Abstract
Primordial germ cells (PGCs) are founder cells of the germ cell lineage, and can be differentiated from stem cells in an induced system in vitro. However, the induction conditions need to be optimized in order to improve the differentiation efficiency. Activin A (ActA) is a member of the TGF-β super family and plays an important role in oogenesis and folliculogenesis. In the present study, we found that ActA promoted PGC-like cells (PGCLCs) formation from mouse skin-derived stem cells (SDSCs) in both embryoid body-like structure (EBLS) differentiation and the co-culture stage in a dose dependent manner. ActA treatment (100 ng/ml) during EBLS differentiation stage and further co-cultured for 6 days without ActA significantly increased PGCLCs from 53.2% to 82.8%, and as well as EBLS differentiation without ActA followed by co-cultured with 100 ng/ml ActA for 4 to 12 days with the percentage of PGCLCs increasing markedly in vitro. Moreover, mice treated with ActA at 100 ng/kg body weight from embryonic day (E) 5.5–12.5 led to more PGCs formation. However, the stimulating effects of ActA were interrupted by Smad3 RNAi, and in an in vitro cultured Smad3−/− mouse skin cells scenario. SMAD3 is thus likely a key effecter molecule in the ActA signaling pathway. In addition, we found that the expression of some epiblast cell markers, Fgf5, Dnmt3a, Dnmt3b and Wnt3, was increased in EBLSs cultured for 4 days or PGCLCs co-cultured for 12 days with ActA treatment. Interestingly, at 16 days of differentiation, the percentage of PGCLCs was decreased in the presence of ActA, but the expression of meiosis-relative genes, such as Stra8, Dmc1, Sycp3 and Sycp1, was increased. In conclusion, our data here demonstrated that ActA can promote PGCLC formation from SDSCs in vitro, at early stages of differentiation, and affect meiotic initiation of PGCLCs in later stages
Introduction
Primordial germ cells (PGCs) are founder cells of the germ cell lineage, which is induced in the epiblasts around embryonic day (E) 6.0 by bone morphogenetic protein 2 and 4 (BMP2 and BMP4) signaling from the extra embryonic ectoderm.Citation1-7 However, mechanisms underlying PGC development is limited. In recent years, great efforts have been made to induce germ cell formation from stem cells in vitro, which will further our understanding of the process of germ cell development. Embryonic stem cells (ESCs), induced pluripotent stem cells (iPSCs) and adult stem cells, such as skin-derived stem cells (SDSCs) and pancreatic stem cells, were used for germ cell induction in vitro from mice,Citation8-16 pig,Citation17 and humans.Citation18-20 SDSCs were shown to be capable of differentiating into different cell types including adipocytes and insulin producing cells in vitro.Citation21 Particularly, it has been reported that SDSCs isolated from fetal porcine or newborn mouse skin can be differentiated into germ cells or granulosa cells.Citation10,17,22-24 Due to its easy access and the differentiation proficiency, SDSCs are thought to be an ideal source for PGC-like cells (PGCLCs) induction in vitro.
Activin A (ActA), a member of the transforming growth factor β (TGF-β) super-family, is produced in the ovary and other organs, Citation25-27 and is considered to be an important modulator of follicle development by promoting granulosa cell proliferation Citation28-29 mediated by SMAD2/3.Citation30 ActA also contributes to germ cell proliferation and survival during the formation of primordial follicles.Citation31-32 In addition, ActA plays an important role in mouse oocyte maturation by promoting the meiosis in vitro.Citation33 This is supported by the finding that ActA can promote meiotic initiation in female germ cells in mice, and regulate spermatogenic cell and sertoli cell proliferation and differentiation, along with its functions in germ cell development in vivo and in vitro.Citation34,35 ActA is thus considered to be an important factor for PGCLC differentiation and development in vitro, as well as meiotic entry.
In this study, we induced PGCLCs from SDSCs of newborn mice in differentiation medium containing ActA, and optimized the concentration to increase the induction efficiency in vitro. Initially we confirmed that ActA promotes PGC formation in vivo by treating mice during the PGC specification period at E 5.5–6.0 to E 12.5, which provided us new insights into the functions of ActA in germ cell development. In addition, ActA can promote PGCLCs formation in our in vitro differentiation system with an applicable dosage, which is significant for improving PGCLCs induction efficiency. Moreover, in our induction system, SDSCs can be induced directly to form embryoid body-like structures (EBLSs) and further differentiate into PGCLCs without iPSCs reprograming. One of the more difficult aspects of inducing competent germ cells differentiation from stem cells in vitro is initiating meiosis. Here, we also investigated the ability of ActA to induce meiotic entry, and found that ActA likely promotes meiotic entry via regulating meiotic gene expression.
Results
PGCLCs formation from cultured SDSCs
Skin-derived stem cells were isolated from new born GFP transgenic or wild type mouse skin and cultured in an in vitro system (). Undifferentiated skin cells, non-adherent spheres were cultured for 2 generations, and then, were dissociated and plated in differentiation medium to induce EBLS formation (). A few PGCLCs appeared during this stage. Then, cells of EBLSs were co-cultured with mouse embryonic fibroblast (MEF) feeder cells for 4 days, 8 days or 12 days to differentiate and proliferate, and some round cells appeared around 6 to 8 days (). These cells derived from GFP transgenic mice expressing GFP with CAG promoter during co-culture stage and indicated that they were differentiated from skin cells (, Fig. S1A). For skin cell derived EBLSs, we confirmed its potential to differentiate into 3 layers cells, including neural epithelium (ectoderm), adipose tissue (mesoderm) and glandular tissue (endoderm) (Fig. S1B).
Figure 1. Skin-derived stem cells (SDSCs) can be induced into primordial germ cell-like cells (PGCLCs). (A). Schematic diagram of the experiments. Different concentrations of Activin A (ActA) was added at the embryoid body-like structure (EBLS) differentiation stage or the co-cultured stage. (B) SDSCs were isolated and cultured in a suspension culture system and passaged for 2 generations. Non-adherent spheres (a) were formed with GFP fluorescence (a’). These cells were cultured in a differentiation medium to form EBLSs (b). (c–e) cells of 4 days in EBLSs were isolated and co-cultured with MEF feeder cells for 4, 8 and 12 days. (f) The round PGCLCs in suspension appeared at day 12.
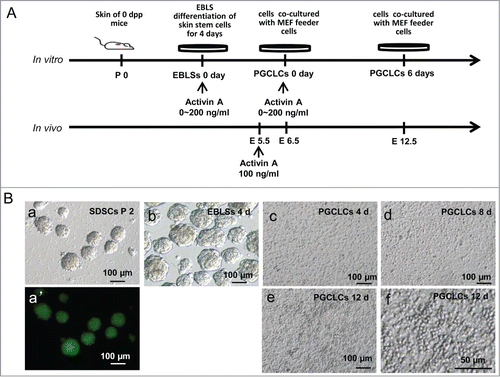
These round cells growing in suspension were collected to identify PGC characteristics. It was shown that these cells expressed germ cell markers STELLA, MVH and DAZL (Fig. S2A). SSEA-1 positive cells sorted by miniMACS also expressed STELLA, DAZL and MVH (). Meanwhile, the PGC markers such as SSEA-1, MVH and STELLA were expressed weakly in SDSCs passed 2 generations (Fig. S2B). The expression level of pluripotency marker Oct4 in SDSCs and EBLSs at 4 days is relative low but increased after 15 days following co-culture with feeder cells. The early PGC markers Fragllis and Stella were also expressed increasingly higher in cells following 15 days co-culture with MEF. Figla, Nobox and Dazl, usually expressed at the late stage of PGC development, were increased in cells cultured for 15 days (), suggesting that SDSCs have the potential to be induced into EB-like cell (EBLCs) and further to be transdifferentiated into PGCLCs that show PGC characteristics.
Epigenetic modification of PGCLCs induced in vitro
PGCs undergo unique epigenetic changes during their development. These epigenetic changes play important roles in PGC-specific gene expression, reprogramming of imprinted genes, and may be necessary for germ cells to acquire totipotency. We evaluated the epigenetic modifications of PGCLCs and compared that with SDSCs, at EBLS day 4, and E 12.5 PGCs. Immunofluorescence analysis revealed that the SSEA-1 positive PGCLCs sorted by miniMACS at day 6 appeared to have reduced cytosine methylation (5mC) and elevated H3K27me3 levels compared with SDSCs and EBLCs, which were just similar to their in vivo E 12.5 PGCs counterparts (). We also determined the level of 5-hydroxymethylcytosine (5hmC), and found that it was increased in PGCLCs differentiated for 6 days when compared with SDSCs and EBLCs, and these dynamic 5hmC changes during PGCLC formation are in accordance with those observed during PGC formation in vivo ().
Figure 3. Epigenetic modification of PGCLCs. Immunofluorescence of (A) 5mC, (B) 5hmC and (C) H3K27me3 in SDSCs passaged 2 generations, EBLCs, PGCLCs co-cultured for 6 days and E 12.5 PGCs as a control. (D) The statistics of fluorescence intensity (gray value) of immunofluorescence staining. The expression level of 5mC decreased from SDSCs to PGCLCs, which was similar to PGC development in vivo, and was low in PGCLCs as well as PGCs. Conversely, 5hmC and H3K27me3 increased during the differentiation process, and the expression profile was in accordance with E 12.5 PGCs.
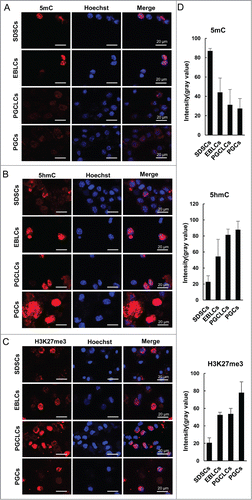
ActA promotes PGCLCs formation in vitro in different induction stages
During EBLS differentiation, a few PGC precursors are specified. To test the effects of different ActA concentrations on PGCLC differentiation at this stage, a final concentration of 0 – 200 ng/ml of ActA was added during EBLS stage, and then EBLCs were continued to co-cultured with MEF feeder and without ActA supplementation for another 6 days (). The percentage of SSEA-1/GFP double-positive putative PGCLCs increased obviously with the increase of ActA concentration from 0 to 100 ng/ml, but did not increase further at higher concentrations (). Thus our data indicates that ActA can promote PGCLCs specification during EBLS differentiation in a dose dependent manner, reaching maximal effect at the concentration of 100 ng/ml.
Figure 4. ActA promotes PGCLC formation. (A) Different concentrations of ActA was added during EBLS differentiation. Suspended and round cells increased with increasing ActA concentration in the EBLS differentiation stage and the co-cultured for 6 days without ActA addition. (B) PGCLCs co-cultured with MEFs for 4 to 16 days. Suspended cell numbers increased with 0 to 100 ng/ml ActA supplementation from 4 to 12 days, but decreased at 16 days. (C, D) FACS analysis of the percentage of SSEA-1 positive cells during EBLS differentiation with different ActA concentrations. (E, F) FACS analysis of the percentage of SSEA-1 positive cells during PGCLCs co-cultured with different ActA concentrations.
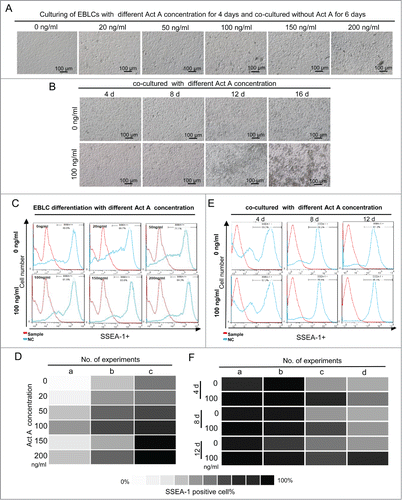
During co-culturing of differentiated EBLCs from day 4 to day 12, the percentage of SSEA-1/GFP double-positive putative PGCLCs also increased with ActA presence in a dose dependent manner from 0 to 100 ng/ml (), but not further increased with 200 ng/ml (data not shown). It is interesting that when further culturing EBLCs with different concentrations of ActA to 16 days, the percentage of PGCLCs decreased with higher ActA doses (). When co-cultured with MEFs feeder in PGC culture medium, PGCLCs underwent generation and proliferation. It seems that ActA plays an important role in this process. Meanwhile, it is worth noting that the dosage of ActA is also a key effect factor, and 100 ng/ml was an optimized concentration in vitro.
ActA could promote PGCLC formation during EBLS differentiation, but whether these PGCLCs have classical PGC characteristics remains to be determinated. Immunofluorescence staining of the PGC markers, SSEA-1 and STELLA showed that they were expressed in the GFP positive cell population, and the percentage of germ cell marker and GFP double positive cells significantly increased at 100 ng/ml ActA comparing to the control group, with a 1.5 folds and 0.7 fold increase, respectively (Fig. S3A and B). Thus these SSEA-1 or STELLA positive cells are differentiated from GFP positive SDSCs to cells expressing key markers of early PGCs, suggesting that ActA plays an important role in PGCLC formation. Moreover, the percentage of SSEA-1 and MVH double-positive PGCLCs differentiated from SDSCs was increased from 37.6% to 61.9% under the impact of ActA ().
Figure 5. Immunofluorescence staining for MVH and SSEA-1 markers used for characterizing transdifferentiated PGCLCs. (A) The expression of MVH and SSEA-1 in PGCLCs co-cultured for 6 days with or without Act A, E 12.5 PGCs and SDSCs. And (B) the percentage of MVH/SSEA-1 double-positive cells was increased with 100 ng/ml ActA treatment compared with 0 ng/ml. **P < 0.01.
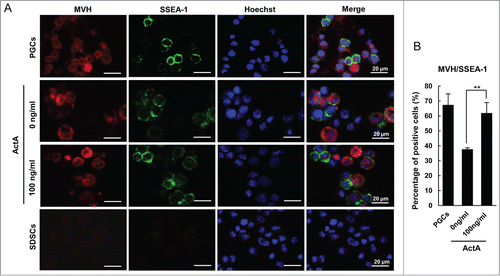
Based on these data, we found that ActA has remarkable effects on PGCLCs formation when inducted in vitro, however, how it play a role is not clear. To verify this, we analyzed the cell cycle of PGCLCs by FACS and found that, after co-cultured for 8, 10 and 12 days, the cell cycle had no obvious differences between 0 and 100 ng/ml ActA (Fig. S4A and B). That suggests cell cycle is not changed by ActA treatment.
ActA promotes PGC generation in vivo
To further study whether ActA has the same effects on PGC development in vivo, we treated pregnant mice at E 5.5 with ActA at 100 ng/kg body weight by tail vein injection, and then genital ridges were isolated at E 12.5 for analysis every 2 days according to the half-life of ActA. Immunofluorescence staining of ACTRI and p-SAMD3 of E 12.5 genital ridge showed that exogenous ActA up-regulated the expression of ACTRI and activated its classical downstream factor, p-SMAD3 in E 12.5 fetal genital ridge (Fig. S5A-C). Meanwhile, the results of western blotting also showed that ACTRI and p-SAMD3 were up-expressed in E 12.5 genital ridge (Fig. S5D). Therefore, we considered that maternal tail-vein ActA injection can effectively impact on the embryonic genital ridge cells. Based on this, immunofluorescence staining of OCT4 of E 12.5 genital ridge cells showed that positive cells significantly increased after ActA treatment with a 0.9 fold increasing compared to the negative control (). In addition, immunofluorescence analysis of genital ridges using a SSEA-1 antibody showed that SSEA-1 positive PGCs were nearly doubled after ActA treatment ().
Figure 6. ActA promotes PGC generation in vivo. (A) Immunofluorescence of OCT4 in E 12.5 genital ridge cells. Pregnant mice were treated with 100 ng/kg ActA by tail vein injection from E 5.5 to E 11.5. The genital ridges were isolated at E 12.5 and digested into single cells. (B) The percentage of OCT4 positive cell increased by ActA treatment. (*P < 0.05). (C) Immunofluorescence of SSEA-1 in E 12.5 genital ridges. (D) The average number of SSEA-1 positive cells in one section also increased by ActA treatment. *P < 0.05.
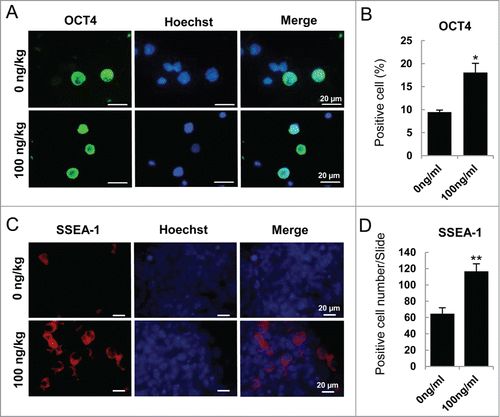
Proximal epiblast cells respond to signals from the extra embryonic tissues at around E 5.5, and form PGC precursor cells at around E 6.5. Followed this, these precursor cells undergo specification and proliferation until E 12.5.Citation4 Thus, we choose this period to give an exogenous ActA treatment, and found that exogenous ActA also can improve PGC numbers effectively.
Effects of ActA on dedifferentiation of SDSCs to epiblast-like cells and epigenetic reprogramming
Recent research reported that PGCLCs differentiated from mESC or iPSCs in vitro undergo dedifferentiation into a epiblast-like cell (EpiLC) stage, a cellular state highly similar to pregastrulation epiblasts, which have a high competence for the PGC fate.Citation8 We next analyzed the expression of epiblast marker genes, Fgf2, Dnmt3a, Dnmt3b and Wnt3, and found that Fgf2, Dnmt3a and Dnmt3b increased with ActA treatment, especially in PGCLCs co-cultured for 6 days. Wnt3 increased more significantly in EBLS stage when differentiated with ActA (). Since Wnt3 in the epiblast ensures its responsiveness to Bmp4, and is required for competence for the PGC fate.Citation36 It implies that PGCLCs differentiation in vitro needs to undergo dedifferentiation into EpiLCs, and ActA promotes PGCLC formation by promoting this process and enhances the ability of these EpiLCs to response Bmp4 by upregulating Wnt3 level.
Recent studies have demonstrated that TET family proteins have potential roles in epigenetic regulation during germ cell reprogramming and meiotic entry of early germ cells through modification of 5mC to 5hmC,Citation37-40 an important member of the TET family is TET1 which catalyzes the conversion of 5mC to 5hmC during PGC reprogramming.Citation41 We thus evaluated the expression levels of TET1 at different culture stages. Treatment with 100 ng/ml ActA during EBLS differentiation or PGC co-culture led to higher TET1 levels, especially during the PGCLC co-culture stage, which is in accordance with high expression levels in E 12.5 PGCs (). TET1 is express-ed in ESCs and is involved in stem cell maintenance or differentiation, so the expression level of TET1 in E 5.5 epiblast cells and new born murine SDSCs is also relatively high. Our data indicated that ActA may have an influence on gene expression during PGCLC development in vitro and make it more close to that of in vivo.
Figure 7. The expression of epiblast marker genes and epigenetic modification key gene TET1. (A) Q-PCR analysis of epiblast marker genes, Dnmt3a, Dnmt3b, Wnt3 and Fgf5. Cells from SDSCs, E 5.5 and E 12.5 were analyzed and E 5.5 and E 12.5 were used as epiblast control and PGCs control, respectively. ActA promoted epiblast marker genes expression both in EBLS differentiation and the co-culture stage. But, Wnt3 was not upregulated in 6 day PGCLCs whether treated with ActA or not. (B) Q-PCR analysis of TET1. The expression level was high in E 12.5 PGCs and SDSCs. In cells differentiated in vitro, its mRNA level was relatively low compared to that in vivo. But ActA treatment resulted in an elevating TET1 expression level.
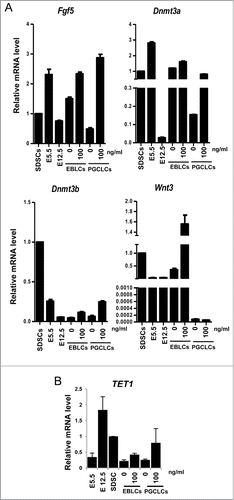
ActA influences PGCLCs generation via the SMAD3 signaling pathway
In different PGCLC induction stages, treatment with ActA could up-regulate the protein level of receptor Activin type II (ACTRII) (Fig. S6A), showing that the Activin pathway was activated. As a member of the TGF-β superfamily, ActA signals mainly through a ligand-specific, receptor associated SMAD pathway and SMAD3 is a key signaling component of it. Citation42 It is thus necessary to confirm if ActA has an influence on PGCLC generation in vitro through SMAD3.
Cells were treated with 100 ng/ml ActA for 10 days, and transfected with siRNA that specifically targets Smad3, or nonsense siRNA as negative control and cultured without RNAi as a control to eliminate the influence of RNAi system on cell culture, and were continually cultured for 2 days (). The protein level of SMAD3 was down-regulated (). The percentage of SSEA-1/GFP double-positive PGCLCs decreased to some extent (). Cell cycle proteins, CCND1 and CDK2, marker genes of proliferation, decreased significantly after Smad3 RNAi (). It seemed that Smad3 knockdown could restrain the effect of ActA on PGCLCs generation.
Figure 8. Positive effects of ActA were repressed by Smad3 RNAi. (A) Treating cells with 100 ng/ml ActA for 10 days and then adding siRNA that specifically targets to Smad3, or nonsense siRNA as negative control and cultured without RNAi as a control to eliminate the influence of RNAi system on cell culture, and continue culturing for 2 days. (B) The protein level of SMAD3 is downregulated by RNAi. (C and D) FACS analysis of SSEA-1/GFP double-positive cells 48 hrs after Smad3 RNAi. (E) Cell cycle genes, CCND1 and CDK2, the marker gene of proliferation, decreased significantly by Smad3 RNAi. n = 3. *P < 0.05.
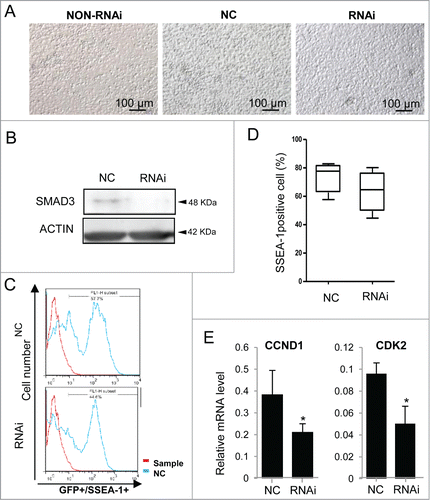
We next used a Smad3 knock-out mouse model to verify our finding that ActA promotes PGCLCs generation mediated by the SMAD3 signaling pathway. Smad3 null mice have little egg ovulated due to abnormal folliculogenesis rather than gametogenesis.Citation43 Therefore, Smad3 knockout has no effect on PGC development in vivo. Based on this, SDSCs derived from Smad3−/− mice had capable of differentiation into PGCLCs, and were induced into PGCLCs with or without ActA for 12 days (). The percentage of SSEA-1 positive cells treated with ActA has no obviously differences to that in the non-treatment group (). The expression levels of PGC markers, Stella, Oct4 and Dazl did not increase significantly after ActA treatment (). Therefore the effects of ActA on PGCLCs differentiation was not evident in the absence of Smad3. PGCLCs differentiation in vitro was thought to undergo an EpiLCs stage, so some epiblast markers were identified by Q-PCR and shown that the expression of Wnt3, Fgf5 and Dnmt3a by ActA treatment was similar to that in the control group (). Based on these observations, Smad3 may be a key downstream signal of ActA involved in promoting PGCLC generation.
Figure 9. ActA has no effect on PGCLCs formation from Smad3 knockout mouse SDSCs. (A) Co-cultured of PGCLCs that derived from Smad3 knockout mouse SDSCs with 0 or 100 ng/ml ActA for 12 days. (B and C) FACS analysis of SSEA-1 positive cells. Treatment with ActA has no obvious effects on PGCLC formation. (D) Expression levels of PGC markers, Stella, Oct4 and Dazl did not increase obviously with ActA treatment compared with control. n = 3. (E) Expression levels of epiblast markers Wnt3, Fgf5 and Dnmt3a did not increase obviously with 100 ng/ml ActA treatment in PGCLCs that derived from Smad3 knockout mouse SDSC and co-cultured for 12 days compared with control. n = 3.
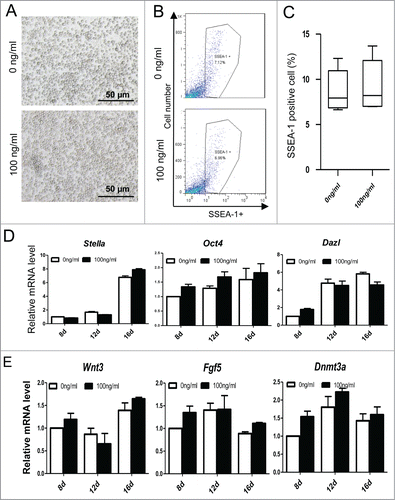
ActA promotes some meiosis genes expression and meiotic initiation in vitro
To determine the effects of ActA on PGCLCs formation in vitro, we used different ActA concentrations from 0 to 200 ng/ml during the co-culture period. The percentage of PGCLCs increased with increasing ActA dose up to 12 days of treatment, but from days 12 to 16, the percentage of PGCLCs decreased in a dose dependent manner ( and Fig. S6B). Interestingly, expression levels of some meiotic genes, Stra8, Scp3, Sycp1 and Dmc1, were relatively low from 8 to 12 days, but increased markedly from days 12 to day 16 in the presence of 100 ng/ml ActA, when compared to that of the non-treatment group (). Meanwhile, at 16 days, levels of these 4 genes showed a correlation with the ActA concentration. It showed that their expression levels increased with ActA concentration from 0 to 100 ng/ml, and reaching a maximal level at 100 ng/ml ActA, while decreasing at a higher concentration of 200 ng/ml (). As an important meiotic protein, Stra8 is elevated markedly during meiosis initiation and previous studies also demonstrated that RA plays an important role on meiosis initiation via activating the expression of Stra8.Citation44-48 Hence, we tried to determinate the expression patterns of the upstream Stra8, RA receptor RAR γ, RAR α and RAR β, and results showed that RAR γ and RAR β were upregulated significantly by ActA treatment at day 16, and RAR α was up-regulated at 12 or 16 days with 100 ng/ml ActA (). Our data show that during later stage of co-culture, ActA may have an effect on PGCLCs further development in vitro and meiosis initiation.
Figure 10. ActA promotes meiotic initiation of PGCLCs in vitro at a later induction stage. (A) Before 12 days culture, the PGCLCs percentage increased with increasing ActA dose, but from 12 to 16 days the percentage of PGCLCs was reduced in a dose dependent manner. (B) Q-PCR analysis of meiosis genes in PGCLCs cultured for 8 days, 12 days and 16 days. It shows that Scp3, Stra8, Dmc1 and Sycp1 increased from 12 days to 16 days with 100 ng/ml ActA present. (C) Q-PCR analysis of meiosis genes of PGCLCs cultured or 16 days with different ActA concentrations. Expression levels of these 4 genes increased from 0 ng/ml to 100 ng/ml but decreased with 200 ng/ml. So 100 ng/ml ActA has the most obvious effects on meiosis gene expression and meiotic entry. (D) Q-PCR analysis of RA receptors. RAR α, and RAR γ were determined and elevated markedly at 16 days with 100 ng/ml ActA, and RAR β was up-regulated to a different extent at 8 days, 12 days and 16 days with 100 ng/ml ActA. Data of day 8 with 0 ng/ml ActA was normalized as 1, n = 3. * P < 0.05.
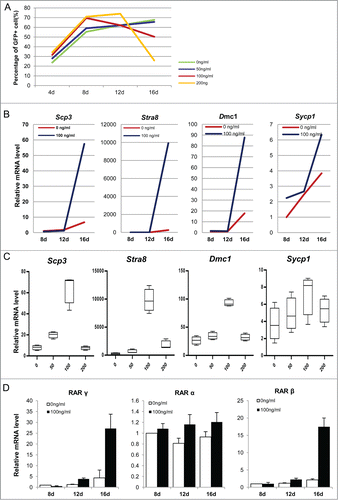
Discussion
In recent years, many efforts were made to induce PGC formation in vitro from pluripotent stem cells, including ESCs, and iPSCs in humans or mouse models. It has been shown cells resembling PGCs can be differentiated from stem cells in vitro.Citation8,16 Skin-derived multipotent stem cells are capable of differentiating into neural cells and adipocytes in mice and pigs.Citation21,49 It indicates that some stem cells in the skin have multiple differentiation potentials. It also has been reported that cells isolated from fetal skin can be cultured in vitro to proliferate as non-adherent spheres in mice.Citation10 or pigs.Citation17 and a subpopulation of these cells possess multi-lineage differentiation potential, as clonal populations expanded from individual cells were able to form neuron-, astrocyte-, and adipocyte-like cells upon induced differentiation.Citation49 A subpopulation of these SDSCs are capable of germ cell specification and can differentiate into PGCLCsCitation17 or even oocyte-like cells (OLCs) when cultured in medium containing follicular fluid and fetal bovine serum.Citation22 More recently, cells derived from human skin were shown to be induced into PGCLCs through iPSCs stages.Citation20 This shows that SDSCs have the capability of differentiation into PGCs in an in vitro induction system. In the current investigation, we found that cells derived from newborn mouse skin have the potential to transdifferentiate into PGCLCs which express key PGC markers, and present similar dynamic changes in epigenetic modulation to that of developing PGCs in vivo. Thus, SDSCs could be one of the possible sources of PGC induction in vitro.
Differentiation of ESCs generally occurs after EBLS formation in suspension culturing in EB medium, and form 3-dimensional cell aggregates comprising the 3 embryonic layers.Citation50 As a kind of adult stem cells, amniotic fluid stem (AFS) cells harbor high differentiation potential and can form EB by cultivating in suspension in vitro, which express 3 germ layer markers in ESC-derived EB.Citation51,52 So, the formation of 3-dimensional aggregates known as EBs maybe a principal step during the differentiation of pluripotent stem cells. In this study, SDSCs were induced to differentiate into PGCLCs through an intermediate step of EBLS formation by culturing in suspension. During this stage, SDSC can form aggregates automatically, and obtain competent to differentiate into 3 germ layers, that is similar with ES-derived EBs. And based on our data, we speculated that SDSCs transdifferentiated into PGCLCs through a EpiLCs stage, which might be happened during EBLS culture stage. It worth to note that, ESCs can be differentiated into EBs comprising the 3 embryonic germ layers due to their pluripotency, but the differentiation potential of SDSCs is limited. Even so, we cultured SDSCs in EB medium to form cell aggregates that have potential to differentiate into 3 germ layers, which is similar with ESC-and AFSCs-derived EBs, despite the differences of ESC- and SDSCs-derived EBs on some aspects, such as epigenetics, transcriptome or proteome, might be exist.
Activin is one of TGF-β family members with major roles in folliculogenesis.Citation53,54 Members of this family have important roles in proliferation, differentiation, adhesion, motility and apoptosis. Many are involved in embryonic development as well as in adult tissue homeostasis.Citation55 Activin is present in the developing human fetal ovary, and may contribute to the regulation of germ cell survival and proliferation.Citation32,55 Some researchers suggested that TGFβ and ActA inhibit proliferation and promote the migration of PGCs in vitro and in vivo.Citation56,57 ActA and TGFβ have a role on pre- or post-migrated PGC proliferation and survival, and this is mainly mediated by the SMAD2/SMAD3 signaling pathway.Citation30 However, it is not clear if ActA has a role in PGC formation during early embryo development. In the current study, we found that ActA has prominent effects on the PGCLCs formation during induction of SDSCs in vitro both in EBLC differentiation and co-culture stages with MEF cells. This finding is further confirmed by our data that it also promotes PGC formation in vivo. Pregnant mice treated with ActA at E 5.5, the time window that the PGC precursors form from the pluripotent proximal epiblast cells in response to BMP signaling,Citation4 had a significantly higher number of PGCs. This finding suggests that in early embryonic development, ActA can promote epiblast differentiation and further have a role in germ cell fate determination. This provides a basis for PGCLCs induction in vitro with ActA.
ActA also has an important role in germ layer differentiation. The most common finding was that ActA can promote the formation of definitive endoderm in a defined differentiation medium, and it works with other proteins such as BMP4, a very important factor in germ cell fate determination during early embryonic development.Citation58,59 In human, ActA plus BMP4 strongly increased germ cell differentiation of hESC.Citation60 It was in accord with our finding that EBLC differentiation with BMP4 increased PGCLCs formation by ActA supplement. Even in co-cultured stage, in which no BMP4 was added, but ActA still promoted PGCLC generation. It suggested that BMP4 has a key role in layer differentiation and PGC fate determination, and ActA works on that basis, to promote epiblast development and germ cell differentiation. In mice, germ cell fate is induced in the epiblast at around E 6.0 by BMP4 signaling from the extra embryonic ectoderm.Citation1 So induction of germ cell fate in vitro might be possible if EpiLCs could be generated in vitro. Interestingly, it was reported that differentiation into EpiLCs was required for ESCs or iPSCs to differentiate into PGCLCs in vitro.Citation8 We have determined levels of some epiblast markers and found that, by treating with ActA, Fgf5, Dnmt3a and Dnmt3b increased, although Dnmt3b was relatively low compared with E 5.5 epiblast cells. In addition, Wnt3 was elevated by treated with ActA during EBLC differentiation. Also, OCT4 positive cells increased nearly 2 times compared to control. Therefore, ActA also has a role in SDSCs differentiation into EpiLCs. It is likely that ActA promotes PGCLC formation by promoting EpiLC differentiation, these EpiLCs further differentiated in to PGC precursors and PGCLCs in our differentiation medium containing BMP4 and other grow factors.
SMAD proteins are central elements in the activin receptor signaling pathway, and when SMAD2 and 3 are activated by phosphorylation they form a dimer with SMAD4 and translocate to the nucleus to modulate target gene expression.Citation61 We found that Smad3 knockdown by RNAi can block the effects of ActA during co-culture. Therefore Smad3 may be a key intracellular component of ActA in the induction, possibly mediated by activating ActA receptors, ACTRI and ACTRII. Citation35 This finding was also confirmed in cells from Smad3 knockout mice.
During the first 12 days of culture, the percentage of PGCLCs increased following treatment with ActA in a dose dependent manner. During this period, PGCLCs were formed quickly and promoted by the presence of ActA. This may have been a result from the effects of ActA on SDSCs dedifferentiation into EpiLCs and further to specialization into PGCLCs. However, at 16 days, the PGCLC number was decreased in the presence of ActA, instead, levels of some meiosis genes were significantly higher and in a dose dependent manner. This suggests that the effect of ActA on germ cell induction in vitro was stage dependent. During co-culture for the first 12 days, PGCLCs can be generated in large amounts, and ActA has an important role in PGCLC formation. At later stages of induction, ActA promotes PGCLCs to further develop and to enter meiosis, which may be accompanied by some apoptotic cell elimination. This is consistent with the concept that some PGCLCs may be defective and unable to begin meiotic initiation, and thereby be eliminated. During this process, ActA regulated the expression of some meiosis genes and may cross-talk with the RA pathway leading to an increase of the RA receptors, RARα, RARβ and RAR γ, and Stra8 levels. Meanwhile, TET1, a key factor in meiosis regulation,Citation42 was increased by ActA treatment. That also revealed an important role of ActA in meiotic entry. It is worth noting that ActA promotes the elimination of cells and the expression of key meiosis genes at 100 ng/ml. Higher dosage (ie. 200 ng/ml) ActA failed to be effective, possibly due to its cytotoxicity at high concentrations. However, in this process, there is likely to be some other members involved in meiotic initiation cooperating with ActA to regulate meiosis genes expression. Future studies should identify these factors and their interactions with ActA in this important process.
In current study, we used SDSCs to induce PGCLC formation in vitro and obtained cells that have the characteristics of PGCs in vivo. The establishment of differentiation system will overcome the ethical issue of ESCs in application. Also, SDSCs are more easily to sample, and thus, is significant for clinic application in infertility treatment. On the other hand, we first report the important role of ActA in PGCLC induction in vitro from SDSCs. This will help us to optimized the induction system and improve the differentiation efficiency of PGCLCs in vitro.
Materials and Methods
Animal care and mating
The mice used in this study included chicken β-actin promoter/enhanced green fluorescence proteins (CAG/EGFP) transgenic mice line (Stock TgN (GFPU) 5 Nagy) carrying a ubiquitously expressed EGFP), and Smad3 null mice kindly provided by Dr. Xiao Yang from the Institute of Biotechnology, Beijing. All mice used were housed and bred under controlled lighting conditions (12 L: 12 D). Timed mating was produced by housing females with males overnight and then checking for vaginal plugs the following morning. All procedures involving animals were approved by the Institutional Animal Care and Use Committees of Qingdao Agricultural University.
Isolation and culture of skin-derived stem cells
The dorsal skin of new born male mice carrying the GFP transgene was removed. Then skin samples were washed in normal saline 3 times, and cut into 1mm2 pieces and digested by 0.2% trypsin-EDTA incubation for 25 min at 37°C. Tissue pieces were then washed twice with PBS, 3 times with DMEM-F12 (1:1) (Hyclone, USA), and then mechanically dissociated by vigorous pipetting. Resulting cell suspensions were passed through 40 mm nylon cell strainers (BD, USA), centrifuged, and resuspended in DMEM/F12 (Gibco, USA) medium containing 1% penicillin/streptomycin supplemented with 1X B-27 (Gibco, USA), EGF (20 ng/ml, Sigma, USA) and bFGF (40 ng/ml, Peprotech, USA). Cells were cultured in 100-mm suspension culture dishes (Sarstedt, Germany) at 37°C, 5% CO2. Non adherent clusters of individual cells formed within 48 hrs of culture. Cells grown as spheres in suspension were cultured for 12 days, during which they were passaged twice prior to use.
Induced differentiation
For EBLS differentiation, skin spheres were centrifuged and mechanically dissociated into single cells by pipetting. Cells were plated at 6 × 105 cells per well (500 µl) in differentiation medium which consists of M199 (Hyclone, USA) supplemented with 150 μg/μl BSA (Sigma, USA), 5 μl/ml ITS (Gibco, USA), 0.23 mM sodium pyruvate (Hyclone, USA), 1 mg/ml Fetuin (calbiochem, Germany), 1 ng/ml EGF (Sigma, USA), 30 ng/ml BMP-4 (R&D, USA) and 0 to 200 ng/ml ActA (Sigma, USA). Cultured for 4 days, change half fresh medium every 2 days. EBLSs formed within 48 hrs of culture and grew quickly during 4 days of culture. Then, EBLSs were centrifuged and dissociated into single cells by digestion with 0.2% trypsin. Cells were plated at 5 × 105 cells per well (500 μl) and co-cultured with the MEF feeders in differentiation medium containing FBS, L-Gln (Gibco, USA), sodium pyruvate (Hyclone, USA), NE-AA (Gibco, USA), 20 ng/ml EGF (Hyclone, USA) and 40 ng/ml bFGF (Hyclone, USA), 40 ng/ml SCF (Sigma, USA) and 0 to 200 ng/ml ActA.
RNA interference (RNAi) on co-cultured cells
Co-cultured cells at different time point were transfected with 100 nM siRNA (GenePharma, China) using LipoFiterTM Liposomal Transfection Reagent (HB-TRLF-1000, China) following supplier's instructions. The medium was changed at the 24th hour after RNAi initiation with replacement of half of the fresh medium without siRNAs. And then, continued to culture for 1day and collected cells for further analysis. The sequences of Smad3 siRNA (GenePharma, China) are 5′ - GCA CAG CCA CCA UGA AUU ATT - 3 and 5′ - GCC UAC CAU GAC AGU AGA UTT - 3′ targeting 291-308 nt and 578-594 nt of Smad3 mRNA, respectively, and the non-targeting control siRNA (GenePharma, China) sequence is 5′ - UUC UCC GAA CGU GUC ACG UTT - 3′ which has no homology to any known mouse mRNAs.
RNA Isolation and RT-PCR
Total RNA was isolated by using RNAprep pure Micro Kit (Aidlab, China) according to the manufacture's protocol. cDNA was synthesized by SYBR Premix ExTaqTM II Kit (TaKaRa, China). Primers designed to amplify target and reference genes are shown in Table S1. Amplification was carried out with a Light Cycler real-time PCR instrument (Roche 480, Germany). The relative transcript abundance was analyzed by using the 2 −△Ct. Each sample was amplified in triplicates to normalize the system and pipetting error. Specificity of all the real-time PCR reactions was also confirmed by a single peak in the melt curves and a single band of the predicted size after agarose gel electrophoresis of the PCR products.
Immunofluorescence staining
Cells were washed 3 times with PBS and fixed in 4% paraformaldehyde for 20 min. After washed 3 times in PBS, cells were incubated in PBS with 0.5% Triton X-100 for 10 min. Next, cells were washed one time in PBS and blocked for 45 min in PBS with 10% normal goat serum and 0.5% Triton X-100, followed by an incubation with primary antibody, either 1:200 anti-STELLA (Abcam, HK, China), anti-DAZL (Abcam, HK, China), anti-OCT4 (CellSciences, USA), anti-GFP (Abcam, HK, China) or anti-VASA (Abcam, HK, Chia), anti-SSEA-1 (Millipore, USA) overnight at 4°C. For methylation analysis, cells were incubated in 4 M HCl for 10 min before incubated with primary antibody, anti-5mC (Millipore, USA), anti-5hmC (Active Motif, USA) and anti-H3K27me3 (Millipore, USA). After washed 3 times in PBS with 1% BSA, cells were incubated with 1: 50 Cy3-conjugated goat anti-rabbit IgG (Beyotime, China), Cy3-conjugated goat anti-mouse IgG (Beyotime, China) or 1:50 FITC-conjugated goat anti-mouse IgG for 2 hrs at 37°C. This was followed with PBS wash and incubation with Hoechst33342 for 5 min. Then cells were observed by using Laser scanning Confocal Microscopy (LEICA TCS SP5, Germany) or Olympus BX51 fluorescence microscope (Japan).
Flow cytometry
To analyze PGCLCs percentage and cell cycle, cells were digested to single cell by using 0.2% trypsin and washing with PBS. For PGCLCs percentage analysis, cells were blocked in PBS with 1% normal goat serum for 40 min at 4°C. The SSEA1 antibody was then added at a 1: 80 dilution and samples were incubated for 30 min at 4°C. Cells were washed and centrifuged 3 times in PBS and then incubated with Cy3-conjugated goat anti-mouse IgG for 1 hr at 37°C. Before analysis, cells were re-suspended in 500 μl PBS. Gates were set based on non-transgenic (no GFP) cells and incubated without primary antibody cells. Analysis was performed by using a FACS caliber flow cytometer (Becton-Dickinson, USA) equipped with FL1-H and FL2-H, respectively. Quantification analysis was completed by using Cell Quest software (Becton-Dickinson). Twenty thousand events were acquired per sample with fluorescence measured on logarithmic scales. Autofluorescence was removed from samples by setting gates on unstained controls. For cell cycle analysis, cells were fixed in 70% cold ethanol, and incubate for a minimum of 20 min. Then pellet cells (1000 rpm for 5–7 min) and carefully invert tubes to decant ethanol, followed by adding 0.5 ml PI-RNAse solution (final concentrations: 50 µg/ml PtdIns + 100 µg/ml RNase Type I-A in PBS). Mixed well, then cells were incubated at 37 °C in the dark for a minimum of 20 min before analysis by flow cytometry. Forty thousand events were acquired per sample. Quantification analysis was completed by using ModFit software.
Western blotting
Total proteins were released from cell samples with RIPA lysis solution (Beyotine, China) for 30 min on ice with frequent overtaxing. Then samples were added 16 µl/ 40 µl of sodium dodecyl sulfate-polyacrylamide gel electrophoresis (SDS-PAGE) sample loading buffer and boiled of 5 min. Lysates were collected by centrifugation (14000 rpm for 5 min). Proteins were separated by SDS-PAGE with a 4% stacking gel and a 10% separating gel for 2 hrs at 100 V, and then transferred onto polyvinylidene fluoride membrane (18 min, 15V) by electrophoresis. After blocked at 4°C for 4 hrs in TBST with 10% BSA, membranes were incubated with specific primary antibodies overnight at 4°C. The antibody used: Activin RIIB (R&D, USA), Activin RIB (R&D, USA), and Rabbit anti-SMAD3 antibody (Abcam, HK, China), at 1:1000 dilution in TBST. Then membranes were washed 3 times in TBST, and incubated with horseradish peroxidase (HRP)-conjugated goat anti-rabbit or mouse IgG (Beyotime, China) at 1:2000 dilution in TBST. BeyoECL plus Kit (Beyotime, China) was used for exposure. β-ACTIN was used as the loading control. The intensity was analyzed by AlphaView SA software.
Immunofluorescence staining of tissue sections
Paraffin embedded tissue sections were cut at 5 µm thickness and deparaffinized by treating with xylene and graded alcohols as per standard protocol. Sections were then washed 3 times in PBS. Antigen retrieval was performed by immersing the slides in 94°C10 mM sodium citrate buffer for 10 min. Sections were washed 3 times for 5 min in PBS, and then blocked by BDT (3% BSA, 10% NDS in TBS) for 30 min at room temperature, and incubated with anti-STELLA antibody (Abcom, HK, China) at a 1:200 dilution in BDT overnight at 4°C. Followed by washing in TBS for 3 times, sections were incubated with Cy3-conjugated goat anti-rabbit IgG (Beyotime, China) for 2 hrs at 37°C. Then sections were analyzed under Olympus BX51 fluorescence microscope (Japan) or Laser scanning Confocal Microscopy (LEICA TCS SP5, Germany).
PGCs and PGCLCs sorting by miniMACS
Using SSEA-1 antibody E 12.5 PGCs and PGCLCs co-cultured for 6 days were sorted by miniMACS. E 12.5 genital ridge or cultured cells were collected and dissociated into single cell by digesting with 0.2% trypsin and passed through 400 mesh cell strainers. After washing one time with cold MACS buffer containing 0.5% BSA, 2 mM EDTA in 0.01 M PBS, cells were incubated with SSEA-1 at 1:80 dilution in MACS buffer for 20 min at 4°C. Washed twice with MACS buffer to remove the unbound antibody, then cells were resuspended in 80 μl MACS buffer supplemented with 20 μl immunomagnetic beads coated with rabbit anti-mouse IgM (Miltenyi Biotec, Germany), and incubated for 15 min at 4°C. After washed twice with cold MACS buffer, cells were resuspended in 500 μl MACS buffer and passed cells through MS column to remove the SSEA-1 negative cells and washed column again with 800 μl buffer. At last, magnetic field was removed and MS column was washed with 800 μl buffer to collect SSEA-1 positive cells into an EP tube for further analysis.
Tail vein injection of ActA
Pregnant mice were treated at E 5.5 with 100 ng/kg body weight ActA by tail vein injection, and treated every 2 days according to the half-life of ActA. As a negative control, pregnant mice were injected with α-MEM medium that was used to dilute the ActA. Genital ridges were isolated at E 12.5 for analysis.
Statistical Analyses
For each set of results, independent experiments were repeated at least 3 times, with data representing the mean SEM of all repeats within an individual experiment. Data were analyzed by unpaired Student's t-Test with 2-tailed distribution of 3-sample of unequal variance to determine statistical differences between groups (denoted by a star) by using GraphPad Prism5 analysis software. P<0.05 denoted a statistically significant difference, while P < 0.01 denoted a highly significant difference.
Disclosure of Potential Conflicts of Interest
No potential conflicts of interest were disclosed.
1078031_supplemental_files.zip
Download Zip (21.7 MB)Funding
This work was supported by National Basic Research Program of China (973 Program, 2012CB944400 and 2013CB947900), National Nature Science Foundation (31171376 and 31471346), and the Taishan Scholar Construction Foundation and Nature Science Foundation of Shandong Province (ZR2013CQ029).
References
- Lawson KA, Dunn NR, Roelen BA, Zeinstra LM, Davis AM, Wright CV, Korving JP, Hogan BL. Bmp4 is required for the generation of primordial germ cells in the mouse embryo. Genes Dev 1999; 13:424-36; PMID:10049358; http://dx.doi.org/10.1101/gad.13.4.424
- Lawson KA, Hage WJ. Clonal analysis of the origin of primordial germ cells in the mouse. Ciba Foundation Symposium 1994; 182:68-84; discussion 84-91; PMID:7835158
- Hayashi K, Kobayashi T, Umino T, Goitsuka R, Matsui Y, Kitamura D. SMAD1 signaling is critical for initial commitment of germ cell lineage from mouse epiblast. Mech Dev 2002; 118:99-109; PMID:12351174; http://dx.doi.org/10.1016/S0925-4773(02)00237-X
- Hayashi K, de Sousa Lopez SM, Surani MA. Germ cell specification in mice. Science 2007; 20:394-6; PMID:17446386; http://dx.doi.org/10.1126/science.1137545
- Ying Y, Zhao GQ. Cooperation of endoderm-derived BMP2 and extraembryonic ectoderm-derived BMP4 in primordial germ cell generation in the mouse. Dev Biol 2001; 232:484-92; PMID:11401407; http://dx.doi.org/10.1006/dbio.2001.0173
- Ying Y, Qi X, Zhao GQ. Induction of primordial germ cells from murine epiblasts by synergistic action of BMP4 and BMP8B signaling pathways. Proc Natl Acad Sci 2001; 98:7858-62; PMID:11427739; http://dx.doi.org/10.1073/pnas.151242798
- Pesce M, Klinger GF, De Felici M. Derivation in culture of primordial germ cells from cells of the mouse epiblast: phenotypic induction and growth control by Bmp4 signaling. Mech Dev 2002; 112:15-24; PMID:11850175; http://dx.doi.org/10.1016/S0925-4773(01)00624-4
- Hayashi , Ohta H, Kurimoto K, Aramaki S, Saitou M. Reconstitution of the mouse germ cell specification pathway in culture by pluripotent stem cells. Cell 2011; 146:1-14; PMID:21820164; http://dx.doi.org/10.1016/j.cell.2011.06.027
- Hayashi K, Ogushi S, Kurimoto K, Shimamoto S, Ohta H, Saitou M. Offspring from oocytes derived from in vitro primordial germ cell-like cells in mice. Science 2012; 338:971-5; PMID:23042295; http://dx.doi.org/10.1126/science.1226889
- Dyce PW, Liu J, Tayade C, Kidder GM, Betts DH, Li J. In vitro and in vivo germ line potential of stem cells derived from newborn mouse skin. PLoS ONE 2011; 6(5):e20339; PMID:21629667; http://dx.doi.org/10.1371/journal.pone.0020339
- Hübner K, Fuhrmann G, Christenson LK, Kehler J, Reinbold R, De La Fuente R, Wood J, Strauss JF 3rd, Boiani M, Schöler HR.. Derivation of oocytes from mouse embryonic stem cells. Science 2003; 300:1251-6; PMID:12730498; http://dx.doi.org/10.1126/science.1083452
- Nayernia K, Nolte J, Michelmann HW, Lee JH, Rathsack K, Drusenheimer N, Dev A, Wulf G, Ehrmann IE, Elliott DJ, et al. In vitro-differentiated embryonic stem cells give rise to male gametes that can generate offspring mice. Dev Cell 2006; 11:125-32; PMID:16824959; http://dx.doi.org/10.1016/j.devcel.2006.05.010
- Danner S, Kajahn J, Geismann C, Klink E, Kruse C. Derivation of oocyte-like cells from a clonal pancreatic stem cell line. Mol Hum Reprod 2007; 13:11-20; PMID:17114208; http://dx.doi.org/10.1093/molehr/gal096
- Eguizabal C, Shovlin TC, Durcova-Hills G, Surani A, McLaren A. Generation of primordial germ cells from pluripotent stem cells. Differentiation 2009; 78:116-23; PMID:19683852; http://dx.doi.org/10.1016/j.diff.2009.07.001
- Nayernia K, Lee JH, Drusenheimer N, Nolte J, Wulf G, Dressel R, Gromoll J, Engel W. Derivation of male germ cells from bone marrow stem cells. Lab Invest 2006; 86:654-63; PMID:16652109; http://dx.doi.org/10.1038/labinvest.3700429
- Wei W, Qing T, Ye X, Liu H, Zhang D, Yang W, Deng H. Primordial germ cell specification from embryonic stem cells. PLoS ONE 2008; 3(12):e4013; PMID:19107197; http://dx.doi.org/10.1371/journal.pone.0004013
- Linher K, Dyce P, Li J. Primordial germ cell-like cells differentiated in vitro from skin-derived stem cells. PLoS ONE 2009; 4(12):e8263; PMID:20011593; http://dx.doi.org/10.1371/journal.pone.0008263
- Easley CA 4th, Phillips BT, McGuire MM, Barringer JM, Valli H, Hermann BP, Simerly CR, Rajkovic A, Miki T, et al. Direct differentiation of human pluripotent stem cells into haploid spermatogenic cells. Cell Rep 2012; 2(3):440-6; PMID:22921399; http://dx.doi.org/10.1016/j.celrep.2012.07.015
- Duggal G, Heindryckx B, Warrier S, O'Leary T, Van der Jeught M, Lierman S, Vossaert L, Deroo T, Deforce D, Chuva de Sousa Lopes SM, et al. Influence of Activin A supplementation during human embryonic stem cell derivation on germ cell differentiation potential. Stem Cells Dev 2013; 22(23):3141-55; PMID:23829223; http://dx.doi.org/10.1089/scd.2013.0024
- Irie N, Weinberger L, Tang WW, Kobayashi T, Viukov S, Manor YS, Dietmann S, Hanna JH, Surani MA. SOX17 is a critical specifier of human primordial germ cell fate. Cell 2015; 160(1–2):253-68; PMID:25543152; http://dx.doi.org/10.1016/j.cell.2014.12.013
- Toma JG, Akhavan M, Fernandes KJ, Barnabé-Heider F, Sadikot A, Kaplan DR, Miller FD Isolation of multipotent adult stem cells from the dermis of mammalian skin. Nat Cell Biol 2001; 3(9):778-84; PMID:11533656; http://dx.doi.org/10.1038/ncb0901-778
- Dyce PW, Wen L, Li J. In vitro germline potential of stem cells derived from fetal porcine skin. Nat Cell Biol 2006; 8:384-90; PMID:16565707; http://dx.doi.org/10.1038/ncb1388
- Park BW, Pan B, Toms D, Huynh E, Byun JH, Lee YM, Shen W, Rho GJ, Li J. Ovarian-cell-like cells from skin stem cells restored estradiol production and estrus cycling in ovariectomized mice. Stem Cells Dev 2014; 23(14):1647-58; PMID:24593690; http://dx.doi.org/10.1089/scd.2014.0029
- Shen W, Park BW, Toms D, Li J. Midkine promotes proliferation of primordial germ cell by inhibiting the expression of the deleted in azoospermia-like gene. Endocrinol 2012; 153(7):3482-92; PMID:2256497; http://dx.doi.org/10.1210/en.2011-1456
- Chapman SC, Kenny HA, Woodruff TK. Activin, Inhibin and Follistatin in the ovary physiology. In: Adashi EY, Leung PC, Eds. The Ovary. second edition, Elsevier Academic Press 2004; 273-88
- Ling N, Ying SY, Ueno N, Shimasaki S, Esch F, Hotta M, Guillemin R. Pituitary FSH is released by a heterodimer of the β-subunits from the two forms of inhibin. Nature 1986; 321:779-82; PMID:3086749; http://dx.doi.org/10.1038/321779a0
- Robertson DM, de Vos FL, Foulds LM, McLachlan RI, Burger HG, Morgan FJ, Hearn MT, de Kretser DM. Isolation of a 31 kDa form of inhibin from bovine follicular fluid. Mol Cell Endocrinol 1986; 44:271-7; PMID:3082696; http://dx.doi.org/10.1016/0303-7207(86)90133-4
- Ethier JF, Findlay JK. Roles of activin and its signal transduction mechanisms in reproductive tissues. Reproduction 2001; 121:667-75; PMID:11427154; http://dx.doi.org/10.1530/rep.0.1210667
- Thomas FH, Armstrong DG, Telfer EE. Activin promotes oocyte development in ovine preantral follicles in vitro. Reprod Biol Endocrinol 2003:1-76; PMID:14613548
- Coutts SM, Childs AJ, Fulton N, Collins C, Bayne RA, McNeilly AS, Anderson RA. Activin signals via SMAD2/3 between germ and somatic cells in the human fetal ovary and regulates kit ligand expression. Dev Biol 2008; 314: 189-99; PMID:18166170; http://dx.doi.org/10.1016/j.ydbio.2007.11.026
- Mendis SH, Meachem SJ, Sarraj MA, Loveland KL. Activin A Balances Sertoli and Germ Cell Proliferation in the Fetal Mouse Testis. Biol Reprod 2011; 84:379-91; PMID:20926807; http://dx.doi.org/10.1095/biolreprod.110.086231
- Martins da Silva SJ, Bayne RA, Cambray N, Hartley PS, McNeilly AS, Anderson RA. Expression of activin subunits and receptors in the developing human ovary: activin A promotes germ cell survival and proliferation before primordial follicle formation. Dev Biol 2004; 266:334-45; PMID:14738881; http://dx.doi.org/10.1016/j.ydbio.2003.10.030
- Zhang ZP, Liang GJ, Zhang XF, Zhang GL, Chao HH, Li L, Sun XF, Min LJ, Pan QJ, Shi QH, et al. Growth of mouse oocytes to maturity from premeiotic germ cells in vitro. PLoS One 2012; 7(7):e41771; PMID:22848595; http://dx.doi.org/10.1371/journal.pone.0041771
- Itman C, Mendis S, Barakat B, Loveland KL. All in the family: TGF-b family action in testis development. Reproduction 2006; 132:233-46; PMID:16885532; http://dx.doi.org/10.1530/rep.1.01075
- Loveland KL, Dias V, Meachem S, Rajpert-De Meyts E. The transforming growth factor-b superfamily in early spermatogenesis: potential relevance to testicular dysgenesis. Int J Androl 2007; 30:377-84; PMID:17705810; http://dx.doi.org/10.1111/j.1365-2605.2007.00785.x
- Ohinata Y, Ohta H, Shigeta M, Yamanaka K, Wakayama T, Saitou M. A signaling principle for the specification of the germ cell lineage in mice. Cell 2009; 137:571-84; PMID:19410550; http://dx.doi.org/10.1016/j.cell.2009.03.014
- Yamaguchi S, Hong K, Liu R, Inoue A, Shen L, Zhang K, Zhang Y. Dynamics of 5-methylcytosine and 5-hydroxymethylcytosine during germ cell reprogramming. Cell Res 2013; 23:329-39; PMID:23399596; http://dx.doi.org/10.1038/cr.2013.22
- Hackett JA, Sengupta R, Zylicz JJ, Murakami K, Lee C, Down TA, Surani MA. Germline DNA demethylation dynamics and imprint erasure through 5-hydroxymethylcytosine. Science 2013; 39:448-52; PMID:23223451; http://dx.doi.org/10.1126/science.1229277
- Vincent JJ, Huang Y, Chen PY, Feng S, Calvopiña JH, Nee K, Lee SA, Le T, Yoon AJ, Faull K, et al. Stage-specific roles for Tet1 and Tet2 in DNA demethylation in primordial germ cells. Cell Stem Cell 2013; 12:470-78; PMID:23415914; http://dx.doi.org/10.1016/j.stem.2013.01.016
- Yamaguchi S, Hong K, Liu R, Shen L, Inoue A, Diep D, Zhang K, Zhang Y. Tet1 controls meiosis by regulating meiotic gene expression. Nature 2012; 492:443-47; PMID:23222611; http://dx.doi.org/10.1038/nature11709
- Tahiliani M, Koh KP, Shen Y, Pastor WA, Bandukwala H, Brudno Y, Agarwal S, Iyer LM, Liu DR, Aravind L, et al. Conversion of 5-methylcytosine to 5-hydroxymethylcytosine in mammalian DNA by MLL partner TET1. Science 2009; 324:930-35; PMID:19372391; http://dx.doi.org/10.1126/science.1170116
- Pangas SA, Woodruff TK. Activin Signal Transduction Pathways. STEM 2000;11,(8):309-14
- Gong X, McGee EA. Smad3 is required for normal follicular follicle-stimulating hormone responsiveness in the mouse. Biol Reprod 2009; 81:730-38; PMID:19535790; http://dx.doi.org/10.1095/biolreprod.108.070086
- Bowles J, Knight D, Smith C, Wilhelm D, Richman J, Mamiya S, Yashiro K, Chawengsaksophak K, Wilson MJ, Rossant J, et al. Retinoid signaling determines germ cell fate in mice. Science 2006; 312:596-600; PMID:16574820; http://dx.doi.org/10.1126/science.1125691
- Baltus AE, Menke DB, Hu YC, Goodheart ML, Carpenter AE, de Rooij DG, Page DC.In germ cells of mouse embryonic ovaries, the decision to enter meiosis precedes premeiotic DNA replication. Nat Genet 2006; 38:1430-4; PMID:17115059; http://dx.doi.org/10.1038/ng1919
- Koubova J, Menke DB, Zhou Q, Capel B, Griswold MD, Page DC. Retinoic acid regulates sex-specific timing of meiotic initiation in mice. Proc Natl Acad Sci USA 2006; 103:2474-9; PMID:16461896; http://dx.doi.org/10.1073/pnas.0510813103
- Anderson EL, Baltus AE, Roepers-Gajadien HL, Hassold TJ, de Rooij DG, van Pelt AM, Page DC. Stra8 and its inducer, retinoic acid, regulate meiotic initiation in both spermatogenesis and oogenesis in mice. Proc Natl Acad Sci USA 2008; 105:14976-80; PMID:18799751; http://dx.doi.org/10.1073/pnas.0807297105
- Griswold MD, Hogarth CA, Bowles J, Koopman P. Initiating meiosis: the case for retinoic acid. Biol Reprod 2012; 86:35; PMID:22075477; http://dx.doi.org/10.1095/biolreprod.111.096610
- Dyce PW, Zhu H, Craig J, Li J. Stem cells with multilineage potential derived from porcine skin. Biochem Biophys Res Commun 2004; 316:651-8; PMID:15033449; http://dx.doi.org/10.1016/j.bbrc.2004.02.093
- Itskovitz Eldor J, Schuldiner M, Karsenti D, Eden A, Yanuka O, Amit M, Soreq H, Benvenisty N. Differentiation of Human Embryonic Stem Cells into Embryoid Bodies Comprising the Three Embryonic Germ Layers. Mol Med 2000; 6:88-95; PMID:10859025
- Valli A, Rosner M, Fuchs C, Siegel N, Bishop CE, Dolznig H, Mädel U, Feichtinger W, Atala A, Hengstschläger M. Embryoid body formation of human amniotic fluid stem cells depends on mTOR. Oncogene 2010; 29:966-77; PMID:19935716; http://dx.doi.org/10.1038/onc.2009.405
- Rosner M, Dolznig H, Schipany K, Mikula M, Brandau O, Hengstschläger M. Human amniotic fluid stem cells as a model for functional studies of genes involved in human genetic diseases or oncogenesis. Oncotarget 2011;2:705-12; PMID:21926447
- Findlay JK, Drummond AE, Dyson ML, Baillie AJ, Robertson DM, Ethier JF. Recruitment and development of the follicle; the roles of the transforming growth factor-β superfamily. Mol Cell Endocrinol 2002; 191:35-43; http://dx.doi.org/10.1016/S0303-7207(02)00053-9
- Knight PG, Glister C. TGF-β superfamily members and ovarian follicle development. Reproduction 2006; 132:191-206; PMID:16885529; http://dx.doi.org/10.1530/rep.1.01074
- Mishina Y, Crombie R, Bradley A, Behringer RR. Multiple roles for activin-like kinase-2 signaling during mouse embryogenesis. Dev Biol 1999; 213:314-26; PMID:10479450; http://dx.doi.org/10.1006/dbio.1999.9378
- Chuva de Sousa Lopes SM, van den Driesche S, Carvalho RL, Larsson J, Eggen B, Surani MA, Mummery CL. Altered primordial germ cell migration in the absence of transforming growth factor signaling via ALK5. Dev Biol 2005; 284:194-203; PMID:15993878; http://dx.doi.org/10.1016/j.ydbio.2005.05.019
- Richards AJ, Enders GC, and Resnick JL. Activin and TGFb limit murine primordial germ cell proliferation. Dev Biol 1999; 207:470-5; PMID:10068477; http://dx.doi.org/10.1006/dbio.1998.9174
- Teo AK, Ali Y, Wong KY, Chipperfield H, Sadasivam A, Poobalan Y, Tan EK, Wang ST, Abraham S, Tsuneyoshi N, et al. Activin and BMP4 synergistically promote formation of definitive endoderm in human embryonic stem cells. Stem Cells 2012;30:631-42; PMID:22893457; http://dx.doi.org/10.1002/stem.1022
- Xu X, Browning VL, Odorico JS. Activin, BMP and FGF pathways cooperate to promote endoderm and pancreatic lineage cell differentiation from human embryonic stem cells. Mech Dev 2011;128:412-27; PMID:21855631; http://dx.doi.org/10.1016/j.mod.2011.08.001
- Duggal G, Heindryckx B, Warrier S, Taelman J, Van der Jeught M, Deforce D, Chuva De Sousa Lopes S, De Sutter P. Exogenous supplementation of Activin A enhances germ cell differentiation of human embryonic stem cells. Mol Hum Reprod 2015; 21(5):410-23; PMID:25634576
- Massagué J. TGF-β signal transduction. Annu Rev Biochem 1998; 67:753-91; PMID:9759503; http://dx.doi.org/10.1146/annurev.biochem.67.1.753