ABSTRACT
Dendrite branching is an essential process for building complex nervous systems. It determines the number, distribution and integration of inputs into a neuron, and is regulated to create the diverse dendrite arbor branching patterns characteristic of different neuron types. The microtubule cytoskeleton is critical to provide structure and exert force during dendrite branching. It also supports the functional requirements of dendrites, reflected by differential microtubule architectural organization between neuron types, illustrated here for sensory neurons. Both anterograde and retrograde microtubule polymerization occur within growing dendrites, and recent studies indicate that branching is enhanced by anterograde microtubule polymerization events in nascent branches. The polarities of microtubule polymerization events are regulated by the position and orientation of microtubule nucleation events in the dendrite arbor. Golgi outposts are a primary microtubule nucleation center in dendrites and share common nucleation machinery with the centrosome. In addition, pre-existing dendrite microtubules may act as nucleation sites. We discuss how balancing the activities of distinct nucleation machineries within the growing dendrite can alter microtubule polymerization polarity and dendrite branching, and how regulating this balance can generate neuron type-specific morphologies.
For the brain to function correctly, neurons must connect through dendrites to receive inputs. The branching patterns and sizes of individual neuronal dendrite arbors determine the number and distribution of these inputs, and also delineate their integration.Citation1,2 Alterations in arbor patterning occur in multiple neuropsychiatric and neurological disorders including intellectual disability syndromes, autism spectrum disorders, schizophrenia, and neurodegenerative diseases.Citation3
A nascent neuron has a simple morphology and must undergo a complex differentiation process to create a highly branched dendrite arbor.Citation4 Moreover, as beautifully illustrated more than 125 years ago by Ramón y Cajal,Citation5 the dendrite differentiation process must be organized in order to create arbor morphologies that fit the specific functional requirements of that neuron type.Citation1 Final arbor morphology is produced by integrating the activities of cell surface receptors and adhesion proteins that regulate dendrite targeting with those of cytoskeletal factors that regulate dendrite branching and outgrowth.Citation4
The microtubule cytoskeleton is critical to provide structure and to exert force in order to create, stabilize, and remodel dendrites during development.Citation6,7 Microtubules also act as tracks for cargo transport by microtubule-based motor proteins.Citation8 In addition, they regulate neuronal plasticity through activity-dependent remodeling, with microtubule invasion events driving spine enlargement and increases in post synaptic density protein levels.Citation9-12 Furthermore, microtubules support channel activityCitation13; for example, the coupling of the transient receptor potential family mechanotransduction channel NompC to an array of microtubules conveys force to gate channel activation.Citation14-16 Dendrite microtubule architecture differs between neuron typesCitation15,17-20 in order to support the specific functional requirements of each type. We illustrate that here for the specialized dendrites of class IV nociceptiveCitation21 and class I proprioceptiveCitation22 Drosophila body wall neurons. The dendrites of these two neuron types have very different microtubule configurations and microtubule densities, features which reflect their differing sensory modalities ().
Figure 1. Microtubule organization varies in the dendrites of different neuron types. (A) The dendrites of Drosophila body wall nociceptive class IV neurons contain a sparse microtubule organization (black arrowheads). (B) The dendrites of Drosophila body wall proprioceptive class I neuron contain dense arrays of microtubules (black arrowhead), which are interlinked by bridges (whited arrowheads). In addition, different modes of linkage between the neurons and the body wall also highlight their divergent functions. (C) Class IV neurons have dendrites embedded in the epithelial cells of the body wall.Citation82,83 (D) Class I dendrite microtubules are embedded in a dense matrix (green arrowheads), and attach to the surface of the epithelial cells by pads of electron dense material (red arrowheads). This specialized architecture in class I neurons is similar to that found in other cells active in mechanotransduction.Citation15,16,84,85 Pseudo-coloration in panels C-E: blue – dendrite; yellow – epithelial cell; uncolored – basement membrane. Scale bars: 0.2 μm.
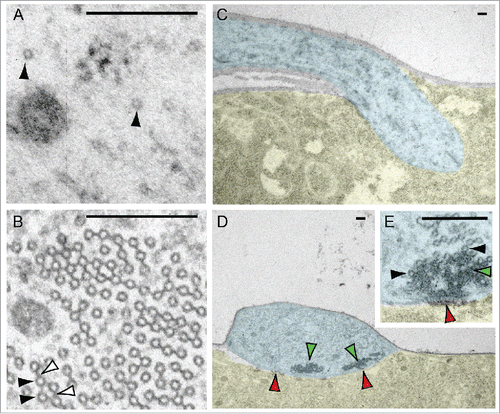
Microtubules are polarized filaments. They are constructed from heterodimers of α- and β-tubulin that attach in a head-to-tail manner, pack laterally, usually with 13 heterodimers (13 protofilaments) per cross section, and fold to form a tube.Citation23,24 In relatively thin cellular processes such as dendrites the length of microtubules exceeds the diameter of the cell, and therefore microtubules are necessarily orientated either plus-end distal and anterograde polymerizing (away from the cell body), or minus-end distal and retrograde polymerizing (toward the cell body).Citation25 Dendrites contain both possible polarities, while microtubules in the mature axon are predominantly oriented in a plus-end distal orientation.Citation25 Because microtubule-based motor proteins are either plus- or minus-end directed, the presence of minus-end distal microtubules in dendrites enables selective delivery of cargo (for example, by dynein motor proteins) facilitating neuron compartmentalization.Citation8,26 Both plus- or minus-end directed microtubule arrays are established in dendrites from the earliest stages of neurite outgrowth.Citation17,20,27-29 One mechanism to achieve this organization is via the action of microtubule motor proteins. Motor proteins either anchored to the cell cortex or spanning two microtubules can facilitate the sliding of preassembled microtubules from the soma into the dendrite.Citation6,25 In addition, as the dendrite arbor elaborates and after it reaches the mature state, microtubule motor proteins maintain and enhance the minus-end distal population by selectively removing plus-end distal microtubulesCitation30 and guiding new polymerization events passing through dendrite branch points in the retrograde direction.Citation31 A second mechanism is via the nucleation of microtubules, i.e., de novo initiation of microtubule polymerization within a nascent dendrite branch. In this article we focus on recent studies that address the mechanisms of microtubule nucleation in dendrites and the role of this process in shaping the branching pattern of the dendrite arbor.
Microtubule seeds in dendrites
Microtubule nucleation is a kinetically unfavorable process; it requires both a seed structure and accessory factors that increase nucleation activity.Citation32 The microtubule seed is commonly provided by a template of γ-tubulin and associated proteins in the γ-tubulin ring complex (γ-TuRC).Citation23,24 γ-tubulin provides the initiation template for polymerization.Citation33 γ-TuRC is central to the majority of cellular microtubule nucleation processes, and it is localized and activated to create microtubule organizing centers within the cell.Citation23,24 Loss of γ-tubulin or γ-TuRC factors reduces microtubule polymerization frequency in both dendrites and axons of hippocampal neuron cultures and in terminal dendrite branches of Drosophila class IV sensory neurons.Citation34,35
Pre-existing microtubule fragments can also act as seeds. In this case nucleation occurs by binding at the β-tubulin surface on the pre-existing fragment; this event is independent of γ-tubulin.Citation32,36 Microtubule fragment seeds are generated when microtubule-severing enzymes break up pre-existing microtubules.Citation37,38 Evidence that microtubule fragment-based nucleation occurs in dendrites comes from the genetic disruption of severing enzymes Spastin, Katanin 60 and Katanin p60-like1, all of which are required for correct dendrite patterning.Citation39-42 Moreover, loss of Katanin p60-like1 reduces the frequency of microtubule polymerization events in the terminal branches of class IV neurons.Citation39 The activity of microtubule-severing enzymes is not restricted to dendrites; they are also required for axonal growth.Citation40,42,43 Spatial regulation of microtubule-severing enzyme activity in neurons is controlled by mechanisms such as the binding of the microtubule-associated protein Tau to microtubules in hippocampal neuron axonsCitation44 and the post-translational modification of dendrite microtubules (polyglutamylation).Citation45
γ-tubulin and microtubule-severing pathways interact in non-neuronal cells, for example to organize meiotic spindle microtubules in C. elegans.Citation46 The extent to which microtubule-severing pathways contribute to dendrite microtubule nucleation and furthermore how γ-tubulin and microtubule-severing pathways interact to shape dendrites remain unclear and require further investigation. On the other hand, recent studies have provided new information about where γ-tubulin-mediated nucleation events occur in developing dendrites, and have revealed molecular mechanisms controlling these nucleation events.
Microtubule nucleation at Golgi outposts
During cell division, the centrosome recruits γ-TuRC to act as the primary microtubule nucleation center. The centrosome also supports nucleation in nascent neuronsCitation47; however, this activity is rapidly lost, and dendrite elaboration occurs after the centrosome has stopped functioning as a microtubule nucleation site.Citation34,47,48 The centrosome maintains a second function as a signal transduction hub, which also influences dendrite growth.Citation49 In the differentiating dendrite, microtubule nucleation and polymerization events occur away from the centrosome and throughout the growing arbor.Citation20,27-29,35
Although microtubule nucleation events are spread throughout the arbor, specific foci repeatedly give rise to microtubules, suggesting that particular subcellular structures within the dendrite have microtubule nucleating activity. Other structures, in addition to the centrosome, can focus γ-tubulin-mediated microtubule nucleation. During mitosis the chromatin and kinetochores act as sites of microtubule nucleation, as do the microtubules of the spindle.Citation23 Membranous organelles also act as nucleation sites in several cell types, including the nuclear envelope in muscle cells, the cell cortex in plant cells, and somatic Golgi.Citation23,50 To identify structures associated with microtubule nucleation within dendrites, Ori-McKenney and colleagues used Drosophila sensory neurons as a model and showed microtubule nucleation at Golgi fragments situated within dendrites,Citation35 called Golgi outposts.Citation51 Endosomes and mitochondria, other common membrane-rich organelles in dendrites, were not found to be sites of nucleation.Citation35
Notably, while γ-tubulin is used in both dendrites and axons to seed microtubules,Citation34 Golgi outposts have only been detected in the dendrites.Citation51 Whether there are regulatory mechanisms to control the positions of microtubule nucleation in the elongating axon is as yet unclear.
Dendrite Golgi outposts and centrosomes share common molecular machinery for organizing microtubule nucleation (). During cell division, Pericentrin/AKAP450 (Pericentrin-like protein (Plp) in Drosophila) and CDK5RAP2 (Centrosomin (Cnn) in Drosophila) cooperate to recruit γ-TuRC to the pericentriolar material (PCM) surrounding the centrosome.Citation52 Studies in mammalian neurons have shown γ-tubulin and PCM material are lost from the centrosome during early neuron differentiation, and PCM components are transported into developing dendrites.Citation53,54 In Drosophila sensory neurons, Cnn and Plp link microtubule nucleation to Golgi outposts.Citation20,35 AKAP450/Plp and CDK5RAP2/Cnn localize to somatic Golgi,Citation50 and Cnn localizes to Golgi outposts in Drosophila sensory neurons.Citation20 Plp promotes the association of Cnn with the Golgi outpost,Citation20 similar to its recruitment and organization roles at the centrosome.Citation52
Figure 2. (A) The centrosome (the structure of interphase centrosome is illustratedCitation86) and (B) somatic Golgi / dendritic Golgi outposts share common molecular machinery for microtubule nucleation. (C) Microtubule nucleation from pre-existing microtubules by the Augmin complex. Illustrations adapted from references.Citation50,86,87
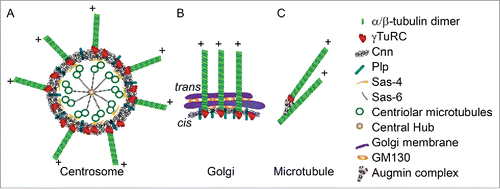
At the centrosome the PCM recruits and concentrates γ-tubulin for microtubule nucleation.Citation52 However, no increase in γ-tubulin has been observed at either somatic or dendritic Golgi,Citation50 and removing Golgi outposts from dendrites using activated kinesin constructs did not disrupt γ-tubulin localization.Citation55 Thus the microtubule nucleation machinery associated with Golgi outposts may not function through the same γ-tubulin recruitment mechanism as the PCM. A recent model proposes that Golgi drive microtubule nucleation by localizing the accessory factors that increase the nucleation activity of γ-TuRC, while γ-TuRC itself remains unenriched in the adjacent cytoplasm.Citation50 CDK5RAP2/Cnn is one potential activating factor as it has been shown to activate γ–TuRC in vitro.Citation56
Golgi outpost microtubule nucleation activity also depends upon outpost structure. In dendrites the biochemically distinct medial and trans compartments, which are characteristically combined into a stack in the soma, are often disconnected into single-compartment Golgi outposts.Citation57 Single-compartment Golgi outposts can nucleate microtubules, but fusion into a multi-compartment structure within dendrites increases outpost microtubule nucleation activity.Citation57 The Golgi structural protein GM130 localizes to outposts and promotes the fusion of single-compartment outposts into a multi-compartment structure.Citation57 In addition to this outpost fusion function, GM130 may also recruit AKAP450/Plp to outposts, as it has been shown to link AKAP450/Plp to somatic Golgi for microtubule nucleation.Citation58
The role of alternative nucleation sites in orienting microtubule nucleation in the dendrite
The orientation of γ-tubulin-mediated microtubule nucleation will affect microtubule polymerization polarities within the dendrite arbor, and a recent study has shown that genetic mutants raising or lowering γ-tubulin activity altered the balance of these polarities.Citation55 The direction of polymerization is important during dendrite differentiation. Immature differentiating dendrites have a larger balance of anterograde distal microtubules than mature dendrites and anterograde polymerization events are concentrated in the growing terminal regions.Citation17,27-29,35 The polymerization of plus-end distal microtubules drives neurite outgrowth during neuron differentiation,Citation59 and live imaging of growing dendrites in vivo shows anterograde polymerizing microtubules extending the termini of emerging branches leading to outgrowth and stabilizationCitation20,35 ().
Figure 3. (A) Anterograde polymerizing microtubule invasion into nascent dendrite branches drives outgrowth. Microtubules nucleated within termini or at the branch site are more likely to drive an extension event.Citation35 At a lower frequency, similar to the invasion of microtubules into the spines of mature dendrites,Citation88 both retrograde- or anterograde-oriented microtubules in the main branch enter nascent dendrite branches, becoming anterograde-directed within the termini.Citation35 (B) The relative contributions of Golgi- and non-Golgi-derived polymerizing microtubules to the dendrite termini. Golgi outpost-associated events contribute approximately equally to both anterograde and retrograde polymerizing microtubules. In cnn mutant neurons, there is an increase in non-Golgi microtubules that polymerize in the anterograde direction; this increase is not accounted for by the loss of Golgi outpost-associated retrograde polymerization events. These data suggest that nucleation machinery at outposts might counteract the activity of additional factors that promote anterograde bias. (C) A model for how arbor complexity can be regulated by modulating the balance between these distinct Golgi- and non-Golgi-associated pathways that orient microtubule polymerization. A single outpost is shown here; it should be noted, however, that throughout the arbor individual Golgi outposts can give rise to either repeated anterograde or retrograde nucleation events. During dendrite outgrowth, Cnn recruits microtubule nucleation to Golgi outposts to suppress an activity (that may be due to Augmin, here illustrated by the Drosophila Augmin component WacCitation62), which in turn promotes anterograde polymerization.
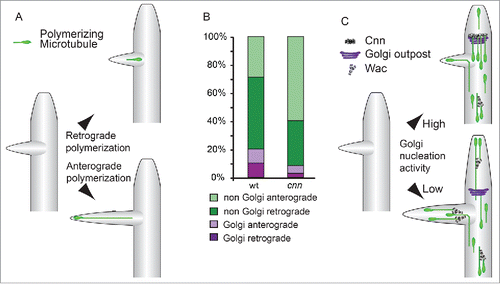
Golgi outposts bias the direction of microtubule polymerization at the local level. Somatic Golgi nucleate microtubules that only polymerize in the same orientation as the trans faceCitation50 (). Similarly, repeated nucleation from an individual Golgi outpost produces a unidirectional sequence of polymerization events.Citation20,35 Nevertheless, when summed through all dendrite termini, Golgi outpost-associated nucleation events contribute approximately equally to both anterograde and retrograde polymerizing microtubules (). Although individual outposts impart polarity on microtubule nucleation, this process is unlikely to support enrichment of anterograde events at dendrite termini. Instead, these data suggest that the orientations of individual Golgi outposts are mixed, approximately half oriented to give rise to anterograde-directed nucleation and half to retrograde-directed nucleation. Further experiments are required to clarify the relationship between outpost polarity and microtubule nucleation polarity. Because the organization of dendrite Golgi outposts differs from somatic Golgi,Citation57 especially for single-compartment outposts, it is possible that microtubule nucleation might be able to initiate on either surface of the outpost (and continue solely from that surface).
Recent data suggest that the interplay between alternative sites of dendrite microtubule nucleation regulates the distribution of microtubule polymerization polarities in the developing dendrite terminiCitation20 (). This is analogous to how the interactions of centrosome, chromatin, and microtubule-dependent microtubule nucleation mechanisms shape the mitotic spindle.Citation60 Decoupling nucleation events from Golgi outposts shifts the balance of microtubule polymerization polarities in dendrite termini in the anterograde direction. Notably the increase in anterograde polymerization events occurs primarily in the population of microtubules that are not nucleating at Golgi outposts ().Citation20 Microtubule nucleation is catalyzed from the sides of microtubules during the maturation of the mitotic spindle by the Augmin complex,Citation61 and loss of the Drosophila Augmin component Wee Augmin (Wac)Citation62 leads to a specific reduction of the additional anterograde polymerization events that are caused when nucleation is decoupled from Golgi.Citation20 Augmin nucleates new microtubules with the same polarity as the pre-existing microtubule templateCitation61 (). Therefore Wac activity might promote anterograde polymerization in dendrite termini through nucleation events that maintain the existing anterograde bias of microtubules that had initially invaded the nascent branchCitation59,63 (). Altering the relative Cnn and Wac levels in developing dendrites regulates dendrite branchingCitation20 (). As branching can be controlled by modulating the balance between distinct microtubule nucleation machineries, this suggests a mechanism for neuron type-specific arbor patterning.
Microtubule nucleation control for dendrite morphogenesis
Neuron diversification is driven by transcription factors that regulate multiple aspects of neuron character including dendrite arbor morphology.Citation4,64 For example, in cortical pyramidal neurons the bHLH transcription factor Ngn2 specifies the characteristic primary apical dendrite.Citation65 The homeodomain factor Cux2 also promotes branch complexity in the apical part of the dendrite arbor, while Cux1 regulates the basal compartment.Citation66 In the somatosensory cortex, the BTB-domain factor BTBD3 regulates the pruning of dendrite branches to enable the establishment of the correct receptive field.Citation67
The role of transcription factors in specifying differences in arbor morphology between different types of Drosophila body wall sensory neurons has been extensively studied over the last decade.Citation4,64 Sensory neuron dendrite patterning is defined by the combinatorial activities of a group of transcription factors, including the homeodomain factor Cut (homolog of Cux1 and 2), the BTB-domain factors Abrupt and Lola, the Krüppel-like factor Dar1, the Iroquois factor Mirror, the bHLH-PAS factor Spineless, the PRDM factor Hamlet, and the EBF factor Knot.Citation41,68-78 These studies have demonstrated that individual transcription factors control specific aspects of dendrite cytoskeleton organization; for example, Cut and Lola were shown to regulate actin organization,Citation41,76,78 while Knot, Dar1, and Abrupt regulate microtubule organization.Citation20,41,77
A complex interacting network of microtubule regulatory events occurs within the differentiating neuron,Citation7 and consequently, a microtubule regulator that is an effector of a transcription factor need not be under the direct transcriptional control of that factor. Nonetheless, elucidating a transcriptional relationship is a valuable approach to identify molecular mechanisms shaping the arbor.Citation64 This approach revealed a key mechanism of how the transcription factor Abrupt represses dendrite branching. In Drosophila class I neurons, Abrupt prevents branching to create the simple arbor morphology characteristic of this class.Citation20,74,75 Cnn was found to be a transcriptional target of Abrupt. Loss of Abrupt leads to Cnn downregulation and the decoupling of microtubule nucleation from Golgi outposts, thereby causing an increase in anterograde microtubule nucleation events and the de-repression of branching.Citation20 In another transcriptional pathway, Cut, which promotes dendrite branching,Citation41,73 regulates Sec31 and other components of the COPII secretory transport pathway to promote Golgi outpost formationCitation79 (the outcome on dendrite microtubule nucleation was not examined).
Golgi outpost compartment organization is different between neuron types, raising the possibility that it may also be used to control neuron type-specific patterning. In class III sensory neurons, outposts exist in both single- and multi-compartment forms.Citation57 In class I neurons, however, although the Golgi structural protein GM130 is present in the dendrites,Citation79 only medial-Golgi markers were detected.Citation20,55 The transcription factor Dar1 is required to create complex dendrite arbor structures. Dar1 also drives the formation of multi-compartment Golgi outposts; the transcriptional pathway responsible for this has not been determined.Citation57 Nevertheless, because multi-compartment outposts drive a higher level of nucleation than single-compartment outposts, controlling differences in Golgi outpost composition between neuron types should be expected to alter microtubule nucleation parameters.Citation57
Taken together these recent studies highlight how the network of microtubule nucleation machineries in growing dendrites can provide a rich substrate through which intrinsic transcription factors modulate branching to create the characteristic dendrite arbor morphologies of different neuron types.
Conclusions
In this article we reviewed recent studies that address the mechanisms and roles of microtubule nucleation during dendrite development, a critical aspect of a complex interacting network of microtubule regulatory events occurring within the differentiating neuron.Citation6,7 The regulation of microtubule nucleation events within a developing dendrite creates a system for guiding the direction of microtubule polymerization at the termini of the growing arbor.Citation20,35 Golgi outposts provide a local guide for microtubule nucleation in dendrites, and they interact with other nucleation sites in order to fine-tune the amplitude and polarity of dendrite microtubule nucleation events.Citation20,35,57 Dendrite microtubules themselves may also act as a nucleation site through the activity of the Augmin complex,Citation20,62 an important question for further study. Diversity in neuron arbors can be achieved by modulating the dendrite nucleation machinery, for example by regulating the activity of Golgi outposts as nucleation sites.Citation20,57
Methods
For transmission electron microscopy, neurons were marked by 2-21-gal4,UAS-lacZ or ppk-Gal4,UAS-lacZ. Third instar larvae were dissected as previously described,Citation80 then fixed in 2.5% glutaraldehyde for 20 min, washed in 0.1 M Tris-HCl (pH 7.3) containing 2 mM MgCl2 for 3 × 5 min, and processed as described previously.Citation81 Following identification of the labeled neuron soma, serial sections were cut and microscopy was performed using a JEOL JEM-1200EXII. Microtubule nucleation localization and polarity quantifications were extracted from previously reported data sets.Citation20
Disclosure of potential conflicts of interest
No potential conflicts of interest were disclosed.
References
- London M, Hausser M. Dendritic computation. Annu Rev Neurosci 2005; 28:503-32; PMID:16033324; http://dx.doi.org/10.1146/annurev.neuro.28.061604.135703
- Spruston N. Pyramidal neurons: dendritic structure and synaptic integration. Nat Rev Neurosci 2008; 9:206-21; PMID:18270515; http://dx.doi.org/10.1038/nrn2286
- Kulkarni VA, Firestein BL. The dendritic tree and brain disorders. Mol Cell Neurosci 2012; 50:10-20; PMID:22465229; http://dx.doi.org/10.1016/j.mcn.2012.03.005
- Lefebvre JL, Sanes JR, Kay JN. Development of Dendritic Form and Function. Annu Rev Cell Dev Biol 2015; 31:741-77; PMID:26422333; http://dx.doi.org/10.1146/annurev-cellbio-100913-013020
- Cajal SR. Estructura de los centros neviosos de las aves. Rev Trim Histol Normal Patologica 1888; 1:1-10
- Kapitein LC, Hoogenraad CC. Building the Neuronal Microtubule Cytoskeleton. Neuron 2015; 87:492-506; PMID:26247859; http://dx.doi.org/10.1016/j.neuron.2015.05.046
- Poulain FE, Sobel A. The microtubule network and neuronal morphogenesis: Dynamic and coordinated orchestration through multiple players. Mol Cell Neurosci 2010; 43:15-32; PMID:19660553; http://dx.doi.org/10.1016/j.mcn.2009.07.012
- Kapitein LC, Hoogenraad CC. Which way to go? Cytoskeletal organization and polarized transport in neurons. Mol Cell Neurosci 2011; 46:9-20; PMID:20817096; http://dx.doi.org/10.1016/j.mcn.2010.08.015
- Hu X, Viesselmann C, Nam S, Merriam E, Dent EW. Activity-dependent dynamic microtubule invasion of dendritic spines. J Neurosci 2008; 28:13094-105; PMID:19052200; http://dx.doi.org/10.1523/JNEUROSCI.3074-08.2008
- Merriam EB, Lumbard DC, Viesselmann C, Ballweg J, Stevenson M, Pietila L, Hu X, Dent EW. Dynamic microtubules promote synaptic NMDA receptor-dependent spine enlargement. PLoS One 2011; 6:e27688; PMID:22096612; http://dx.doi.org/10.1371/journal.pone.0027688
- Jaworski J, Kapitein LC, Gouveia SM, Dortland BR, Wulf PS, Grigoriev I, Camera P, Spangler SA, Di Stefano P, Demmers J, et al. Dynamic microtubules regulate dendritic spine morphology and synaptic plasticity. Neuron 2009; 61:85-100; PMID:19146815; http://dx.doi.org/10.1016/j.neuron.2008.11.013
- Hu X, Ballo L, Pietila L, Viesselmann C, Ballweg J, Lumbard D, Stevenson M, Merriam E, Dent EW. BDNF-induced increase of PSD-95 in dendritic spines requires dynamic microtubule invasions. J Neurosci 2011; 31:15597-603; PMID:22031905; http://dx.doi.org/10.1523/JNEUROSCI.2445-11.2011
- Smani T, Dionisio N, Lopez JJ, Berna-Erro A, Rosado JA. Cytoskeletal and scaffolding proteins as structural and functional determinants of TRP channels. Biochim Biophys Acta 2014; 1838:658-64; PMID:23333715; http://dx.doi.org/10.1016/j.bbamem.2013.01.009
- Zhang W, Cheng LE, Kittelmann M, Li J, Petkovic M, Cheng T, Jin P, Guo Z, Gopfert MC, Jan LY, et al. Ankyrin Repeats Convey Force to Gate the NOMPC Mechanotransduction Channel. Cell 2015; 162:1391-403; PMID:26359990; http://dx.doi.org/10.1016/j.cell.2015.08.024
- Liang X, Madrid J, Gartner R, Verbavatz JM, Schiklenk C, Wilsch-Brauninger M, Bogdanova A, Stenger F, Voigt A, Howard J. A NOMPC-dependent membrane-microtubule connector is a candidate for the gating spring in fly mechanoreceptors. Curr Biol 2013; 23:755-63; PMID:23583554; http://dx.doi.org/10.1016/j.cub.2013.03.065
- Liang X, Madrid J, Howard J. The microtubule-based cytoskeleton is a component of a mechanical signaling pathway in fly campaniform receptors. Biophys J 2014; 107:2767-74; PMID:25517144; http://dx.doi.org/10.1016/j.bpj.2014.10.052
- Baas PW, Deitch JS, Black MM, Banker GA. Polarity orientation of microtubules in hippocampal neurons: uniformity in the axon and nonuniformity in the dendrite. Proc Natl Acad Sci U S A 1988; 85:8335-9; PMID:3054884; http://dx.doi.org/10.1073/pnas.85.21.8335
- Burton PR. Dendrites of mitral cell neurons contain microtubules of opposite polarity. Brain Res 1988; 473:107-15; PMID:3264743; http://dx.doi.org/10.1016/0006-8993(88)90321-6
- Burton PR. Ultrastructure of the olfactory neuron of the bullfrog: the dendrite and its microtubules. J Comp Neurol 1985; 242:147-60; PMID:3878850; http://dx.doi.org/10.1002/cne.902420202
- Yalgin C, Ebrahimi S, Delandre C, Yoong LF, Akimoto S, Tran H, Amikura R, Spokony R, Torben-Nielsen B, White KP, et al. Centrosomin represses dendrite branching by orienting microtubule nucleation. Nat Neurosci 2015; 18:1437-45; PMID:26322925; http://dx.doi.org/10.1038/nn.4099
- Hwang RY, Zhong L, Xu Y, Johnson T, Zhang F, Deisseroth K, Tracey WD. Nociceptive neurons protect Drosophila larvae from parasitoid wasps. Curr Biol 2007; 17:2105-16; PMID:18060782; http://dx.doi.org/10.1016/j.cub.2007.11.029
- Hughes CL, Thomas JB. A sensory feedback circuit coordinates muscle activity in Drosophila. Mol Cell Neurosci 2007; 35:383-96; PMID:17498969; http://dx.doi.org/10.1016/j.mcn.2007.04.001
- Petry S, Vale RD. Microtubule nucleation at the centrosome and beyond. Nat Cell Biol 2015; 17:1089-93; PMID:26316453; http://dx.doi.org/10.1038/ncb3220
- Kollman JM, Merdes A, Mourey L, Agard DA. Microtubule nucleation by gamma-tubulin complexes. Nat Rev Mol Cell Biol 2011; 12:709-21; PMID:21993292; http://dx.doi.org/10.1038/nrm3209
- Baas PW, Lin S. Hooks and comets: The story of microtubule polarity orientation in the neuron. Dev Neurobiol 2011; 71:403-18; PMID:21557497; http://dx.doi.org/10.1002/dneu.20818
- Kapitein LC, Schlager MA, Kuijpers M, Wulf PS, van Spronsen M, MacKintosh FC, Hoogenraad CC. Mixed microtubules steer dynein-driven cargo transport into dendrites. Curr Biol 2010; 20:290-9; PMID:20137950; http://dx.doi.org/10.1016/j.cub.2009.12.052
- Yau KW, Schatzle P, Tortosa E, Pages S, Holtmaat A, Kapitein LC, Hoogenraad CC. Dendrites In Vitro and In Vivo Contain Microtubules of Opposite Polarity and Axon Formation Correlates with Uniform Plus-End-Out Microtubule Orientation. J Neurosci 2016; 36:1071-85; PMID:26818498; http://dx.doi.org/10.1523/JNEUROSCI.2430-15.2016
- Hill SE, Parmar M, Gheres KW, Guignet MA, Huang Y, Jackson FR, Rolls MM. Development of dendrite polarity in Drosophila neurons. Neural Dev 2012; 7:34; PMID:23111238; http://dx.doi.org/10.1186/1749-8104-7-34
- Kollins KM, Bell RL, Butts M, Withers GS. Dendrites differ from axons in patterns of microtubule stability and polymerization during development. Neural Dev 2009; 4:26; PMID:19602271; http://dx.doi.org/10.1186/1749-8104-4-26
- Yan J, Chao DL, Toba S, Koyasako K, Yasunaga T, Hirotsune S, Shen K. Kinesin-1 regulates dendrite microtubule polarity in Caenorhabditis elegans. Elife 2013; 2:e00133; PMID:23482306; http://dx.doi.org/10.7554/eLife.00133
- Mattie FJ, Stackpole MM, Stone MC, Clippard JR, Rudnick DA, Qiu Y, Tao J, Allender DL, Parmar M, Rolls MM. Directed microtubule growth, +TIPs, and kinesin-2 are required for uniform microtubule polarity in dendrites. Curr Biol 2010; 20:2169-77; PMID:21145742; http://dx.doi.org/10.1016/j.cub.2010.11.050
- Wieczorek M, Bechstedt S, Chaaban S, Brouhard GJ. Microtubule-associated proteins control the kinetics of microtubule nucleation. Nat Cell Biol 2015; 17:907-16; PMID:26098575; http://dx.doi.org/10.1038/ncb3188
- Kollman JM, Polka JK, Zelter A, Davis TN, Agard DA. Microtubule nucleating gamma-TuSC assembles structures with 13-fold microtubule-like symmetry. Nature 2010; 466:879-82; PMID:20631709; http://dx.doi.org/10.1038/nature09207
- Yau KW, van Beuningen SF, Cunha-Ferreira I, Cloin BM, van Battum EY, Will L, Schatzle P, Tas RP, van Krugten J, Katrukha EA, et al. Microtubule Minus-End Binding Protein CAMSAP2 Controls Axon Specification and Dendrite Development. Neuron 2014; 82:1058-73; PMID:24908486; http://dx.doi.org/10.1016/j.neuron.2014.04.019
- Ori-McKenney KM, Jan LY, Jan YN. Golgi outposts shape dendrite morphology by functioning as sites of acentrosomal microtubule nucleation in neurons. Neuron 2012; 76:921-30; PMID:23217741; http://dx.doi.org/10.1016/j.neuron.2012.10.008
- Mitchison TJ. Localization of an exchangeable GTP binding site at the plus end of microtubules. Science 1993; 261:1044-7; PMID:8102497; http://dx.doi.org/10.1126/science.8102497
- Sharp DJ, Ross JL. Microtubule-severing enzymes at the cutting edge. J Cell Sci 2012; 125:2561-9; PMID:22595526; http://dx.doi.org/10.1242/jcs.101139
- Roll-Mecak A, McNally FJ. Microtubule-severing enzymes. Curr Opin Cell Biol 2010; 22:96-103; PMID:19963362; http://dx.doi.org/10.1016/j.ceb.2009.11.001
- Stewart A, Tsubouchi A, Rolls MM, Tracey WD, Sherwood NT. Katanin p60-like1 promotes microtubule growth and terminal dendrite stability in the larval class IV sensory neurons of Drosophila. J Neurosci 2012; 32:11631-42; PMID:22915107; http://dx.doi.org/10.1523/JNEUROSCI.0729-12.2012
- Mao CX, Xiong Y, Xiong Z, Wang Q, Zhang YQ, Jin S. Microtubule-severing protein Katanin regulates neuromuscular junction development and dendritic elaboration in Drosophila. Development 2014; 141:1064-74; PMID:24550114; http://dx.doi.org/10.1242/dev.097774
- Jinushi-Nakao S, Arvind R, Amikura R, Kinameri E, Liu AW, Moore AW. Knot/Collier and cut control different aspects of dendrite cytoskeleton and synergize to define final arbor shape. Neuron 2007; 56:963-78; PMID:18093520; http://dx.doi.org/10.1016/j.neuron.2007.10.031
- Yu W, Qiang L, Solowska JM, Karabay A, Korulu S, Baas PW. The microtubule-severing proteins spastin and katanin participate differently in the formation of axonal branches. Mol Biol Cell 2008; 19:1485-98; PMID:18234839; http://dx.doi.org/10.1091/mbc.E07-09-0878
- Leo L, Yu W, D'Rozario M, Waddell EA, Marenda DR, Baird MA, Davidson MW, Zhou B, Wu B, Baker L, et al. Vertebrate Fidgetin Restrains Axonal Growth by Severing Labile Domains of Microtubules. Cell Rep 2015; 12:1723-30; PMID:26344772; http://dx.doi.org/10.1016/j.celrep.2015.08.017
- Qiang L, Yu W, Andreadis A, Luo M, Baas PW. Tau protects microtubules in the axon from severing by katanin. J Neurosci 2006; 26:3120-9; PMID:16554463; http://dx.doi.org/10.1523/JNEUROSCI.5392-05.2006
- Zempel H, Luedtke J, Kumar Y, Biernat J, Dawson H, Mandelkow E, Mandelkow EM. Amyloid-β oligomers induce synaptic damage via Tau-dependent microtubule severing by TTLL6 and spastin. EMBO J 2013; 32:2920-37; PMID:24065130; http://dx.doi.org/10.1038/emboj.2013.207
- McNally K, Audhya A, Oegema K, McNally FJ. Katanin controls mitotic and meiotic spindle length. J Cell Biol 2006; 175:881-91; PMID:17178907; http://dx.doi.org/10.1083/jcb.200608117
- Stiess M, Maghelli N, Kapitein LC, Gomis-Ruth S, Wilsch-Brauninger M, Hoogenraad CC, Tolic-Norrelykke IM, Bradke F. Axon extension occurs independently of centrosomal microtubule nucleation. Science 2010; 327:704-7; PMID:20056854; http://dx.doi.org/10.1126/science.1182179
- Nguyen MM, Stone MC, Rolls MM. Microtubules are organized independently of the centrosome in Drosophila neurons. Neural Dev 2011; 6:38; PMID:22145670; http://dx.doi.org/10.1186/1749-8104-6-38
- Yamada T, Yang Y, Bonni A. Spatial organization of ubiquitin ligase pathways orchestrates neuronal connectivity. Trends Neurosci 2013; 36:218-26; PMID:23332798; http://dx.doi.org/10.1016/j.tins.2012.12.004
- Sanders AA, Kaverina I. Nucleation and Dynamics of Golgi-derived Microtubules. Front Neurosci 2015; 9:431; PMID:26617483; http://dx.doi.org/10.3389/fnins.2015.00431
- Horton AC, Ehlers MD. Dual modes of endoplasmic reticulum-to-Golgi transport in dendrites revealed by live-cell imaging. J Neurosci 2003; 23:6188-99; PMID:12867502
- Conduit PT, Wainman A, Raff JW. Centrosome function and assembly in animal cells. Nat Rev Mol Cell Biol 2015; 16:611-24; PMID:26373263; http://dx.doi.org/10.1038/nrm4062
- Ferreira A, Palazzo RE, Rebhun LI. Preferential dendritic localization of pericentriolar material in hippocampal pyramidal neurons in culture. Cell Motil Cytoskeleton 1993; 25:336-44; PMID:8402954; http://dx.doi.org/10.1002/cm.970250404
- Yonezawa S, Shigematsu M, Hirata K, Hayashi K. Loss of gamma-tubulin, GCP-WD/NEDD1 and CDK5RAP2 from the Centrosome of Neurons in Developing Mouse Cerebral and Cerebellar Cortex. Acta Histochem Cytochem 2015; 48:145-52; PMID:26633906; http://dx.doi.org/10.1267/ahc.15023
- Nguyen MM, McCracken CJ, Milner ES, Goetschius DJ, Weiner AT, Long MK, Michael NL, Munro S, Rolls MM. gamma-tubulin controls neuronal microtubule polarity independently of Golgi outposts. Mol Biol Cell 2014; 25(13):2039-50; PMID:24807906
- Choi YK, Liu P, Sze SK, Dai C, Qi RZ. CDK5RAP2 stimulates microtubule nucleation by the gamma-tubulin ring complex. J Cell Biol 2010; 191:1089-95; PMID:21135143; http://dx.doi.org/10.1083/jcb.201007030
- Zhou W, Chang J, Wang X, Savelieff MG, Zhao Y, Ke S, Ye B. GM130 Is Required for Compartmental Organization of Dendritic Golgi Outposts. Curr Biol 2014; 24:1227-33; PMID:24835455; http://dx.doi.org/10.1016/j.cub.2014.04.008
- Rivero S, Cardenas J, Bornens M, Rios RM. Microtubule nucleation at the cis-side of the Golgi apparatus requires AKAP450 and GM130. EMBO J 2009; 28:1016-28; PMID:19242490; http://dx.doi.org/10.1038/emboj.2009.47
- Lewis TL, Jr., Courchet J, Polleux F. Cell biology in neuroscience: Cellular and molecular mechanisms underlying axon formation, growth, and branching. J Cell Biol 2013; 202:837-48; PMID:24043699; http://dx.doi.org/10.1083/jcb.201305098
- Hayward D, Metz J, Pellacani C, Wakefield JG. Synergy between multiple microtubule-generating pathways confers robustness to centrosome-driven mitotic spindle formation. Dev Cell 2014; 28:81-93; PMID:24389063; http://dx.doi.org/10.1016/j.devcel.2013.12.001
- Sanchez-Huertas C, Luders J. The augmin connection in the geometry of microtubule networks. Curr Biol 2015; 25:R294-9; PMID:25829017; http://dx.doi.org/10.1016/j.cub.2015.02.006
- Meireles AM, Fisher KH, Colombie N, Wakefield JG, Ohkura H. Wac: a new Augmin subunit required for chromosome alignment but not for acentrosomal microtubule assembly in female meiosis. J Cell Biol 2009; 184:777-84; PMID:19289792; http://dx.doi.org/10.1083/jcb.200811102
- Moore AW. Intrinsic mechanisms to define neuron class-specific dendrite arbor morphology. Cell Adh Migr 2008; 2:81-2; PMID:19262120; http://dx.doi.org/10.4161/cam.2.2.6395
- Santiago C, Bashaw GJ. Transcription factors and effectors that regulate neuronal morphology. Development 2014; 141:4667-80; PMID:25468936; http://dx.doi.org/10.1242/dev.110817
- Hand R, Bortone D, Mattar P, Nguyen L, Heng JI, Guerrier S, Boutt E, Peters E, Barnes AP, Parras C, et al. Phosphorylation of Neurogenin2 specifies the migration properties and the dendritic morphology of pyramidal neurons in the neocortex. Neuron 2005; 48:45-62; PMID:16202708; http://dx.doi.org/10.1016/j.neuron.2005.08.032
- Cubelos B, Briz CG, Esteban-Ortega GM, Nieto M. Cux1 and Cux2 selectively target basal and apical dendritic compartments of layer II-III cortical neurons. Dev Neurobiol 2015; 75:163-72; PMID:25059644; http://dx.doi.org/10.1002/dneu.22215
- Matsui A, Tran M, Yoshida AC, Kikuchi SS, U M, Ogawa M, Shimogori T. BTBD3 controls dendrite orientation toward active axons in mammalian neocortex. Science 2013; 342:1114-8; PMID:24179155; http://dx.doi.org/10.1126/science.1244505
- Kim MD, Jan LY, Jan YN. The bHLH-PAS protein Spineless is necessary for the diversification of dendrite morphology of Drosophila dendritic arborization neurons. Genes Dev 2006; 20:2806-19; PMID:17015425; http://dx.doi.org/10.1101/gad.1459706
- Karim MR, Moore AW. Convergent local identity and topographic projection of sensory neurons. J Neurosci 2011; 31:17017-27; PMID:22114271; http://dx.doi.org/10.1523/JNEUROSCI.2815-11.2011
- Crozatier M, Vincent A. Control of multidendritic neuron differentiation in Drosophila: the role of Collier. Dev Biol 2008; 315:232-42; PMID:18234173; http://dx.doi.org/10.1016/j.ydbio.2007.12.030
- Moore AW, Jan LY, Jan YN. hamlet, a binary genetic switch between single- and multiple- dendrite neuron morphology. Science 2002; 297:1355-8; PMID:12193790; http://dx.doi.org/10.1126/science.1072387
- Hattori Y, Sugimura K, Uemura T. Selective expression of Knot/Collier, a transcriptional regulator of the EBF/Olf-1 family, endows the Drosophila sensory system with neuronal class-specific elaborated dendritic patterns. Genes Cells 2007; 12:1011-22; PMID:17825045; http://dx.doi.org/10.1111/j.1365-2443.2007.01107.x
- Grueber WB, Jan LY, Jan YN. Different levels of the homeodomain protein cut regulate distinct dendrite branching patterns of Drosophila multidendritic neurons. Cell 2003; 112:805-18; PMID:12654247; http://dx.doi.org/10.1016/S0092-8674(03)00160-0
- Sugimura K, Satoh D, Estes P, Crews S, Uemura T. Development of morphological diversity of dendrites in Drosophila by the BTB-zinc finger protein abrupt. Neuron 2004; 43:809-22; PMID:15363392; http://dx.doi.org/10.1016/j.neuron.2004.08.016
- Li W, Wang F, Menut L, Gao FB. BTB/POZ-zinc finger protein abrupt suppresses dendritic branching in a neuronal subtype-specific and dosage-dependent manner. Neuron 2004; 43:823-34; PMID:15363393; http://dx.doi.org/10.1016/j.neuron.2004.08.040
- Ferreira T, Ou Y, Li S, Giniger E, van Meyel DJ. Dendrite architecture organized by transcriptional control of the F-actin nucleator Spire. Development 2014; 141:650-60; PMID:24449841; http://dx.doi.org/10.1242/dev.099655
- Ye B, Kim JH, Yang L, McLachlan I, Younger S, Jan LY, Jan YN. Differential regulation of dendritic and axonal development by the novel Kruppel-like factor Dar1. J Neurosci 2011; 31:3309-19; PMID:21368042; http://dx.doi.org/10.1523/JNEUROSCI.6307-10.2011
- Nagel J, Delandre C, Zhang Y, Forstner F, Moore AW, Tavosanis G. Fascin controls neuronal class-specific dendrite arbor morphology. Development 2012; 139:2999-3009; PMID:22764047; http://dx.doi.org/10.1242/dev.077800
- Iyer SC, Ramachandran Iyer EP, Meduri R, Rubaharan M, Kuntimaddi A, Karamsetty M, Cox DN. Cut, via CrebA, transcriptionally regulates the COPII secretory pathway to direct dendrite development in Drosophila. J Cell Sci 2013; 126:4732-45; PMID:23902691; http://dx.doi.org/10.1242/jcs.131144
- Karim MR, Moore AW. Morphological analysis of Drosophila larval peripheral sensory neuron dendrites and axons using genetic mosaics. J Vis Exp 2011; 57:e3111; PMID:22158135.
- Masahira N, Ding L, Takebayashi H, Shimizu K, Ikenaka K, Ono K. Improved preservation of X-gal reaction product for electron microscopy using hydroxypropyl methacrylate. Neurosci Lett 2005; 374:17-20; PMID:15631888; http://dx.doi.org/10.1016/j.neulet.2004.10.023
- Han C, Wang D, Soba P, Zhu S, Lin X, Jan LY, Jan YN. Integrins regulate repulsion-mediated dendritic patterning of drosophila sensory neurons by restricting dendrites in a 2D space. Neuron 2012; 73:64-78; PMID:22243747; http://dx.doi.org/10.1016/j.neuron.2011.10.036
- Kim ME, Shrestha BR, Blazeski R, Mason CA, Grueber WB. Integrins establish dendrite-substrate relationships that promote dendritic self-avoidance and patterning in drosophila sensory neurons. Neuron 2012; 73:79-91; PMID:22243748; http://dx.doi.org/10.1016/j.neuron.2011.10.033
- Krieg M, Dunn AR, Goodman MB. Mechanical systems biology of C. elegans touch sensation. Bioessays 2015; 37:335-44; PMID:25597279; http://dx.doi.org/10.1002/bies.201400154
- Dettman RW, Turner FR, Hoyle HD, Raff EC. Embryonic expression of the divergent Drosophila beta3-tubulin isoform is required for larval behavior. Genetics 2001; 158:253-63; PMID:11333234
- Mennella V, Keszthelyi B, McDonald KL, Chhun B, Kan F, Rogers GC, Huang B, Agard DA. Subdiffraction-resolution fluorescence microscopy reveals a domain of the centrosome critical for pericentriolar material organization. Nat Cell Biol 2012; 14:1159-68; PMID:23086239; http://dx.doi.org/10.1038/ncb2597
- Hsia KC, Wilson-Kubalek EM, Dottore A, Hao Q, Tsai KL, Forth S, Shimamoto Y, Milligan RA, Kapoor TM. Reconstitution of the augmin complex provides insights into its architecture and function. Nat Cell Biol 2014; 16:852-63; PMID:25173975; http://dx.doi.org/10.1038/ncb3030
- Merriam EB, Millette M, Lumbard DC, Saengsawang W, Fothergill T, Hu X, Ferhat L, Dent EW. Synaptic regulation of microtubule dynamics in dendritic spines by calcium, F-actin, and drebrin. J Neurosci 2013; 33:16471-82; PMID:24133252; http://dx.doi.org/10.1523/JNEUROSCI.0661-13.2013