ABSTRACT
Eukaryotic translation initiation factor 4E (eIF4E)-binding protein 1 (4E-BP1) is a member of a family of translation repressor proteins, and a well-known substrate of mechanistic target of rapamycin (mTOR) signaling pathway. Phosphorylation of 4E-BP1 causes its release from eIF4E to allow cap-dependent translation to proceed. Recently, 4E-BP1 was shown to be phosphorylated by other kinases besides mTOR, and overexpression of 4E-BP1 was found in different human carcinomas. In this review, we summarize the novel findings on mTOR independent 4E-BP1 phosphorylation in carcinomas. The implications of overexpression and possible multi-function of 4E-BP1 are also discussed.
mTOR/4E-BP1 signaling pathway
The mechanistic target of rapamycin (mTOR) is a central controller of organismal growth and survival in response to growth factors, cytokines, hormones and nutrients.Citation1 It is a PI3K-related kinase that forms two distinct protein complexes called mTOR complex 1 or mTORC1 and mTOR complex 2 or mTORC2. In response to upstream stimuli, mTORC1 phosphorylates S6 kinase 1 (S6K1) and eukaryotic translation initiation factor 4E (eIF4E)-binding protein 1 (4E-BP1) to stimulate protein synthesis,Citation2 while mTORC2 phosphorylates AKT to promote cell survival.Citation3 The mTOR pathway regulates major cellular processes and is implicated in several pathological conditions, including cancer, obesity, type 2 diabetes, and neurodegeneration.Citation4
Typically, translation is regulated at the initiation phase, when a ribosome is recruited to the 5′-cap of an mRNA. 4E-BP1 interdicts translation initiation by binding to the translation factor eIF4E, which is a limiting component of the multi-subunit complex that recruits 40S ribosomal subunits to the 5′-cap of mRNAs. Interaction of hypophosphorylated 4E-BP1 with eIF4E prevents its interaction with eIF4G, inhibits complex assembly and represses translation. Upon activation, mTORC1 remains at the mRNA 5′-cap, where it is well positioned to phosphorylate 4E-BP1 resulting in its dissociation from eIF4E and the recruitment of eIF4G to the 5′-cap, thereby allowing cap-dependent translation initiation to proceed.Citation2 As one of the two most established mTOR substrates, 4E-BP1 plays a pivotal role in mTORC1 signaling to control translation and cell proliferation.Citation2,5,6
4E-BP1 phosphorylation: conventional and novel findings
Phosphorylated 4E-BP1 has been generally accepted as a marker of activated mTOR signaling. Seven phosphorylation sites have been identified in 4E-BP1: Thr 37, Thr 46, Ser 65, Thr 70, Ser 83, Ser 101, and Ser 112 (numbering based on human 4E-BP1; in rodents, the numbers are lower by one).Citation7 The first five phosphorylation sites are phylogenetically conserved among all species, and phosphorylation of Thr 37 and Thr 46 serves as a priming event, which is followed by Thr 70 phosphorylation and finally Ser 65 phosphorylation.Citation8,9 Although mTOR immunoprecipitates phosphorylated Thr 37 and Thr 46 in vitro, whether mTOR phosphorylates 4E-BP1 on all or some of these sites remains unknown. A kinase activity specific to Ser 65 and Thr 70 was reported to be liberated from an mTOR immunoprecipitate, suggesting the presence of a second kinase.Citation10
Possible kinases involved in 4E-BP1 phosphorylation
Recently, several kinases have been shown to phosphorylate 4E-BP1 dependent or independent of mTOR, indicating that mTOR may not be the only kinase that phosphorylates 4E-BP1, challenging the conventional mTOR/4E-BP1 cascades.Citation11 mTOR inhibitor resistance (for allosteric inhibitors, e.g. rapamycin and rapalogs, and the more potent ATP-competitive mTOR kinase inhibitors) is correlated with the inability of these inhibitors to block 4E-BP1 phosphorylation in some cancer cells.Citation12-15 Phosphorylation of mTORC1 substrates is subject to multiple regulatory mechanisms and feedback loops that extend far beyond the simple sequence motif of each phosphorylation site.Citation16 In the case of 4E-BP1, which is regarded as a point of convergence of various signaling pathways, it is possible that other kinases cooperate with or independent of mTORC1 regulate its phosphorylation.Citation17,18 We have shown that mTOR kinase inhibitors sustained on-target inhibition of mTOR in colorectal cancer (CRC) cells, but only transiently attenuated 4E-BP1 phosphorylation in drug-resistant CRC cells because 4E-BP1 was re-phosphorylated in an mTOR-independent manner ().Citation12 Several candidates were recently suggested to be responsible for 4E-BP1 phosphorylation. Since GSK3β phosphorylates 4E-BP1 at Thr 37 and Thr 46 and thereby decreases its association with eIF4E, it is also possible that GSK-3 could regulate the phosphorylation of other sites (S 65 and/or T 70) in some cancer cells.Citation19 MAP kinase p38 may increase 4E-BP1 protein by hyperphosphorylation in response to several cellular stresses such as viral infection, osmotic shock, UV irradiation, and TNFα/CHX-mediated apoptosis.Citation20,21 UVB-induced phosphorylation of 4E-BP1 at multiple sites including Thr 36, Thr 45, Ser 64 and Thr 69 in the mouse epidermal JB6 cell line was mediated by p38MAPK and its downstream kinase MSK1, which provides a novel mechanism for UV-promoted tumorigenesis.Citation22 ERK phosphorylates 4E-BP1 at Ser 65, and also blocks TSC2, leading to greater mTOR activity and increased phosphorylation of 4E-BP1. It is likely that ERK acts directly on 4E-BP1 and indirectly via TSC2/mTOR following ionizing radiation (IR) and stimulates protein synthesis via ATM-dependent ERK phosphorylation.Citation23,24 4E-BP1 phosphorylation makes it resistant to rapamycin, which has been correlated to the induction of PIM kinases.Citation25 PIM2 could work directly in the cytoplasm to phosphorylate 4E-BP1 at Ser 65 Citation26 or regulate the activity of specific phosphatases.Citation27,28 ATM, the protein product of the ATM gene, phosphorylates 4E-BP1 at Ser 111 in vitro. Insulin treatment induces phosphorylation of 4E-BP1 at Ser 112 in vivo in an ATM-dependent manner.Citation29 Cyclin-dependent kinase, cdc2/CDK1, can phosphorylate 4E-BP1 at Thr 70 in HeLa cells.Citation30 Paclitaxel (PTX) induces the hyperphosphorylation of 4E-BP1 in the breast cancer cell line, MDA-MB-231, through a CDK1-dependent mechanism.Citation31 LRRK2, leucine-rich repeat kinase 2, is one of the physiological kinases for 4E-BP. LRRK2 exerts an effect on 4E-BP1 primarily at the Thr 37/46 sites.Citation32 Some studies have shown that mTOR kinase inhibitors block p4E-BP1 more effectively than rapalogs, suggesting that mTORC2 is a candidate for 4E-BP1 phosphorylation.Citation4 But whether rapamycin-resistant and PIM-kinase-dependent 4E-BP1 phosphorylation is mediated by the mTORC2 complex Citation33 remains unknown ().
Figure 1. mTOR-independent 4E-BP1 phosphorylation is associated with colorectal cancer (CRC) resistance to mTOR kinase inhibitors (mTKIs). TIEK: TOR- independent 4E-BP1 kinase.
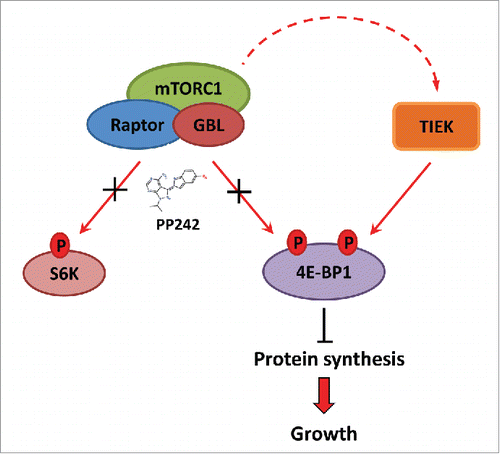
Table 1. Possible kinases for mTOR-independent 4E-BP1 phosphorylation.
These findings add to our understanding of the mechanisms by which cancer cells are resistant to mTOR inhibitor treatment. Some important questions remain regarding whether these potential kinases contribute to the intrinsic or acquired drug resistance, and why mTOR substrate 4E-BP1 is phosphorylated by certain kinases in some cancer cells but not in others?
Overexpression of 4E-BP1 in carcinomas
High level of phosphorylated 4E-BP1 was widely reported in human cancers, and has been associated with a worse outcome in several malignancies. However, the pattern of 4E-BP1 expression is not clearly understood. Because 4E-BP1 belongs to the family of translation repressor proteins, it is reasonable to assume that 4E-BP1 expression is lower in carcinomas as compared to normal tissue. However, overexpression of 4E-BP1 has been found in various carcinomas, which is counterintuitive to its known suppressive role in translation. Based on data from Oncomine (https://www.oncomine.com), most studies on brain, CNS, CRC, kidney and other cancer types have shown a higher level of 4E-BP1 in cancer tissue as compared to normal tissue ().
Figure 2. Overexpression of 4E-BP1 has been found in various carcinomas. Original data from https://www.oncomine.com was analyzed and plotted into a bar graph. Studies that showed higher 4E-BP1 level in cancer tissue vs. normal tissue are depicted in red and others in blue.
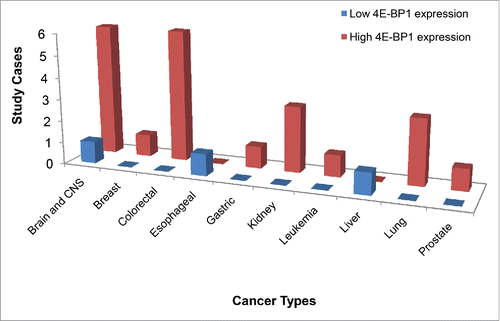
4E-BP1 overexpression is strongly associated with prostate cancer. Expression of 4E-BP1 was elevated in prostate intraepithelial neoplasia (PIN, the pre-cancerous lesion of prostate cancer), and was significantly higher in prostate cancer as compared to normal tissue. 4E-BP1 was highly overexpressed in 40% of cancers and at least some overexpression was observed in the other 60% of cancers in a tissue array. Nearly all cancer samples had over 3.3-fold higher IHC staining scores than normal lumenal cells.Citation34 4E-BP1 was also overexpressed in several large advanced breast cancers.Citation35 High cytoplasmic 4E-BP1 protein levels predict less benefit from endocrine treatment.Citation36 4E-BP1 overexpression also occurs in head, neck and some gastrointestinal cancers.Citation37-39
Possible mechanisms for 4E-BP1 overexpression in cancer
Amplification of the 4E-BP1 gene is one possible mechanism for its overexpression in tumors. The gene encoding 4E-BP1 is located at the chromosomal region 8p12, which is commonly amplified in breast cancer. Gene amplification and the resultant high expression of 4E-BP1 were shown to indicate a poor prognosis.Citation40,41 Another suggested mechanism is through transcription. High 4E-BP1 mRNA, independent of phosphorylation status, was associated with an adverse outcome in breast cancer, especially in ER-positive subgroup.Citation36 4E-BP1 protein accumulates under different conditions by different transcription factors. In prostate cancer, amplification or induced expression of cMyc has been shown to promote its binding to the 4E-BP1 gene promoter and increase expression.Citation42 ATF4 mediates induction of 4E-BP1 in pancreatic beta-cells under endoplasmic reticulum stress.Citation43 In pancreatic cancer cell lines, hypoxia-triggered induction of 4E-BP1 is dependent on HIF-1α and SMAD4.Citation44
Since hyperphosphorylation and overexpression of 4E-BP1 occurs simultaneously in human cancers, is there a possible correlation between 4E-BP1 phosphorylation and its expression level? Phosphorylation is the most common mechanism by which a stable protein can be targeted for ubiquitination and subsequent degradation. However, phosphorylation can also have an opposing effect of stabilizing a short-lived protein, especially those proteins that require multi-site phosphorylation.Citation45-48 Because more than one kinase is involved in the phosphorylation of multiple sites, dephosphorylation of a single site is not sufficient for ubiquitination and degradation. Thus, multi-site phosphorylation could set a higher threshold for susceptibility to degradation.Citation46,49 Therefore, hyperphosphorylation of 4E-BP1 at multiple sites may play an important role in its stabilization and overexpression.
Possible functions of overexpressed 4E-BP1 in cancer
Approximately 30% of the 4E-BP1 expressed in cells is located in the nucleus, where it regulates the availability of EIF4E for the cytoplasmic translational machinery, by retaining EIF4E in the nucleus.Citation36 Thus, what the remaining (70% or more) 4E-BP1 does in the cytosol remains unknown. A recent study revealed that cytoplasmic overexpressed 4E-BP1 orchestrates a hypoxia-activated switch from cap-dependent to cap-independent mRNA translation that promotes increased tumor angiogenesis and growth at the level of selective mRNA translation. Eukaryotic cells perform both cap-dependent and cap-independent (IRES-mediated) protein synthesis. Under normal growth conditions cap-dependent translation is dominant, while under stress such as low nutrients and hypoxia in advanced cancer, cap-dependent translation is restricted but some cellular mRNAs can utilize cap-independent (IRES-mediated) mechanism of initiation, including those that orchestrate angiogenesis (VEGF-A), hypoxia responses (transcription factor HIF1α),Citation50 and inhibition of apoptosis (Bcl2).Citation50 So, in advanced cancer, 4E-BP1 acts as a hypoxia-inducible switch, promoting cap-independent over cap-dependent translation, resulting in selective translation of IRES-containing mRNAs such as VEGF, HIF1α, and Bcl2 that promote tumor angiogenesis, survival,Citation35 and may be involved in the PI3K/mTOR inhibitor adaptive resistance in matrix-attached cancer cells.Citation51 MYC expression is reported to abrogate sensitivity to rapamycin through increased expression of 4E-BP1 and reduced autophagy in prostate cancer.Citation42 PC-1/PrLZ confers resistance to rapamycin in prostate cancer cells through increased 4E-BP1 stability.Citation52 Thus, besides translation repression, 4E-BP1 may also act as a tumor promoter under certain cellular conditions, indicating that 4E-BP1 is a multi-functional protein. This hypothesis is currently being tested.
While these findings explain how certain advanced tumors keep progressing in an unfavorable environment, some important questions remain regarding whether 4E-BP1 overexpression is a cause for tumor development or a consequence of tumor overgrowth. Also, the involvement of any other factors dependent on the cellular context in the switch in 4E-BP1 functions needs to be investigated.
Conclusion
Several candidates are assumed to phosphorylate 4E-BP1, suggesting that the regulation of 4E-BP1 may be multifactorial, and thus highly dependent on the cellular context. Indeed, it is plausible to assume that cancer cells adapt by deregulating additional kinases, such as GSK3β, that substitute for mTOR in the phosphorylation and inactivation of some mTOR substrates, such as 4E-BP1. We believe that finding alternative kinases responsible for 4E-BP1 phosphorylation will be an important future task, especially to identify new targets for cancer therapy.
4E-BP1 overexpression is nearly universal in cancer, although the degree of overexpression varies, suggesting that dysregulation of translation may occur in cancer or may be responsible for cancer progression. Aberrant 4E-BP1 expression may contribute to the inability of cells to properly translate, or gaining the ability of selective mRNA translation. Transcriptional regulation and post-translational modification work together to modulate the level and function of 4E-BP1, making it a complex controller of protein synthesis and a powerful transfer station far beyond mTOR signaling pathway.
Abbreviations
4E-BP1 | = | Eukaryotic translation initiation factor 4E (eIF4E)-binding protein 1 |
mTOR | = | mechanistic target of rapamycin |
S6K1 | = | S6 kinase 1 |
PTX | = | Paclitaxel |
PIN | = | prostate intraepithelial neoplasia |
mTKIs | = | mTOR kinase inhibitors |
TIEK | = | TOR- independent 4E-BP1 kinase |
Disclosure of potential conflicts of interest
No potential conflicts of interest were disclosed.
Author contributions
Qin X, Jiang B and Zhang Y designed and wrote the review. Zhang Y took responsibility for revising the manuscript and final approval of the version to be published.
Funding
This work was supported by National Nature Science Foundation of China 81270035 and Shanghai Pujiang Program 15PJ1404900 (Zhang Y).
References
- Wang XW, Zhang YJ. Targeting mTOR network in colorectal cancer therapy. World J Gastroenterol 2014; 20:4178-88; PMID:24764656; http://dx.doi.org/10.3748/wjg.v20.i15.4178
- Ma XM, Blenis J. Molecular mechanisms of mTOR-mediated translational control. Nat Rev Mol Cell Biol 2009; 10:307-18; PMID:19339977; http://dx.doi.org/10.1038/nrm2672
- Foster KG, Fingar DC. Mammalian target of rapamycin (mTOR): conducting the cellular signaling symphony. J Biol Chem 2010; 285:14071-7; PMID:20231296; http://dx.doi.org/10.1074/jbc.R109.094003
- Laplante M, Sabatini DM. mTOR signaling in growth control and disease. Cell 2012; 149:274-93; PMID:22500797; http://dx.doi.org/10.1016/j.cell.2012.03.017
- Lv T, Wang Q, Cromie M, Liu H, Tang S, Song Y, Gao W. Twist1-mediated 4E-BP1 regulation through mTOR in non-small cell lung cancer. Oncotarget 2015; 6:33006-18; PMID:26360779; http://dx.doi.org/10.18632/oncotarget.3400
- Shull AY, Noonepalle SK, Awan FT, Liu J, Pei L, Bollag RJ, Salman H, Ding Z, Shi H. RPPA-based protein profiling reveals eIF4G overexpression and 4E-BP1 serine 65 phosphorylation as molecular events that correspond with a pro-survival phenotype in chronic lymphocytic leukemia. Oncotarget 2015; 6:14632-45; PMID:25999352; http://dx.doi.org/10.18632/oncotarget.4104
- Martineau Y, Azar R, Bousquet C, Pyronnet S. Anti-oncogenic potential of the eIF4E-binding proteins. Oncogene 2013; 32:671-7; PMID:22508483; http://dx.doi.org/10.1038/onc.2012.116
- Gingras AC, Raught B, Gygi SP, Niedzwiecka A, Miron M, Burley SK, Polakiewicz RD, Wyslouch-Cieszynska A, Aebersold R, Sonenberg N. Hierarchical phosphorylation of the translation inhibitor 4E-BP1. Genes Dev 2001; 15:2852-64; PMID:11691836; http://dx.doi.org/10.1101/gad.887201
- Wang X, Li W, Parra JL, Beugnet A, Proud CG. The C terminus of initiation factor 4E-binding protein 1 contains multiple regulatory features that influence its function and phosphorylation. Mol Cell Biol 2003; 23:1546-57; PMID:12588975; http://dx.doi.org/10.1128/MCB.23.5.1546-1557.2003
- Heesom KJ, Denton RM. Dissociation of the eukaryotic initiation factor-4E/4E-BP1 complex involves phosphorylation of 4E-BP1 by an mTOR-associated kinase. FEBS Lett 1999; 457:489-93; PMID:10471835; http://dx.doi.org/10.1016/S0014-5793(99)01094-7
- Sikalidis AK, Mazor KM, Kang M, Liu H, Stipanuk MH. Total 4EBP1 Is Elevated in Liver of Rats in Response to Low Sulfur Amino Acid Intake. J Amino Acids 2013; 2013:864757; PMID:24089634; http://dx.doi.org/10.1155/2013/864757
- Zhang Y, Zheng XF. mTOR-independent 4E-BP1 phosphorylation is associated with cancer resistance to mTOR kinase inhibitors. Cell Cycle (Georgetown, Tex) 2012; 11:594-603
- Choo AY, Yoon SO, Kim SG, Roux PP, Blenis J. Rapamycin differentially inhibits S6Ks and 4E-BP1 to mediate cell-type-specific repression of mRNA translation. Proc Natl Acad Sci USA 2008; 105:17414-9; PMID:18955708; http://dx.doi.org/10.1073/pnas.0809136105
- Ducker GS, Atreya CE, Simko JP, Hom YK, Matli MR, Benes CH, Hann B, Nakakura EK, Bergsland EK, Donner DB, et al. Incomplete inhibition of phosphorylation of 4E-BP1 as a mechanism of primary resistance to ATP-competitive mTOR inhibitors. Oncogene 2014; 33:1590-600; PMID:23542178; http://dx.doi.org/10.1038/onc.2013.92
- Mi W, Ye Q, Liu S, She QB. AKT inhibition overcomes rapamycin resistance by enhancing the repressive function of PRAS40 on mTORC1/4E-BP1 axis. Oncotarget 2015; 6:13962-77; PMID:25961827; http://dx.doi.org/10.18632/oncotarget.3920
- Kang SA, Pacold ME, Cervantes CL, Lim D, Lou HJ, Ottina K, Gray NS, Turk BE, Yaffe MB, Sabatini DM. mTORC1 phosphorylation sites encode their sensitivity to starvation and rapamycin. Science 2013; 341:1236566; PMID:23888043; http://dx.doi.org/10.1126/science.1236566
- She QB, Halilovic E, Ye Q, Zhen W, Shirasawa S, Sasazuki T, Solit DB, Rosen N. 4E-BP1 is a key effector of the oncogenic activation of the AKT and ERK signaling pathways that integrates their function in tumors. Cancer Cell 2010; 18:39-51; PMID:20609351; http://dx.doi.org/10.1016/j.ccr.2010.05.023
- Yoon SO, Roux PP. Rapamycin resistance: mTORC1 substrates hold some of the answers. Curr Biol 2013; 23:R880-3; PMID:24112984; http://dx.doi.org/10.1016/j.cub.2013.08.030
- Shin S, Wolgamott L, Tcherkezian J, Vallabhapurapu S, Yu Y, Roux PP, Yoon SO. Glycogen synthase kinase-3beta positively regulates protein synthesis and cell proliferation through the regulation of translation initiation factor 4E-binding protein 1. Oncogene 2014; 33:1690-9; PMID:23584478; http://dx.doi.org/10.1038/onc.2013.113
- Walsh D, Mohr I. Phosphorylation of eIF4E by Mnk-1 enhances HSV-1 translation and replication in quiescent cells. Genes Dev 2004; 18:660-72; PMID:15075293; http://dx.doi.org/10.1101/gad.1185304
- Janzen C, Sen S, Cuevas J, Reddy ST, Chaudhuri G. Protein phosphatase 2A promotes endothelial survival via stabilization of translational inhibitor 4E-BP1 following exposure to tumor necrosis factor-α. Arteriosclerosis Thrombosis Vascular Biol 2011; 31:2586-94; PMID:21903942; http://dx.doi.org/10.1161/ATVBAHA.111.230946
- Liu G, Zhang Y, Bode AM, Ma WY, Dong Z. Phosphorylation of 4E-BP1 is mediated by the p38/MSK1 pathway in response to UVB irradiation. J Biol Chem 2002; 277:8810-6; PMID:11777913; http://dx.doi.org/10.1074/jbc.M110477200
- Haystead TA, Haystead CM, Hu C, Lin TA, Lawrence JC, Jr. Phosphorylation of PHAS-I by mitogen-activated protein (MAP) kinase. Identification of a site phosphorylated by MAP kinase in vitro and in response to insulin in rat adipocytes. J Biol Chem 1994; 269:23185-91; PMID:8083223
- Braunstein S, Badura ML, Xi Q, Formenti SC, Schneider RJ. Regulation of protein synthesis by ionizing radiation. Mol Cell Biol 2009; 29:5645-56; PMID:19704005; http://dx.doi.org/10.1128/MCB.00711-09
- Tamburini J, Green AS, Bardet V, Chapuis N, Park S, Willems L, Uzunov M, Ifrah N, Dreyfus F, Lacombe C, et al. Protein synthesis is resistant to rapamycin and constitutes a promising therapeutic target in acute myeloid leukemia. Blood 2009; 114:1618-27; PMID:19458359; http://dx.doi.org/10.1182/blood-2008-10-184515
- Fox CJ, Hammerman PS, Cinalli RM, Master SR, Chodosh LA, Thompson CB. The serine/threonine kinase Pim-2 is a transcriptionally regulated apoptotic inhibitor. Genes Dev 2003; 17:1841-54; PMID:12869584; http://dx.doi.org/10.1101/gad.1105003
- Chen WW, Chan DC, Donald C, Lilly MB, Kraft AS. Pim family kinases enhance tumor growth of prostate cancer cells. Mol Cancer Res 2005; 3:443-51; PMID:16123140; http://dx.doi.org/10.1158/1541-7786.MCR-05-0007
- Asano J, Nakano A, Oda A, Amou H, Hiasa M, Takeuchi K, Miki H, Nakamura S, Harada T, Fujii S, et al. The serine/threonine kinase Pim-2 is a novel anti-apoptotic mediator in myeloma cells. Leukemia 2011; 25:1182-8; PMID:21475253; http://dx.doi.org/10.1038/leu.2011.60
- Yang DQ, Kastan MB. Participation of ATM in insulin signalling through phosphorylation of eIF-4E-binding protein 1. Nat Cell Biol 2000; 2:893-8; PMID:11146653; http://dx.doi.org/10.1038/35046542
- Heesom KJ, Gampel A, Mellor H, Denton RM. Cell cycle-dependent phosphorylation of the translational repressor eIF-4E binding protein-1 (4E-BP1). Curr Biol 2001; 11:1374-9; PMID:11553333; http://dx.doi.org/10.1016/S0960-9822(01)00422-5
- Greenberg VL, Zimmer SG. Paclitaxel induces the phosphorylation of the eukaryotic translation initiation factor 4E-binding protein 1 through a Cdk1-dependent mechanism. Oncogene 2005; 24:4851-60; PMID:15897904; http://dx.doi.org/10.1038/sj.onc.1208624
- Imai Y, Gehrke S, Wang HQ, Takahashi R, Hasegawa K, Oota E, Lu B. Phosphorylation of 4E-BP by LRRK2 affects the maintenance of dopaminergic neurons in Drosophila. EMBO J 2008; 27:2432-43; PMID:18701920; http://dx.doi.org/10.1038/emboj.2008.163
- Nawijn MC, Alendar A, Berns A. For better or for worse: the role of Pim oncogenes in tumorigenesis. Nat Rev Cancer 2011; 11:23-34; PMID:21150935; http://dx.doi.org/10.1038/nrc2986
- Kremer CL, Klein RR, Mendelson J, Browne W, Samadzedeh LK, Vanpatten K, Highstrom L, Pestano GA, Nagle RB. Expression of mTOR signaling pathway markers in prostate cancer progression. Prostate 2006; 66:1203-12; PMID:16652388; http://dx.doi.org/10.1002/pros.20410
- Braunstein S, Karpisheva K, Pola C, Goldberg J, Hochman T, Yee H, Cangiarella J, Arju R, Formenti SC, Schneider RJ. A hypoxia-controlled cap-dependent to cap-independent translation switch in breast cancer. Mol Cell 2007; 28:501-12; PMID:17996713; http://dx.doi.org/10.1016/j.molcel.2007.10.019
- Karlsson E, Perez-Tenorio G, Amin R, Bostner J, Skoog L, Fornander T, Sgroi DC, Nordenskjöld B, Hallbeck AL, Stål O. The mTOR effectors 4EBP1 and S6K2 are frequently coexpressed, and associated with a poor prognosis and endocrine resistance in breast cancer: a retrospective study including patients from the randomised Stockholm tamoxifen trials. Breast Cancer Res 2013; 15:R96; PMID:24131622; http://dx.doi.org/10.1186/bcr3557
- Nathan CO, Amirghahari N, Abreo F, Rong X, Caldito G, Jones ML, Zhou H, Smith M, Kimberly D, Glass J. Overexpressed eIF4E is functionally active in surgical margins of head and neck cancer patients via activation of the Akt/mammalian target of rapamycin pathway. Clin Cancer Res 2004; 10:5820-7; PMID:15355912; http://dx.doi.org/10.1158/1078-0432.CCR-03-0483
- Martin ME, Perez MI, Redondo C, Alvarez MI, Salinas M, Fando JL. 4E binding protein 1 expression is inversely correlated to the progression of gastrointestinal cancers. Int J Biochem Cell Biol 2000; 32:633-42; PMID:10785360; http://dx.doi.org/10.1016/S1357-2725(00)00007-8
- Chao MW, Wang LT, Lai CY, Yang XM, Cheng YW, Lee KH, Pan SL, Teng CM. eIF4E binding protein 1 expression is associated with clinical survival outcomes in colorectal cancer. Oncotarget 2015; 6:24092-104; PMID:26204490; http://dx.doi.org/10.18632/oncotarget.4483
- Karlsson E, Waltersson MA, Bostner J, Perez-Tenorio G, Olsson B, Hallbeck AL, Stål O. High-resolution genomic analysis of the 11q13 amplicon in breast cancers identifies synergy with 8p12 amplification, involving the mTOR targets S6K2 and 4EBP1. Genes Chromosomes Cancer 2011; 50:775-87; http://dx.doi.org/10.1002/gcc.20900
- Ray ME, Yang ZQ, Albertson D, Kleer CG, Washburn JG, Macoska JA, Ethier SP. Genomic and expression analysis of the 8p11-12 Alicon in human breast cancer cell lines. Cancer Res 2004; 64:40-7; PMID:14729606; http://dx.doi.org/10.1158/0008-5472.CAN-03-1022
- Balakumaran BS, Porrello A, Hsu DS, Glover W, Foye A, Leung JY, Sullivan BA, Hahn WC, Loda M, Febbo PG. MYC activity mitigates response to rapamycin in prostate cancer through eukaryotic initiation factor 4E-binding protein 1-mediated inhibition of autophagy. Cancer Res 2009; 69:7803-10; PMID:19773438; http://dx.doi.org/10.1158/0008-5472.CAN-09-0910
- Yamaguchi S, Ishihara H, Yamada T, Tamura A, Usui M, Tominaga R, Munakata Y, Satake C, Katagiri H, Tashiro F, et al. ATF4-mediated induction of 4E-BP1 contributes to pancreatic β cell survival under endoplasmic reticulum stress. Cell Metab 2008; 7:269-76; PMID:18316032; http://dx.doi.org/10.1016/j.cmet.2008.01.008
- Azar R, Lasfargues C, Bousquet C, Pyronnet S. Contribution of HIF-1alpha in 4E-BP1 gene expression. Mol Cancer Res 2013; 11:54-61; PMID:23175522; http://dx.doi.org/10.1158/1541-7786.MCR-12-0095
- Musti AM, Treier M, Bohmann D. Reduced ubiquitin-dependent degradation of c-Jun after phosphorylation by MAP kinases. Science 1997; 275:400-2; PMID:8994040; http://dx.doi.org/10.1126/science.275.5298.400
- Mukherjee S, Poddar R, Deb I, Paul S. Dephosphorylation of specific sites in the kinase-specificity sequence domain leads to ubiquitin-mediated degradation of the tyrosine phosphatase STEP. Biochem J 2011; 440:115-25; PMID:21777200; http://dx.doi.org/10.1042/BJ20110240
- Chang L, Zhou B, Hu S, Guo R, Liu X, Jones SN, Yen Y. ATM-mediated serine 72 phosphorylation stabilizes ribonucleotide reductase small subunit p53R2 protein against MDM2 to DNA damage. Proc Natl Acad Sci U S A 2008; 105:18519-24; PMID:19015526; http://dx.doi.org/10.1073/pnas.0803313105
- La P, Yang G, Dennery PA. Mammalian target of rapamycin complex 1 (mTORC1)-mediated phosphorylation stabilizes ISCU protein: implications for iron metabolism. J Biol Chem 2013; 288:12901-9; PMID:23508953; http://dx.doi.org/10.1074/jbc.M112.424499
- Lee JH, Kim HS, Lee SJ, Kim KT. Stabilization and activation of p53 induced by Cdk5 contributes to neuronal cell death. J Cell Sci 2007; 120:2259-71; PMID:17591690; http://dx.doi.org/10.1242/jcs.03468
- Sherrill KW, Byrd MP, Van Eden ME, Lloyd RE. BCL-2 translation is mediated via internal ribosome entry during cell stress. J Biol Chem 2004; 279:29066-74; PMID:15123638; http://dx.doi.org/10.1074/jbc.M402727200
- Muranen T, Selfors LM, Worster DT, Iwanicki MP, Song L, Morales FC, Gao S, Mills GB, Brugge JS. Inhibition of PI3K/mTOR Leads to Adaptive Resistance in Matrix-Attached Cancer Cells. Cancer Cell 2012; 21:227-39; PMID:22340595; http://dx.doi.org/10.1016/j.ccr.2011.12.024
- Yu L, Shang ZF, Wang J, Wang H, Huang F, Zhang Z, Wang Y, Zhou J, Li S. PC-1/PrLZ confers resistance to rapamycin in prostate cancer cells through increased 4E-BP1 stability. Oncotarget 2015; 6:20356-69; PMID:26011939; http://dx.doi.org/10.18632/oncotarget.3931