ABSTRACT
Autophagy plays important roles in self-renewal and differentiation of stem cells. Hepatic progenitor cells (HPCs) are thought to have the ability of self-renewal as well as possess a bipotential capacity, which allows them to differentiate into both hepatocytes and bile ductular cells. However, how autophagy contributes to self-renewal and differentiation of hepatic progenitor cells is not well understood. In this study, we use a well-established rat hepatic progenitor cell lines called WB-F344, which is treated with 3.75 mM sodium butyrate (SB) to promote the differentiation of WB-F344 along the biliary phenotype. We found that autophagy was decreased in the early stage of biliary differentiation, and maintained a low level at the late stage. Activation of autophagy by rapamycin or starvation suppressed the biliary differentiation of WB-F344. Further study reported that autophagy inhibited Notch1 signaling pathway, which contributed to biliary differentiation and morphogenesis. In conclusions, autophagy regulates biliary differentiation of hepatic progenitor cells through Notch1 signaling pathway.
Introduction
Macroautophagy (hereafter referred to as autophagy), characterized as an important process of cellular homeostasis, takes place in all eukaryotic cells and involves the sequestration of cytoplasmic components in double membrane autophagosomes, which is a basic mechanism involving cell degradation of unnecessary or dysfunctional cellular components by lysosomes.Citation1,2 Autophagy takes part in physiological and pathological processes including cancer, metabolic disease, neurodegenerative disorders, cell growth, cell death and ontogeny.Citation3 Recent studies have demonstrated autophagy plays important roles in self-renewal and differentiation of stem cells.Citation4-7 However, how autophagy contributes to self-renewal and differentiation of hepatic progenitor cells is not well understood.
Hepatic progenitor cells (HPCs) are thought to have the ability of self-renewal as well as possess a bipotential capacity, which allows them to differentiate into both hepatocytes and bile ductular cells.Citation8,9 Its differential process is controlled by many signaling pathways, such as Notch, Wnt, BMP, TGF-B, HGF and FGF signaling pathways.Citation10,11
Notch signaling pathway is a highly evolutionarily conserved pathway. During ontogeny, Notch signaling pathway controls liver development by regulating biliary differentiation.Citation12-16 Notch signaling pathway is required for normal bile duct development, and Notch pathway ablation results in bile duct paucity, a characteristic of Alagilles syndrome.Citation17-19 In mammals, 2 Jagged proteins (Jagged1 and Jagged2) and 3 Deltas (Dll1, Dll3 and Dll4) have been identified as Notch ligands. Four Notch receptors(Notch1-4) interacted with Notch ligands belong to the Delta-Serrate-Lag-2 (DSL) family.Citation20,21 The Notch signaling pathway is initiated by the interaction between the receptors and ligands, which triggers a metalloprotease cleavage of the Notch receptor followed by its intramembrane proteolysis by the γ-secretase complex.Citation22 The Notch intracellular domain (NICD) then translocates to the nucleus where it forms a NICD/RBPJk complex.Citation23 The heteromeric transcriptional complex induces protein targets such as Hes and Hey family.Citation24
Autophagy is reported to be related with Notch signaling pathway. In Drosophila oogenesis, the loss of autophagy leads to the activation of the Notch signaling pathway.Citation25 In cardiac differentiation, Autophagy eliminates cytoplasmic NICD to promote cardiac differentiation.Citation26 However, whether autophagy regulates Notch signaling pathways in biliary differentiation is not well understood. Here we report that autophagy regulates biliary differentiation of hepatic progenitor cells through Notch1 signaling pathway.
Results
Autophagy is decreased in the early stage of biliary differentiation of WB-F344 cells and maintains a low level at the late stage
To determine whether autophagy is involved in biliary differentiation of WB-F344 cells, we examine the morphology and expression of biliary lineage markers of WB-F344 cells cultured with 3.75 mM Sodium butyrate (SB). WB-F344 cells are small and polygonal and have a high nuclear/cytoplasmic ratio when cultured in normal media. However, when WB-F344 cells are treated with SB for 5 days, the sizes of them are larger than control (). In addition, the nuclear/cytoplasmic ratio is also reduced (). We also analyze the early biliary transcription factor Hnf1b and the mature biliary lineage makers Hnf6, Krt19 and γ-GT, which are notably increased when treated with SB for 5 d ().Moreover, under Sodium butyrate induced differentiation conditions, proliferation is decreased relative to control (). These results show that WB-F344 cells are treated with 3.75 mM SB to promote the differentiation along the biliary phenotype. At the same time, we detect the level of LC3-II/LC3-I and P62 during the whole stage of differentiation. We observe that the LC3-II/LC3-I level is high in WB-F344 cells; when treated with SB, LC3-II/LC3-I level is rapidly decreased in the first 3 days, and maintains a low level until the fifth day().On the contrary, P62 level (an indicator of autophagy) is increased(). We quantify normalized LC3-II/LC3-I that is also decreased ().In order to observe the formation of autophagic vacuoles intuitively, the electron microscopy is employed. Without SB induction, the autophagic vacuoles in WB-F344 cells are easy to detect. On the contrary, the autophagic vacuoles are decreased in WB-F344 cells treated with SB for 5 d (). These findings indicate that autophagy is decreased in the early stage of biliary differentiation of WB-F344 cells and maintains a low level at the late stage.
Figure 1. Autophagy is decreased in the early stage of biliary differentiation of WB-F344 cells and maintains a low level at the late stage. (A) The morphology of WB-F344 cells cultured in normal media (Left panel, Ctrl, Control) or treated with 3.75 mM Sodium butyrate (SB) for 5 day (Right panel, SB, Sodium butyrate); (Scale bars = 20 μm, Inset: 2X magnification). (B) qRT-PCR analyzing the expression of biliary lineage markers, the early biliary transcription factor (Hnf1b) and the mature biliary lineage makers (Hnf6, Krt19 and γ-GT) in WB-F344 cells treated with SB for 5 d. (C) The proliferation of WB-F344 cells cultured in normal media (Ctrl, red) or treated with 3.75 mM Sodium butyrate (SB) for 5 day (SB, Green).(D)Western blot showing autophagy in SB-induced WB-F344 cells. The level of LC3-II/LC3-I and P62 at the indicated time-points are determined and Actin is used as an internal control. (E) Densitometric analysis of LC3-II relative to LC3-I normalized to time 0d (0d vs 1d-5d).(F) Autophagic vacuoles (arrowheads) were observed at WB-F344 cells cultured in normal media(Ctrl) or treated with 3.75 mM Sodium butyrate for 5 day (SB) under an electron microscope (Scale bars = 1 μm). WB-F344 cells without treatment of 3.75 mM Sodium butyrate (SB) are used as control. Data represent mean ± SEM *P < 0.05; **P < 0.01. n = 3.
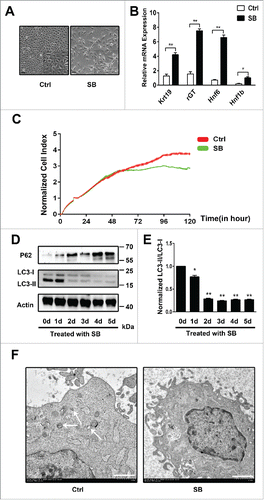
Activation of autophagy suppresses the biliary differentiation of WB-F344 cells
To investigate whether induction of autophagy has an effect on the biliary differentiation of WB-F344 cells, we examine the effect of 2 autophagy stimuli, the mTOR inhibitor rapamycin and starvation, on the biliary differentiation of WB-F344 cells.WB-F344 cells are treated with SB for 4 days, and co-culture DMSO, rapamycin or starvation at the fifth day. Western blot analysis indicates that LC3-II/LC3-I level is increased and P62 level is decreased by rapamycin () or starvation (). Rapamycin decreases the mRNA expression of Hnf1b, Hnf6, Krt19 and γ-GT relative to DMSO (). Starvation also attenuates the mRNA expression of Hnf1b, Hnf6, Krt19 and γ-GT relative to DMSO (). These results indicate that Activation of autophagy suppresses the biliary differentiation of WB-F344 cells.
Figure 2. Activation of autophagy suppresses the biliary differentiation of WB-F344 cells. (A) Western blot showing the level of LC3-II/LC3-I and P62 in WB-F344 cells treated with 3.75 mM SB for 4 d and co-culture DMSO, or rapamycin (Sigma, 200 nM; Rapa) for 24h. (B) qRT-PCR analyzing the expression of biliary lineage markers (Hnf1b, Hnf6, Krt19 and γ-GT) in WB-F344 cells treated with 3.75 mM SB for 4 d and co-culture DMSO, or rapamycin (200 nM; Rapa) for 24h. (C) Western blot showing the level of LC3-II/LC3-I and P62 in WB-F344 cells treated with 3.75 mM SB for 4 d and co-culture DMSO, or starvation (serum-free medium; Starve) for 24h. (D) qRT-PCR analyzing the expression of biliary lineage markers (Hnf1b, Hnf6, Krt19 and γ-GT) in WB-F344 treated with 3.75 mM SB for 4 d and co-culture DMSO, or starvation (serum-free medium; Starve) for 24h. Data represent mean ± SEM *P < 0.05, **P < 0.01. n = 3.
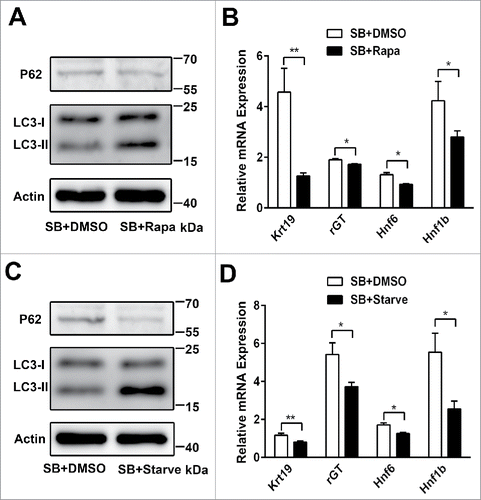
Notch1 signaling pathway is up-regulated in the biliary differentiation of WB-F344 cells
We have demonstrated that activation of autophagy suppresses the biliary differentiation of WB-F344 cells. Next, we explore how autophagy influences the biliary differentiation. We firstly investigate whether Notch signaling pathway takes part in the biliary differentiation of WB-F344 cells. After exposing to SB for 5 days, the mRNA and protein expression of Jagged1, Notch1 are elevated(); Notch1 protein level is enhanced by immunofluorescence().The mRNA expression of Hes1, Hes5, Hey1 and Heyl, the target genes of the Notch1 signaling pathway, are increased during the biliary differentiation of WB-F344 cells(). Western blot assay also indicate that the expression of Hes1 is increased (). These findings show that Notch1 signaling pathway is upregulated in the biliary differentiation of WB-F344 cells.
Figure 3. Notch1 signaling pathway is up-regulated in the biliary differentiation of WB-F344 cells. (A) Western blot showing the level of Jagged1, Notch1 and Hes1 in WB-F344 cells treated with 3.75 mM SB for 5 d. (B) Immunofluorescence staining Notch1(red; arrow) and DAPI(blue; DNA) in WB-F344 cells treated with 3.75 mM SB for 5 days(Scale bars = 20 μm).(C)qRT-PCR analyzing the mRNA expression of Jagged1, Notch1 in WB-F344 cells treated with 3.75 mM SB for 5 d. (D) qRT-PCR analyzing the expression of the target genes of the Notch signaling pathway (Hes1, Hes5, Hey1 and Heyl) in WB-F344 cells treated with 3.75 mM SB for 5 d. WB-F344 cells without treatment of 3.75 mM Sodium butyrate (SB) are used as control. Data represent mean ± SEM *P < 0.05, **P < 0.01. n = 3.
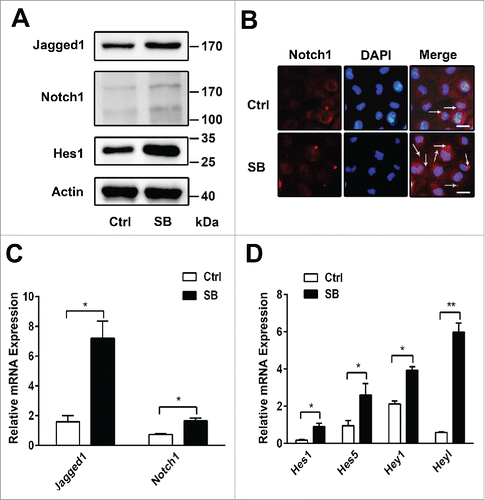
Autophagy inhibits Notch1 signaling pathway during the biliary differentiation of WB-F344 cells
It has been reported that autophagy can interfere Notch signaling pathway in Drosophila oogenesis and cardiac differentiation.Citation25,26 Therefore, we investigate whether Notch1 signaling pathway can be regulated by autophagy during the biliary differentiation of WB-F344 cells. We also examine the effect of 2 autophagy stimuli, the mTOR inhibitor rapamycin and nutrient deprivation medium, on the biliary differentiation of WB-F344 cells. Activation of autophagy by rapamycin, the mRNA and protein expression of Hes1, Hes5, Hey1 and Heyl are decreased () relative to DMSO. Similarly, starvation also attenuates the mRNA and protein expression of Hes1, Hes5, Hey1 and Heyl () relative to DMSO. These data demonstrate that the Notch1 signaling pathway is inhibited by autophagy during the biliary differentiation of WB-F344 cells.
Figure 4. Autophagy inhibits Notch1 signaling pathway during the biliary differentiation of WB-F344 cells. (A) qRT-PCR analyzing the expression of the target genes of the Notch signaling pathway(Hes1, Hes5,Hey1 and Heyl) in WB-F344 cells treated with 3.75 mM SB for 4 d and co-culture DMSO, or rapamycin( 200 nM,Rapa) for 24h. (B) Western blot showing the level of Hes1, Hes5, Hey1 and Heyl in WB-F344 cells treated with 3.75 mM SB for 4 d and co-culture DMSO, or rapamycin( 200 nM,Rapa) for 24 h. (C)qRT-PCR analyzing the expression of the target genes of the Notch signaling pathway(Hes1, Hes5,Hey1 and Heyl) in WB-F344 cells treated with 3.75 mM SB for 4 d and co-culture DMSO, or starvation (serum-free medium, Starve) for 24h. (D) Western blot showing the level of Hes1, Hes5, Hey1 and Heyl in WB-F344 cells treated with 3.75 mM SB for 4 d and co-culture DMSO, or starvation (serum-free medium, Starve) for 24h. Data represent mean ± SEM *P < 0.05, **P < 0.01, n = 3.
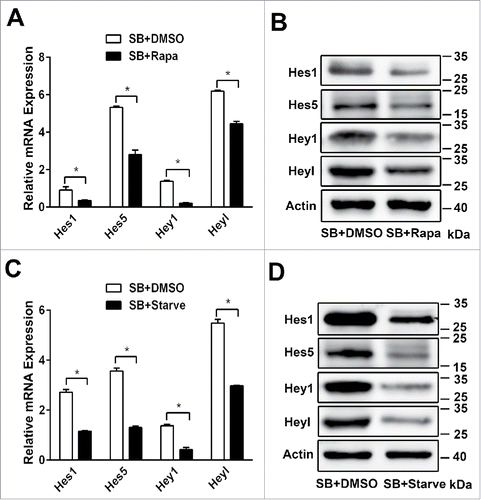
To further explore whether Notch1 signaling pathway is able to regulate autophagy, we co-culture either DMSO or DAPT (a γ- secretase inhibitor) in differentiation medium. WB-F344 cells are treated with SB for 4 days, and co-culture DMSO, DAPT at the fifth day. Inhibition of Notch1 signaling pathway by DAPT results in the lower mRNA expression of Hes1, Hes5, Hey1 and Heyl () and the lower protein level of Hes1, Hes5, Hey1 and Heyl (). The lower Hes and Hey mRNA expression are associated with the lower mRNA expression of Hnf1b, Hnf6, Krt19 and γ-GT (), but there is no effect on LC3-II/LC3-I and P62 levels () when Notch1 signaling pathway is Inhibited. These results indicate that autophagy is not able to be influenced by Notch1 signaling pathway during the biliary differentiation of WB-F344 cells.
Figure 5. Autophagy is not able to be influenced by Notch1 signaling pathway during the biliary differentiation of WB-F344 cells. (A) qRT-PCR analyzing the expression of the target genes of the Notch1 signaling pathway (Hes1, Hes5, Hey1 and Heyl) in WB-F344 cells treated with 3.75 mM SB for 4 d and co-culture DMSO, or DAPT (Sigma, 25 μM) for 24h. (B)Western blot showing the level of Hes1, Hes5, Hey1 and Heyl in WB-F344 cells treated with 3.75 mM SB for 4 d and co-culture DMSO, or DAPT (25 μM) for 24h. (C) qRT-PCR analyzing the expression of biliary lineage markers (Hnf1b, Hnf6, Krt19 and γ-GT) in WB-F344 cells treated with 3.75 mM SB for 4 d and co-culture DMSO, or DAPT (25μM) for 24h. (D) Western blot showing the level of LC3-II/LC3-I and P62 in WB-F344 cells treated with 3.75 mM SB for 4 d and co-culture DMSO, or DAPT (25μM) for 24h. Data represent mean ± SEM *P < 0.05, **P < 0.01. n = 3.
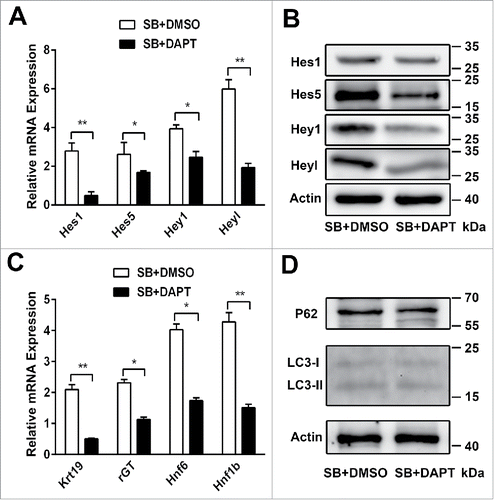
Discussion
In this study, we demonstrate that autophagy regulates biliary differentiation of hepatic progenitor cells through Notch1 signaling pathway (). Autophagy is decreased in the early stage of biliary differentiation of WB-F344 cells and maintains a low level at the late stage. Activation of autophagy by rapamycin or starvation suppresses the biliary differentiation of WB-F344 cells. Autophagy plays important roles in self-renewal and differentiation of stem cells.Citation4-7 In haematopoietic stem cells (HSCs), mesenchymal stem cells (MSCs), dermal stem cells (DSCs), epidermal stem cells (Epi-S) and adipocyte, basal autophagy level is higher.Citation27-33 After induced differentiation, autophagic level is decreased. On the contrary, autophagy is increased during their differentiation in neural stem cells (NSCs), cardiac stem cells (CSCs), embryonic stem cells (ESCs).Citation34-39 It has been reported that autophagy plays a critical role in regulating stemness of hepatic progenitor cells(HPCs).Citation40 High autophagic activity is observed in most of stem cells relative to differentiation cells, which is crucial for the maintenance of cellular homeostasis and stemness. In the future, we will explore that the role and the mechanism of autophagy in hepatic differentiation of HPCs.
Figure 6. (A) Model for how autophagy regulates Notch1 signaling pathway in biliary differentiation of hepatic progenitor cells. (B) Schematic illustration of how the mTOR-Notch pathway regulates cell differentiation through the mTOR-STAT3-Notch cascade and the mTOR-autophagy-Notch cascade.
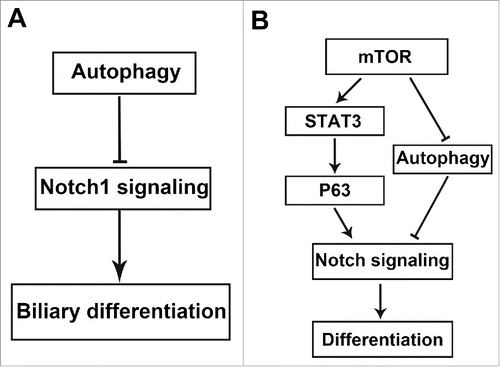
Our results also confirm that Notch1 signaling pathway is up-regulated in the biliary differentiation of WB-F344 cells, inhibition of Notch1 signaling pathway suppresses biliary differentiation. These results support past studies that Notch1 signaling pathway controls liver development by regulating biliary differentiation.Citation12-16
Further studies suggest that activation of autophagy by rapamycin or starvation attenuates Notch1 signaling pathway. These results demonstrate that autophagy inhibits Notch1 signaling pathway, which contributes to biliary differentiation and morphogenesis. These results are consistent with previous reports showing the loss of autophagy leads to the activation of the Notch signaling in Drosophila oogenesisCitation25 and autophagy eliminates cytoplasmic NICD to promote cardiac differentiation.Citation26 Autophagy is activated by mammalian target of rapamycin protein kinase pathway (mTOR).Citation41 mTOR is a center of autophagy regulation molecule, which is inhibited autophagy associated gene(ATG) to regulate autophagy. Autophagy is initiated by the inhibition of mTOR.Citation42 Autophagy is a novel activator of mTORC2 signaling, leading to CTGF induction and myofibroblast differentiation.Citation43 mTOR-STAT3-P63-Jagged-Notch signaling pathway plays an important role in regulating cell differentiation.Citation44 We find mTOR signaling is increased in biliary differentiation (data not shown).These findings suggest that mTOR-autophagy-Notch signaling pathway is important for cell differentiation ().
Furthermore, autophagy may exert a multifactorial influence on the initiation and progression of cancer.Citation45 Notch signaling pathway is also considered to have a dual role in tumor development.Citation46 There are a growing number of evidences show that abnormal regulation of signaling pathway results in aberrant differentiation, which most likely lead to liver cancer stem cells that give rise to hepatocellular carcinoma as well as cholangiocarcinoma.Citation10,11,47,48 These findings indicate autophagy and Notch signaling pathway plays crucial roles in the initiation and progression of cancer. Further studies are needed to fully explain the mechanisms how autophagy regulates Notch signaling under physiological and pathological conditions.
Conclusion
The present results describe a direct link between autophagy and Notch1 signaling pathway, both of them regulate biliary differentiation. Firstly, autophagy is decreased in the early stage of biliary differentiation of WB-F344 cells and maintains a low level at the late stage. Activation of autophagy by rapamycin or starvation suppresses the biliary differentiation of WB-F344 cells. Secondly, Notch1 signaling pathway is upregulated in the biliary differentiation of WB-F344, inhibition of Notch signaling pathway suppresses biliary differentiation. At last, activation of autophagy by rapamycin or starvation attenuates Notch1 signaling pathway. These results demonstrate that autophagy regulates biliary differentiation of hepatic progenitor cells through Notch1 signaling pathway.
Materials and methods
Cell culture and treatments
WB-F344 cells were cultured in Dulbecco's Modified Eagle Medium (DMEM, Gibco-BRL, Gaithersburg, MD, USA) containing 10% FBS and antibiotics (100 mg/L penicillin and 100mg/L streptomycin) in a humidified incubator under 5% CO2 at 37°C.
WB-F344 cells had been used as a good model to study the mechanism of biliary differentiation. To induce biliary differentiation, WB-F344 cells were treated with 3.75 mM sodium butyrate (Sigma-Aldrich).Citation49,50 The medium was changed every 2 d. The days of differentiation were numbered consecutively beginning at the first day of the SB treatment (0d) to the last day (5d).
Real-time quantitative PCR
Total RNA was isolated using TRIZOL (Invitrogen) and cDNA synthesis was performed using the Prime Script RT reagent Kit (Takara) according to the manufacturer's instructions. The primers are listed in . Quantitative PCR was performed using SYBR Green PCR Kit (Applied BI) according to the manufacturer's instructions. The relative quantities of mRNAs were obtained by using the comparative Ct method and were normalized with glyceraldehydes-3-phosphate dehydrogenase (GAPDH). The primers were presented in .
Table 1. Primers used for quantitative real-time RT-PCR.
Western blotting analysis
Protein samples were collected directly by cell extraction buffer (Beyotime, P0013) containing a protease inhibitors PMSF. Proteins were separated by electrophoresis on 10–12%SDS-polyacrylamide gels, transferred to Polyvinylidene Fluoride membrane, and incubated with corresponding primary antibodies overnight at 4°C. Following incubated with secondary antibody 1h at room temperature. The immunoreactive proteins were detected by enhanced Chemoluminescence Substrate (Millipore), and the blot was scanned and densitometric analysis with Image J software. The primary antibodies used in our experiment including: P62 (CST, #5114, diluted 1:1000), LC3A/B (CST, #4108, diluted 1:1000),Notch1(CST, #3608, diluted 1:1000),Hes1(CST, #11988, diluted 1:1000),Hes5(abcam, ab194111, diluted 1:1000),Hey1(Sigma, SAB2106265, diluted 1:1000),Heyl(Sigma, AV31866, diluted 1:1000),Jagged1(Novus, NBP-95754, diluted 1:1000) and Actin (Bioworld, AP0060, diluted 1:5000).
Immunofluorescence staining
After treatment, cells in 24 well plate were fixed with 4% paraformaldehyde and incubated with Notch1(CST, #3608, diluted 1:200)overnight at 4°C. And then incubated with Alexa Fluor 488-labeled secondary antibodies (Molecular Probes, Invitrogen, Paisley, UK, diluted1:200) for 1h at 37°C. DAPI (Sigma-Aldrich) was used to stain the nuclei. Fluorescence intensity was evaluated by using a confocal microscope (Leica TCS SP2).
Cell proliferation assay
The measurement of cell proliferation was performed by E-Plate 16. Fisrtly, 100 µl culture medium was added to each well and established background line. After 1h, Cells were seeded in each well (5000 cells per well) and started to measured dynamic of cell proliferation.
Statistical analysis
Analysis of variance was performed by GraphPad Prism 6.0 (GraphPad Software). Quantitative data were expressed as mean ± S.E.M for each experiment. Significance between the experimental groups and control groups were performed by Student's test. P < 0.05 was considered statistically significant.
Abbreviations
HPCs | = | Hepatic progenitor cells |
WB-F344 cells | = | rat hepatic progenitor cell lines |
γ-GT | = | γ-glutamyltranspeptidase |
SB | = | sodium butyrate |
NICD | = | Notch intracellular domain |
Rapa | = | rapamycin |
Starve | = | starvation |
DAPT | = | N-[N-(3,5-difluorophenacetyl)–l–alanyl]–S-phenylglycinet-butylester |
HSCs | = | haematopoietic stem cells |
MSCs | = | mesenchymal stem cells |
DSCs | = | dermal stem cells |
Epi-S | = | epidermal stem cells |
NSCs | = | neural stem cells |
CSCs | = | cardiac stem cells |
ESCs | = | embryonic stem cells |
mTOR | = | the mammalian target of rapamycin |
ATG | = | autophagy associated gene |
Disclosure of potential conflicts of interest
No potential conflicts of interest were disclosed.
Funding
This project was supported by the Key Basic Research Project of China (Grant NO. 2012CBA01303); National Natural Science Foundation of China (Grant No. 81372312, 81472737, 81402018, 81402020, 81401308, 81402026, 81372330, 81572444, 81502417, 81502543); Special Funds for National Key Sci-Tech Special Project of China (Grant No. 2012ZX10002-016, 2012ZX10002011-011); Shanghai Science and Technology Committee (Grant No.14ZD1900403,14ZR1409200, 15PJ1410600); Shanghai Municipal Education Commission (Grant No. 14ZZ086).
References
- Levine B, Kroemer G. Autophagy in the Pathogenesis of Disease. Cell 2008; 132:27-42; PMID:18191218; http://dx.doi.org/10.1016/j.cell.2007.12.018
- Mizushima N, Levine B, Cuervo AM, Klionsky DJ. Autophagy fights disease through cellular self-digestion. Nature 2008; 451:1069-75; PMID:18305538; http://dx.doi.org/10.1038/nature06639
- Choi AMK, Ryter SW, Levine B. Autophagy in Human Health and Disease. N Engl J Med 2013; 368:651-62; PMID:23406030; http://dx.doi.org/10.1056/NEJMra1205406
- Pan H, Cai N, Li M, Liu GH, Izpisua Belmonte JC. Autophagic control of cell ‘stemness’. EMBO Mol Med 2013; 5:327-31; PMID:23495139; http://dx.doi.org/10.1002/emmm.201201999
- Guan JL, Simon AK, Prescott M, Menendez JA, Liu F, Wang F, Wang C, Wolvetang E, Vazquez-Martin A, Zhang J. Autophagy in stem cells. Autophagy 2013; 9:830-49; PMID:23486312; http://dx.doi.org/10.4161/auto.24132
- Mizushima N, Levine B. Autophagy in mammalian development and differentiation. Nat Cell Biol 2010; 12:823-30; PMID:20811354; http://dx.doi.org/10.1038/ncb0910-823
- Phadwal K, Watson AS, Simon AK. Tightrope act: autophagy in stem cell renewal, differentiation, proliferation, and aging. Cell Mol Life Sci 2013; 70:89-103; PMID:22669258; http://dx.doi.org/10.1007/s00018-012-1032-3
- Miyajima A, Tanaka M, Itoh T. Stem/progenitor cells in liver development, homeostasis, regeneration, and reprogramming. Cell Stem Cell 2014; 14:561-74; PMID:24792114; http://dx.doi.org/10.1016/j.stem.2014.04.010
- De Alwis N, Hudson G, Burt AD, Day CP, Chinnery PF. Human liver stem cells originate from the canals of hering. Hepatology 2009; 50:992-3; PMID:19714715; http://dx.doi.org/10.1002/hep.23160
- Yamashita T, Wang XW. Cancer stem cells in the development of liver cancer. J Clin Invest 2013; 123:1911-8; PMID:23635789; http://dx.doi.org/10.1172/JCI66024
- Mishra L, Banker T, Murray J, Byers S, Thenappan A, He AR, Shetty K, Johnson L, Reddy EP. Liver stem cells and hepatocellular carcinoma. Hepatology 2009; 49:318-29; PMID:19111019; http://dx.doi.org/10.1002/hep.22704
- Morell CM, Strazzabosco M. Notch signaling and new therapeutic options in liver disease. J Hepatol . 2014;60(4):885-90.
- Tanimizu N, Miyajima A. Notch signaling controls hepatoblast differentiation by altering the expression of liver-enriched transcription factors. J Cell Sci 2004; 117:3165-74; PMID:15226394; http://dx.doi.org/10.1242/jcs.01169
- Lozier J, McCright B, Gridley T. Notch signaling regulates bile duct morphogenesis in mice. PloS one 2008; 3:e1851; PMID:18365007; http://dx.doi.org/10.1371/journal.pone.0001851
- Zong Y, Panikkar A, Xu J, Antoniou A, Raynaud P, Lemaigre F, Stanger BZ. Notch signaling controls liver development by regulating biliary differentiation. Development 2009; 136:1727-39; PMID:19369401; http://dx.doi.org/10.1242/dev.029140
- Boulter L, Govaere O, Bird TG, Radulescu S, Ramachandran P, Pellicoro A, Ridgway RA, Seo SS, Spee B, Van Rooijen N, et al. Macrophage-derived Wnt opposes Notch signaling to specify hepatic progenitor cell fate in chronic liver disease. Nat Med 2012; 18:572-9; PMID:22388089; http://dx.doi.org/10.1038/nm.2667
- McCright B, Lozier J, Gridley T. A mouse model of Alagille syndrome: Notch2 as a genetic modifier of Jag1 haploinsufficiency. Development 2002; 129:1075-82; PMID:11861489
- Li L. Alagille syndrome is caused by mutations in human Jagged1, which encodes a ligand for Notch1. Nat Genet 1997; 16:243-51; PMID:9207788; http://dx.doi.org/10.1038/ng0797-243
- McDaniell R. NOTCH2 mutations cause Alagille syndrome, a heterogeneous disorder of the notch signaling pathway. Am J Hum Genet 2006; 79:169-73; PMID:16773578; http://dx.doi.org/10.1086/505332
- Bray SJ. Notch signalling: a simple pathway becomes complex. Nat Rev Mol Cell Biol 2006; 7:678-89; PMID:16921404; http://dx.doi.org/10.1038/nrm2009
- Kopan R, Ilagan MXG. The canonical notch signaling pathway: unfolding the activation mechanism. Cell 2009; 137:216-33; PMID:19379690; http://dx.doi.org/10.1016/j.cell.2009.03.045
- Schweisguth F. Regulation of notch signaling activity. Curr Biol 2004; 14:R129-R38; PMID:14986688; http://dx.doi.org/10.1016/j.cub.2004.01.023
- Fortini ME. Notch signaling: the core pathway and its posttranslational regulation. Dev Cell 2009; 16:633-47; PMID:19460341; http://dx.doi.org/10.1016/j.devcel.2009.03.010
- Iso T, Kedes L, Hamamori Y. HES and HERP families: multiple effectors of the Notch signaling pathway. J Cell Physiol 2003; 194:237-55; PMID:12548545; http://dx.doi.org/10.1002/jcp.10208
- Barth JMI, Hafen E, Köhler K. The lack of autophagy triggers precocious activation of Notch signaling during Drosophila oogenesis. BMC Dev Biol 2012; 12:35; PMID:23217079; http://dx.doi.org/10.1186/1471-213X-12-35
- Jia Z, Wang J, Wang W, Tian Y, XiangWei W, Chen P, Ma K, Zhou C. Autophagy eliminates cytoplasmic β-catenin and NICD to promote the cardiac differentiation of P19CL6 cells. Cell Signal 2014; 26:2299-305; PMID:25101857; http://dx.doi.org/10.1016/j.cellsig.2014.07.028
- Liu F, Lee JY, Wei H, Tanabe O, Engel JD, Morrison SJ, Guan JL. FIP200 is required for the cell-autonomous maintenance of fetal hematopoietic stem cells. Blood 2010; 116:4806-14; PMID:20716775; http://dx.doi.org/10.1182/blood-2010-06-288589
- Mortensen M, Soilleux EJ, Djordjevic G, Tripp R, Lutteropp M, Sadighi-Akha E, Stranks AJ, Glanville J, Knight S, Jacobsen SE, et al. The autophagy protein Atg7 is essential for hematopoietic stem cell maintenance. J Exp Med 2011; 208:455-67; PMID:21339326; http://dx.doi.org/10.1084/jem.20101145
- Warr MR. FOXO3A directs a protective autophagy program in haematopoietic stem cells. Nature 2013; 494:323-7; PMID:23389440; http://dx.doi.org/10.1038/nature11895
- Oliver L, Hue E, Priault M, Vallette FM. Basal autophagy decreased during the differentiation of human adult mesenchymal stem cells. Stem Cells Dev 2012; 21:2779-88; PMID:22519885; http://dx.doi.org/10.1089/scd.2012.0124
- Salemi S, Yousefi S, Constantinescu MA, Fey MF, Simon HU. Autophagy is required for self-renewal and differentiation of adult human stem cells. Cell Res 2012; 22:432-5; PMID:22184008; http://dx.doi.org/10.1038/cr.2011.200
- Singh R, Xiang Y, Wang Y, Baikati K, Cuervo AM, Luu YK, Tang Y, Pessin JE, Schwartz GJ, Czaja MJ. Autophagy regulates adipose mass and differentiation in mice. J Clin Invest 2009; 119:3329-39; PMID:19855132; http://dx.doi.org/10.1172/JCI35541
- Goldman SJ, Zhang Y, Jin S. Autophagic degradation of mitochondria in white adipose tissue differentiation. Antioxid Redox Signal 2011; 14:1971-8; PMID:21126221; http://dx.doi.org/10.1089/ars.2010.3777
- Vázquez P, Arroba AI, Cecconi F, de la Rosa EJ, Boya P, De Pablo F. Atg5 and Ambra1 differentially modulate neurogenesis in neural stem cells. Autophagy 2012; 8:187-99; http://dx.doi.org/10.4161/auto.8.2.18535
- Wang S, Li B, Qiao H, Lv X, Liang Q, Shi Z, Xia W, Ji F, Jiao J. Autophagy-related gene Atg5 is essential for astrocyte differentiation in the developing mouse cortex. EMBO Rep 2014; 15:1053-61; PMID:25227738; http://dx.doi.org/10.15252/embr.201338343
- Wang C, Liang CC, Bian ZC, Zhu Y, Guan JL. FIP200 is required for maintenance and differentiation of postnatal neural stem cells. Nat Neurosci 2013; 16:532-42; PMID:23542691; http://dx.doi.org/10.1038/nn.3365
- Zhang J, Liu J, Huang Y, Chang JY, Liu L, McKeehan WL, Martin JF, Wang F. FRS2alpha-mediated FGF signals suppress premature differentiation of cardiac stem cells through regulating autophagy activity. Circ Res 2012; 110:e29-39; PMID:22207710; http://dx.doi.org/10.1161/CIRCRESAHA.111.255950
- Mizushima N. Dissection of autophagosome formation using Apg5-deficient mouse embryonic stem cells. J Cell Biol 2001; 152:657-67; PMID:11266458; http://dx.doi.org/10.1083/jcb.152.4.657
- Tra T GL, Kao L-P, Li X-L, Grandela C, Devenish RJ, Wolvetang E, Prescott M. Autophagy in Human Embryonic Stem Cells. PLoS One 2011; 6(11): e27485; PMID:22110659; http://dx.doi.org/10.1371/journal.pone.0027485
- Cheng Y, Wang B, Zhou H, Dang S, Jin M, Shi Y, Hao L, Yang Z, Zhang Y. Autophagy is required for the maintenance of liver progenitor cell functionality. Cell Physiol Biochem 2015; 36:1163-74; PMID:26111576; http://dx.doi.org/10.1159/000430287
- Ganley IG, Lam du H, Wang J, Ding X, Chen S, Jiang X. ULK1.ATG13.FIP200 complex mediates mTOR signaling and is essential for autophagy. J Biol Chem 2009; 284:12297-305; PMID:19258318; http://dx.doi.org/10.1074/jbc.M900573200
- Kim J, Kundu M, Viollet B, Guan K-L. AMPK and mTOR regulate autophagy through direct phosphorylation of Ulk1. Nat Cell Biol 2011; 13:132-41; PMID:21258367; http://dx.doi.org/10.1038/ncb2152
- Bernard M, Dieude M, Yang B, Hamelin K, Underwood K, Hebert MJ. Autophagy fosters myofibroblast differentiation through MTORC2 activation and downstream upregulation of CTGF. Autophagy 2014; 10:2193-207; PMID:25495560; http://dx.doi.org/10.4161/15548627.2014.981786
- Ma J, Meng Y, Kwiatkowski DJ, Chen X, Peng H, Sun Q, Zha X, Wang F, Wang Y, Jing Y, et al. Mammalian target of rapamycin regulates murine and human cell differentiation through STAT3/p63/Jagged/Notch cascade. J Clin Invest 2010; 120:103-14; PMID:20038814; http://dx.doi.org/10.1172/JCI37964
- White E, DiPaola RS. The double-edged sword of autophagy modulation in cancer. Clin Cancer Res 2009; 15:5308-16; PMID:19706824; http://dx.doi.org/10.1158/1078-0432.CCR-07-5023
- Yuan X, Wu H, Xu H, Xiong H, Chu Q, Yu S, Wu GS, Wu K. Notch signaling: An emerging therapeutic target for cancer treatment. Cancer Lett 2015; 369:20-7
- Hussain SP, Schwank J, Staib F, Wang XW, Harris CC. TP53 mutations and hepatocellular carcinoma: insights into the etiology and pathogenesis of liver cancer. Oncogene 2007; 26:2166-76; PMID:17401425; http://dx.doi.org/10.1038/sj.onc.1210279
- Saha SK, Parachoniak CA, Ghanta KS, Fitamant J, Ross KN, Najem MS, Gurumurthy S, Akbay EA, Sia D, Cornella H, et al. Mutant IDH inhibits HNF-4alpha to block hepatocyte differentiation and promote biliary cancer. Nature 2014; 513:110-4; PMID:25043045; http://dx.doi.org/10.1038/nature13441
- Couchie D, Holic N, Chobert M-N, Corlu A, Laperche Y. In vitro differentiation of WB-F344 rat liver epithelial cells into the biliary lineage. Differentiation 2002; 69:209-15; PMID:11841479; http://dx.doi.org/10.1046/j.1432-0436.2002.690414.x
- Wang P, Cong M, Liu TH, Yang AT, Cong R, Wu P, Tang SZ, Xu Y, Wang H, Wang BE, et al. Primary isolated hepatic oval cells maintain progenitor cell phenotypes after two-year prolonged cultivation. J Hepatol 2010; 53:863-71; PMID:20739084; http://dx.doi.org/10.1016/j.jhep.2010.05.014