cell-associated proteolysis through MT1-MMP and MMP2. J Cell Sci 2008; 121:2054–61; PMID:18505793; http://dx.doi.org/10.1242/jcs.025817
- Wang J, Taba Y, Pang J, Yin G, Yan C, Berk BC. GIT1 mediates VEGF-induced podosome formation in endothelial cells: critical role for PLCgamma. Arterioscler Thromb Vasc Biol 2009; 29:202–8; PMID:19023093; http://dx.doi.org/10.1161/ATVBAHA.108.174391
- Rottiers P, Saltel F, Daubon T, Chaigne-Delalande B, Tridon V, Billottet C, Reuzeau E, Genot E. TGFbeta-induced endothelial podosomes mediate basement membrane collagen degradation in arterial vessels. J Cell Sci 2009; 122:4311–8; PMID:19887587; http://dx.doi.org/10.1242/jcs.057448
- Primo L, Seano G, Roca C, Maione F, Gagliardi PA, Sessa R, Martinelli M, Giraudo E, di Blasio L, Bussolino F. Increased expression of alpha6 integrin in endothelial cells unveils a proangiogenic role for basement membrane. Cancer Res 2010; 70:5759–69; PMID:20570893; http://dx.doi.org/10.1158/0008-5472.CAN-10-0507
- Gligorijevic B, Wyckoff J, Yamaguchi H, Wang Y, Roussos ET, Condeelis J. N-WASP-mediated invadopodium formation is involved in intravasation and lung metastasis of mammary tumors. J Cell Sci 2012; 125:724–34; PMID:22389406; http://dx.doi.org/10.1242/jcs.092726
- Beaty BT, Wang Y, Bravo-Cordero JJ, Sharma VP, Miskolci V, Hodgson L, Condeelis J. Talin regulates moesin-NHE-1 recruitment to invadopodia and promotes mammary tumor metastasis. J Cell Biol 2014; 205:737–51; PMID:24891603; http://dx.doi.org/10.1083/jcb.201312046
- Hagedorn EJ, Yashiro H, Ziel JW, Ihara S, Wang Z, Sherwood DR. Integrin acts upstream of netrin signaling to regulate formation of the anchor cell's invasive membrane in C. elegans. Dev Cell 2009; 17:187–98; PMID:19686680; http://dx.doi.org/10.1016/j.devcel.2009.06.006