Abstract
DNA topoisomerase II (TopoII) regulates DNA topology by its strand passaging reaction, which is required for genome maintenance by resolving tangled genomic DNA. In addition, TopoII contributes to the structural integrity of mitotic chromosomes and to the activation of cell cycle checkpoints in mitosis. Post-translational modification of TopoII is one of the key mechanisms by which its broad functions are regulated during mitosis. SUMOylation of TopoII is conserved in eukaryotes and plays a critical role in chromosome segregation. Using Xenopus laevis egg extract, we demonstrated previously that TopoIIα is modified by SUMO on mitotic chromosomes and that its activity is modulated via SUMOylation of its lysine at 660. However, both biochemical and genetic analyses indicated that TopoII has multiple SUMOylation sites in addition to Lys660, and the functions of the other SUMOylation sites were not clearly determined. In this study, we identified the SUMOylation sites on the C-terminal domain (CTD) of TopoIIα. CTD SUMOylation did not affect TopoIIα activity, indicating that its function is distinct from that of Lys660 SUMOylation. We found that CTD SUMOylation promotes protein binding and that Claspin, a well-established cell cycle checkpoint mediator, is one of the SUMOylation-dependent binding proteins. Claspin harbors 2 SUMO-interacting motifs (SIMs), and its robust association to mitotic chromosomes requires both the SIMs and TopoIIα-CTD SUMOylation. Claspin localizes to the mitotic centromeres depending on mitotic SUMOylation, suggesting that TopoIIα-CTD SUMOylation regulates the centromeric localization of Claspin. Our findings provide a novel mechanistic insight regarding how TopoIIα-CTD SUMOylation contributes to mitotic centromere activity.
Abbreviations
aa | = | amino acid |
Chk1 | = | Checkpoint kinase 1 |
CSF | = | cytostatic factor |
CTD | = | C-terminal domain |
SENP2 | = | sentrin-specific protease 2 |
SIM | = | SUMO-interacting motif |
SUMO | = | small ubiquitin-like modifier |
XEE | = | Xenopus egg extract. |
Introduction
DNA topoisomerase II (TopoII) is an essential enzyme that resolves topological constraints in genomic DNA.Citation1,2 During mitosis, TopoII activity is a requisite for chromosome segregation to untangle centromeric DNA as anaphase commences.Citation3-Citation7 Recent results have indicated a connection between TopoII strand passaging activity and mitotic checkpoint activation.Citation8 However, the molecular mechanism of this linkage has not yet been clearly determined. TopoII is a conserved target of SUMO modification from yeast to humans,Citation9-Citation12 and this modification occurs only during mitosis in Xenopus egg extract (XEE) assays.Citation13 Our previous results demonstrated that SUMOylation at Lys660 of Xenopus TopoIIα regulates its enzymatic activity at the centromere, highlighting an important role of mitotic SUMOylation in centromeric resolution.Citation14 However, TopoIIα contains multiple unidentified SUMO acceptors in addition to Lys660, and their roles in mitotic progression remain unknown.
Previous studies in budding yeast identified multiple SUMO acceptor lysines on TopoII.Citation11,15 Yeast expressing the non-SUMOylatable TopoII showed defects in centromeric cohesionCitation11 and chromosome transmission fidelity.Citation15 However, all SUMOylation sites identified in the yeast TopoII reside in the C-terminal domain (CTD), a region dispensable for its catalytic activity.Citation16 Thus, CTD SUMOylation may function independent of TopoII activity regulation; however, how CTD SUMOylation contributes to mitotic events remains uncertain.
In this report, we identified SUMO acceptor sites in the CTD of Xenopus TopoIIα. CTD SUMOylation did not contribute to the inhibition of TopoIIα DNA decatenation activity. Instead, TopoIIα-CTD SUMOylation had a unique role in mediating protein interactions. We identified Claspin as a SUMOylation-dependent CTD-binding protein by mass spectrometry analysis. Claspin interacts with mitotic chromosomes via SIMs and TopoIIα-CTD SUMOylation. Claspin localization is enriched at the mitotic centromeres when SUMO modification occurs. Taken together, our results suggest that defects in TopoIIα-CTD SUMOylation could cause alterations in the recruitment of proteins on mitotic centromeres, as demonstrated by Claspin, and that TopoII SUMOylation could regulate TopoII enzymatic and its protein binding activities.
Results
SUMOylation of TopoIIα-CTD does not contribute to the inhibition of TopoIIα activity
Our initial attempt to search for the SUMOylation sites of TopoIIα using LC-MS/MS and endogenously modified TopoIIα identified only Lys660 as a SUMO acceptor site, with approximately 50% coverage of the TopoIIα primary sequence.Citation14 The CTD of TopoIIα is enriched with lysine and arginine in its primary sequence. Thus, obtaining a peptide length analyzable by LC-MS/MS after protease digestion of the sample was difficult. To overcome this problem, we took advantage of an in vitro reconstituted SUMOylation system to identify the remaining SUMOylation sites. We demonstrated previously that in vitro assays using a physiological concentration of SUMOylation enzymes recapitulate in vivo conditions.Citation14,17 We tested various truncations of TopoIIα in the SUMOylation assays. TopoIIα-CTD (1,220–1,579 aa) was among the efficiently SUMOylated substrates in both E2- and E3 (PIASy)-dependent assays ().Citation14 Using a SUMOylation site prediction program,Citation18 we found 7 canonical SUMOylation sites predicted on the CTD with high probability. Those sites were subsequently mutated and examined for their SUMOylation status using in vitro assays. In addition to these sites, other 6 lysines which were either adjacent to the predicted sites or non-canonical SUMOylation sites with low probability were chosen to be replaced by arginine. Three of the 13 examined lysines (Lys1235, Lys1276, and Lys1298) exhibited reduced modifications compared to wild-type (WT) TopoIIα-CTD (, bold with line). The sequence surrounding the 3 sites was highly conserved between amphibian and human TopoIIα and was closely positioned to the genetically identified SUMOylation sites in budding yeast.Citation11,15 Substitution of the 3 lysines to arginines (CTD-3KR) resulted in SUMO modification resistance in both E2-dependent (, upper panel) and E3-dependent reactions (, lower panel).
Figure 1. Identification of 3 major SUMOylation sites on TopoIIα-CTD. (A) In vitro SUMOylation was performed for T7-tagged TopoIIα-CTD fragments under the indicated conditions. The reactions were analyzed by immunoblotting with HRP-conjugated anti-T7 antibody. Bracket indicates SUMOylated TopoIIα-CTD (S-CTD), and a bar indicates non-SUMOylated TopoIIα-CTD. (B) TopoIIα-CTD primary sequence. The bold letters indicate the lysines tested for SUMOylation capability. SUMO acceptor lysines were underlined. (C) The 3 underlined lysines in (B) were mutated to arginine (CTD-3KR). Both CTD and CTD-3KR were subjected to SUMOylation assays with the indicated concentrations of enzymes and collected at the indicated time points. S-CTD and CTD are shown with a bracket and bar, respectively. (D) XEEs were immunodepleted using non-specific IgG (Cont.) or anti-TopoIIα antibody (−TopoIIα) and the depletion efficiency was confirmed by immunoblotting the extracts (left 2 lanes, labeled XEE). WT or mutant TopoIIα (3KR) was added to the TopoIIα-depleted extracts. The mitotic chromosomes were analyzed by immunoblotting with anti-TopoIIα. The reaction with dominant negative mutant Ubc9 (dnUbc9) was included as a negative control (+dn). SUMOylated TopoIIα is indicated with a bracket.
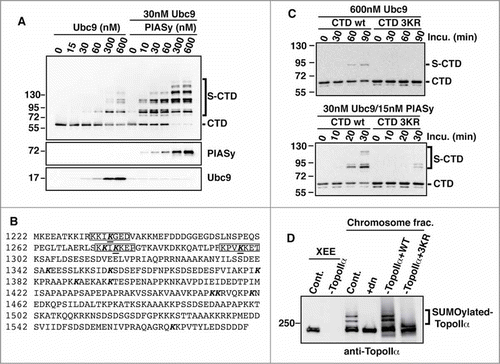
To confirm these results under physiological conditions, we introduced the same mutations into the full-length TopoIIα. Both the WT and CTD mutant (3KR) proteins were prepared using a yeast (Pichia pastoris) expression system as reported previously.Citation14 Then, endogenous TopoIIα was replaced with either recombinant WT or 3KR in XEE via immunodepletion/add-back. Mitotic chromosomes from the replaced XEEs were examined for the SUMOylation status of TopoIIα by anti-TopoIIα immunoblotting. Consistent with the in vitro assays, the 3KR proteins showed dramatically reduced SUMOylation on mitotic chromosomes (bracket in ), indicating that the identified lysines were bona fide SUMO acceptors. Considering the results of both the in vitro and XEE assays, we concluded that TopoIIα has at least 3 major SUMOylation sites within its CTD.
Because TopoIIα SUMOylation inhibits its enzymatic activity,Citation14 we examined whether TopoIIα-CTD SUMOylation was also involved in regulating this activity. We prepared another TopoIIα construct that had mutations in both the K660R and 3KR acceptors (hereafter referred to as 4KR). Recombinant TopoIIα WT, K660R, 3KR, and 4KR proteins were subjected to SUMOylation in vitro. For mock SUMOylation, non-conjugatable SUMO was used in the reactions. Subsequently, the mock and SUMOylated TopoIIα proteins were incubated with kinetoplast DNA to determine their enzymatic activity. Elimination of the CTD SUMO acceptors (3KR) did not affect SUMOylation-mediated inhibition of the decatenation activity (, indicated by an arrow). In contrast, the activities of 4KR and K660R were resistant to SUMOylation (). Taken together, we verified our previous finding that among all SUMOylation sites, K660 SUMOylation is uniquely required for regulating TopoIIα activity and concluded that the CTD SUMOylation is not involved in inhibiting TopoIIα activity.
Figure 2. The SUMOylation of TopoIIα-CTD is not required for the SUMOylation-dependent inhibition of decatenation activity. (A) Both unSUMOylated (−) and SUMOylated TopoIIα (+) WT, K660R, 3KR, and 4KR were incubated with kinetoplast DNA (kDNA) for the indicated time. The reactions were resolved in an agarose gel to separate decatenated (bracket) from catenated DNA (arrow). (B, C) The band intensity of catenated kDNA from 3 independent experiments was measured as described in the Materials and methods. The relative amount of remaining catenated kDNA is presented as % catenated kDNA (F), and the inhibition rate by SUMOylation of each reaction was calculated by (% catenated DNA in +SUMO reaction)/(% catenated DNA in -SUMO reaction) in each assay with standard error.
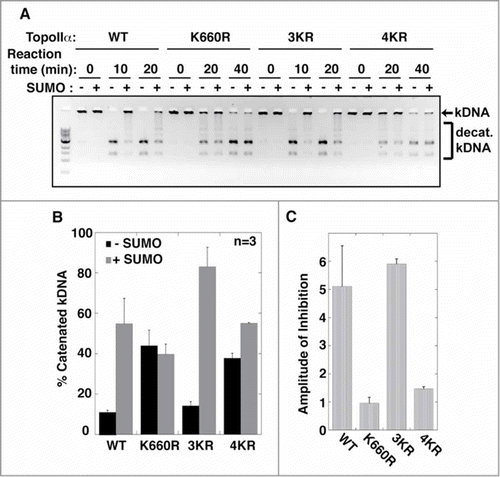
SUMOylation of TopoIIα-CTD promotes protein interactions
SUMO conjugation of target proteins creates a new binding site for proteins that possess a SUMO-interacting motif (SIM).Citation19 Thus, we speculated that CTD SUMOylation might alter TopoIIα/protein interactions during mitosis. To examine TopoIIα-binding proteins, S-tagged CTD was SUMOylated (S-CTD) in vitro and then both the CTD and S-CTD proteins were immobilized onto S-protein agarose beads. The beads with the CTD and S-CTD proteins attached were incubated in mitotic XEEs supplemented with a deSUMOylation inhibitor (iodoacetamide), and the proteins bound to the beads were analyzed by SDS-PAGE followed by silver staining. As shown in , multiple proteins (indicated with asterisks) precipitated with the S-CTD from XEE (compare lanes 3 and 4), while few precipitated with the CTD from XEE, suggesting that CTD SUMOylation promotes specific protein binding.
Figure 3. SUMOylation of TopoIIα-CTD promotes protein interactions. (A) Both mock-SUMOylated (CTD) and SUMOylated CTD (S-CTD) were bound to S-agarose beads and incubated with either XEE (+XEE) or buffer (−XEE). Empty S-agarose beads were used as a negative control (indicated as beads). Proteins that bound to the beads were visualized with silver staining. The asterisks indicate bands observed only in the S-CTD-bound beads when incubated with XEE. (B) The pulled-down beads were incubated with the SENP2 catalytic domain (SENP2-CD, indicated with an arrow), and soluble fractions were visualized with silver staining. Digested SUMO2 moiety from the S-CTD is indicated with an arrow. The eluted binding protein of approximately 250 kDa (an asterisk) was identified as Claspin by LC-MS/MS analysis. (C) The SENP2 elution fractions in panel B) were analyzed by immunoblotting with anti-Claspin antibody. The input represents 10% of the XEE used for the pull-down assays. (D) The pulled-down fractions with SUMO chain (GFP-SUMO2x4) were analyzed by immunoblotting using anti-Claspin and anti-PICH antibodies.
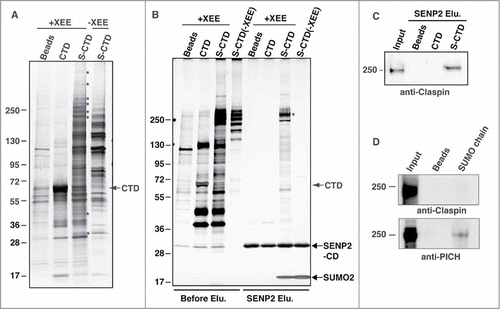
To confirm whether the precipitated proteins are dependent on SUMOylation, we introduced a SENP2-cleavage step in the pull-down assays. SENP2 catalytic domain (SENP2-CD) actively cleaves SUMO moieties from SUMOylated substrates.Citation20 Thus, incubating S-CTD with SENP2-CD will remove the SUMO moiety from the S-CTD and promote the dissociation of proteins that depend on SUMOylation for their binding. As expected, SUMO was efficiently removed from the S-CTD (, bottom arrow). Moreover, various proteins were eluted from the S-CTD beads by the deSUMOylation step, whereas few, if any, proteins were eluted from any other beads by the same step. One of the prominent bands (approximately 250 kDa; , asterisk) was isolated and subjected to LC-MS/MS analysis for identification (performed in Dr. William Lane's laboratory at Harvard University). The analysis indicated Claspin as the most likely candidate. Immunoblot analyses with anti-Claspin antibody indeed showed that Claspin was detected only in SENP2-eluted fractions from the S-CTD precipitates (). In contrast to Polo-like kinase 1-interacting checkpoint helicase (PICH), which we recently identified as a SUMO-binding protein,Citation21 the SUMO chain was not sufficient to pull down Claspin (). This finding suggests that Claspin is not a promiscuous SUMO-binding protein. Considering these results, we concluded that TopoIIα-CTD SUMOylation modulates TopoIIα/protein interactions and that Claspin is one of the SUMOylation-dependent TopoIIα-CTD-binding proteins.
Claspin binds to mitotic chromosomes in a SUMOylation-dependent manner
Because TopoIIα SUMOylation occurs on mitotic chromosomes in XEE assays,Citation14,22 we hypothesized that TopoIIα-CTD SUMOylation might mediate Claspin recruitment onto chromosomes. To address this hypothesis, mitotic chromosomes were prepared in XEEs with or without SUMOylation.Citation13 Then, the isolated chromosomes were analyzed for Claspin by immunoblotting. We observed that Claspin associated with mitotic chromosomes and, more importantly, that the chromosomal association of Claspin was drastically reduced when mitotic SUMOylation was inhibited (). Normalization of the Claspin amounts using RCC1 indicated that approximately 70% of the Claspin proteins required SUMOylation for their binding to mitotic chromosomes (). To further examine whether TopoIIα-CTD SUMOylation is specifically responsible for the chromosomal association of Claspin, mitotic chromosomes were prepared from TopoIIα WT or 3KR/4KR-replaced CSF-XEE, and chromosomal Claspin was analyzed by immunoblotting. Replacing endogenous TopoIIα with recombinant WT clearly showed SUMOylation-dependent binding of Claspin to mitotic chromosomes similar to the results shown in . Conversely, replacing endogenous TopoIIα with either 3KR or 4KR disrupted the SUMOylation-dependent binding of Claspin (). These results strongly suggested that Claspin interacts with chromosomes according to mitotic SUMOylation and that TopoIIα-CTD SUMOylation is primarily responsible for SUMOylation-dependent Claspin binding to mitotic chromosomes.
Figure 4. TopoIIα-CTD SUMOylation is required for the robust binding of Claspin to mitotic chromosomes. (A) Mitotic chromosomes were prepared from XEE with (Cont.) or without (+dn) mitotic SUMOylation. The isolated chromosomes were analyzed for the indicated proteins by immunoblotting. SUMOylation of TopoIIα and PARP1, both of which are established mitotic chromosomal SUMOylated proteins, is inhibited by addition of dnUbc9. Molecular weight markers are shown on each side (kDa). (B) Four independent experiments as in A) were performed. The relative amount of chromosome-bound Claspin was normalized with RCC1 and measured as described in the Materials and methods. “Cont.” and “+dn” indicate the conditions with and without SUMOylation, respectively. Standard error is shown with a bar. (C) Endogenous TopoIIα was replaced with either recombinant TopoIIα-WT, K3R or K4R. The efficiency of depletion was confirmed by immunoblotting (left panel, Extracts). Mitotic chromosomes from TopoIIα-replaced XEEs were analyzed by immunoblotting with the indicated antibodies. The SUMOylated form of TopoIIα is indicated by a bracket.
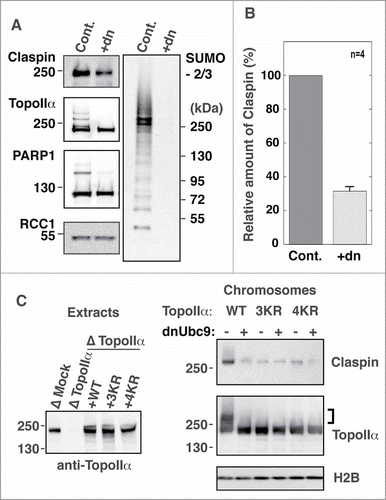
Claspin localizes at the mitotic centromere in a SUMOylation-dependent manner
Because TopoIIα SUMOylation is observed on the centromeric region of chromosomes, we hypothesized that Claspin might display localization similar to SUMOylated TopoIIα. To examine this hypothesis, we analyzed mitotic chromosomes obtained from XEE assays by immunofluorescence staining. As shown in , Claspin was observed at mitotic chromosomes with an apparent enrichment at the centromere, and the Claspin foci were co-localized with the SUMO2/3 foci.Citation14,22 The centromeric accumulation of Claspin drastically decreased when mitotic chromosomal SUMOylation was inhibited by the addition of dnUbc9 but did not affect the centromeric localization of TopoIIα (). These results indicate that SUMOylation is important for the efficient recruitment of Claspin on centromeres of mitotic chromosomes.
Figure 5. The centromeric localization of Claspin is regulated by mitotic SUMOylation. Mitotic chromosomes prepared from XEEs were subjected to immunofluorescence staining with the indicated antibodies. The right panels are merged images of TopoIIα in red, Claspin in green and DNA in blue. Upper panels) A group of chromosomes. Lower panels) A single pair of sister chromatids. Bars indicate 10 μm.
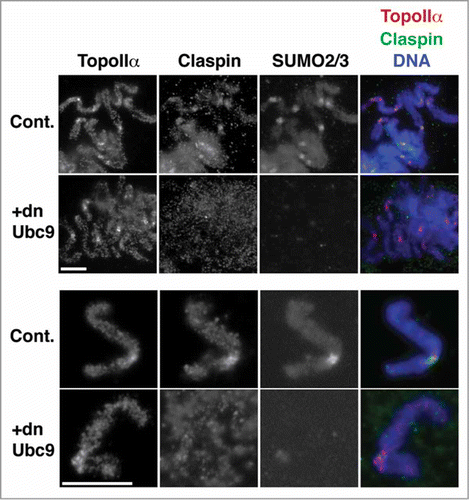
We sought to examine whether SIMs are involved in this mechanism of Claspin association with mitotic chromosomes. We found 2 potential SIMsCitation23-Citation25 which both have an acidic amino acid adjacent to a stretch of hydrophobic amino acids in the Claspin primary sequence. The primary sequences observed as SIMs at amino acids 65 to 68 (NSIM) and 928 to 931 (MSIM) are highly conserved between frog and human. We introduced mutations in both SIM sequences as shown in . Mutant Claspin at either one of the SIMs (indicated as NSIM or MSIM) or both (dSIM) were expressed in rabbit reticulocyte lysates along with WT separately (Fig. SA). These reticulocyte lysates were used to replace endogenous Claspin after immunodepletion in XEE (Fig. SB). We observed modest reductions of Claspin binding to chromosomes when endogenous Claspin was replaced to MSIM or NSIM, while dSIM showed clearly additive defect of the SIMs on the binding to chromosomes (Fig. SC). In an attempt to equalize the amount of added-back Claspin to that of endogenous Claspin in the Claspin-depleted extract, we produced recombinant proteins of Claspin WT and dSIM mutant by using a yeast expression system. The replacement of endogenous Claspin with recombinant WT Claspin consistently showed its SUMOylation-dependent interaction with mitotic chromosomes. In contrast, the dSIM mutant not only lost robust chromosome association but also no longer displayed SUMOylation dependency (). This finding indicated that the robust chromosomal association of Claspin is mediated via the interaction of its SIMs and SUMOylated proteins. Together with the results from TopoIIα-replacement analysis (), we concluded that the interaction between SUMOylated TopoIIα-CTD and the SIMs of Claspin is required for the robust binding of Claspin with centromeres on mitotic chromosomes.
Figure 6. SIMs on Claspin are required for its SUMOylation-dependent binding to mitotic chromosomes. (A) A schematic of the primary structure of Claspin. The SIMs and Chk1 binding domainsCitation42 are indicated as red and black boxes, respectively. The point mutations in each SIM are red. The residue numbers are indicated on the side. (B) Endogenous Claspin was replaced with either recombinant WT or a dSIM mutant by immunodepletion/add-back. The efficiency of depletion and amount of supplemented recombinant proteins were confirmed by immunoblotting (left panel, Extracts). Mitotic chromosomes from Claspin-replaced XEEs were analyzed by immunoblotting with the indicated antibodies. The SUMOylated form of TopoIIα is indicated by a bracket. (C) A proposed model of the dual function of TopoIIα SUMOylation on mitotic centromeres.
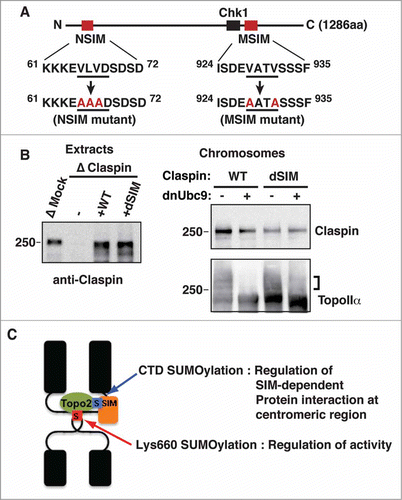
Discussion
Based on our previous and current data, we propose a dual function for TopoIIα SUMOylation in mitotic chromosome segregation: one function is the regulation of TopoIIα activity via SUMOylation on Lys660, and the other function is the recruitment of binding proteins on the centromere through SUMOylation on the CTD () as demonstrated by Claspin in this report. SUMOylation-dependent protein/protein interactions are involved in many nuclear functions.Citation26 The most well-defined example is PML body organization via SUMO/SIM interactions.Citation27 Our recent observation of Daxx relocation from PML to PIAS1-mediated SUMO2/3 foci also suggests that SUMO modification is fundamental to the modulation of protein/protein interactions via the SIM motif.Citation28 The SUMO/SIM-mediated assembly of a protein complex facilitates centromeric composition during mitosis in accordance with the previously proposed mechanism of CENP-E recruitment to the kinetochore.Citation29 Our findings in this report further suggest that TopoII-CTD SUMOylation could contribute to the recruitment of centromeric components during mitosis. The centromeric cohesion phenotype observed in budding yeast with defective TopoII-CTD SUMOylationCitation11 could be mediated by defective SUMO-dependent recruitment of proteins to the centromeres. Multiple proteins interact with SUMOylated TopoIIα-CTD () in addition to Claspin. Therefore, comprehensive identification of these proteins will most likely shed light on the mechanism by which TopoIIα-SUMOylation modulates mitotic centromere functions.
Surprisingly, TopoIIα-CTD SUMOylation recruits Claspin on mitotic centromeres; the best understood role of Claspin to date is its involvement in the regulation of the S-phase checkpoint with stalled DNA replication forks.Citation30,31 Our results suggest a novel mitotic function of Claspin. Claspin binds to Chk1 to mediate S-phase checkpoint activation.Citation32,33 Chk1 is involved in the activation of Aurora B kinase by phosphorylating Aurora B at serine 331Citation34 and that activation is important for resolving merotelic spindle attachment on kinetochores.Citation35 Our findings provide an intriguing hypothesis that SUMOylation may be involved in the Chk1/Aurora B pathway through recruiting the Claspin/Chk1 complex at mitotic centromeres and thus contribute to the regulation of Aurora B-dependent checkpoint activity.
A recent study in budding yeast showed that TopoII mutants, which are defective in different stages of the strand passaging reaction, promote distinct effects on mitotic checkpoint activation.Citation8 This finding suggests that conformational differences in TopoII during the strand passaging reaction could cause distinct consequences for checkpoint activation. Notably, TopoII inhibitors that affect different stages of the TopoII strand passaging reaction showed distinct effects on the SUMOylation of TopoII on mitotic chromosomes.Citation10 Thus, whether the SUMOylation of TopoII could be involved in the strand passage reaction-mediated checkpoint activation via the SUMO/SIM interaction that we found in this study is an intriguing question.
In summary, we have demonstrated functional variations of SUMOylation regarding the modification sites on TopoIIα. The SUMOylation of TopoIIα at Lys660 is responsible for regulating TopoIIα enzymatic activity, and TopoIIα-CTD SUMOylation is responsible for recruiting SIM-containing proteins such as Claspin to the centromeres of mitotic chromosomes.
Materials and Methods
DNA constructs, site-directed mutagenesis, recombinant protein expression and purification, and antibodies
The lysine to arginine substitution of TopoIIα () and the mutations of the SIMs in Claspin () were generated by site-directed mutagenesis using a QuikChangeII XL kit (Agilent) according to the manufacturer's protocol. These mutated cDNAs and WT Claspin cDNA were subcloned into a pPIC3.5 vector that had a calmodulin-binding protein (CBP)-T7 tag sequence.Citation14 An N-terminal 271-amino acid fragment of Claspin was amplified by PCR from full-length Claspin and subcloned into pET28a and pGEX-4T1 vectors. The SENP2 catalytic domain (363–589 aa) was amplified by PCR and subcloned into the pET28a vector. The TopoIIα CTD (1,220–1,579 aa) was amplified from full-length TopoIIα and subcloned into pET28a and pET30a vectors. All of the constructs were verified by DNA sequencing.
The recombinant TopoIIα and Claspin proteins, which were fused to CBP- and T7-tags at their N-termini, were expressed in the GS115 strain of Pichia pastoris yeast and then purified as described previously.Citation14 The E1 complex (Aos1/Uba2 heterodimer), PIASy, Ubc9, dominant-negative form of Ubc9 (dnUbc9-C93S/L97S), and SUMO-2 were expressed in BL21 (DE3) or Rosetta (DE3) bacteria and purified as described previously.Citation17,22 The protein concentrations were measured using the Bradford method (Bio-Rad), with BSA as the standard.
The anti-Claspin antibody was raised against a His6-T7-tagged N-terminal fragment of Claspin (1–271 residues) and affinity-purified through GST fusion of the same fragment. The anti-SUMO2/3 polyclonal antibody, the polyclonal antibody against the TopoIIα C-terminus (1,358–1,579 aa), and the polyclonal antibody against RCC1 were described previously.Citation14,36
In vitro SUMOylation and decatenation assays
The SUMOylation reactions were performed as described previously.Citation17 The samples were separated on 8–16% Tris-HCl gradient gels by SDS-PAGE and analyzed by immunoblotting with HRP-conjugated anti-T7 monoclonal antibody (EMD Millipore). The decatenation assays were performed as described previously.Citation14 After the SUMOylation reaction with recombinant TopoIIα proteins, the reactions were further incubated with 6.2 ng/ml of kDNA (TopoGEN) and terminated by adding one-third volume of 6X DNA dye (30% glycerol, 0.1% SDS, 10 mM EDTA, and 0.2 mg/ml bromophenol blue). The samples were separated on a 1% agarose gel by electrophoresis. The amount of kDNA remaining in the wells was measured using an Image Station 4000R (Carestream Health). The standard error was calculated using KaleidaGraph (Synergy Software).
XEE, pull-down, and immunodepletion/add-back assays
Sperm nuclei and low-speed extracts of X. laevis eggs arrested in metaphase with cytostatic factor (CSF) were prepared following standard protocols.Citation37,38 The immunodepletion was performed as reported previously with protein A–conjugated magnetic beads (Life Technologies).Citation39 For add-back experiments, the purified recombinant proteins were added to the immunodepleted extracts in amounts equivalent to their endogenous concentrations. The chromosome isolation and SUMOylation analysis were performed as described previously.Citation40 The precipitated chromosomes were boiled in SDS-PAGE sample buffer, and the extracted proteins were subjected to immunoblotting with the indicated antibodies.
The XEE pull-down assay was performed as described previouslyCitation41 with 10 mM iodoacetamide supplemented in the buffers to prevent deSUMOylation activity in the XEE. For SENP2-CD elution, the beads were incubated in the dilution buffer (20 mM Na phosphate [pH 7.8], 18 mM b-glycerol phosphate [pH 7.5], 5 mM MgCl2, 50 mM NaCl, and 5% glycerol) containing 35 mg/ml SENP2-CD for 30 minutes at 25°C, and the soluble fractions were isolated using spin columns. SDS-PAGE samples of the fractions were prepared by adding a half volume of 3X SDS-PAGE sample buffer. The SUMO chain pull-down assay was performed with GFP-fused SUMO2x4 protein that was captured on GFP trap magnetic beads (Bulldog Bio Inc..).Citation21 All the samples were separated on 8–16% gradient gels (Life Technologies) by SDS-PAGE and analyzed with silver staining and/or immunoblotting as indicated in .
Immunofluorescence
The mitotic chromosomes used in the immunofluorescence analyses were prepared as described previously.Citation22 For the inhibition of mitotic SUMOylation, 150 ng/ml of a dominant-negative form of Ubc9 (dnUbc9) was added immediately before the induction of mitosis. The antibodies used for immunofluorescence staining were as follows: TopoII (mouse monoclonal, clone AK5 from Enzo Life Sciences), SUMO2/3 (guinea pig polyclonal), and Claspin (rabbit polyclonal). Anti-rabbit Alexa 488, anti-guinea pig Alexa 684, and anti-mouse Alexa 568 (Life Technologies) were used as the secondary antibodies. The DNA was counterstained with Hoechst 33342 (EMD Millipore), and the samples were mounted in Vectashield (Vector Laboratory). The specimens were observed using Volocity Imaging Software (Improvision) on a Nikon TE2000-U microscope with a Plan Apo 100x/1.40 objective, and images were acquired with a Retiga SRV CCD camera (QImaging). Adobe Photoshop and Adobe Illustrator were used to process the obtained images regarding the intensity and size according to the instructions of the journal.
Disclosure of Potential Conflicts of Interest
No potential conflicts of interest were disclosed.
1066537_supplemental_fig_and_caption.zip
Download Zip (340.7 KB)Acknowledgments
We thank for D. Clarke and H. Funabiki for their critical discussion of this project. We thank NPG Language editing for editorial assistance.
Funding
This project was supported by NIH/NIGMS, GM80278 and by bridge funding from the University of Kansas. W.G. Dunphy and A. Kumagai are supported by NIH/NIGMS (GM043974 and GM070891). M. Dasso is supported by NICHD (project no. HD001902).
Supplemental Material
Supplemental data for this article can be accessed on the publisher's website.
References
- Nitiss JL. DNA topoisomerase II and its growing repertoire of biological functions. Nat Rev Cancer 2009; 9:327-37; PMID:19377505; http://dx.doi.org/10.1038/nrc2608
- Porter AC, Farr CJ. Topoisomerase II: untangling its contribution at the centromere. Chromosome Res 2004; 12:569-83; PMID:15289664; http://dx.doi.org/10.1023/B:CHRO.0000036608.91085.d1
- Coelho PA, Queiroz-Machado J, Carmo AM, Moutinho-Pereira S, Maiato H, Sunkel CE. Dual role of topoisomerase II in centromere resolution and aurora B activity. PLoS Biol 2008; 6:e207; PMID:18752348; http://dx.doi.org/10.1371/journal.pbio.0060207
- Clarke DJ, Johnson RT, Downes CS. Topoisomerase II inhibition prevents anaphase chromatid segregation in mammalian cells independently of the generation of DNA strand breaks. J Cell Sci 1993; 105 (Pt 2):563-9; PMID:8408285
- Downes CS, Mullinger AM, Johnson RT. Inhibitors of DNA topoisomerase II prevent chromatid separation in mammalian cells but do not prevent exit from mitosis. Proc Natl Acad Sci U S A 1991; 88:8895-9; PMID:1656458; http://dx.doi.org/10.1073/pnas.88.20.8895
- Shamu CE, Murray AW. Sister chromatid separation in frog egg extracts requires DNA topoisomerase II activity during anaphase. J Cell Biol 1992; 117:921-34; PMID:1315785; http://dx.doi.org/10.1083/jcb.117.5.921
- Wang LH, Schwarzbraun T, Speicher MR, Nigg EA. Persistence of DNA threads in human anaphase cells suggests late completion of sister chromatid decatenation. Chromosoma 2008; 117:123-35; PMID:17989990; http://dx.doi.org/10.1007/s00412-007-0131-7
- Furniss KL, Tsai HJ, Byl JA, Lane AB, Vas AC, Hsu WS, Osheroff N, Clarke DJ. Direct monitoring of the strand passage reaction of DNA topoisomerase II triggers checkpoint activation. PLoS Genet 2013; 9:e1003832; PMID:24098144; http://dx.doi.org/10.1371/journal.pgen.1003832
- Dawlaty MM, Malureanu L, Jeganathan KB, Kao E, Sustmann C, Tahk S, Shuai K, Grosschedl R, van Deursen JM. Resolution of sister centromeres requires RanBP2-mediated SUMOylation of topoisomerase IIalpha. Cell 2008; 133:103-15; PMID:18394993; http://dx.doi.org/10.1016/j.cell.2008.01.045
- Agostinho M, Santos V, Ferreira F, Costa R, Cardoso J, Pinheiro I, Rino J, Jaffray E, Hay RT, Ferreira J. Conjugation of human topoisomerase 2 alpha with small ubiquitin-like modifiers 2/3 in response to topoisomerase inhibitors: cell cycle stage and chromosome domain specificity. Cancer Res 2008; 68:2409-18; PMID:18381449; http://dx.doi.org/10.1158/0008-5472.CAN-07-2092
- Bachant J, Alcasabas A, Blat Y, Kleckner N, Elledge SJ. The SUMO-1 isopeptidase Smt4 is linked to centromeric cohesion through SUMO-1 modification of DNA topoisomerase II. Mol Cell 2002; 9:1169-82; PMID:12086615; http://dx.doi.org/10.1016/S1097-2765(02)00543-9
- Mao Y, Desai SD, Liu LF. SUMO-1 conjugation to human DNA topoisomerase II isozymes. J Biol Chem 2000; 275:26066-73; PMID:10862613; http://dx.doi.org/10.1074/jbc.M001831200
- Azuma Y, Arnaoutov A, Dasso M. SUMO-2/3 regulates topoisomerase II in mitosis. J Cell Biol 2003; 163:477-87; PMID:14597774; http://dx.doi.org/10.1083/jcb.200304088
- Ryu H, Furuta M, Kirkpatrick D, Gygi SP, Azuma Y. PIASy-dependent SUMOylation regulates DNA topoisomerase IIalpha activity. J Cell Biol 2010; 191:783-94; PMID:21079245; http://dx.doi.org/10.1083/jcb.201004033
- Takahashi Y, Yong-Gonzalez V, Kikuchi Y, Strunnikov A. SIZ1/SIZ2 control of chromosome transmission fidelity is mediated by the sumoylation of topoisomerase II. Genetics 2006; 172:783-94; PMID:16204216; http://dx.doi.org/10.1534/genetics.105.047167
- Dickey JS, Osheroff N. Impact of the C-terminal domain of topoisomerase IIalpha on the DNA cleavage activity of the human enzyme. Biochemistry 2005; 44:11546-54; PMID:16114891; http://dx.doi.org/10.1021/bi050811l
- Ryu H, Al-Ani G, Deckert K, Kirkpatrick D, Gygi SP, Dasso M, Azuma Y. PIASy mediates SUMO-2/3 conjugation of poly(ADP-ribose) polymerase 1 (PARP1) on mitotic chromosomes. J Biol Chem 2010; 285:14415-23; PMID:20228053; http://dx.doi.org/10.1074/jbc.M109.074583
- Ren J, Gao X, Jin C, Zhu M, Wang X, Shaw A, Wen L, Yao X, Xue Y. Systematic study of protein sumoylation: Development of a site-specific predictor of SUMOsp 2.0. Proteomics 2009; 9:3409-12; http://dx.doi.org/10.1002/pmic.200800646
- Gareau JR, Lima CD. The SUMO pathway: emerging mechanisms that shape specificity, conjugation and recognition. Nat Rev Mol Cell Biol 2010; 11:861-71; PMID:21102611; http://dx.doi.org/10.1038/nrm3011
- Reverter D, Lima CD. Structural basis for SENP2 protease interactions with SUMO precursors and conjugated substrates. Nat Struct Mol Biol 2006; 13:1060-8; PMID:17099700; http://dx.doi.org/10.1038/nsmb1168
- Sridharan V, Park H, Ryu H, Azuma Y. SUMOylation regulates polo-like kinase 1-interacting checkpoint helicase (PICH) during mitosis. J Biol Chem 2015; 290:3269-76; PMID:25564610; http://dx.doi.org/10.1074/jbc.C114.601906
- Azuma Y, Arnaoutov A, Anan T, Dasso M. PIASy mediates SUMO-2 conjugation of Topoisomerase-II on mitotic chromosomes. EMBO J 2005; 24:2172-82; PMID:15933717; http://dx.doi.org/10.1038/sj.emboj.7600700
- Hecker CM, Rabiller M, Haglund K, Bayer P, Dikic I. Specification of SUMO1- and SUMO2-interacting motifs. J Biol Chem 2006; 281:16117-27; PMID:16524884; http://dx.doi.org/10.1074/jbc.M512757200
- Song J, Durrin LK, Wilkinson TA, Krontiris TG, Chen Y. Identification of a SUMO-binding motif that recognizes SUMO-modified proteins. Proc Natl Acad Sci U S A 2004; 101:14373-8; PMID:15388847; http://dx.doi.org/10.1073/pnas.0403498101
- Errico A, Costanzo V, Hunt T. Tipin is required for stalled replication forks to resume DNA replication after removal of aphidicolin in Xenopus egg extracts. Proc Natl Acad Sci U S A 2007; 104:14929-34; PMID:17846426; http://dx.doi.org/10.1073/pnas.0706347104
- Heun P. SUMOrganization of the nucleus. Curr Opin Cell Biol 2007; 19:350-5; PMID:17467254; http://dx.doi.org/10.1016/j.ceb.2007.04.014
- Matunis MJ, Zhang XD, Ellis NA. SUMO: the glue that binds. Dev Cell 2006; 11:596-7; PMID:17084352; http://dx.doi.org/10.1016/j.devcel.2006.10.011
- Sudharsan R, Azuma Y. The SUMO ligase PIAS1 regulates UV-induced apoptosis by recruiting Daxx to SUMOylated foci. J Cell Sci 2012; 125:5819-29; PMID:22976298; http://dx.doi.org/10.1242/jcs.110825
- Zhang XD, Goeres J, Zhang H, Yen TJ, Porter AC, Matunis MJ. SUMO-2/3 modification and binding regulate the association of CENP-E with kinetochores and progression through mitosis. Mol Cell 2008; 29:729-41; PMID:18374647; http://dx.doi.org/10.1016/j.molcel.2008.01.013
- Lou H, Komata M, Katou Y, Guan Z, Reis CC, Budd M, Shirahige K, Campbell JL. Mrc1 and DNA polymerase epsilon function together in linking DNA replication and the S phase checkpoint. Mol Cell 2008; 32:106-17; PMID:18851837; http://dx.doi.org/10.1016/j.molcel.2008.08.020
- Lee J, Gold DA, Shevchenko A, Dunphy WG. Roles of replication fork-interacting and Chk1-activating domains from Claspin in a DNA replication checkpoint response. Mol Biol Cell 2005; 16:5269-82; PMID:16148040; http://dx.doi.org/10.1091/mbc.E05-07-0671
- Kumagai A, Dunphy WG. Claspin, a novel protein required for the activation of Chk1 during a DNA replication checkpoint response in Xenopus egg extracts. Mol Cell 2000; 6:839-49; PMID:11090622; http://dx.doi.org/10.1016/S1097-2765(05)00092-4
- Chini CC, Chen J. Human claspin is required for replication checkpoint control. J Biol Chem 2003; 278:30057-62; PMID:12766152; http://dx.doi.org/10.1074/jbc.M301136200
- Petsalaki E, Akoumianaki T, Black EJ, Gillespie DA, Zachos G. Phosphorylation at serine 331 is required for Aurora B activation. J Cell Biol 2011; 195:449-66; PMID:22024163; http://dx.doi.org/10.1083/jcb.201104023
- Petsalaki E, Zachos G. Chk1 and Mps1 jointly regulate correction of merotelic kinetochore attachments. J Cell Sci 2013; 126:1235-46; PMID:23321637; http://dx.doi.org/10.1242/jcs.119677
- Dasso M, Nishitani H, Kornbluth S, Nishimoto T, Newport JW. RCC1, a regulator of mitosis, is essential for DNA replication. Mol Cell Biol 1992; 12:3337-45; PMID:1630449
- Kornbluth S, Yang J, Powers M. Analysis of the Cell Cycle Using Xenopus Egg Extracts. In: M Yamada K, ed. Current Protocols in Cell Biology. New York, NY: John Wiley & Sons, Inc., 2001; 11:1-3.
- Murray AW. Cell cycle extracts. Methods Cell Biol 1991; 36:581-605; PMID:1839804; http://dx.doi.org/10.1016/S0091-679X(08)60298-8
- Arnaoutov A, Dasso M. The Ran GTPase regulates kinetochore function. Dev Cell 2003; 5:99-111; PMID:12852855; http://dx.doi.org/10.1016/S1534-5807(03)00194-1
- Azuma Y. Analysis of SUMOylation of topoisomerase IIalpha with Xenopus egg extracts. Methods Mol Biol 2009; 582:221-31; PMID:19763953; http://dx.doi.org/10.1007/978-1-60761-340-4_17
- Ryu H, Azuma Y. Rod/Zw10 complex is required for PIASy-dependent centromeric SUMOylation. J Biol Chem 2010; 285:32576-85; PMID:20696768; http://dx.doi.org/10.1074/jbc.M110.153817
- Kumagai A, Dunphy WG. Repeated phosphopeptide motifs in Claspin mediate the regulated binding of Chk1. Nat Cell Biol 2003; 5:161-5; PMID:12545175; http://dx.doi.org/10.1038/ncb921