Abstract
The role of the G1-phase Cyclin D-CDK 4/6 regulatory module in linking germline stem cell (GSC) proliferation to nutrition is evolutionarily variable. In invertebrate Drosophila and C. elegans GSC models, G1 is nearly absent and Cyclin E is expressed throughout the cell cycle, whereas vertebrate spermatogonial stem cells have a distinct G1 and Cyclin D1 plays an important role in GSC renewal. In the invertebrate, chordate, Oikopleura, where germline nuclei proliferate asynchronously in a syncytium, we show a distinct G1-phase in which 2 Cyclin D variants are co-expressed. Cyclin Dd, present in both somatic endocycling cells and the germline, localized to germline nuclei during G1 before declining at G1/S. Cyclin Db, restricted to the germline, remained cytoplasmic, co-localizing in foci with the Cyclin-dependent Kinase Inhibitor, CKIa. These foci showed a preferential spatial distribution adjacent to syncytial germline nuclei at G1/S. During nutrient-restricted growth arrest, upregulated CKIa accumulated in arrested somatic endoreduplicative nuclei but did not do so in germline nuclei. In the latter context, Cyclin Dd levels gradually decreased. In contrast, the Cyclin Dbβ splice variant, lacking the Rb-interaction domain and phosphodegron, was specifically upregulated and the number of cytoplasmic foci containing this variant increased. This upregulation was dependent on stress response MAPK p38 signaling. We conclude that under favorable conditions, Cyclin Dbβ-CDK6 sequesters CKIa in the cytoplasm to cooperate with Cyclin Dd-CDK6 in promoting germline nuclear proliferation. Under nutrient-restriction, this sequestration function is enhanced to permit continued, though reduced, cycling of the germline during somatic growth arrest.
Abbreviations
GA | = | Growth Arrest |
GSC | = | Germline Stem Cell |
TOR | = | Target Of Rapamycin |
MAPK | = | Mitogen Activated Protein Kinase |
ERK | = | Extracellular signal-regulated kinases |
G-phase | = | Gap phase |
S-phase | = | DNA Synthesis phase |
M-phase | = | Mitotic phase |
CDK | = | Cyclin-Dependent Kinase |
CKI | = | CDK inhibitor |
Rb | = | Retinoblastoma protein |
PCNA | = | Proliferating cell nuclear antigen |
NLS | = | Nuclear Localization Sequence |
CAK | = | CDK Activating Kinase |
MSK | = | Mitogen and Stress activating Kinase |
CREB | = | CRE Binding protein |
SCF complex | = | Skp, Cullin, F-box containing complex |
CRM | = | Chromosome Region Maintenance |
GFP | = | Green Fluorescent Protein |
IdU | = | 5-Iodo-2′-deoxyuridine. |
Introduction
Stem cells form the basis of development, repair and regeneration during the lifetime of an organism. Accordingly, the cell cycle and gene expression are regulated by a coordinated network of cell signaling mechanisms in both somatic and germline stem cells (GSCs). Signals from stromal cells in the surrounding microenvironment (or niche) are often involved in controlling the renewal and differentiation of stem cells.Citation1 In addition, stem cells are also influenced by external stimuli such as nutrients, hormones or physical injuries.Citation1-3 For instance, in C. elegans and Drosophila, nutritional signaling modulates the proliferation and differentiation of GSCs.Citation4-6 Nutritional signaling also activates adult stem cell proliferation by acting on the G1-phase regulatory complex, Cyclin D-CDK4/6.Citation7
In this study, we used a semelparous, invertebrate, chordate, Oikopleura dioica, to explore the role of D cyclins in germline proliferation under nutrient-dependent growth arrest (GA).Citation8 O. dioica, an abundant component of marine zooplankton communities, is a member of the closest extant sister group to vertebrates.Citation9 During the first half of its short, 6-day, lifecycle, germline nuclei in mitotic cycles proliferate asynchronously in a syncytium.Citation10-12 The germline is present as a single, giant, multi-nucleate cell, surrounded by a single layer of follicle cells enclosed by a monolayer epithelium. During the day 3 to 4 transition, ovarian syncytial germline nuclei give rise to equivalent numbers (thousands) of nuclei in prophase I of meiosis and those that enter endocycles as nurse nuclei. Each nuclear population is distributed homogenously throughout the common “coenocyst” cytoplasm. Unlike the iteroparous model organisms, C. elegans and Drosophila, meiotic entry occurs only during this short time window in O. dioica, and the GSC population is not subsequently maintained.
In G1, cell cycle progression is linked to integration of extracellular stimuli via nutrition-dependent Insulin and Target Of Rapamycin (TOR) signaling.Citation13 Mitogenic or stress signaling mediated by MAPK ERK1/2Citation14,15 and MAPK p38Citation16 also regulate the G1/S transition by activating D-type cyclin expression in mammalian cells. Classically, Cyclin D-CDK4/6 was thought to processively hypo-phosphorylate the retinoblastoma protein (Rb) prior to Rb hyper-phosphorylation by Cyclin E-CDK2. This stimulates cell cycle progression by releasing the E2F transcription factor, a regulator of the G1/S transition transcriptional program. Recently it is proposed that Cyclin D-CDK4/6 catalyzes mono-phosphorylation of Rb to activate early G1 functions of multiple, mono-phospho Rb isoforms and to prevent cell cycle exit to G0, regulated in part by un-phosphorylated Rb.Citation17 Importantly, Cyclin D-CDK4/6 complexes can activate Cyclin E-CDK2 indirectly by sequestering G1-phase p21/p27-type Cyclin-dependent Kinase Inhibitors (CKIs), which inhibit Cyclin E-CDK2 activity.
Recently, we demonstrated that a reduction in TOR activity during GA of O. dioica, resulted in immediate Cyclin-Dependent Kinase Inhibitor a (CKIa) upregulation and somatic cell cycle arrest.Citation8 Response to GA in the mitotically proliferating germline nuclei was different. Cell cycle arrest was not immediate and instead, occurred gradually over a period of several days. This suggests modulation of the cell cycle arrest mechanism in the O. dioica germline as compared to somatic cells. In invertebrate Drosophila and C. elegans GSCs, G1 phase is nearly absent.Citation18-21 In this context, cell cycle profiles of Cyclin E-CDK activity are altered with Cyclin E present throughout the GSC cell cycle.Citation18,21 In these invertebrates, proliferative renewal of GSCs is nonetheless still responsive to insulin and TOR signalingCitation2,22 but these nutrient-dependent controls operate during G2, as opposed to G1. In contrast, mouse spermatogonial stem cells proliferate with a distinct G1 phase and Cyclin D plays a major role in the renewal of stem cells.Citation23 In comparison to other invertebrates, Cyclin D paralogs and their splice variants have been considerably amplified in O. dioica, with several exhibiting expression in the ovary.Citation24 Therefore, we wished to determine if the O. dioica germline exhibits a G1 phase and whether some of these Cyclin D paralogs and their splice variants have roles in regulating germline nuclear proliferation and in overcoming immediate CKIa-mediated germline cell cycle arrest during nutrient-restricted GA.
The activity of CKI in GSCs is reduced to promote proliferation in Drosophila, C. elegans and mouse. C.elegans CKI-1 is required to maintain germline quiescence of starved L1 arrested larvaeCitation25,26 and RNA depletion of CKI-1 induces hyperproliferation of GSC during dauer formation.Citation25 Mutants of Cul-2 (a component of the SCF-degradation complex) allow CKI-1 protein levels to accumulate, resulting in arrest of GSC proliferation.Citation27 Suppression of C. elegans CKI-2 activity, through the RNA-binding protein FBF2, which inhibits translation of CKI-2 mRNA, is also critical for GSC proliferation.Citation28 Similarly, in Drosophila, the CKI, Dacapo, accumulates and induces cell cycle arrest in GSCs during starvation. Under favorable conditions, nutrition-dependent expression of dicer-1 negatively regulates Dacapo levels.Citation29 These findings indicate that suppressing CKI activity through nutrition-dependent signaling, either by reducing CKI mRNA levels or CKI protein stability, positively regulates GSC proliferation.
D-type Cyclins have been reported in several studies to mediate sequestration of p21 and p27 either in the cytoplasm or nucleus of mammalian cells under nutrient-deprived conditions.Citation30-34 Furthermore, mutations in the Cyclin D1, CDK activating residue (T156), or phospho degradation residue (T286), are dominant-negative toward cell cycle progression. These mutants sequester CDK4 in the cytoplasm in an inactive complex in mammalian fibroblasts.Citation35 Interestingly Cyclin D2SV, a mouse Cyclin D2 splice variant, lacks the Cyclin-dependent kinase activating residue (T156), and degradation residue (T286).Citation36 It is expressed in the mouse ovaryCitation37 and sequesters CDK4 and p27 in the cytoplasm, possibly targeting them for ubiquitin-dependent protein degradation.Citation36,38,39 Human Cyclin D2SV is overexpressed in some primary human brain tumors.Citation37 The D2SV splice variant is responsive to nutrient-dependent signaling and is upregulated during nutrient limitation.Citation40 Among the amplified O. dioica Cyclin D paralogs there are intriguing splice variants of the Db isoform where the Rb-binding motif is absent (variants α and β) and/or the C-terminal putative phospho degron is deleted (variants β and δ).Citation24 Thus the Cyclin Db isoforms with altered stability or impaired Rb-interaction capacity might be expected to play distinct and possibly opposing roles, in the regulation of cell cycle progression.
Here, we show that O. dioica mitotic germline nuclei proliferate asynchronously in the syncytium and have a distinct G1-phase, unlike other model invertebrates such as C. elegans and Drosophila. In mitotic germ stem nuclei, G1 is regulated by Cyclin Dd during their proliferative phase. Nutrition-dependant TOR signaling controls Cyclin Dd expression as previously reported in somatic endocycling cells.Citation8 Reduced TOR signaling in the germline of growth-arrested O. dioica activates stress-induced MAPK p38-MSK1 signaling, and the Cyclin Dbβ splice variant, lacking the Rb binding domain, is transcriptionally activated. We further demonstrate the existence of Cyclin Dbβ foci in the germline cytoplasm which colocalize with CKI during somatic GA. We propose that activation of MAPKp38 signaling under reduced TOR activity induces Cyclin Dbβ, which in turn forms complexes to sequester upregulated CKIa in mitotic germline cytoplasm, to permit continued proliferation of germline nuclei during the initial period of nutrient-restricted somatic growth arrest.
Results
Asynchronously proliferating O. dioica germ nuclei have a distinct G1-phase
Cyclin D regulates self-renewal in mammalian adult stem cellsCitation41 and spermatogonial germ stem cells,Citation23 but is not essential for GSC proliferation in Drosophila and C. elegans.Citation18,19,21 The O. dioica Cyclin D variants, Da, Db, Dc and Dd are encoded by 4 different genes.Citation24 Previously, we have shown that O. dioica Cyclin Dd regulates G1-phase in mitotically proliferating cells during early development and in post-metamorphic somatic endocycling cells.Citation24 Comparative analysis (SMART, http://smart.embl-heidelberg.de, and ELM, http://elm.eu.org) of human, mouse and O. dioica Cyclin D variants (all accession numbers given in Table S1) revealed that unlike mammalian Cyclin Ds, O. dioica Db/c/d variants retain the N-terminal, but lack the C-terminal, cyclin box (). The exception is the Cyclin Da variant that retains a divergent C-terminal cyclin box.Citation24 O. dioica Cyclin Dd differs from Cyclin Db splice variants (): it contains 2 Rb binding motifs (LxCxE), versus one or none; it has 3 predicted nuclear localization sequences (NLS) vs. none, as well as 2 nuclear exporter CRM binding motifs, versus one. To determine the cell cycle profile of Cyclin Dd in the germline, pre-meiotic animals were immunostained for Cyclin Dd, IdU (S-phase) and H3pS28(M-phase). Cyclin Dd levels peaked during G1, decreased upon entry into S-phase and were undetectable during M-phase (). Thus, Cyclin Dd oscillations are consistent with a role in the regulation of the G1 to S transition during proliferation of germline nuclei, similar to its role in somatic cells.Citation24
Figure 1. Functional domains in human and Oikopleura dioica Cyclin D splice variants. (A) Human Cyclin D1 and mouse Cyclin D2 and their splice variants, D1b and D2SV, respectively. (B) O. dioica Cyclin Db splice variants cover all presence/absence combinations (+/+, −/+, +/−, −/−) of the Retinoblastoma(Rb)-binding and phosphodegron domains, respectively. (C) O. dioica Cyclin Dd. NLS, Nuclear Localization Sequence; CRM, (Chromosomal maintenance 1 or Exportin 1) interaction motif; LxCxE Rb-binding motif. All motifs were predicted by SMARTCitation65 and ELM.Citation66 Cyclin-dependent Kinase 6 (CDK6) and CDK inhibitor (CKI) binding regions on O. dioica Cyclin D splice variants were based on Zwicker et al.Citation44 Unique C-terminal sequences arising in splice variants are indicated by different stippling patterns. BLAST and secondary structure assessments (PsiPred) of the C-termini of O. dioica Cyclin D variants show residual signatures of a second Cyclin box with low confidence scores.Citation24 Hs, Human; Mm, Mouse; Od, O. dioica.
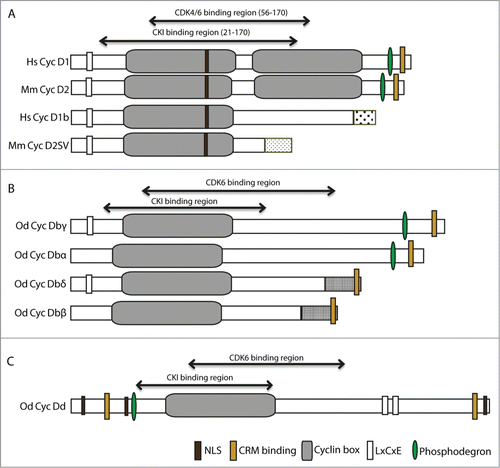
Figure 2. Asynchronous proliferation of germ nuclei with a distinct G1-phase. (A) Immunostaining of asynchronous mitotic germ nuclei show different levels of Cyclin Dd expression (n = 10 animals). Actin network within the single-cell coenocyst is stained in green. Cyclin Dd expression peaks in G1 (arrows), persisted at reduced levels in early S (arrowheads; Campsteijn et al.Citation24) and was absent thereafter. Cyclin Dd expression was not seen in nuclei expressing the mitotic marker H3pS28. (B) Larger overview of germline nuclei indicating proportions in G- (no staining), S- (IdU incorporation) and M-phase (H3pS28 staining) prior to meiotic entry. (C) Proportion of germ nuclei in each of the proliferative cell cycle phases was assessed (n = 10 animals) by immunostaining in combination for markers: Cyclin Dd (G1), short IdU pulse (S), and H3pS28 (M). Nuclei that were negative for all of these markers were in G2. Error bar indicates standard error. Scale bars = 10 µm.
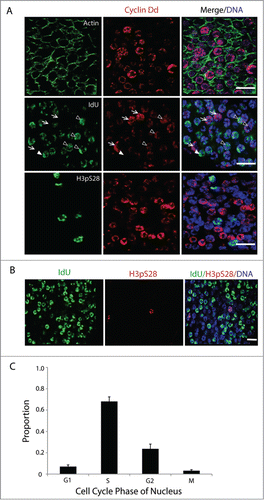
In C. elegans, germ nuclei in the mitotic zone proliferate asynchronously even though they are present in a syncytium that shares a common cytoplasm within an enclosed lumen.Citation42 The mitotic germ nuclei of O. dioica are also present in a syncytiumCitation10,12, and similar to C. elegans, proliferate asynchronously during the pre-meiotic phase (). Based on Cyclin Dd (G1 marker), IdU (S marker) and H3pS28 (M marker) staining, calculated indexes () revealed that 7% of germline nuclei were in G1 (cyclin Dd positive/IdU negative), 65% were in S (IdU positive), 3% were in M (H3pS28 positive), and the remaining 25% were negative for all markers (G2 or early G1). This shows that asynchronously proliferating O. dioica mitotic germline nuclei have a distinct, regulated, G1-phase as seen in mouse spermatogonial stem cells, and contrasting observations in Drosophila and C. elegans.
Cyclin Dbβ/δ variants occur in cytoplasmic foci adjacent to early S-phase germ nuclei
Alternative splicing of O. dioica Cyclin Db produces 4 variants in which all presence/absence combinations of the Rb-binding motif/phosphodegron are represented: −/+ Cyclin Dbα; −/− Dbβ; +/+ Dbγ; and +/− Dbδ (). Lack of the exon expressing the putative phosphodegron produces short Cyclin Dbβ and δ splice variants, which have an altered, unique sequence of 34 amino acids at the C-terminus (Fig. S1). Despite Cyclin Dbδ and β having a distinct C-terminal sequence, the C-terminal CRM1 consensus is conserved, unlike human splice variants Cyclin D1b and D2SV, where it is absent. Notably, all Cyclin Db splice variants lack a predicted NLS. When the mitotic germline was immunostained with antibodies specific to the C-terminal region of Cyclin Dbβ/δ, cytoplasmic foci were observed adjacent to nuclei (). These isoforms were never observed within nuclei at any stage of the cell cycle. Interestingly, the Cyclin Dbβ/δ foci were rarely found adjacent to nuclei in G or M-phase, but were commonly found adjacent to nuclei in S-phase (). Thus, there exists a negative correlation between the occurrence of Cyclin Dbβ/δ cytoplasmic foci and G-phase germline nuclei or M-phase chromatin. More precisely, we observed that a high number of early S-phase nuclei have cytoplasmic Cyclin Dbβ/δ foci adjacent to them as compared to mid or late S-phase, or G2 nuclei (). It was also apparent that approximately 10% of the germline nuclei were associated with an adjacent Cyclin Dbβ/δ foci (). It is known that the human and mouse truncated Cyclin D2 splice variant, D2SV, with a unique C terminus is also observed in cytoplasmic foci.Citation38 Our results indicate a possible cytoplasmic role of Cyclin Dbβ/δ variants during germline proliferation in O. dioica, with preferred localizations adjacent to syncytial nuclei during S-phase entry.
Figure 3. Cyclin Dbβ/δ cytoplasmic foci and the G1/S transition in germline nuclei. (A) Immunostaining of mitotic germline nuclei with antibodies specific to C-terminal regions of Cyclin Db splice variants β/δ. Cyclin Dbβ/δ were present as foci in the syncytial germline cytoplasm adjacent to nuclei. These foci were not observed adjacent to nuclei in M-phase (H3pS28 staining) and were also not preferentially associated with nuclei staining most intensely for Cyclin Dd (n = 10 animals). Inset depicts magnification of a cytoplasmic Cyclin Dbβ/δ focus adjacent to a nucleus. (B) Cytoplasmic foci of Cyclin Dbβ/δ were adjacent to nuclei with low amounts of IdU incorporation (arrows) but were not adjacent to nuclei with no IdU incorporation (G-phase; empty arrowheads) or nuclei with large amounts of IdU incorporation (filled arrowheads). (C) Cyclin Dbβ/δ foci were selected (n = 10 animals; 1000 foci) and the cell cycle phase of the closest nucleus was assessed based on extent of IdU incorporation and H3pS28 staining. (C') Reciprocally, nuclei in G1 (no incorporation of IdU, Cyclin Dd staining), G1/S (weak incorporation of IdU, weak Cyclin Dd staining) S or G2 (increased IdU staining, no Cyclin Dd staining) or M (H3pS28 staining) were selected (n = 10 animals; 900 nuclei) and assessed for the presence or absence of adjacent Cyclin Dbβ/δ foci. Error bars indicate standard errors. Scale bars = 10 µm.
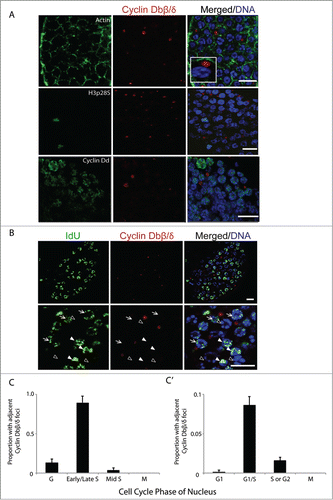
Interaction of Oikopleura dioica CDK6 with Cyclins Db, Dd and CKIa
Human Cyclin D1b and mouse D2SV splice variants, despite being truncated at the C-terminus, bind CDK4 efficiently but are deficient in phosphorylating Rb.Citation36,39,43 Binding sites of CDK6 and CKIa on O. dioica Cyclin Db splice variants were predicted from the known binding sites on human Cyclin D1Citation44 and were present on Cyclin Dbβ and δ variants (Fig. 1B and Fig. S1). Given the presence of these putative binding sites, we next investigated whether there was a physical interaction of CDK6 (O. dioica does not have CDK4Citation24), with Cyclin Dd or Cyclin Db splice variants, and CKIa. Western blots of the immunoprecipitated fractions revealed that CDK6 interacts with Cyclin Dd, Cyclin Db splice variants (α,γ,β,δ) and CKIa (). The interaction of CDK6 with Cyclin Dbβ and δ splice variants suggests that Cyclin Db β/δ contain the minimum region required for binding CDK6. Residues K112 and T156 found on human Cyclin D1 mediate binding of the Cyclin D-CDK4/6 complexes with CDK activating Kinase (CAK) which in turn catalytically activates the Cyclin D-CDK4/6 complex. Mutations at these Cyclin D1 residues inhibit activation by CAK but permit sequestration of both CDK4 and CKI, blocking their activities.Citation35,45 Residues corresponding to human Cyclin D1, K112 and T154, are conserved in O. dioica Cyclin Db splice variants including Dbδ and β (Fig. S1). Whereas the Cyclin Dbδ splice variant contains an Rb-interaction motif, Cyclin Dbβ lacks this motif. Combined with what appears to be a strictly cytoplasmic localization, this suggests that a function of Cyclin Dbβ may be the cytoplasmic sequestration of CDK6 and CKI in the regulation of the syncytial germ nuclei in response to nutritive conditions.
Nutrient-restricted growth arrest gradually reduces cyclin Dd levels in the germline
Nutrient-dependent TOR signaling regulates GSC proliferation and differentiation in Drosophila and C. elegans.Citation2 We have recently found that inhibition of TOR signaling prevents translation of cyclin Dd mRNA, thus causing cell cycle arrest in somatic endocycling cells during GA.Citation8 However, the cell cycle of germ nuclei did not arrest immediately, instead exhibiting a more gradual slowing as somatic GA persisted. Therefore, we examined the effects of nutrient-restricted GA on Cyclin Dd expression in regulating germ nuclei proliferation compared to levels observed under standard culture conditions. Relative levels of cyclin Dd transcripts did not change during GA (Subramaniam et al.Citation8 and ), but immunofluorescent staining in GA animals (Day 3, Day 6 and Day 12) showed a gradual reduction in Cyclin Dd levels and IdU incorporation (S-phase entry) in germ nuclei (). Thus, nutrient restriction resulting in GA also reduced Cyclin Dd levels in the germline, though at a slower rate than in O. dioica somatic cells.
Figure 5. Cyclin Dd levels in the germline are gradually reduced when growth arrest occurs in response to nutrient limitation. Oikopleura dioica were pulsed with the S-phase marker IdU for 1 h at day 3 when cultured under standard conditions or for 1 h at days 3, 6 and 12 when cultured under dense conditions. IdU incorporation and Cyclin Dd immunostaining were then assessed. Levels of Cyclin Dd during the early period of growth arrest remained similar to that observed under standard conditions but then gradually decreased as growth arrest persisted (n = 8 animals). Scale bars = 5 μm.
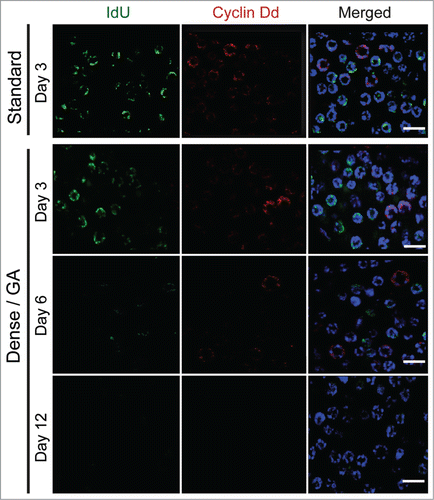
Figure 6 (See previous page). Increased cyclin Dbβ levels during TOR inhibition require MAPK-p38 signaling. (A) Cyclin Db expression increased (*P < 0.05) and persisted at higher levels in O. dioica cultured under dense conditions where growth-arrest occurs. Under these same conditions, no significant change in expression of cyclin Da, cyclin Dc, cyclin Dd was observed. (B) The observed increase in cyclin Db expression during growth arrest was restricted to the β splice variant, as levels of the α, γ and δ splice variants were unaffected. (C) When animals were cultured under standard conditions but in the presence of the TOR inhibitor CCI-779 for 24 h, cyclin Db transcripts were upregulated (*P < 0.05) whereas cyclin Da, Dc, and Dd transcripts were not. (D) Similar to the results in (B) under growth arrest, at dense culture conditions, inhibition of TOR signaling under standard culture conditions resulted in upregulation of the Cyclin Dbβ splice variant (*P < 0.05), whereas levels of the α, γ and δ splice variants were unaffected. (E) When animals were cultured under standard conditions, but in the presence of the MAPK p38 inhibitor, SB203580, for 24 h at day 3, cyclin Db transcript levels were reduced (*P < 0.05), whereas cyclin Da, cyclin Dc, cyclin Dd transcript levels were not. Expression levels are relative to those observed in day 3 animals cultured under standard conditions (with addition of DMSO in controls when chemical inhibitors were used). Error bars indicate standard errors. (F) Western blots showed that Cyclin Dbα/γ levels were similar under standard culture or growth arrested (GA) conditions, whereas Cyclin Dbβ/δ levels increased under the latter conditions. Levels of all Cyclin Db splice variants decreased in the presence of MAPK p38 inhibitor SB203580.
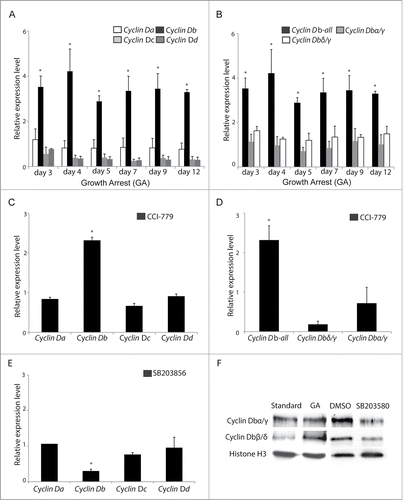
cyclin Dbβ expression is stimulated by MAPK p38 signaling under TOR inhibition
Human cyclin D2 mRNA and protein levels increase upon starvation in primary fibroblasts.Citation31 In 2 other mammalian cell lines, density-dependent growth arrest or starvation decreased cyclin D1 expression and increased cyclin D2 expression.Citation46 To follow expression of O. dioica D-type cyclin genes during GA, transcripts of cyclin Da, cyclin Db, cyclin Dc and cyclin Dd were analyzed at days 3, 4, 5, 7, 9 and 12 of sustained nutrient deprivation. Interestingly, compared to animals cultured under standard conditions, relative transcript levels of cyclin Da, cyclin Dc and cyclin Dd were not affected but there was a significant increase in cyclin Db transcripts (primers recognize all cyclin Db forms α, β, γ and δ) (). Further assessment revealed that the relative expression of cyclin Db splice variants α, γ and δ were unchanged (2 primers sets recognize cyclin Db α and γ, or Db γ and δ, respectively) (). Thus, the general increase in overall cyclin Db expression during GA was due to cyclin Dbβ transcripts alone. Similar results were obtained when animals were cultured in the presence of the TOR inhibitor, CCI-779, under standard culture conditions ().
During GA in O. dioica, TOR signaling was inhibited ( in Subramaniam et alCitation8) whereas survival MAPK p38-MSK1 signaling was activated ( in Subramaniam et alCitation8). Similar activation of p38 was observed under direct TOR inhibition alone ( in Subramaniam et alCitation8). In mammalian fibroblasts, MAPK p38 acts as a mitogenic signal and regulates cyclin D1 transcription.Citation16 Mitogen and Stress activating Kinase 1 (MSK1), a MAPK p38 downstream effector, regulates levels of D-type Cyclins by activating transcription factor CREB (CRE binding protein).Citation47-49 Interestingly, a CREB-binding consensus sequence was identified in the cyclin Db promoter (Fig. S2). To determine the effect of MAPK p38-MSK1 on transcription of O. dioica D cyclins, animals were cultured in the presence of MAPK p38 inhibitor SB203580 for 24 h and the expression of the D-type cyclins was examined. Inhibition of MAPK p38 decreased expression of cyclin Db but did not affect the expression of other D-type cyclins (). Western blots revealed that levels of Cyclin Dbβ/δ increased during GA, when there is reduced TOR signaling, whereas the levels of these splice variants decreased when p38 was inhibited (). This suggests that during GA/TOR inhibition, activated MAPK p38 signaling increases the levels of Cyclin Dbβ.
Cyclin Dbβ and CKIa foci colocalize in mitotic germline cytoplasm and such foci increase in number during growth arrest
Increased levels of Cyclin D-CDK4 complexes can regulate the G1/S transition by sequestering CDK inhibitors (CKI) that would otherwise inhibit the catalytic activity of Cyclin E-CDK2.Citation33,50 Under density-dependent cell cycle arrest in human cells, Cyclin D2 is induced and forms the Cyclin D2-CDK4-p27 complex, replacing Cyclin D1 and sequestering p27.Citation51 Furthermore, cytoplasmic foci of the mouse Cyclin D2 splice variant, D2SV, sequester positive regulators of cell cycle progression and act as a growth suppressor.Citation39 Regarding GSCs, it is known in C. elegans, Drosophila and mouse spermatogonial stem cells that suppression of CKI activity is critical for self-renewal of GSC.Citation28,29,52 Intringuingly, our recent studies showed that under conditions of GA or direct inhibition of TOR signaling, upregulated CKIa did not immediately arrest the proliferation of germline nuclei.Citation8 Therefore, we wished to explore the interaction of O. dioica Cyclin Ds and CKIa in the pre-meiotic germline.
Immunostaining revealed that CKIa formed foci exclusively in cytoplasm of the mitotic germline and that these CKIa foci colocalized with Cyclin Dbβ/δ foci (). This was also observed when GFP-fused Cyclin Dbβ or CKIa capped mRNA constructs were microinjected into gonads (). Finally, GFP-fused CKIa colocalized with endogenous, immunostained Cyclin Dbβ/δ foci (), confirming their colocalization in cytoplasmic foci. We also observed, the number of such foci in the germline cytoplasm significantly increased upon entry into GA (). Taken together, these findings suggest that upregulated Cyclin Dbβ sequesters CKIa in the pre-meiotic germline, permitting GSC cell cycle progression to continue during the intial period of nutrient-restricted somatic growth arrest.
Figure 7. Cyclin Dbβ/δ foci colocalize with CKIa in the syncytial germline cytoplasm and the number of these foci increase during growth arrest. (A) Antibody staining revealed that Cyclin Dbβ/δ foci co-localized with CKIa (n = 8 animals). Upper panels show an overview of colocalisation in the gonad; lower panels show colocalization at higher magnification. (B) Cytoplasmic foci were also observed when capped mRNAs generated for GFP-fused Cyclin Dbβ or CKIa constructs were microinjected into the gonads of day 3 animals (n = 8 animals). (C) GFP-fused CKIa colocalized with endogenous Cyclin Dbβ foci in germline cytoplasm (n = 8 animals). (D) The number of colocalized Cyclin Db-CKIa foci relative to the number of nuclei increased (*P < 0.05) in the cytoplasm of mitotic germ line during growth arrest (GA). Error bars indicate standard errors. Scale bars = 10 µm.
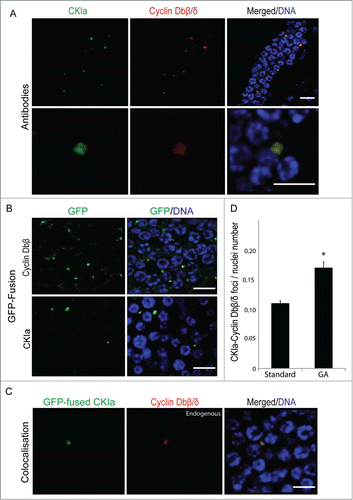
Discussion
The activity of Cyclin D-CDK4/6 complexes is linked to downstream activation of Cyclin E-CDK2 complexes through 2 major mechanisms: nuclear phosphorylation of the Rb protein and sequestration of CKIs that otherwise, inhibit Cyclin E-CDK2 activity. There is evolutionary plasticity in the relative extent to which these linking mechanisms are employed, and in the details that control the interaction, localization and activity of Cyclin D-CDK4/6-(CKI) complexes. In GSCs, there is also evolutionary diversity in the extent to which a G1 regulatory phase is present. Finally, whereas those invertebrates that have been studied possess a single Cyclin D variant, vertebrates which exhibit the greatest degree of cell-type diversity, have 3 Cyclin Ds (D1-3) along with additional splice-variants of these. O. dioica, a member of the urochordate subphylum placed phylogenetically near the invertebrate-vertebrate transition, also has a diversified Cyclin D complement of 4 variants (Da-d) and associated splice variants.Citation24 We have shown that 2 O. dioica Cyclin D variants exhibit distinct temporal-spatial localizations during germline nuclear proliferation. They also respond differently to nutrient-limited conditions that induce somatic cell cycle and GA but permit continued, though reduced, cycling in the germline.
Cyclin Dd and Dbβ cooperate to promote pre-meiotic germline nuclear proliferation
In contrast to regulation of cycling of invertebrate C. elegans and Drosophila pre-meiotic GSCs, where a G1 is nearly absent and Cyclin E is expressed throughout the cycle,Citation18,21 we show that O. dioica, pre-meiotic, germline nuclei have a G1-phase. During the period of syncytial germline nuclear proliferation, both the Cyclin Db and Dd variants are expressed. However, whereas Cyclin Dd located to germline nuclei, Cyclin Dbβ/δ variants remained strictly cytoplasmic. These latter variants co-localized with odCKIa in cytoplasmic foci. The partnership of Cyclin D-CDK4/6 complexes with CKIs and their respective roles in controlling nuclear localization are evolutionarily variable. In mammalian cells, phosphorylation at tyrosine 88 (Y88)Citation53 of the CKI, p27, allows it to act as an assembly factor to form active nuclear Cyclin D-CDK4/6 complexes.Citation54 There is evidence from 2-hybrid data that C. elegans CKI-1 also binds Cyclin D.Citation25,26 In contrast, the Drosophila CKI, Dacapo, does not bind Cyclin D-CDK4 complexes.Citation55 O. dioica CKIa and Cyclin Dd both have NLS whereas odCDK6 and Cyclin Db do not. The Cyclin Dd variant contains both Rb-binding and phosophodegron motifs whereas the Cyclin Dbβ splice-variant contains neither regulatory module. This could suggest that the Cyclin Dbβ splice variant might have a stabilized dominant negative function with respect to cell cycle progression through combination with CDK6 in complexes unable to phosphorylate Rb. However, our data show that the Dbβ splice variant is present in a context where nuclei are actively cycling, that it is found in cytoplasmic foci where CKIa is colocalized, and that in the syncytium, these foci preferentially localize adjacent to nuclei that are entering S-phase. This leads to an alternative explanation that in the germline syncytium, Cyclin Dd and Dbβ variants cooperate in promoting cell cycle progression: Cyclin Dd through translocation with CDK6 into the nucleus to phosphorylate Rb during G1, whereas Cyclin Dbβ acts to locally sequester CKIa in the syncytial cytoplasm, thus relieving inhibition of Cyclin E-CDK2 activity. Such an interpretation is further supported by the differential response of somatic endocycling cells (Cyclin Dd present; Cyclin Dbβ absent) and the germline (Cyclin Dd present; Cyclin Dbβ present) to nutrient-restricted conditions.
During growth arrest, Cyclin Dbβ is upregulated in the germline whereas Cyclin Dd is not
Studies of GSC in C. elegans,Citation56 DrosophilaCitation29 and mouse spermatogonial stem cellsCitation52,57 have shown that repression of CKI activity is critical for proliferation of GSCs. This is supported by the observation in C. elegans Cul-2 mutants (component of SCF-degradation complex) that CKI-1 levels accumulate and cause cell cycle arrest at the G2-M transition in GSC.Citation27 It is also known that under nutrient-limited conditions, CKI levels increase in GSC of C. elegans,Citation58 and Drosophila,Citation29 resulting in cell cycle arrest. This can be reversed during growth-arrested dauer formation in C. elegans through depletion of CKI-1 by RNA-mediated interference, causing hyperproliferation of GSC.Citation25 Similarly, in Drosophila, nutrient limitation leads to lower expression of dicer-1 and the accumulation of Dacapo,Citation29 causing reduced cycling of GSC.
In O. dioica somatic cells, nutrient-limitation that causes growth-arrest is accompanied by pronounced nuclear accumulation of CKIa.Citation8 However, pre-meiotic germline nuclei continue to cycle under such conditions and nuclear accumulation of CKIa is not observed. The Cyclin Dd variant is expressed in both somatic tisssues and the germline whereas Cyclin Db expression is restricted to the germline. During GA, mRNA levels of cyclin Dd remained stable, though Cyclin Dd protein levels gradually decreased over time. On the other hand, nutrient-limitation specifically stimulated an increase in the level of cyclin Dbβ mRNA. These same effects were observed when TOR signaling was directly inhibited by the rapamycin analog CCI-779. Nutrient-limited GA also resulted in a significant increase in the number of Cyclin Dbβ foci found in the germline syncytial cytoplasm. This specific upregulation of Cyclin Dbβ levels in the germline could permit increased cytoplasmic sequestration of CKIa thus reducing the rate of germline vs. somatic cell cycle arrest.
Altered Cyclin D variant expression in response to MAPK p38 signaling
It is known that there is altered expression of mammalian Cyclin D variants (including splice variants) under conditions of stress such as nutrient limitation. Levels of Cyclin D2 and D2SV increase during cell cycle arrest or under serum-starved culture conditions in mammalian cellsCitation31,40 and like Cyclin Dbβ, D2SV is observed to form cytoplasmic foci. A further similarity to Cyclin Dbβ is that both the mammalian Cyclin D2SV and D1b splice variants have an altered C-terminus and lack the phosphodegron motif. Under conditions of nutrient restriction, increased levels of mouse cytoplasmic D2SV splice variantsCitation40 exert a suppressive role on cell cycle progression by sequestering key cell cycle regulators including cyclin D2, CDK4, Cyclin B and possibly p27.Citation36,38,39
Previously we have shown that the survival MAPK p38-MSK-1 signaling pathway is activated in the O. dioica mitotic germline during nutrient-dependent GA or when TOR signaling is inhibited.Citation8 Common responses to stress-induced p38 activation are cell cycle arrest or apoptosis.Citation59,60 Cell cycle arrest achieved through p38 signaling acts through inhibition of cyclin D transcription.Citation61 However, p38 activation is also reported to promote cancer cell growth and survival.Citation60 It appears that the level and/or duration of p38 activation determines cell cycle arrest or proliferative outcomes.Citation16 Mitogenic stimuli (serum) lead to weak and transient phosphorylation of p38, resulting in increased cyclin D transcription and cell cycle progression. Strong, durable activation of p38 by chemical stress (anisomycin) has the opposite effect, inhibiting cyclin D transcription and causing cell cycle arrest.Citation16 MAPK p38-MSK1 signaling phosphorylates the transcription factor CREB1, which in turn stimulates cyclin D transcription.Citation47-49 In this study, we have shown that cyclin Dbβ mRNA levels were upregulated under TOR inhibition or nutrient-restricted GA, whereas the transcription of other cyclin D variants was unaffected. When p38 signaling was inhibited, the transcription of cyclin Da, Dc and Dd variants was similarly unaffected, but in this case, cyclin Db transcription was significantly reduced. The increased Cyclin Dbβ levels in the mitotic germline appear to be part of a survival response in O. dioica during nutrient-restricted GA.
Conclusions
During the short life cycle of O. dioica, somatic endocycling cells drive rapid growth, while a persistent syncytial organization of the germline allows integration of nutritional history to regulate reproductive output over 3 orders of magnitude.Citation10,62 These 2 cellular contexts respond differently to nutrient-restricted GA (Subramaniam et al.Citation8 this study). Whereas somatic endocycling cells show complete and uniform cell cycle arrest at the mid-point (day 3) of the life cycle, syncytial germline nuclei continue to cycle for many days at a gradually slowing rate but do not enter meiosis as they would under favorable nutritive conditions. We show that the Cyclin D-CDK6-CKI regulatory module is implicated in this differential response through the deployment of different combinations of Cyclin D variants. Cyclin Dd, expressed in both cell types, contains Rb-interaction and phosphodegron motifs, and is found in both somatic endocyling nuclei and germline diploid nuclei. In contrast, Cyclin Db is found only in the germline and the Cyclin Dbβ splice variant, lacking both of the above motifs, localizes strictly to the cytoplasm where it is found in foci along with CKIa. Upon GA, the strong down-regulation of Cyclin Dd in somatic endocycling cells, was more gradual in the germline, where in addition, an opposite, specific, up-regulation of Cyclin Dbβ simultaneously occured. The transcriptional upregulation of this variant in response to nutrient-restricted stress was mediated by the MAPK p38 signaling pathway. In concert, up-regulated CKIaCitation8 accumulated in nuclei of somatic endocycling cells, but was not detected in germline nuclei (this study). With respect to the axes through which Cyclin D-CDK4/6 activity is linked to downstream Cyclin E-CDK2 activity (Rb phosphorylation and CKI sequestration), germline Cyclin Dbβ appears to have specialized in the cytoplasmic sequestration of CKI to positively regulate germline nuclear proliferation in O. dioica. Under normal nutrient conditions, the preferential appearance of Cyclin Db foci adjacent to syncytial germline nuclei in early S-phase is also indicative of a spatial role in organizing the asynchronous cycling of these nuclei in a common cytoplasm. Interestingly, under adequate nutritive conditions, the expression of cyclin Db variants peaks in the day 2 to 3 windowCitation24 when mitotic germline proliferation is maximal. After this juncture, when half of the germline nuclei enter prophase I arrest of meiosis, and the other half enter asynchronous endocycles as nurse nuclei,Citation10,12 cyclin Db mRNA levels decline considerably. This coincides with an inability of O. dioica to enter GA when nutrient restriction is encountered after meiotic entry.Citation8 It also suggests that the regulatory role of Cyclin Dbβ with respect to syncytial germline diploid nuclear proliferation, prior to the midpoint of the life cycle, is largely supplanted by other mechanisms in the more complex coenocyst, when both different and asynchronous cell cycles continue to occupy a common, giant, single-cell cytoplasm.
Materials and Methods
Oikopleura culture and induction of growth arrest (GA)
Collection, culture and induction of growth arrest in O. dioica were done as previously.Citation8,63
Treatment with pharmacological inhibitors
Treatment with the TOR inhibitor CCI-779 (LC chemicals) or MAPK p38 inhibitor SB 203580 (LC chemicals) and DMSO controls were perfomed as previously.Citation8 Under standard culture conditions, O. dioica were treated with 7.5 μM CCI-779, or 15 μM SB 203580 for 24 h in a 3-liter beaker. Treated and control animals were collected and used for quantitative RT-PCR and western blot analysis.
RNA isolation and quantitative RT-PCR
Total RNA was isolated using RNeasy (Qiagen) extraction on samples of 200 animals from day 3 cultures under standard or GA conditions, or from day 3 animals that had been exposed to CCI-779, SB 203580 or DMSO for 24 h. Following DNase treatment (Amp grade-Invitrogen), 1 μg of purified total RNA was used to make cDNA using MMLV reverse transcriptase (Invitrogen). Aliquots of 10 μl of 50-fold diluted cDNA were used to set up 20 μl PCR reaction mixes containing 500 nM each of forward and reverse primers (Table S2) and 2× power SYBR green PCR master mix (Qiagen). Reactions were run in triplicate using the CFX 96 Real time system (Biorad). After initial denaturation for 5 min at 95°C, 40 cycles of 95°C for 15 s, 58°C for 20 s and 72°C for 20 s were conducted with final extension at 72°C for 5 min. Negative controls (without MMLV enzyme) were run 40 cycles. Primer pair specificities were confirmed by melting curve evaluation and sequencing of amplicons.Citation24
Capped mRNA synthesis from eGFP-fusion constructs and microinjection
Open reading frames (ORF) and 3′ UTRs for CKIa and Cyclin Db β were amplified from cDNA using specific primers (Table S3) and cloned into TOPO 2.1 vectors (Life Technologies). The T7 promoter driven eGFP-fused ORF constructs were cloned using restriction sites EcoRV and BamHI. 3′UTRs were inserted using restriction sites BsrGI and XmaI. The eGFP fusion constructs were then linearized using XmaI. Capped mRNA was synthesized using mMessage mMachine (Ambion) and the poly(A) tailing kit (Ambion). Purified capped mRNA (400 ng/µl), along with Alexa Fluor→ 568 fluorescent dye (100 ng/µl; Molecular Probes), was injected into gonads of anesthetized early day 3 animals (pre-meiotic stage) using a Nikon Eclipse TE2000-S microscope equipped with Narishige micromanipulators and transjector 5246 microinjector (Eppendorf). Injected animals were transferred to watch glasses containing filtered seawater and then cultured 8 h in 3 L beakers on a standard diet. Animals were collected for immunofluorescence as described below.
Antibodies
Affinity purified polyclonal anti-Cyclin Dd (GDVDLKKFADHLSIPFEFLRC),Citation8,24 anti-CKIa (CPRSNLDTKVQKSAIKKS),Citation8 Cyclin Db all (LKLHFDVSEVTFPDFYP), and Cyclin Db β/δ (PRRFSRASKMLGQLHANLAEF) antibodies were produced by 21st Century Biochemical (Malbora MA). Primary anti-Histone H3 and anti-CDK6 (Sigma), anti-phospho H3pS28 (Abcam), anti-GFP (Abcam) and anti-IdU (Accurate Chemicals) were used for immunofluorescence staining and protein gel blot analysis as described below.
Immunofluorescence staining
Animals were fixed in 4% paraformaldehyde (PFA)/0.1 M MOPS (pH 7.0)/0.5 M NaCl/5 mM EGTA/0.2% TritonX-100 at 4°C overnight (O/N). Samples were then washed once with PBSTE (1× PBS, 1 mM EDTA, 0.1% TritonX-100) and 3 times with PBSTG (PBSTE+100 mM glycine) at room temperature (RT). Samples were blocked with 3% BSA in PBSTE at 4°C O/N. Incubation of primary antibodies (1:100) was done in 3% BSA in PBSTE at 4°C for 5 days. Samples were then washed 6 times with PBSTE at RT and post-fixed O/N in 4% PFA in PBSTE. Samples were then washed once with PBSTE, 3 times with PBSTG and twice with PBSTE. Following 4-6 days of secondary antibody incubation at 4°C, samples were washed 6 times with PBSTE at RT. DNA was counterstained with 1 μM To-Pro-3 iodide (Molecular Probes) and mounted in vector shield (Vector Laboratories). Samples were analyzed using a Leica TCS laser scanning confocal microscope and Leica (LAS AF v2.3) software. Anti-rabbit and anti-rat Alexa488 (Molecular Probes), and anti-Goat 488, anti-mouse 488 and anti-mouse 568 (Invitrogen) secondary antibodies were used at 1:500 dilution.
Replication pulse labeling
Replication IdU pulse labeling using IdU (Sigma), was done as previously.Citation24,64 For IdU pulse experiments, animals were transferred to fresh sea water containing 0.5 mM IdU in a watch glass and incubated with mild shaking for 45 min. Fixed animals (4% PFA, at 4°C O/N) were washed once with PBST (PBS, 0.2% Tween 20) and 3 times with PBSTG, followed by incubation with DNaseI (5 U/ml amp grade, Invitrogen) in PBSTG, containing 0.5% bovine serum albumin (BSA), and 1 mM MgCl2 for 1 h at RT. Reactions were stopped by adding PBSTG/10 mM EDTA, followed by 6 washes in PBSTE. The samples were blocked overnight with 3% BSA at 4°C and processed for immunostaining as mentioned above.
Co-immunoprecipitation
Animals cultured under standard conditions were collected in fresh seawater and washed once with ice cold PBS prior to snap freezing in liquid nitrogen. To couple antibodies to beads for immunoprecipitation, 3 μg anti-CDK6 or rabbit IgG antibodies were incubated with 10 μl protein G magnetic beads (Invitrogen) for 1 h at RT. Cell lysates from fresh or frozen animals were prepared in RIPA buffer (50 mM Tris (pH7.4), 150 mM Nacl, 1 mM EDTA, 1 mM PMSF (sigma), 1× Protease cocktail (Sigma), 0.25 mM DTT). Lysates were passed through a 20-gauge syringe and centrifuged at 13000 rpm at 4°C to remove cell debris. Supernatants were collected and protein concentrations estimated by Bradford assay (Pierce). Preclearing of the lysate was done with 30 μl Protein G magnetic beads for 1 h at 4°C. Aliquots of 1 mg of precleared lysates were incubated with preconjugated anti-CDK6 or IgG O/N at 4°C. Immunoprecipitated beads were washed with ice cold PBS containing 0.5% triton X 100 3 times. Immune complexes were collected by boiling in 2× Laemmli sample buffer (50 mM Tris pH6.8, 2% SDS, 10% glycerol, 250 mM β–mercaptoethanol, 0.001% bromophenol blue) at 95°C for 10 min and analyzed on 10% SDS polyacrylamide gels.
Whole cell lysate preparation and Western blotting
Animals collected in fresh seawater were washed once with ice-cold PBS prior to snap freezing in liquid nitrogen for future analysis. Whole cell lysates were prepared either from fresh or frozen animals by boiling in 1× Laemmli sample buffer at 95°C for 10 min followed by vortexing and centrifuging 5 min at 13000 g to remove cellular debris. Equal amounts of protein were applied to 10–12% SDS Polyacrylamide gels and transferred to nitrocellulose membranes (Whatman) in 1× transfer buffer (250 mM Tris, 190 mM glycine, 0.5% SDS, 20% methanol). Post-transfer, the membrane was rinsed with 1× Tris Buffer Saline (TBS: 50 mM Tris-Cl, pH 7.6; 150 mM NaCl) and blocked for a minimum of 1 h in 3% BSA in 1× TBST (1× TBS + 0.1% Tween 20) at RT. Membranes were incubated with primary antibodies (1:500 dilution, except for anti-Histone H3 at 1:10000) overnight at 4°C in 3% BSA/TBST. Following primary antibody incubation the membrane was washed 3 times with TBST at RT followed by incubation with HRP-conjugated secondary antibodies(Goat anti-rabbit from Zymed, 1:10000; protein G HRP from Millipore 1:5000) in 3% BSA/TBST for 1 h at RT. Following washes, HRP generated chemiluminescent signal was detected using either the Chemi Doc XRS+ image system (Biorad) or X-ray film after addition of the ECL rapid chemiluminescent detection reagent (Calbiochem).
Disclosure of Potential Conflicts of Interest
No potential conflicts of interest were disclosed.
Author Contributions
GS, CC, and EMT conceived and designed the experiments. GS performed experiments. GS, CC, and EMT analyzed the data. GS and EMT wrote the manuscript. All authors edited and approved the manuscript.
1041690_supplemental_files.pdf
Download PDF (2.1 MB)Acknowledgments
We thank J.-M. Bouquet, M. Reeve and A. Aasjord from Appendic Park for providing animals from the Oikopleura culture.
funding
This work was supported by grants 183690/S10 NFR-FUGE and 133335/V40 from the Norwegian Research Council (EMT).
Supplemental Material
Supplemental data for this article can be accessed on the publisher's website
References
- Spradling A, Drummond-Barbosa D, Kai T. Stem cell and their niche. Nature 2001; 414:98-104; PMID:11689954; http://dx.doi.org/10.1038/35102160
- Narbonne P, Roy R. Regulation of germline stem cell proliferation downstream of nutrient sensing. Cell Div 2006; 1:1-29; PMID:16759411; http://dx.doi.org/10.1186/1747-1028-1-29
- Gancz D, Gilboa L. Hormonal Control of Stem Cell Systems. Annu Rev Cell Dev Biol 2013; 29:137-62; PMID:23875645; http://dx.doi.org/10.1146/annurev-cellbio-101512-122331
- Angelo G, Van Gilst MR. Starvation protects germline stem cells and extends reproductive longevity in C. elegans. Science 2009; 326:954-58; PMID:19713489; http://dx.doi.org/10.1126/science.1178343
- Fielenbach N, Antebi A. C. elegans dauer formation and the molecular basis of plasticity. Genes Dev 2008; 22:2149-165; PMID:18708575; http://dx.doi.org/10.1101/gad.1701508
- Tatar M, Yin C. Slow aging during insect reproductive diapause: why butterflies, grasshoppers and flies are like worms. Exp Gerontol 2001; 36:723-738; PMID:11295511; http://dx.doi.org/10.1016/S0531-5565(00)00238-2
- Bertoli C, Skotheim JM, de Bruin RAM. Control of cell cycle transcription during G1 and S phases. Nat Rev Mol Cell Biol 2013; 14:518-28; PMID:23877564; http://dx.doi.org/10.1038/nrm3629
- Subramaniam G, Campsteijn C, Thompson EM. Lifespan extension in a semelparous chordate occurs via developmental growth arrest just prior to meiotic entry. PLoS one 2014; 9:e93787; PMID:24695788; http://dx.doi.org/10.1371/journal.pone.0093787
- Delsuc F, Tsagkogeorga G, Lartillot N, Philippe H. Additional molecular support for the new chordate phylogeny. Genesis 2008; 46:592-94; PMID:19003928; http://dx.doi.org/10.1002/dvg.20450
- Ganot P, Bouquet JM, Kallesøe T, Thompson EM. The Oikopleura coenocyst, a unique chordate germ cell permitting rapid, extensive modulation of oocyte production. Dev Biol 2007; 302:591-600; PMID:17126826; http://dx.doi.org/10.1016/j.ydbio.2006.10.021
- Ganot P, Bouquet JM, Thompson EM. Comparative organization of follicle, accessory cells and spawning anlagen in dynamic semelparous clutch manipulators, the urochordate Oikopleuridae. Biol Cell 2006; 98:389-401; PMID:16478443; http://dx.doi.org/10.1042/BC20060005
- Ganot P, Kallesoe T, Thompson EM. The cytoskeleton organizes germ nuclei with divergent fates and asynchronous cycles in a common cytoplasm during oogenesis in the chordate Oikopleura. Dev Biol 2007; 302:577-90; PMID:17123503; http://dx.doi.org/10.1016/j.ydbio.2006.10.022
- Wang X, Proud CG. Nutrient control of TORC1, a cell-cycle regulator. Trends Cell Biol 2009; 19:260-67; PMID:19419870; http://dx.doi.org/10.1016/j.tcb.2009.03.005
- Balmanno K, Cook SJ. Sustained MAP kinase activation is required for the expression of cyclin D1, p21Cip1 and a subset of AP-1 proteins in CCL39 cells. Oncogene 1999; 18:3085-97; PMID:10340380; http://dx.doi.org/10.1038/sj.onc.1202647
- Shaulian E, Karin M. AP-1 in cell proliferation and survival. Oncogene 2001; 20:2390-400; PMID:11402335; http://dx.doi.org/10.1038/sj.onc.1204383
- Faust D, Schmitt C, Oesch F, Oesch-Bartlomowicz B, Schreck I, Weiss C, Dietrich C. Differential p38-dependent signalling in response to cellular stress and mitogenic stimulation in fibroblasts. Cell Commun Signal 2012; 10:1-6; PMID:22404972; http://dx.doi.org/10.1186/1478-811X-10-6
- Narasimha AM, Kaulich M, Shapiro GS, Choi YJ, Sicinski P, Dowdy SF. Cyclin D activates the Rb tumor suppressor by mono-phosphorylation. ELife 2014; 3:e02872; http://dx.doi.org/10.7554/eLife.02872
- Fox PM, Vought VE, Hanazawa M, Lee MH, Maine EM, Schedl T. Cyclin E and CDK-2 regulate proliferative cell fate and cell cycle progression in the C. elegans germline. Development 2011; 138:2223-234; PMID:21558371; http://dx.doi.org/10.1242/dev.059535
- Hsu HJ, LaFever L, Drummond-Barbosa D. Diet controls normal and tumorous germline stem cells via insulin-dependent and -independent mechanisms in Drosophila. Dev Biol 2008; 313:700-12; PMID:18068153; http://dx.doi.org/10.1016/j.ydbio.2007.11.006
- Ables ET, Drummond-Barbosa D. The Steroid hormone ccdysone functions with intrinsic chromatin remodeling factors to control female germline stem cells in Drosophila. Cell Stem Cell 2010; 7:581-92; PMID:21040900; http://dx.doi.org/10.1016/j.stem.2010.10.001
- Ables ET, Drummond-Barbosa D. Cyclin E controls Drosophila female germline stem cell maintenance independently of its role in proliferation by modulating responsiveness to niche signals. Development 2013; 140:530-40; PMID:23293285; http://dx.doi.org/10.1242/dev.088583
- LaFever L, Feoktistov A, Hsu HJ, Drummond-Barbosa D. Specific roles of Target of rapamycin in the control of stem cells and their progeny in the Drosophila ovary. Development 2010; 137:2117-127; PMID:20504961; http://dx.doi.org/10.1242/dev.050351
- Beumer TL, Roepers-Gajadien HL, Gademan IS, Kal HB, de Rooij DG. Involvement of the D-type cyclins in germ cell proliferation and differentiation in the mouse. Biol Reprod 2000; 63:1893-898; PMID:11090462; http://dx.doi.org/10.1095/biolreprod63.6.1893
- Campsteijn C, Øvrebø JI, Karlsen BO, Thompson EM. Expansion of cyclin D and CDK1 paralogs in Oikopleura dioica, a chordate employing diverse cell cycle variants. Mol Biol Evol 2012; 29:487-502; PMID:21734012; http://dx.doi.org/10.1093/molbev/msr136
- Hong Y, Roy R, Ambros V. Developmental regulation of a cyclin-dependent kinase inhibitor controls postembryonic cell cycle progression in Caenorhabditis elegans. Development 1998; 125:3585-597; PMID:9716524
- Boxem MS, van den Heuvel S. lin-35 Rb and cki-1 Cip/ Kip cooperate in developmental regulation of G1 progression in C. elegans. Development 2001; 128:4349-359; PMID:11684669
- Feng H, Zhong W, Punkosdy G, Gu S, Zhou L, Seabolt EK, Kipreos ET. CUL 2 is required for the G1-to-S-phase transition and mitotic chromosome condensation in Caenorhabditis elegans. Nat Cell Biol 1999; 1:486-92; PMID:10587644; http://dx.doi.org/10.1038/70272
- Kalchhauser I, Farley BM, Pauli S, Ryder SP, Ciosk R. FBF represses the Cip/Kip cell-cycle inhibitor CKI-2 to promote self-renewal of germline stem cells in C. elegans. EMBO J 2011; 30:3823-829; PMID:21822213; http://dx.doi.org/10.1038/emboj.2011.263
- Yu JY, Reynolds SH, Hatfield SD, Shcherbata HR, Fischer KA, Ward EJ, Long D, Ding Y, Ruohola-Baker H. Dicer-1-dependent Dacapo suppression acts downstream of Insulin receptor in regulating cell division of Drosophila germline stem cells. Development 2009; 136:1497-507; PMID:19336466; http://dx.doi.org/10.1242/dev.025999
- Kim J, Jonasch E, Alexander A, Short JD, Cai SL, Wen SJ, Tsavachidou D, Tamboli P, Czerniak BA, Do KA, et al. Cytoplasmic Sequestration of p27 via AKT Phosphorylation in Renal Cell Carcinoma. Clin Cancer Res 2009; 15:81-90; PMID:19118035; http://dx.doi.org/10.1158/1078-0432.CCR-08-0170
- Meyyappan M, Wong H, Hull C, Riabowol KT. Increased expression of cyclin D2 during multiple states of growth arrest in primary and established cells. Mol Cell Biol 1998; 18:3163-172; PMID:9584157
- Narbonne P, Roy R. Inhibition of germline proliferation during C. elegans dauer development requires PTEN, LKB1 and AMPK signalling. Development 2006; 133:611-19; PMID:16407400; http://dx.doi.org/10.1242/dev.02232
- Perez-Roger I, Kim SH, Griffiths B, Sewing A, Land H. Cyclins D1 and D2 mediate Myc-induced proliferation via sequestration of p27(Kip1) and p21(Cip1). EMBO J 1999; 18:5310-320; PMID:10508164; http://dx.doi.org/10.1093/emboj/18.19.5310
- Bouchard C, Thieke K, Maier A, Saffrich R, Hanley-Hyde J, Ansorge W, Reed S, Sicinski P, Bartek J, Eilers M. Direct induction of cyclin D2 by Myc contributes to cell cycle progression and sequestration of p27. EMBO J 1999; 18:5321-333; PMID:10508165; http://dx.doi.org/10.1093/emboj/18.19.5321
- Diehl JA, Sherr CJ. A dominant-negative cyclin D1 mutant prevents nuclear import of cyclin-dependent kinase 4 (CDK4) and its phosphorylation by CDK-activating kinase. Mol Cell Biol 1997; 17:7362-374; PMID:9372967
- Denicourt C, Legault P, McNabb FA, Rassart E. Human and mouse cyclin D2 splice variants: transforming activity and subcellular localization. Oncogene 2008; 27:1253-262; PMID:17873913; http://dx.doi.org/10.1038/sj.onc.1210750
- Denicourt C, Kozak CA, Rassart E. Gris1, a new common integration site in Graffi murine leukemia virus-induced leukemias: Overexpression of a truncated cyclin D2 due to alternative splicing. J Virol 2003; 77:37-44; PMID:12477808; http://dx.doi.org/10.1128/JVI.77.1.37-44.2003
- Sun Q, Zhang FX, Wafa K, Baptist T, Pasumarthi KB. A splice variant of cyclin D2 regulates cardiomyocyte cell cycle through a novel protein aggregation pathway. J Cell Sci 2009; 122:1563-573; PMID:19401331; http://dx.doi.org/10.1242/jcs.047738
- Wafa K, MacLean J, Zhang FX, Pasumarthi KB. Characterization of growth suppressive functions of a splice variant of cyclin D2. PLoS One 2013; 8:e53503; PMID:23326442; http://dx.doi.org/10.1371/journal.pone.0053503
- Wafa K. The Role of a Cyclin D2 Splice Variant in the Regulation of the Cell Cycle: Connecting Cell Cycle to Cancer Biology. Ph.D Thesis, 2014; Dalhousie University, Canada. http://dalspace.library.dal.ca//handle/10222/53133
- Pietras EM, Warr MR, Passegue E. Cell cycle regulation in hematopoietic stem cells. J Cell Biol 2011; 195:709-20; PMID:22123859; http://dx.doi.org/10.1083/jcb.201102131
- Crittenden SL, Leonhard KA, Byrd DT, Kimble J. Cellular analyses of the mitotic region in the Caenorhabditis elegans adult germ line. Mol Biol Cell 2006; 17:3051-61; PMID:16672375; http://dx.doi.org/10.1091/mbc.E06-03-0170
- Solomon DA, Wang Y, Sr F, Lambeck TC, Giesting S, Lan Z, Senderowicz AM, Conti CJ, Knudsen ES. Cyclin D1 splice variants – Differential effects on localization, RB phosphorylation, and cellular transformation. J Biol Chem 2003; 278:30339-47; PMID:12746453; http://dx.doi.org/10.1074/jbc.M303969200
- Zwicker J, Brüsselbach S, Jooss KU, Sewing A, Behn M, Lucibello FC, Müller R. Functional domains in cyclin D1: pRb-kinase activity is not essential for transformation. Oncogene 1999; 18:19-25; PMID:9926916; http://dx.doi.org/10.1038/sj.onc.1202286
- Landis MW, Pawlyk BS, Li T, Sicinski P, Hinds PW. Cyclin D1-dependent kinase activity in murine development and mammary tumorigenesis. Cancer Cell 2006; 9:13-22; PMID:16413468; http://dx.doi.org/10.1016/j.ccr.2005.12.019
- Perez-Roger I, Kim SH, Griffiths B, Sewing A, Land H. Cyclins D1 and D2 mediate myc-induced proliferation via sequestration of p27(Kip1) and p21(Cip1). EMBO J 1999; 18:5310-320; PMID:10508164; http://dx.doi.org/10.1093/emboj/18.19.5310
- Deak M, Clifton AD, Lucocq JM, Alessi DR. Mitogen- and stress-activated protein kinase-1 (MSK1) is directly activated by MAPK and SAPK2/p38, and may mediate activation of CREB. EMBO J 1998; 17:4426-441; PMID:9687510; http://dx.doi.org/10.1093/emboj/17.15.4426
- Iordanov M, Bender K, Ade T, Schmid W, Sachsenmaier C, Engel K, Gaestel M, Rahmsdorf HJ, Herrlich P. CREB is activated by UVC through a p38/HOG-1-dependent protein kinase. EMBO J 1997; 16:1009-22; PMID:9118940; http://dx.doi.org/10.1093/emboj/16.5.1009
- Wiggin GR, Soloaga A, Foster JM, Murray-Tait V, Cohen P, Arthur JS. MSK1 and MSK2 are required for the mitogen-and stress-induced phosphorylation of CREB and ATF1 in fibroblasts. Mol Cell Biol 2002; 22:2871-881; PMID:11909979; http://dx.doi.org/10.1128/MCB.22.8.2871-2881.2002
- Sherr CJ, Roberts JM. CDK inhibitors: positive and negative regulators of G1-phase progression. Genes Dev 1999; 13:1501-512; PMID:10385618; http://dx.doi.org/10.1101/gad.13.12.1501
- Groth A, Willumsen BM. High-density growth arrest in Ras-transformed cells: low Cdk kinase activities in spite of absence of p27(Kip) Cdk-complexes. Cell Signal 2005; 17:1063-73; PMID:15993748; http://dx.doi.org/10.1016/j.cellsig.2004.11.021
- Beumer TL, Roepers-Gajadien HL, Gademan LS, Rutgers DH, de Rooij DG. P21(Cip1/WAF1) expression in the mouse testis before and after X irradiation. Mol Reprod Dev 1997; 47:240-47; PMID:9170103; http://dx.doi.org/10.1002/(SICI)1098-2795(199707)47:3%3c240::AID-MRD2%3e3.0.CO;2-L
- Blain SW. Switching cyclin D-Cdk4 kinase activity on and off. Cell Cycle 2008; 7:892-98; PMID:18414028; http://dx.doi.org/10.4161/cc.7.7.5637
- Larrea MD, Liang JY, Da Silva T, Hong F, Shao SH, Han K, Dumont D, Slingerland JM. Phosphorylation of p27(Kip1) regulates assembly and activation of cyclin D1-Cdk4. Mol Cell Biol 2008; 28:6462-472; PMID:18710949; http://dx.doi.org/10.1128/MCB.02300-07
- Meyer CA, Jacobs HW, Datar SA, Du W, Edgar BA, Lehner CF. Drosophila Cdk4 is required for normal growth and is dispensable for cell cycle progression. EMBO J 2000; 19:4533-542; PMID:10970847; http://dx.doi.org/10.1093/emboj/19.17.4533
- Kalchhauser I, Farley BM, Pauli S, Ryder SP, Ciosk R. FBF represses the Cip/Kip cell-cycle inhibitor CKI-2 to promote self-renewal of germline stem cells in C. elegans. EMBO J 2011; 30:3823-829
- Beumer TL, Kiyokawa H, Roepers-Gajadien HL, van den Bos LA, Lock TM, Gademan IS, Rutgers DH, Koff A, de Rooij DG. Regulatory role of p27kip1 in the mouse and human testis. Endocrinology 1999; 140:1834-840; PMID:10098522
- Fukuyama M, Gendreau SB, Derry WB, Rothman JH. Essential embryonic roles of the CKI-1 cyclin-dependent kinase inhibitor in cell-cycle exit and morphogenesis in C. elegans. Dev Biol 2003; 260:273-86; PMID:12885569; http://dx.doi.org/10.1016/S0012-1606(03)00239-2
- Han JH, Sun PQ. The pathways to tumor suppression via route p38. Trends Biochem Sci 2007; 32:364-71; PMID:17624785; http://dx.doi.org/10.1016/j.tibs.2007.06.007
- Junttila MR, Li SP, Westermarck J. Phosphatase-mediated crosstalk between MAPK signalling pathways in the regulation of cell survival. FASEB J 2008; 22:954-65; PMID:18039929; http://dx.doi.org/10.1096/fj.06-7859rev
- Lavoie JN, L'Allemain G, Brunet A, Müller R, Pouysségur J. Cyclin D1 expression is regulated positively by the p42/p44MAPK and negatively by the p38/HOGMAPK pathway. J Biol Chem 1996 271:20608-616; PMID:8702807; http://dx.doi.org/10.1074/jbc.271.34.20608
- Ganot P, Thompson EM. Patterning through differential endoreduplication in epithelial organogenesis of the chordate, Oikopleura dioica. Dev Biol 2002; 252:59-71; PMID:12453460; http://dx.doi.org/10.1006/dbio.2002.0834
- Bouqet JM, Spriet E, Troedsson C, Ottera H, Chourrout D, Thompson EM. Culture optimization for the emergent zooplanktonic model organism Oikopleura dioica. J Plankton Res 2009; 31:359-70; PMID:19461862; http://dx.doi.org/10.1093/plankt/fbn132
- Spada F, Chioda M, Thompson EM. Histone H4 post-translational modifications in chordate mitotic and endoreduplicative cell cycles. J Cell Biochem 2005; 95:885-901; PMID:15937898; http://dx.doi.org/10.1002/jcb.20416
- Schultz J, Milpetz F, Bork P, Ponting CP. SMART, a simple modular architecture research tool: identification of signaling domains. Proc Natl Acad Sci U S A 1998; 95:5857-864; PMID:9600884; http://dx.doi.org/10.1073/pnas.95.11.5857
- Dinkel H, Van Roey K, Michael S, Davey NE, Weatheritt RJ, Born D, Speck T, Krüger D, Grebnev G, Kuban M, Strumillo M, et al. The eukaryotic linear motif resource ELM: 10 years and counting. Nucleic Acids Res 2014; 42:259-66; PMID:24214962; http://dx.doi.org/10.1093/nar/gkt1047