ABSTRACT
Low extracellular pH promotes in melanoma cells a malignant phenotype characterized by an epithelial-to-mesenchymal transition (EMT) program, endowed with mesenchymal markers, high invasiveness and pro-metastatic property. Here, we demonstrate that melanoma cells exposed to an acidic extracellular microenvironment, 6.7±0.1, shift to an oxidative phosphorylation (Oxphos) metabolism. Metformin, a biguanide commonly used for type 2 diabetes, inhibited the most relevant features of acid-induced phenotype, including EMT and Oxphos. When we tested effects of lactic acidosis, to verify whether sodium lactate might have additional effects on acidic melanoma cells, we found that EMT and Oxphos also characterized lactic acid-treated cells. An increased level of motility was the only gained property of lactic acidic-exposed melanoma cells. Metformin treatment inhibited both EMT markers and Oxphos and, when its concentration raised to 10 mM, it induced a striking inhibition of proliferation and colony formation of acidic melanoma cells, both grown in protons enriched medium or lactic acidosis. Thus, our study provides the first evidence that metformin may target either proton or lactic acidosis-exposed melanoma cells inhibiting EMT and Oxphox metabolism. These findings disclose a new potential rationale of metformin addition to advanced melanoma therapy, e.g. targeting acidic cell subpopulation.
Introduction
Proliferating cancer cells exhibit an increased glycolysis regardless of oxygen tension, a phenomenon known as “Warburg effect.”Citation1,2 Aerobic glycolysis is often accompanied by increased glucose uptake, and this phenomenon may be visualized in tumor-bearing patients using 18F-deoxyglucose positron emission tomography (FDG–PET) imaging.Citation3 However, abnormal vasculature can result in regional variation in oxygenation implying a dynamic change in metabolism of tumor cells from an aerobic to an anaerobic type of glycolysis. Hypoxic cells are low proliferating cells and the hypoxia-inducible factor (HIF)-1α transcription factor drives a glycolysis pathway leading to protons and LDH-A-dependent lactate production.Citation4 To avoid intracellular acidosis, up-regulation of monocarboxylate transporter (MCT)-4 carries out of the cells lactate and protons, with the help of sodium-proton exporters and other pH regulating systems.Citation4,5 Thus, tumor microenvironment acidification is not only related to acidosis from Oxphos and ATP hydrolysis but also to lactic acidosis, although it is not yet clear which of the two represents the major driver of reduced pH (ranging from pH 6.7 to 7.1) in certain tumor regions. Acidosis of tumor extracellular compartments represents a new adaptive hallmark of cancer associated with poor prognosis,Citation6,7 resistance to chemotherapy,Citation8,9 and suppression of tumoricidal activity of cytotoxic lymphocytes and natural killer cells.Citation10,11 Decreased extracellular pH (pHe) promotes several characteristics in tumor cells useful to promote cancer progression, it contributes to aggressiveness of tumor cells via stimulation of increased mutation rate,Citation12 invasiveness into host tissues Citation13,14 and promotion of VEGF-A and C release.Citation15,16 In addition, an elevated level of lactate correlates with poor prognosis in several tumor-bearing patients stimulating tumor aggressiveness.Citation6
Our laboratory has contributed to unravel that acidity triggers in melanoma cells an epithelial-to-mesenchymal transition (EMT)-like profile, characterized by mesenchymal markers, high invasivity and attitude to produce metastasis. Citation17
In this study we provide evidence supporting that a transient exposure to low pH, either obtained increasing proton concentration or protons plus lactate, drives human melanoma cells to shift to an EMT phenotype coupled with an oxidative phosphorylation (Oxphos) metabolic program. Further, 1 mM metformin, a biguanide that inhibits mitochondrial complex I, was found to prevent the invasive mesenchymal phenotype of acidic melanoma cells. In addition, rising metformin concentration to 10 mM, a non toxic dose for melanoma cells grown in a standard pH medium, leads to an almost complete inhibition of proliferation and colony formation of acidic cells, both grown in proton or lactic acidosis.
Results
Human melanoma cells grown in an acidified medium express a mesenchymal phenotype and switch to oxidative phosphorylation
To analyze the influence of a reduced pH on human melanoma phenotype, we exposed A375M6 cells for 24 hours to a pH 6.7 acidified medium. Extracellular pH of melanoma frequently ranges between 6.7–6.9.Citation18,19,20 Exposure to an acidic medium did not change viability of melanoma cells although promoting a spindle and dendritic shape concomitant with an upregulation of N-cadherin and down regulation of E-cadherin, all characteristics of EMT, as we have already demonstrated.Citation17 Analysis of metabolic genes revealed that acidic melanoma cells express a profile compatible with a change to Oxphos, being GLUT 1 and 3, HK2, G6PD and LDHA significantly down regulated (). Also mRNA and protein of M2 splice isoform of pyruvate kinase (PK), an enzyme that shifts glucose metabolism toward aerobic glycolysis, was reduced (). Studying monocarboxylate transporters, we found a reduction of MCT4 (promoter of lactate efflux), that was accompanied by an increased level of MCT1 (promoter of lactate influx) (). Indeed, acidic melanoma cells reduce their lactate secretion () and protein synthesis (), whereas they increase lactate usage for CO2 production (). Quantitative PCR analyses showed specific upregulation of genes associated with mitochondrial biogenesis and oxidative phosphorylation, such as peroxisome proliferator-activated receptor gamma, coactivator 1 alpha (PGC-1α) and cytochrome c (CytC), whereas cytochrome c oxidase, 5B subunit (Cox5b) was found unmodified (). Overall, these observations suggest a conversion of acidic melanoma cells toward Oxphos metabolism. A possible increase of ATP production concomitant with the acquisition of a respiratory metabolism might be responsible of a reduction of AMP-activated protein kinase α (AMPKα) and its phosphorylated form (pAMPKα), as we found in acidic melanoma cells (). This finding also parallels reduction of glucose transporters and glycolysis.Citation21
Figure 1. Metabolic profile of acidic melanoma cells: A) Quantitative real-time PCR of GLUT1, GLUT3, HK2, G6PD, PKM2, LDHA expression in melanoma cells grown for 24 hours in acidic (pH 6.7) or non-acidic medium (pH 7.4). B) Western blot analysis of MCT-1, MCT-4 and PKM2 in acidic and non-acidic melanoma cells. Each band of Western blot was quantified by densitometric analysis and a corresponding histogram was constructed as relative to β-tubulin. Representative Western blot panels on the left. C) Lactate extruded by acidic or non-acidic melanoma cells normalized on number of cells. D) Incorporation of [14C] into proteins and E) respiration of [14C]-lactate, evaluated as [14C]-CO2 released. F) Expression of Oxphos genes (COX5B, CytC, PGC1α) and, G) AMPKα1 expression and phosphorylation (pAMPKα1). Values presented are mean ± SEM of 3 independent experiments. * p < 0.05.
![Figure 1. Metabolic profile of acidic melanoma cells: A) Quantitative real-time PCR of GLUT1, GLUT3, HK2, G6PD, PKM2, LDHA expression in melanoma cells grown for 24 hours in acidic (pH 6.7) or non-acidic medium (pH 7.4). B) Western blot analysis of MCT-1, MCT-4 and PKM2 in acidic and non-acidic melanoma cells. Each band of Western blot was quantified by densitometric analysis and a corresponding histogram was constructed as relative to β-tubulin. Representative Western blot panels on the left. C) Lactate extruded by acidic or non-acidic melanoma cells normalized on number of cells. D) Incorporation of [14C] into proteins and E) respiration of [14C]-lactate, evaluated as [14C]-CO2 released. F) Expression of Oxphos genes (COX5B, CytC, PGC1α) and, G) AMPKα1 expression and phosphorylation (pAMPKα1). Values presented are mean ± SEM of 3 independent experiments. * p < 0.05.](/cms/asset/bfc08d46-6896-48e3-93e6-6f667cd56c62/kccy_a_1191706_f0001_b.gif)
Metformin inhibits both mesenchymal profile and metabolic adaptation of acidic melanoma cells
Emerging evidence from clinical and experimental studies have demonstrated that biguanides, such as metformin and phenformin, in addition to be used for type 2 diabetes, also possess antitumor activity possibly related to AMPKα oncosuppressor promotion favored by the inhibition of complex I of mitochondrial respiratory chain.Citation22 Thus, metabolic trait of acidic melanoma cells represents a suitable target of biguanides. Metformin (1 mM final concentration) was able to restore N- and E-cadherin levels of acidic melanoma cells to those of cells grown in standard pH medium (), and E-cadherin was significantly up-regulated by metformin also in non-acidic melanoma cells. Further, the higher invasiveness of acidic melanoma cells reduced after metformin treatment to a level of control cells (). Metformin was also able to reconstitute GLUT1 and 3, HK2, G6PD, PKM2 and LDHA expression to that of non-acidic cells (). As expected, metformin stimulated AMPKα expression () and phosphorylation () in non-acidic cells. A comparable stimulation of AMPKα was found in metformin-treated acidic melanoma cells (), a finding also disclosing metformin efficiency in a 6.7 ± 0.1 pH environment. Indeed, many drugs used in oncology are weak bases (such as anthracyclines, antraquinones and vinca alkaloids) and for that reason may be inactivated by an acidic microenvironment.Citation23
Figure 2. Metformin inhibition mesenchymal profile and metabolic adaptation of acidic melanoma cells: A) Evaluation by quantitative real-time PCR of N- and E-Cadherin expression in metformin-treated (1 mM for 24 hours) melanoma cells grown in acidic (pH 6.7) or non-acidic medium (pH 7.4). B) Change in invasiveness through Matrigel-coated filters of metformin-treated acidic melanoma cells. C) Quantitative real-time PCR of GLUT1, GLUT3, HK2, G6PD, PKM2, LDHA and AMPKα1 expression in metformin-treated acidic or non-acidic melanoma cells. D) Metformin promotion of AMPKα1 activity evaluated by western blot of pAMPKα1. Values presented are mean ± SEM of 3 independent experiments. * p < 0.05.
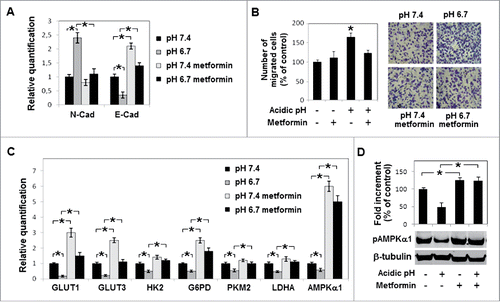
Human melanoma cells grown in an acidified medium containing lactate express a mesenchymal phenotype characterized by an enhanced motility and switch to oxidative phosphorylation
We added 10 mM sodium lactate in an acidified medium (final pH 6.7) and melanoma cells were grown for 24 hours. Experiments were conducted using melanoma cells grown in standard pH (7.3–7.4), melanoma cells grown in acidified medium (pH 6.7) or melanoma cells grown in standard pH medium enriched with sodium lactate (pH 7.3–7.4) as controls. Melanoma cells exposed to lactic acidosis express a spindle and dendritic shape similar to that of acidic cells () and their proliferative rate was inhibited in a similar way of that of acidic cells (). Furthermore, level of N-, E-cadherin () and invasiveness through Matrigel () were comparable to those of acidic cells. Lactic acidosis, as we show in , contributes to EMT profile of acidic melanoma cells stimulating motility, at an equivalent level to cells grown in standard pH medium. α-Cyano-4-hydroxycinnamate (CHC) treatment inhibits lactate-induced motility and invasiveness, and restores E-cadherin level, whereas effect on N-cadherin was less evident considering the promoting role of CHC on standard pH-exposed cells (). GLUT1 and 3, HK2, PKM2 and LDHA expression in melanoma cells adapted to lactic acidosis was found similar to that of acidic cells (). Analysis of mitochondrial respiration indicates that lactic acidosis promotes CytC and PGC-1α expression, although at a lower level than that we found using acidic cells (). Alongside, addition of sodium lactate into acidic medium reduced MCT4 and promoted MCT1 (). On the whole, lactate addition to low pH medium did not substantially modify oxidative profile of acidic melanoma cells in any of the investigated metabolic markers.
Figure 3. Effects of sodium lactate addition to the acidic medium on melanoma cells: A) Cell morphology and B) growth of A375M6 human melanoma cells in acidic medium containing 10 mM sodium lactate. C) Quantitative real-time PCR of N- and E-Cadherin expression in melanoma cells grown in acidic (pH 6.7) medium containing or not sodium lactate. 18s ribosomal RNA was used as reference gene. D) Invasiveness through Matrigel-coated filters of melanoma cells grown in acidic medium containing or not sodium lactate, and in the presence or absence of Ilomastat. Migration is reported as a percentage of control cells. E) Wound healing assay of melanoma cells grown in acidic (pH 6.7) or non-acidic (pH 7.4) medium with or without sodium lactate. Efficiency of closure was evaluated 18 and 24 hours after wounding (see histogram and statistics at the 24th hour). Data are expressed as mean ± SEM of 3 independent experiments.* p < 0.05.
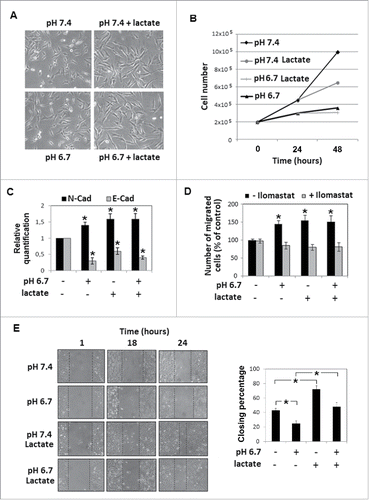
Figure 4. Effects of CHC treatment on melanoma cells grown in an acidic medium with sodium lactate: Quantitative real-time PCR of A) N-Cadherin and B) E-Cadherin mRNA expression in melanoma cells grown in acidic (pH 6.7) or non-acidic medium in the presence or absence of 10 mM sodium lactate and treated with 5mM CHC. C) Change in invasiveness through Matrigel-coated filters of CHC-treated A375M6 melanoma cells grown in an acidic medium containing sodium lactate. Data are expressed as mean ± SEM of 3 independent experiments. * p < 0.05. D) Wound healing assay of melanoma cells grown in acidic (pH 6.7) or non-acidic (pH 7.4) medium with or without sodium lactate. The wound closure was evaluated 18 hours since its creation (see histograms and statistics). *p < 0.05.
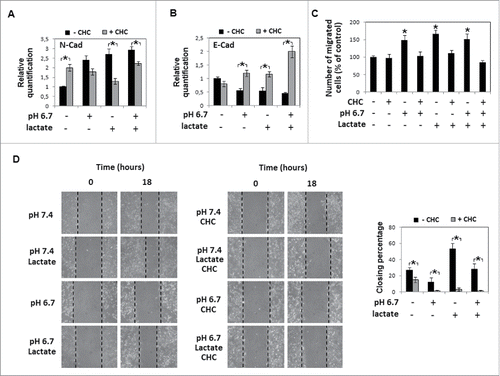
Figure 5. Metabolic profile of acidic cells treated with sodium lactate: A) Quantitative real-time PCR of glucose transporters GLUT1 and GLUT3, glycolytic enzymes HK2, PKM2, LDHA and oxphos genes (COX5B, CytC, PGC1α) expression in melanoma cells grown in acidic (pH 6.7) or non-acidic medium (pH 7.4) in the presence or absence of 10 mM sodium lactate. B) Western blot analysis of MCT-1 and MCT-4 expression in melanoma cells grown for 24 hours in acidic medium in the presence or absence of sodium lactate. Each band of western blot was quantified by densitometric analysis and the corresponding histogram was constructed as relative to β-tubulin. Representative Western blot panels on the left. Data are expressed as mean ± SEM of 3 independent experiments. * p< 0.05.
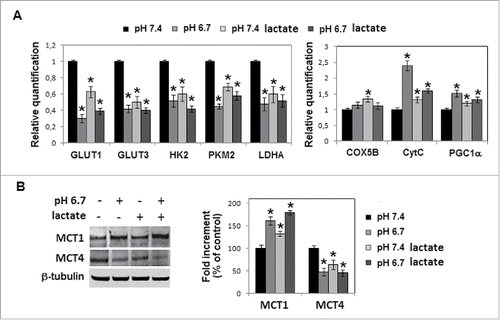
Metformin inhibits both mesenchymal profile and metabolic adaptation of lactic acidosis-exposed melanoma cells, disclosing a potential cytotoxic effect
Now, we tested metformin, a biguanide inhibitor of mitochondrial oxidative phosphorylation, to verify its possible effect on lactic acidosis exposed melanoma cells. Metformin reduced N-cadherin expression () and invasiveness () that were upregulated growing melanoma cells in acidified medium containing sodium lactate. Oxphos metabolic profile acquired by acidic cells also in the presence of sodium lactate was re-converted by metformin to a more glycolytic metabolism, as suggested by promotion of GLUT 1 and 3, HK2, LDHA (), PKM2, MCT4 (), and inhibition of MCT1 (), CytC and PGC-1α (). Level of Cox5B, even though did not change after acidic exposure, was downregulated by metformin treatment (). As expected, metformin promoted AMPKα expression () and phosphorylation () in melanoma cells exposed to sodium lactate as well as to sodium lactate added to an acidic medium.
Figure 6. Metformin inhibition of both mesenchymal profile and metabolic adaptation of lactic acidosis-exposed melanoma cells: A) Densitometric analysis of N-cadherin protein level, B) invasiveness and C) quantitative real-time PCR of GLUT1, GLUT3, HK2, LDHA, AMPKα1, COX5B, CytC and PGC1α in melanoma cells grown in acidic (pH 6.7) or non-acidic medium containing sodium lactate and treated with 1mM metformin. D) Western blot analysis of MCT-1, MCT-4, PKM2 and pAMPKα1 of melanoma cells grown in acidic medium in the presence of sodium lactate and/or metformin. Each band of western blot was quantified by densitometric analysis and the corresponding histogram was constructed as relative to β-tubulin. Representative Western blot panels on the left. Data are expressed as mean ± SEM of 3 independent experiments. * p < 0.05.
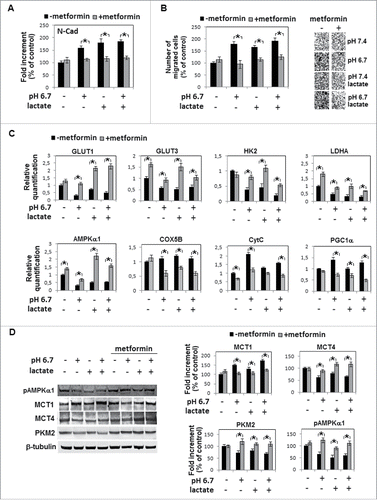
These findings prompted us to test whether metformin might exert at 5 to 10 mM concentration a more dramatic and cytotoxic effect on acidic melanoma cells. We found that metformin induced in acidic melanoma cells a dose (from 1 to 10 mM)-dependent inhibition of proliferation () and colony formation (), at both number and dimension levels, not observed in cells grown in standard pH and exposed to the same amount of metformin. Also, metformin used at 1 to 10 mM final concentration, exerted a progressively more cytostatic and cytotoxic effect on lactic acidosis-exposed melanoma cells, e.g., 10 mM metformin inhibits almost completely growth and colony formation ().
Figure 7. Viability and colony-forming ability of melanoma cells exposed to proton or lactic acidosis and treated with metformin at different dosages: A) Growth of melanoma cells for 24 hours in a medium of pH 7.4 or 6.7 and treated with 1, 5 or 10 mM metformin. Histograms refer percentage of viable (in gray) and dead (in black) cells. B) Representative images and C) histograms of diameter and number of colonies obtained by seeding, in a 4 cm dish, 4 × 103 cells collected from metformin-treated (1, 5 or 10 mM for 24 hours) acidic and non-acidic melanoma cultures. Data are expressed as mean ± SEM of 3 independent experiments. * p < 0.05.
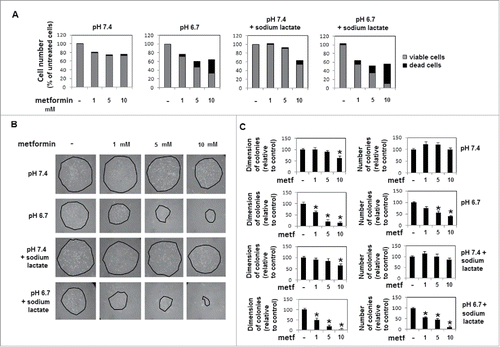
Discussion
It is likely that cancer cells use various metabolic solutions to perform different tasks involved in their progression, and information regarding metabolic adaption of tumor cells is critical to elaborate a useful strategy of therapy. A side effect of metabolic adaptation is considered the reduced pH which characterized extracellular microenvironment of the most tumor histotypes, including melanoma. Considering that perfusion of tumor tissues may change every time in an unexpected way, we used a model system of transient acidosis. Here, we show that an acidic pH medium promotes an Oxphos metabolism in human melanoma cells that underwent an EMT profile, as previously reported.Citation17 The enhanced invasive properties of an EMT program coupled with a respiratory metabolism of acidic melanoma cells was also reported for circulating tumor cells by LeBleu VS et al.Citation24 Evidence confirms that intracellular concentration of H+ influences glycolysis by directly influencing the activity of phosphofructokinase,Citation25 and inhibition of HIF-1α in acidosis might favor a respiratory metabolism.Citation26,27 In line with this change PKM2, the splice variant of PKM1 which dephosphorylates phosphoenolpyruvate into pyruvate, the last step in glycolysis crucial for subsequent metabolic flow to lactate, tricarboxylic acid cycle or biosynthetic pathway, is reduced in acidic melanoma cells. PKM2 is found in proliferating tumor cells of different histological subtypes Citation28 and is required for Warburg effect.Citation29 Acidosis induces metabolic reprogramming to Oxphos also in breast carcinoma cells Citation30 and malignant neuroblastoma cells.Citation31 Burd RCitation32 demonstrated that melanoma cells adapted at low pH do not undergo Crabtree effect, signifying that also in the presence of a high level of glucose cells continue to use a mitochondrial respiration; and Ho J reported the importance of Oxphos pathway in advanced melanoma using a clinical setting.Citation33 We contributed to demonstrate that extracellular acidity reprograms mesenchymal stem cells to Oxphos, a metabolic change that was associated with an ability to promote in melanoma cells an aggressive phenotype. Citation34
We also found a reduced expression and activation of AMPKα in acidic melanoma cells. AMPK controls energy homeostasis and when cells enter metabolic stress AMPK is activated shutting down energy-consuming processes such as fatty acid and protein synthesis. Further, JT Thaiparambil et al.Citation35 showed that AMPK depletion causes a mitotic delay and a reduced number and length of astral microtubules suggesting a clear association between AMPK and cell proliferation. Reduction of AMPK might favor usage of other substrates instead of glucose, and an enhanced synthesis of protein and fatty acids and sterol might have a role in cell survival. It is known that most acyl groups in tumor lipids result from de novo synthesis rather than from the import of fatty acids of the extracellular milieu.Citation36
A mitochondrial respiration poison able to activate AMPK is well represented by metformin, which was found to promote 30% reduction in cancer risk in diabetic patients.Citation37 Metformin restores and even stimulates AMPK activation in acidic BRAF-mutant melanoma cells; in parallel, it reverts EMT profile of acidic cells to a less invasive and more epithelial-like style, endoweed with a more glycolytic metabolism. The ability of metformin in increasing protein levels of key glycolytic molecules as GLUT1 and LDHA in melanoma cells has already been observed by Chaube et al.Citation38
Considering that microenvironmental acidosis of tumors depends not only on the increase of H+ concentration but also on lactic acidosis, we performed a series of experiments to investigate significance of sodium lactate addition to H+. On the whole, we found that lactic acidosis promotes EMT characters and potentiation of cell motility was the phenotypic aspect acquired by the EMT profile of lactic acidosis-exposed melanoma cells. Lactic acidosis-exposed cells also showed an Oxphos metabolism.
Metformin concentrations between 1 to 10 mM produced significant cytostatic and cytotoxic effects on acidic melanoma cells, either grown in proton or lactic acidosis. Thus, abrogation of colony formation exerted by 10 mM metformin on acidic melanoma cells discloses a potential target of metformin.
It is known that metformin preferentially targets cancer stem cells of a population of transformed MCF-10A or MCF-7 breast cancer cells and synergistically with doxorubicin kills both cancer stem cells and non-stem-cancer cells.Citation39 Metformin also inhibits growth of osteosarcoma cells amplifying the effect of cisplatin.Citation40 Regarding melanoma, Tomic T et al. Citation41 showed that metformin inhibits A375 melanoma cells (a BRAF mutated melanoma cell line) stimulating autophagy and apoptosis, although MJ Martin et al. Citation42 found that metformin accelerates growth of different BRAF-mutant melanoma cells, including A375 cells. These differences were justified considering some discrepancies during in vivo treatment. On the other hand, it was demonstrated a Vemurafenib and metformin synergism in BRAF-mutated melanoma treatment.Citation43 A synergistic activity was also ascertained combining proton pump inhibitors and chemotherapy in several tumors, supporting new trials for patients.Citation44,45
To conclude, our study provides evidences that metformin is useful to combat subpopulations of melanoma cells located within an acidic niche, thereby amplifying metformin possible therapeutic use. This is of a particular importance considering the high resistance to apoptosis and chemotherapy elicited by an acidic microenvironment on malignant cells.Citation46
Material and methods
Cell lines and culture conditions
In this study, we used the melanoma cell line A375M6, isolated in our laboratory from lung metastasis of SCID bg/bg mice i.v. injected with A375 human melanoma cell line, obtained from American Type Culture Collection (ATCC, Rockville, MD). Melanoma cells were cultivated in Dulbecco's Modified Eagle Medium high glucose (DMEM 4500, EuroClone, MI, Italy) supplemented with 10% foetal bovine serum (FBS, Boerhinger Mannheim, Germany), at 37°C in humidified atmosphere containing 90% air and 10% CO2.
Cells were harvested from subconfluent cultures by incubation with a trypsin-EDTA solution (EuroClone, MI, Italy), and propagated every 3 days. Viability of the cells was determined by trypan blue exclusion test. Cultures were periodically monitored for mycoplasma contamination using Chen's fluorochrome test.Citation47
Acidic treatment
Acidified medium was obtained by the addition of HCl 1N in DMEM 4500 10%FBS, pH value was monitored by a pHmeter (Orion PH Meter 520A-1). As pH value was stable at 6.7 ± 0.1, acidified medium was added to cell cultures and the seal cap was tightly closed to prevent buffering. After 24 hours, at the end of exposure, pH was also evaluated. For inhibition assays, 1–10 mM metformin (Sigma-Aldrich, St. Louis, Missouri), or 5 mM α-Cyano-4-hydroxycinnamic acid (CHC, Sigma-Aldrich) were added 1 h before acidic treatment to cell cultures and then leaved in acidic medium until the end of incubation time. In some experiments, 10 mM sodium lactate (Sigma-Aldrich) was added to acidified medium.
Western blotting analysis
Cells were washed with ice cold PBS containing 1 mM Na4VO3, and lysed in 100 μl of cell RIPA lysis buffer (Merk Millipore, Vimodrone, MI, Italy) containing PMSF (Sigma-Aldrich), sodium orthovanadate (Sigma-Aldrich) and protease inhibitor cocktail (Calbiochem). Aliquots of supernatants containing equal amounts of protein (60 μg) in Laemmli buffer were separated on Bolt® Bis-Tris Plus gels 4–12% precast polyacrylamide gels (Life Technologies, Monza, Italy). Fractionated proteins were transferred from the gel to a PVDF nitrocellulose membrane using an electroblotting apparatus (Bio-Rad, Segrate, MI, Italy). Blots were stained with Ponceau red to ensure equal loading and complete transfer of proteins, then they were blocked for 1 hours, at room temperature, with Odyssey blocking buffer (Dasit Science, Cornaredo, MI, Italy). Subsequently, the membrane was probed at 4°C overnight with primary antibodies diluited in a solution of 1:1 Odyssey blocking buffer/T-PBS buffer. The primary antibodies were: rabbit anti-N-Cadherin (Biorbyt, Cambridge, UK), rabbit anti-MCT-1, rabbit anti-MCT-4 (Santa Cruz Biotechnology, Santa Cruz, California), rabbit anti PKM2 and rabbit anti p-AMPKα (Cell signaling Technology, Danvers, MA, US). The membrane was washed in T-PBS buffer, incubated for 1 hour at room temperature with goat anti-rabbit IgG Alexa Flour 680 antibodies (Invitrogen, Monza, Italy), and then visualized by an Odyssey Infrared Imaging System (LI-COR® Bioscience). Mouse anti-α-tubulin monoclonal antibody (Sigma, Saint Louis, MO, USA) was used to assess equal amount of protein loaded in each lane.
RNA isolation and quantitative PCR (qPCR)
Total RNA was extracted from cells by using TRI Reagent (Sigma). The amount and purity of RNA were determined spectrophotometrically. cDNA synthesis was obtained by incubating 2 μg of total RNA with 4 U/μl of M-MLV reverse transcriptase (Promega, San Luis Obispo, California) according to the manufacturer's instructions.
Quantitative real time PCR (qPCR) was performed using the GoTaq® Probe Systems (Promega). The qPCR analysis was carried out in triplicate using an Applied Biosystems 7500 Sequence Detector with the default PCR setting: 40 cycles of 95° for 15 seconds and 60°C for 60 seconds. mRNA was quantified with the ▵▵Ct method as described.Citation48 mRNA levels were normalized to 18 S as an endogenous control. Primer sequences are reported in .
Table 1. Primer sequences for PCR.
Invasion assay
Invasion of A375M6 melanoma cells was determined in vitro on Matrigel-coated polycarbonate filters (8 µm pore size, 6.5 mm diameter), 12.5 μg Matrigel/filter, mounted in Boyden's chambers. 1.5 × 10Citation5 cells suspended in 200 µL of their own growth medium were seeded in the upper compartment, while in the lower chamber fresh complete medium was added as chemo attractant. Cells were incubated for 6 hours at 37°C, 10% CO2 in air. In some experiments, invasion was performed in the presence of Ilomastat, a MMPs inhibitor (Millipore, Billerica, Massachussets). After incubation, filters were removed and the non-invading cells on the upper surface were wiped-off mechanically with a cotton swab. Cells on the lower side of the filters were fixed overnight in ice-cold methanol, then stained using a Diff-Quick kit (BD Biosciences) and pictures of randomly chosen fields were taken.
Wound healing assay
Cell migration was evaluated by an in vitro wound healing assay. Cells were grown at 80–90% confluence in 35 mm dishes; the cell layer was wounded with a sterile 200 μl pipette tip and incubated in 1% FBS culture medium for 24 hours. The wound was observed after 18 or 24 hours and pictures were taken using phase contrast microscopy.
Lactate assay
Lactate was measured in cultured media with Lactate Assay kit (Source Bioscience Life Sciences; Nottingham, UK) according to the manufacturer's instruction. In brief, samples were prepared in 50 μl/well with Lactate Assay Buffer in a 96-well plate. 50 μl Reaction Mix containing 46 μl Lactate assay buffer, 2 μl Probe, 2 μl Enzyme Mix were added to each well and the reaction was incubated for 30 minutes at room temperature, protected from light. Lactate reacts with enzyme mix to generate a product, which interacts with probe to produce fluorescence.
Incorporation of lactate into proteins
Cells were grown in acidic medium for 24 hours and then [U-14C] lactate was added for other 24 hours (2 μCi/ml, final concentration). Cells were then resuspended in 20% trichloroacetic acid, placed on ice for 30 minutes and centrifuged at 12,000 x g for 15 minutes at room temperature. The supernatant was removed and the pellet resuspended with 200 μl of water. The resuspended pellet was assayed for [14C] labeled proteins by scintillator. Citation49
Detection of released CO2 by radioactive lactate
Cells were grown in acidic medium for 24 hours and then 0.2 μCi/ml D-[U-14C] lactate was added for 15 minutes in incubator at 37°C, 5% CO2. Each dish had a taped piece of Whatman paper facing the inside of the dish wetted with 100 μl of phenyl-ethylamine-methanol (1:1) to trap the CO2. Then 200 μl of 4 M H2SO4 was added to cells. Finally, Whatman paper was removed, transferred to scintillation vials and radioactive CO2 released was counted by scintillator.
Plate Colony forming assay
Cells treated with metformin were counted and approximately 200 cells/ml were seeded in fresh medium into each well of a 6-well culture plate and incubated for 6 days at 37°C. Cells were washed twice in PBS, fixed with cold methanol, stained with Giemsa solution and washed with ddH2O. The stained cells were photographed with a digital camera. The number of colonies in each well was counted.
Statistical analysis
Densitometric data are expressed as means ± standard errors of the mean (SEM) depicted by vertical bars from at least 3 independent experiments. Statistical analysis of the data was performed by Student's t-test, and p ≤ 0.05 was considered statistically significant.
Abbreviations
HIF-1α | = | hypoxia-inducible factor 1α |
EMT | = | epithelial-to-mesenchymal transition |
Oxphos | = | oxidative phosphorylation |
MCT | = | monocarboxylated transporter |
VEGF | = | vascular endothelial growth factor |
LDH | = | lactate dehydrogenase |
GLUT | = | glucose transporter |
HK2 | = | hexokinase 2 |
G6PD | = | glucose-6-phosphate dehydrogenase |
AMPK | = | AMP-activated protein kinase |
PKM2 | = | pyruvate kinase isozymes M2 |
Disclosure of potential conflicts of interest
No potential conflicts of interest were disclosed.
Funding
This study was financially supported by grants from Istituto Toscano Tumori, Ente Cassa di Risparmio di Firenze and AIRC (#14266).
References
- Gatenby RA, Gillies RJ. Why do cancers have high aerobic glycolysis? Nat Rev Cancer 2004; 4:891-9; PMID:15516961; http://dx.doi.org/10.1038/nrc1478
- Vander Heiden MG, Cantley LC, Thompson CB. Understanding the Warburg effect: the metabolic requirements of cell proliferation. Science 2009; 324:1029-33; PMID:19460998; http://dx.doi.org/10.1126/science.1160809
- Delbeke D, Coleman RE, Guiberteau MJ, Brown ML, Royal HD, Siegel BA, Townsend DW, Berland LL, Parker JA, Hubner K, et al. Procedure guideline for tumor imaging with 18F-FDG PET/CT 1.0. J Nucl Med 2006; 47:885-95; PMID:16644760
- Denko NC. Hypoxia, HIF1 and glucose metabolism in the solid tumour. Nat Rev Cancer 2008; 8:705-713; PMID:19143055; http://dx.doi.org/10.1038/nrc2468
- Spugnini EP, Sonveaux P, Stock C, Perez-Sayans M, De Milito A, Avnet S, Garcìa AG, Harguindey S, Fais S. Proton channels and exchangers in cancer. Biochim Biophys Acta 2015; 1848:2715-26; PMID:25449995; http://dx.doi.org/10.1016/j.bbamem.2014.10.015
- Walenta S, Wetterling M, Lehrke M, Schwickert G, Sundfør K, Rofstad EK, Mueller-Klieser W. High lactate levels predict likelihood of metastases, tumor recurrence, and restricted patient survival in human cervical cancers. Cancer Res 2000; 60:916-21; PMID:10706105
- Calorini L, Peppicelli S, Bianchini F. Extracellular acidity as favouring factor of tumor progression and metastatic dissemination. Exp Oncol 2012; 34:79-84; PMID:23013757
- Raghunand N, Mahoney B, van Sluis R, Baggett B, Gillies RJ. Acute metabolic alkalosis enhances response of C3H mouse mammary tumors to the weak base mitoxantrone. Neoplasia 2001; 3:227-35; PMID:11494116; http://dx.doi.org/10.1038/sj.neo.7900151
- Trédan O, Galmarini CM, Patel K, Tannock IF. Drug resistance and the solid tumor microenvironment. J Natl Cancer Inst 2007; 99:1441-54; http://dx.doi.org/10.1093/jnci/djm135
- Fischer K, Hoffmann P, Voelkl S, Meidenbauer N, Ammer J, Edinger M, Gottfried E, Schwarz S, Rothe G, Hoves S, et al. Inhibitory effect of tumor cell-derived lactic acid on human T cells. Blood 2007; 109:3812-9; PMID:17255361; http://dx.doi.org/10.1182/blood-2006-07-035972
- Calcinotto A, Filipazzi P, Grioni M, Iero M, De Milito A, Ricupito A, Cova A, Canese R, Jachetti E, Rossetti M, et al. Modulation of microenvironment acidity reverses anergy in human and murine tumor-infiltrating T lymphocytes. Cancer Res 2012; 72:2746-56; PMID:22593198; http://dx.doi.org/10.1158/0008-5472.CAN-11-1272
- Morita T, Nagaki T, Fukuda I, Okumura K. Clastogenicity of low pH to various cultured mammalian cells. Mutat Res 1992; 268:297-305; PMID:1379335; http://dx.doi.org/10.1016/0027-5107(92)90235-T
- Rofstad EK, Mathiesen B, Kindem K, Galappathi K. Acidic extracellular pH promotes experimental metastasis of human melanoma cells in athymic nude mice. Cancer Res 2006; 66:6699-707; PMID:16818644; http://dx.doi.org/10.1158/0008-5472.CAN-06-0983
- Moellering RE, Black KC, Krishnamurty C, Baggett BK, Stafford P, Rain M, Gatenby RA, Gillies RJ. Acid treatment of melanoma cells selects for invasive phenotypes. Clin Exp Metastasis 2008; 25:411-25; PMID:18301995; http://dx.doi.org/10.1007/s10585-008-9145-7
- Shi Q, Le X, Wang B, Abbruzzese JL, Xiong Q, He Y, Xie K. Regulation of vascular endothelial growth factor expression by acidosis in human cancer cells. Oncogene 2001; 20:3751-3756; PMID:11439338; http://dx.doi.org/10.1038/sj.onc.1204500
- Peppicelli S, Bianchini F, Contena C, Tombaccini D, Calorini L. Acidic pH via NF-κB favours VEGF-C expression in human melanoma cells. Clin Exp Metastasis 2013; 30:957-67; PMID:23784694; http://dx.doi.org/10.1007/s10585-013-9595-4
- Peppicelli S, Bianchini F, Torre E, Calorini L. Contribution of acidic melanoma cells undergoing epithelial-tomesenchymal transition to aggressiveness of non-acidic melanoma cells. Clin Exp Metastasis 2014; 31:423-433; PMID:24469963; http://dx.doi.org/10.1007/s10585-014-9637-6
- Vaupel P, Kallinowski F, Okunieff P. Blood flow, oxygen and nutrient supply, and metabolic microenvironment of human tumors: a review. Cancer Res 1989; 49:6449-6465; PMID:2684393
- Wike-Hooley JL, Haveman J, Reinhold HS. The relevance of tumour pH to the treatment of malignant disease. Radiother Oncol 1984; 2:343-66; PMID:6097949; http://dx.doi.org/10.1016/S0167-8140(84)80077-8
- De Milito A, Canese R, Marino ML, Borghi M, Iero M, Villa A, Venturi G, Lozupone F, Iessi E, Logozzi M, et al. pH-dependent antitumor activity of proton pump inhibitors against human melanoma is mediated by inhibition of tumor acidity. Int J Cancer 2010 Jul 1; 127(1):207-19; http://dx.doi.org/10.1002/ijc.25009
- Hardie DG. AMP-activated/SNF1 protein kinases: conserved guardians of cellular energy. Nat Rev Mol Cell Biol 2007; 8:774-85; PMID:17712357; http://dx.doi.org/10.1038/nrm2249
- Pollak MN. Investigating metformin for cancer prevention and treatment: the end of the beginning. Cancer Discov 2012; 2:778-90; PMID:22926251; http://dx.doi.org/10.1158/2159-8290.CD-12-0263
- Wojtkowiak JW, Verduzco D, Schramm KJ, Gillies RJ. Drug resistance and cellular adaptation to tumor acidic pH microenvironment. Mol Pharm 2011; 8:2032-8; PMID:21981633; http://dx.doi.org/10.1021/mp200292c
- LeBleu VS, O'Connell JT, Gonzalez Herrera KN, Wikman H, Pantel K, Haigis MC, de Carvalho FM, Damascena A, Domingos Chinen LT, Rocha RM, et al. PGC-1α mediates mitochondrial biogenesis and oxidative phosphorylation in cancer cells to promote metastasis. Nat Cell Biol 2014; 16:992-1003, 1–15; http://dx.doi.org/10.1038/ncb3039
- Erecińska M, Deas J, Silver IA. The effect of pH on glycolysis and phosphofructokinase activity in cultured cells and synaptosomes. J Neurochem 1995; 65:2765-72; PMID:7595576; http://dx.doi.org/10.1046/j.1471-4159.1995.65062765.x
- Parks SK, Mazure NM, Counillon L, Pouysségur J. Hypoxia promotes tumor cell survival in acidic conditions by preserving ATP levels. J Cell Physiol 2013; 228:1854-62; PMID:23459996; http://dx.doi.org/10.1002/jcp.24346
- Tang X, Lucas JE, Chen JL, LaMonte G, Wu J, Wang MC, Koumenis C, Chi JT. Functional interaction between responses to lactic acidosis and hypoxia regulates genomic transcriptional outputs. Cancer Res 2012; 72:491-502; PMID:22135092; http://dx.doi.org/10.1158/0008-5472.CAN-11-2076
- Altenberg B, Greulich KO. Genes of glycolysis are ubiquitously overexpressed in 24 cancer classes. Genomics 2004; 84:1014-20; PMID:15533718; http://dx.doi.org/10.1016/j.ygeno.2004.08.010
- Christofk HR, Vander Heiden MG, Harris MH, Ramanathan A, Gerszten RE, Wei R, Fleming MD, Schreiber SL, Cantley LC. The M2 splice isoform of pyruvate kinase is important for cancer metabolism and tumour growth. Nature 2008; 452:230-3; PMID:18337823; http://dx.doi.org/10.1038/nature06734
- Lamonte G, Tang X, Chen JL, Wu J, Ding CK, Keenan MM, Sangokoya C, Kung HN, Ilkayeva O, Boros LG, et al. Acidosis induces reprogramming of cellular metabolism to mitigate oxidative stress. Cancer Metab 2013; 1:23; PMID:24359630; http://dx.doi.org/10.1186/2049-3002-1-23
- Mazzio EA, Boukli N, Rivera N, Soliman KF. Pericellular pH homeostasis is a primary function of the Warburg effect: inversion of metabolic systems to control lactate steady state in tumor cells. Cancer Sci 2012; 103:422-32; PMID:22320183; http://dx.doi.org/10.1111/j.1349-7006.2012.02206.x
- Burd R, Wachsberger PR, Biaglow JE, Wahl ML, Lee I, Leeper DB. Absence of Crabtree effect in human melanoma cells adapted to growth at low pH: reversal by respiratory inhibitors. Cancer Res 2001; 61:5630-5; PMID:11454717
- Ho J, de Moura MB, Lin Y, Vincent G, Thorne S, Duncan LM, Hui-Min L, Kirkwood JM, Becker D, Van Houten B, et al. Importance of glycolysis and oxidative phosphorylation in advanced melanoma. Mol Cancer 2012; 11:76; PMID:23043612; http://dx.doi.org/10.1186/1476-4598-11-76
- Peppicelli S, Bianchini F, Toti A, Laurenzana A, Fibbi G, Calorini L. Extracellular acidity strengthens mesenchymal stem cells to promote melanoma progression. Cell Cycle 2015; 14:3088-100; PMID:26496168; http://dx.doi.org/10.1080/15384101.2015.1078032
- Thaiparambil JT, Eggers CM, Marcus AI. AMPK regulates mitotic spindle orientation through phosphorylation of myosin regulatory light chain. Mol Cell Biol 2012; 32:3203-17; PMID:22688514; http://dx.doi.org/10.1128/MCB.00418-12
- Kannan R, Lyon I, Baker N. Dietary control of lipogenesis in vivo in host tissues and tumors of mice bearing Ehrlich ascites carcinoma. Cancer Res 1980; 40:4606-11; PMID:7438094
- Evans JM, Donnelly LA, Emslie-Smith AM, Alessi DR, Morris AD. Metformin and reduced risk of cancer in diabetic patients. BMJ 2005; 330:1304-5; PMID:15849206; http://dx.doi.org/10.1136/bmj.38415.708634.F7
- Chaube B, Malvi P, Singh SV, Mohammad N, Meena AS, Bhat MK. Targeting metabolic flexibility by simultaneously inhibiting respiratory complex I and lactate generation retards melanoma progression. Oncotarget 2015; 6:37281-99; PMID:26484566
- Hirsch HA, Iliopoulos D, Tsichlis PN, Struhl K. Metformin selectively targets cancer stem cells, and acts together with chemotherapy to block tumor growth and prolong remission. Cancer Res 2009; 69:7507-11; PMID:19752085; http://dx.doi.org/10.1158/0008-5472.CAN-09-2994
- Quattrini I, Conti A, Pazzaglia L, Novello C, Ferrari S, Picci P, Benassi MS. Metformin inhibits growth and sensitizes osteosarcoma cell lines to cisplatin through cell cycle modulation. Oncol Rep 2014; 31:370-5; PMID:24253938
- Tomic T, Botton T, Cerezo M, Robert G, Luciano F, Puissant A, Gounon P, Allegra M, Bertolotto C, Bereder JM, et al. Metformin inhibits melanoma development through autophagy and apoptosis mechanisms. Cell Death Dis 2011; 2:e199; PMID:21881601; http://dx.doi.org/10.1038/cddis.2011.86
- Martin MJ, Hayward R, Viros A, Marais R. Metformin accelerates the growth of BRAF V600E-driven melanoma by upregulating VEGF-A. Cancer Discov 2012; 2:344-55; PMID:22576211; http://dx.doi.org/10.1158/2159-8290.CD-11-0280
- Yuan P, Ito K, Perez-Lorenzo R, Del Guzzo C, Lee JH, Shen CH, Bosenberg MW, McMahon M, Cantley LC, Zheng B. Phenformin enhances the therapeutic benefit of BRAF(V600E) inhibition in melanoma. Proc Natl Acad Sci U S A 2013; 110:18226-31; PMID:24145418; http://dx.doi.org/10.1073/pnas.1317577110
- Fais S. Proton pump inhibitor-induced tumour cell death by inhibition of a detoxification mechanism. J Intern Med 2010; 267:515-25; PMID:20433578; http://dx.doi.org/10.1111/j.1365-2796.2010.02225.x
- Fais S. Evidence-based support for the use of proton pump inhibitors in cancer therapy. J Transl Med 2015; 13:368; PMID:26597250; http://dx.doi.org/10.1186/s12967-015-0735-2
- Wojtkowiak JW, Verduzco D, Schramm KJ, Gillies RJ. Drug resistance and cellular adaptation to tumor acidic pH microenvironment. Mol Pharm 2011; 8:2032-8; PMID:21981633; http://dx.doi.org/10.1021/mp200292c
- Chen TR. In situ detection of mycoplasma contamination in cell cultures by fluorescent Hoechst 33258 stain. Exp Cell Res 104:2 55–2 62, 19 7 7.
- Livak KJ, Schmittgen TD. Analysis of relative gene expression datausing real-time quantitativePCR andthe2[-Delta Delta C(T)] Method. Methods 2001; 25:402-408; PMID:11846609; http://dx.doi.org/10.1006/meth.2001.1262
- Fiaschi T, Marini A, Giannoni E, Taddei ML, Gandellini P, De Donatis A, Lanciotti M, Serni S, Cirri P, Chiarugi P. Reciprocal metabolic reprogramming through lactate shuttle coordinately influences tumor-stroma interplay. Cancer Res. 2012; 72:5130-40; PMID:22850421; http://dx.doi.org/10.1158/0008-5472.CAN-12-1949