ABSTRACT
Polo-like kinase 1 (Plk1)-interacting checkpoint helicase (PICH) localizes at the centromere and is critical for proper chromosome segregation during mitosis. However, the precise molecular mechanism of PICH's centromeric localization and function at the centromere is not yet fully understood. Recently, using Xenopus egg extract assays, we showed that PICH is a promiscuous SUMO binding protein. To further determine the molecular consequence of PICH/SUMO interaction on PICH function, we identified 3 SUMO-interacting motifs (SIMs) on PICH and generated a SIM-deficient PICH mutant. Using the conditional expression of PICH in cells, we found distinct roles of PICH SIMs during mitosis. Although all SIMs are dispensable for PICH's localization on ultrafine anaphase DNA bridges, only SIM3 (third SIM, close to the C-terminus end of PICH) is critical for its centromeric localization. Intriguingly, the other 2 SIMs function in chromatin bridge prevention. With these results, we propose a novel SUMO-dependent regulation of PICH's function on mitotic centromeres.
Introduction
Proper sister chromatid disjunction during mitosis is a complex regulatory phenomenon that ensures genome stability. This complex regulation involves multiple families of proteins including kinases, phosphatases, histone modifiers and chromatin remodelers, coordinating their functions spatially and temporally.Citation1-4 Of these proteins, Polo-like kinase 1 (Plk1) interacting checkpoint helicase (PICH), a SNF2 family member of chromatin remodelers was identified as a binding partner and substrate of mitotic kinase, Plk1.Citation5 PICH localizes at the centromere/kinetochore region of chromosomes only during mitosis. Additionally, PICH localizes to ultrafine anaphase DNA bridges (UFBs), which are, specialized mitotic DNA structures that cannot be visualized by conventional DNA staining dyes.Citation5,6 The kinase activity of Plk1 is critical for the dynamic localization of PICH as depletion of Plk1 results in mislocalization of PICH on chromosomal arm regions.Citation5,7 Furthermore, mutant PICH without its ATPase activity localizes on chromosome arms even in the presence of Plk1.Citation8 Therefore in addition to Plk1, DNA-dependent ATPase activity of PICH is also required for the elimination of PICH from the arm regions of chromosomes. Although a few mechanisms for the removal of PICH from chromosomal arm regions have been proposed, the specific mechanism for the positive recruitment of PICH at the centromere/kinetochore region of chromosomes is not clear. The elimination of PICH by RNAi or genome editing results in chromatin bridge formation in mitosis and micro-nucleation after cytokinesis.Citation8,9 A PICH ATPase-deficient mutant could not rescue this phenotype, suggesting that ATP-dependent translocase activity of PICH is required for resolving chromatin bridges in mitosis.Citation8,9 While the essential function of PICH is known (i.e. to properly complete chromosome segregation), questions about how PICH localizes to the centromere and its precise function at the centromere and UFB at a molecular level are still under investigation.
Recently, we reported that PICH can covalently bind to SUMO through SUMOylation and also interact non-covalently.Citation10 SUMOylation is a posttranslational modification that regulates diverse cellular processes. SUMO modification of a protein can mediate novel protein-protein interaction and alter the protein's localization, activity, and stability.Citation11-14 SUMO promotes novel binding via SUMO-interacting motifs (SIMs) on the binding protein.Citation14-16 Proteins with SIM have been shown to regulate multiple biological processes including DNA replication, PML-body assembly and function, and mitosis.Citation14,17-19 Several genetic studies in model organisms and cell biological studies show that SUMOylation is a critical modification in dictating proper chromosome segregation during mitosis.Citation20 For instance, SUMO protease, SENP2 overexpression has been demonstrated to cause mitotic defects by induction of prometaphase arrest.Citation21,22 Additionally, human cells lacking SUMO E3 ligase PIASy results in a prolonged metaphase.Citation23 However, comprehensive roles of mitotic SUMOylation on chromosome segregation at the molecular level are not fully understood yet.
In this study, we identified a novel mechanism of localization of PICH on centromeres likely through PICH/SUMO interaction. Additionally, we uncovered the distinct function of each SIM on PICH in the regulation of PICH at mitotic centromeres. One of the PICH SIMs was important for robust PICH localization at the centromere and others were critical to PICH's function for resolving chromatin bridges during anaphase. Altogether, our results suggest a complex spatial and temporal regulation of PICH by its SUMO interaction and we propose a novel molecular mechanism for PICH's dynamic localization and activity during mitosis involving PICH/SUMO interaction.
Results
Multiple SIM domains in PICH mediate interaction with SUMOylated substrate in vitro
We identified PICH to be a promiscuous SUMO-binding protein by pull-down assay using Xenopus egg extract (XEE) and the PICH-SUMO interaction was conserved in humans.Citation10 To investigate the functional significance of the PICH/SUMO interaction, we utilized human PICH throughout this study and aimed to ascertain potential SIM sequences on PICH. In order to identify the SUMO-interacting regions on PICH, we made PICH truncations. Three truncation mutants covering and overlapping the N- and C-terminus portions of PICH were utilized for in vitro pull-down assays using SUMOylated-PARP1 (1–650 a.a.) with XEE expressing the mutants by mRNA addition to the XEE.Citation10 The C-terminal portion of PICH with amino acids 616–1250 was highly enriched, specifically on SUMOylated-PARP1 (1–650 a.a.) beads (). The SIM prediction programCitation24 suggested 4 potential SIM sequences within PICH 616–1250 a.a. Two were overlapping SIMs at amino acid 912–917, one at 1013–1016 and one at 1236–1239 () (henceforth referred to as SIM1, SIM2, and SIM3, respectively). We mutated the SIM sequences individually by changing the hydrophobic amino acids to alanine () and analyzed their interactions with SUMOylated-PARP1. Individual SIM mutants within the context of truncated PICH (616–1250 a.a.) were expressed in XEE and a pull-down assay was performed. None of the individual SIM mutations showed a clear reduction in SUMO-PICH interaction in vitro (Fig. S1A). Therefore, we mutated all the SIMs in full-length PICH (PICH d3SIM) and expressed it in XEE for pull-down assay. Immunoblotting analysis of the pulled-down samples showed a drastic reduction of PICH d3SIM binding to the SUMOylated-PARP1 (). Because SUMO/SIM interactions are relatively weak with dissociation constants between 60–90 μMCitation16 we surmise that all SIMs together contribute to the robust interaction of PICH to SUMOylated protein in the pull-down assay.
Figure 1. Polo-like kinase 1 interacting checkpoint helicase (PICH) has multiple SUMO-interacting motifs (SIMs). (A) C-terminus portion of human PICH (616–1250 a.a.) interacts robustly with SUMOylated-PARP1 (SPARP1) Xenoups laevis. Human PICH truncations with GFP tags were expressed in Xenopus egg extract by addition of mRNAs to the extract and pull-down assay was performed. The pulled-down samples were analyzed by immunoblotting using anti-GFP antibody. S-tag HRP depicted the amount of bait in the pull-down samples. (B) Schematic diagram of PICH with SIM sequences at a.a. 912–917 (VSIIEI), 1013–1016 (VVVK) and 1236–1239 (VMLL). Point mutations in each SIM are indicated in blue. (C) Mutations in all SIMs in PICH (PICH d3SIM) drastically reduced PICH binding to SUMOylated-PARP1 (SPARP1). XEE expressing GFP-tagged PICH WT and d3SIM were subjected to pull-down assays. Pulled-down samples were analyzed by immunoblotting using GFP antibody. S-protein HRP was used to detect the amount of bait in the samples. (D) PICH d3SIM is enzymatically active in vitro. Concentration- and time-dependent DNA translocase activity of PICH WT and d3SIM was tested in vitro by triplex assay. Alexa 488 tagged oligo was detected by Typhoon Imager. Positions of DNA triplex and released oligo are indicated at right side. (E and F) SIMs in PICH does not alter PICH's association with UFBs. Ectopically expressed EGFP-tagged PICH WT and PICH d3SIM induced in HeLa Tet-ON cells were detected by fluorescence microscopy. White bar on the merged images indicates 10 μm.
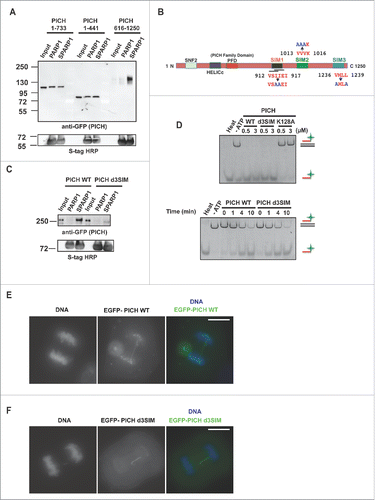
PICH d3SIM is enzymatically active in vitro
Since multiple amino acids were mutated to obtain a SUMO-interacting deficient PICH, we wanted to ensure that mutations do not affect the activity of PICH and thereby justify the use of PICH d3SIM in our future studies. PICH is a DNA-dependent ATPase and an ATP-dependent translocase.Citation25 For the verification of PICH activity, we tested the ability of PICH d3SIM to translocate on dsDNA by measuring the displacement of a fluorescently tagged oligo from a DNA duplex in vitro (DNA-triplex assay).Citation25 PICH d3SIM was enzymatically active and displaced the triplex-forming oligo similarly to PICH WT in a time- and concentration-dependent manner, whereas PICH K128A (ATPase-deficient mutant) had no activity, consistent with a previous study ().Citation25 These results suggest that mutations on SIMs do not affect DNA binding or the enzymatic activity of PICH.
PICH SIMs are critical for mitotic centromere localization of PICH but not for localization on UFBs
Localization of PICH on chromosomes has been shown to be highly dynamic. PICH localizes in the cytoplasm during interphase and is enriched at the centromere/kinetochore (KT) region during mitosis.Citation5 Additionally, PICH localizes to the ultra-fine DNA bridges (UFBs) during anaphase.Citation5 To test if SIM sequences are important for PICH's localization on the UFBs during anaphase, we generated tetracycline-inducible constructs of EGFP-fused PICH WT and PICH d3SIM to express exogenous PICH in HeLa Tet-ON cells.Citation26,27 We tested and confirmed the cytoplasmic localization of both EGFP-PICH WT and d3SIM during interphase, as previously reported (Fig. S1B).Citation5 Anaphase cells expressing exogenous EGFP-fused PICH WT and PICH d3SIM were visualized. We observed that both PICH WT and PICH d3SIM associated with UFBs without distinguishable differences during anaphase (). Interestingly, PICH d3SIM did not show chromosomal foci in contrast to WT, indicating a defect in the d3SIM mutant's association with the centromere/KT region. To test if SIM sequences are important for PICH's localization on the mitotic centromeres, we isolated different stages of mitotic cells and examined PICH centromere/KT region association. In mitotic cells, WT was enriched at the centromere/KT region as previously shown ().Citation5 On the other hand, PICH d3SIM's localization at the centromere/KT region was clearly reduced (). These results suggest that PICH SIM interaction with SUMO is likely essential for PICH localization and retention at the mitotic centromeres/KT region and the mechanism of PICH localization at the centromere/KT region is different from that at UFBs.
Figure 2. SIMs in PICH regulates centromere localization of PICH. (A) PICH WT localizes at the centromere/KT region during mitosis as previously shown. Ectopically expressed EGFP-PICH WT induced by doxycycline visualized in different mitotic stages of HeLa Tet-ON cells. Cells were subjected to immunofluorescence staining for CENPA antibody as a centromere marker. Right panel is the merged images with PICH in green and CENPA in red. White bar on the top right panel indicates 10 μm. (B) Localization of PICH d3SIM is drastically reduced at the centromere during mitosis. Similar to PICH WT, EGFP-tagged PICH d3SIM expression was induced and detected along with CENPA by immunofluorescence. White bar on the top right panel indicates 10 μm.
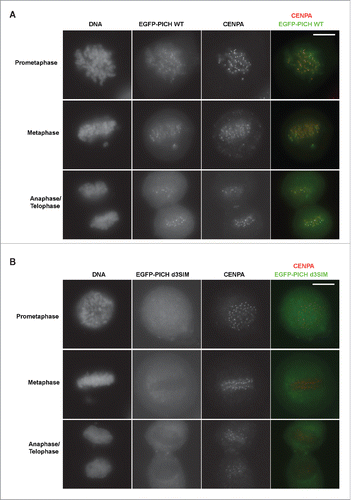
PICH SIM3 is more critical for centromere localization of PICH than SIM1&2
Although results from in vitro pull-down assays suggested that all SIMs on PICH contribute to effective SUMO binding, it is plausible that the outcome may be different for the in vivo centromeric PICH localization. To test this, we made individual SIM mutations in inducible PICH constructs (dSIM1, dSIM2 and dSIM3) and analyzed mitotic HeLa cells by immunostaining. Interestingly, PICH dSIM3 mutation by itself drastically reduced PICH's association with mitotic centromeres in contrast to the effects of dSIM1&2 combined (). Combinations of other SIM sequence mutants were also tested. Mutations in SIM1&3 and SIM2&3 were observed to reduce PICH's association with mitotic chromosomes when compared to dSIM1&2. (Figs. S2A, S2B, & 3B). We quantified the centromeric PICH signal of the mutants (d3SIM, dSIM3 and dSIM1&2) relative to PICH WT by normalizing with CENPA levels. Centromeres from different mitotic stages (prometaphase, metaphase and anaphase) were analyzed in each set. Regardless of the mitotic stage, the result indicated that the amount of centromere-associated PICH when SIM3 was mutated (d3SIM and dSIM3) was significantly lower than the amount of centromere-associated PICH when the other two SIMs were mutated (dSIM1&2) (). These observations shed light on the different role(s) of each SIM on PICH and suggest that SIM3 plays a critical role in the robust centromeric localization of PICH.
Figure 3. SIM3 is most critical for centromeric localization of PICH. (A) Mutation in SIM3 of PICH drastically reduces centromeric association of PICH. EGFP-tagged PICH dSIM3 transiently expressed and induced in HeLa Tet-ON cells. Different stages of mitotic cells isolated and EGFP-PICH dSIM3 visualized along with centromere marker, CENPA by immunofluorescence staining. White bar on the top right panel indicates 10 μm. (B) SIM1 and SIM2 of PICH does not affect centromere localization of PICH in HeLa Tet-ON cells. Similar to PICH dSIM3, PICH dSIM1&2 was ectopically induced and expressed in HeLa Tet-ON cells. PICH mutant was visualized by EGFP along with CENPA by immunostaining analysis. White bar on the top right panel indicates 10 μm. (C) PICH signals of the mutants (d3SIM, dSIM3, and dSIM1&2) at the centromeres were normalized to CENPA levels and quantified relative to PICH WT. Values represent the average of at least 30 different centromeres analyzed in at least 3 different cells in each set (n, n = 3). Error bars indicate standard deviation and statistical analysis by paired student t-test is indicated as **p < 0.01, ***p < 0.001.
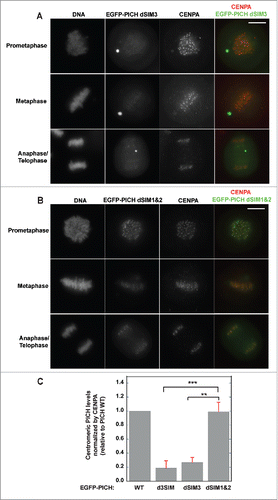
Robust centromeric localization of PICH is not essential to prevent chromatin bridges in anaphase
Depletion of PICH is known to cause chromosome segregation defects, such as the formation of chromatin bridges and micronuclei.Citation8,26 We wanted to test if SUMO-interaction of PICH is critical to prevent this chromatin bridge phenotype. To test this, we replaced endogenous PICH with mutant forms using PICH depletion with siRNA and expression of siRNA resistant EGFP-PICH. We confirmed the resistance of exogenous PICH to siRNA by immunoblotting analysis (Fig. S3A). For measuring chromatin bridges, we established stable inducible EGFP-PICH cell lines with HeLa Tet-ON cells that express different mutant. Expression of EGFP-PICH WT and mutants in stable cell lines was confirmed by immunoblotting analysis (Fig. S3B). Three independent clones were isolated from each mutant. Isolated cell lines were synchronized as shown in with endogenous PICH-replacement by siRNA followed by tetracycline induction. Anaphase cells were enriched for the analysis of chromatin bridge phenotype by mitotic shake off and cells with chromatin bridges were counted as shown in . Anaphase cells with chromatin bridges and the total number of anaphase cells in a set were counted to calculate the percentage of anaphase cells with chromatin bridges in each sample. Cells expressing EGFP-PICH WT with endogenous PICH depleted were able to rescue the chromatin bridge phenotype in comparison to cells expressing just EGFP. A PICH mutant with loss of ATPase activity (PICH K128A) was included as a negative control because it is known to not rescue the chromatin bridge phenotypeCitation8 (). Interestingly, PICH dSIM3 mutant that was not robustly associated to centromere/KT region was able to rescue the chromatin bridge phenotype, similar to PICH WT. More interestingly, PICH dSIM1&2 mutant that did not show defective localization at the centromere/KT region was unable to rescue the bridge phenotype. All clones isolated for each mutant showed a consistent phenotype. The results suggest that SIM1&2 functions differently from SIM3, and SIM1&2 is required for PICH activity at mitotic centromeres to resolve chromatin bridges. Taken together, we propose that PICH SIMs have two separate functions in the regulation of PICH at mitotic centromeres (). SIM3 is important for stable PICH localization at the centromere whereas PICH SIM1&2 is most likely required for the activity of PICH at centromeres for resolving chromatin bridges. We expect this difference to be due to distinct interacting proteins for each SIM, which most likely are SUMOylated on mitotic chromosomes.
Figure 4. PICH's SIMs role in localization is different from its role in chromatin bridge resolution. (A) Schematic representation of the synchronization method used in the chromatin bridge analysis with thymidine (Thy), doxycycline (Dox), and nocodazole (Noc) addition. (B) Robust defective centromere localization of PICH dSIM3 does not result in chromatin bridge phenotype whereas mutations in SIM1&2 (PICH dSIM1&2) causes chromatin bridge phenotype. Stable HeLa Tet-ON cell lines expressing different PICH constructs were made. Endogenous PICH was depleted by siRNA and nocodazole-arrested cells were released to analyze for anaphase cells. Representative images of different PICH constructs cell lines are shown. White bar on the top right panel indicates 10 μm. (C) Percentage of anaphase cells with chromatin bridges per total anaphase cells were counted and graphed. At least 30 anaphase cells to a maximum of 360 anaphase cells were counted in each experiment (n = 3). Error bars indicate standard deviation and statistical analysis by paired student t-test is indicated as *p < 0.05, **p < 0.005.
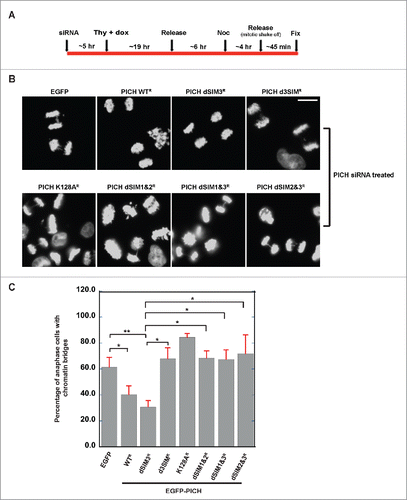
Figure 5. Schematic representation of PICH with proposed functions of SIMs. Proposed functions of the different SIMs on PICH and remaining questions to be answered about each SIM. SIM3 is critical for centromeric localization of PICH whereas SIM1&2 is critical to for PICH's function in the resolution of chromatin bridges during mitosis through binding to distinct SUMOylated chromosomal proteins.
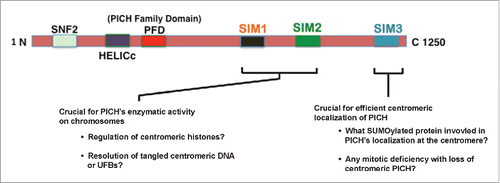
Discussion
PICH was found to be associated with centromere/KT region and UFBs during mitosis.Citation5 Multiple studies since PICH's discovery have identified different factors that are critical to prevent chromosomal arm localization of PICH including the Plk1-dependent phosphorylationCitation5 and the ATPase activity of PICH.Citation8 However, the precise molecular mechanism for how PICH localizes to the centromere/KT region is not known. Our results implicate a novel mechanism of centromeric localization of PICH via SIMs (). This localization could be due to PICH's direct interaction with a SUMOylated centromeric protein. Histone SUMOylation, including CENPA SUMOylation, at the heterochromatin loci has been shown previously.Citation28,29 PICH could potentially be interacting with SUMOylated CENPA at the centromere. Additionally, DNA topoisomerase IIα (TopoIIα) is known to be SUMOylated at the centromereCitation30,31 and multiple studies have linked TopoIIα's function with PICH.Citation9,32,33 It is plausible that TopoIIα SUMOylation assists in the recruitment and retention of PICH at the centromere.
Interestingly, our results also suggest that SIM3 is more important for centromeric recruitment and retention whereas SIM1 and SIM2 are critical to resolve chromatin bridges during anaphase (). This difference could be because different SUMOylated proteins interact with different SIMs. While SIM3 could be interacting with SUMOylated histones or TopoIIα at the centromere as discussed above, SIM1 and SIM2 could be interacting with a different SUMOylated protein to resolve chromatin bridges. One candidate SUMOylated protein is the DNA helicase BLM mutated in Bloom's syndrome,Citation34 and inhibition of BLM has been previously shown to cause chromatin bridges.Citation26,35 Additionally, PICH is a SUMO substrate itselfCitation10 and it may be possible that SUMOylation can cause conformational changes through intra-molecular interaction between SUMOylated PICH and SIM1&2 (). Although we propose that PICH interaction to SUMOylated proteins through its SIMs play a critical role in our findings, it is plausible that the centromeric localization defect and the enzymatic activity defect of PICH in chromatin bridge resolution is caused by SIM mutations independent from SUMO-PICH SIM interaction. Further studies to identify specific SUMOylated binding proteins of PICH to SIM3 that mediate centromeric localization and to SIM1&2 for its activity, will provide further insight into the molecular mechanism controlling PICH-localization and function at the centromere.
Materials and methods
DNA constructs, cell transfection, and antibodies
PICH cDNA was obtained from pEGFP PICH construct from Addgene (plasmid 41163: Nigg CB62) and cloned into pTGFC70 plasmid for mRNA expression in XEE as previously described using AclI and SpeI restriction sites.Citation10,36 PICH N- and C-terminus truncation mutants (PICH 1–733 a.a., PICH 1–414 a.a. and PICH 616–1250 a.a.) were amplified and cloned into pTGFC70 as the full length PICH. EGFP-PICH was cloned into pTRE3G Tet-ON vector (Clonetech Laboratories, Mountain view, CA) using SalI and EagI restriction sites. PICH SIM mutants were generated using the QuikChange II XL Site-Directed Mutagenesis kit from Agilent Technologies (Santa Clara, CA). PICH siRNA target sequence was purchased from Life Technologies (Carlsbad, CA) (5′-GCUUGCUACUUUAUCGAGATT-3′) and cells were transfected with 40 pmols of siRNA using Lipofectamine RNAiMAX (Invitrogen, Carlsbad, CA). PICH RNAi resistant mutant was generated by introducing 4 silent mutations in PICH DNA that disrupt siRNA binding using Quikchange II XL Site-Directed Mutagenesis kit (Agilent Technologies, Santa Clara, CA). HeLa Tet-ON cells were grown in McCoy's medium (Mediatech Inc., Manassas, VA) supplemented with 10% FBS and transfected using Clonetech's Xfect protein transfection reagent as per manufacturer's instructions. PICH WT and mutant expression was induced by the addition of doxycycline (100 ng/ml) for 10–12 hours for transient transfection.
The following antibodies were used in this study. For immunoblotting, antibodies used were mouse monoclonal anti-GFP (JL-8) (Clonetech; 1:1000), S-protein-HRP (EMD Millipore, Billerica, MA; 1:1000), and mouse monoclonal anti-β-tubulin (Sigma-Aldrich; 1:1000). For immunofluorescence analysis, antibodies used were mouse anti-CENPA (MBL, Japan; 1:200), and goat anti-mouse Alexa 568 (Life Technologies, Carlsbad, CA; 1:1000)
Recombinant protein purification and in vitro SUMOylation assay
Recombinant human PICH cDNA was cloned into yeast protein expression vector pPIC3.5 that had T7 and calmodulin-binding protein (CBP) tags. PICH expressed in GS115 strain of Pichia pastoris was extracted using the following buffer (150 mM NaCl, 2 mM CaCl2, 1 mM MgCl2, 50 mM Tris (pH 7.8), 5% glycerol) and affinity purified by calmodulin sepharose (GE healthcare) as previously described.Citation37 PICH mutant proteins used in this study were cloned and purified the same way as WT. Recombinant Xenopus laevis PARP1 (1–650 a.a.) used for in vitro pull-down assay was expressed at 15°C in the presence of 2.5% ethanol and 0.1 μM ZnCl2 and affinity purified using Talon cobalt affinity resin (Clonetech) followed by SP-Sepharose column chromatography (GE healthcare) as previously described.Citation37 Recombinant proteins used for SUMOylation assay: E1 complex (Aos1 and Uba2) subcloned into pRSF Duet vector with His-6 tag, Ubc9 subcloned into pT7-7, SUMO2-GG subcloned into pGEX4T-1 with a cleavable N-terminal GST tag, PIASy and SENP2 catalytic domain (SENP2 CD) (363–589 a.a.) subcloned into pET28a vectors with a N-terminal His-6 tag. All proteins were expressed either at 15°C (E1 complex, PIASy and SENP2 CD) or 30°C (Ubc9 and SUMO2-GG). His-6 tagged proteins (PIASy and SENP2 CD) were purified using Cobalt affinity beads (Clonetech) followed by ion-exchange chromatography. Ubc9 was purified by passing the soluble fraction onto anion exchange column followed by cation exchange column and sephacryl S-100 gel filtration. E1 complex was purified using cobalt affinity beads (Clonetech) and elutions were loaded onto a NHS-sepharose conjugated GST-SUMO1-GG affinity column in the presence of ATP. Bound E1 complex was eluted by 10 mM DTT and further purified by anion exchange column. GST-SUMO2-GG was purified using Glutathione-sepharose beads (GE healthcare) and the GST-tag was cleaved with thrombin. Untagged SUMO2-GG was purified by anion exchange chromatography followed by sephacryl S-100 gel filtration. All proteins were concentrated and stored by snap freeze with liquid nitrogen in storage buffer (100 mM NaCl, 1 mM MgCl2, 20 mM HEPES (pH 7.8), 5% glycerol, and 0.5 mM TCEP).
In vitro SUMOylation assay was performed with 40 nM E1, 40 nM PIASy, 80 nM Ubc9, 3 μM PARP1 (1–650 a.a.) and 30 μM SUMO2-GG in SUMOylation buffer (20 mM HEPES (pH 7.8), 5% glycerol, 100 mM NaCl, 0.05% Tween 20 and 5 mM MgCl2) for 2 hours at 25°C.
Construction of a DNA triplex and translocation assay
Construction of DNA triplex and translocation assay were performed as described previously, with a few modifications.Citation25 Two oligonucleotides with sequences (5′-CGCAAGAAAAGAAAGAAGAAAGAAACCGAGCT-3′ and 5′-CGGTTTCTTTCTTCTTTCTTTTCTTGCGGTAC-3′) were annealed and the duplex was cloned into pBluescript KS+ vector. A 400 bp fragment containing the duplex sequence was PCR amplified. Triplex forming oligonucleotide (10 pmol) (5′- TTCTTTTCTTTCTTCTTTCTTT-3′) was end-labeled with Alexa 488, and was then annealed to an equimolar amount of the 400 bp fragment in buffer containing 10 mM MgCl2, 50 mM NaCl and 40 mM MES, pH 5.5. The reaction was placed on a heat block (80°C) for 2 minutes and allowed to cool to room temperature overnight.
For the translocation assay, 25 nM of the triplex substrate and 0.5 μM or 3 μM PICH were used per reaction. Reactions were performed at room temperature for 25 min or specified time in buffer containing 20 mM Tris-HCL, pH 7.0, 100 mM NaCl, 2 mM MgCl2, 1 mM DTT, 2 mM ATP and 0.1 mg/ml BSA. Reactions were stopped by the stop buffer containing 0.5% SDS, 0.04 mg/ml proteinase K, 10% glycerol, and 20 mM MES, pH 5.5 and incubated at 37°C for 15 min. The reactions were loaded onto a 10% native polyacrylamide gel and ran at 100V for 2 hours in pre-chilled TAM buffer (40 mM Tris/HAc, pH 5.5, 1 mM MgCl2). The gel was imaged using a Typhoon Imager (GE Healthcare).
Pull-down assays
Pull-down (PD) assays were performed as previously described.Citation10 GFP-tagged PICH (WT, mutants or truncations) mRNA was generated using mMESSAGE mMACHINE SP6 transcription kit (Life Technologies, Carlsbad, CA) and was expressed in Xenopus egg extract for pull-down assay.Citation36 Non-SUMOylated and SUMOylated bait (Xenopus laevis PARP1 (1–650 a.a.) fused to S-tag) were bound to S-protein-agarose beads (EMD Millipore, Billerica, MA). XEE was first diluted 3 times with the PD buffer (20 mM NaPi, pH 7.8, 18 mM β-glycerol phosphate, pH 7.5, 5 mM MgCl2, 100 mM NaCl, 1 mM CaCl2, and 10 μM ZnCl2) and clarified by centrifugation at 25,000 x g for 30 min at 4°C. The soluble fraction was diluted with equal volume of PD buffer supplemented with 0.2% Tween 20 and 0.2% Triton X-100 followed by centrifugation. Diluted and clarified XEE was incubated with bait bound S-protein beads for an hour at room temperature. Bound proteins samples were digested by SENP2-CD (50 ng/μl) for 30 min at room temperature and resolved by SDS-PAGE on 8–16% gradient gels (Life Technologies, Carlsbad, CA) for immunoblotting analysis.
Immunofluorescence analysis
Transfected cells were fixed with 4% paraformaldehyde and permeabilized using 0.2% Triton-X 100. Cells were blocked in 5% BSA and 2.5% fish gelatin in PBS-T (1X PBS with 0.1% tween 20) for 30 minutes. Cells were stained with primary antibody (1:200 dilution in blocking buffer) followed by Alexa dye-tagged secondary antibody (1:1000) for an hour each at room temperature. DNA was stained with Hoechst 33342 (EMD Millipore) and the cells were mounted using Vectashield mounting medium (Vector laboratories, Burlingame, CA). The specimens were analyzed using a Nikon TE2000-U microscope with a Plan Apo 100X/1.40 objective, and the images were taken with a Retiga SRV CCD camera (QImaging) using the Volocity imaging software (Improvision) at 100X and 60X, room temperature. Obtained images were processed to intensities according to JCB policy using Photoshop CS6 and Illustrator software (Adobe). Quantification of the PICH signal was through Adobe Photoshop CS6 software. Intensity around PICH was measured and was normalized with CENPA signal. Average of the PICH intensities from at least 30 centromeres from different mitotic stages cells (prometaphase, metaphase and anaphase) per set were calculated. The average and standard deviation of 3 sets was determined for each PICH construct. Graph and paired student t-test were plotted using KaleidaGraph software.
Stable cell line generation and synchronization for chromatin bridge analysis
Inducible siRNA-resistant EGFP-PICH DNA constructs were co-transfected into HeLa Tet-ON cells along with a linear selection marker, hygromycin resistance using Clonetech's Xfect transfection reagent as per manufacturer's instructions. Stable clones were isolated using cloning cylinders and screened for expression. PICH in stable cell lines was induced by 500 ng/ml doxycycline for 24 hours. HeLa Tet-ON cells stably expressing different PICH constructs were treated with PICH siRNA (40 pmols). Five hours later, cells were synchronized with 2 mM thymidine. 500 ng/ml doxycycline was added along with the thymidine. Approximately 19 hours later, cells were released from thymidine into fresh media with doxycycline. Six hours post release from thymidine, 100 ng/ml nocodazole added to cells. Four hours after nocodazole addition, cells collected by mitotic shake off and released for 45 minutes before fixation. Anaphase cells with chromatin bridges were counted manually. The average and standard deviation from 3 independent experiments was determined. Graph and paired student t-test were plotted with KaleidaGraph software.
Abbreviations
dnUbc9 | = | dominant-negative Ubc9 |
PARP1 | = | Poly-ADP ribose polymerase 1 |
PICH | = | Polo-like kinase 1 interacting checkpoint helicase 1 |
SENP2 CD | = | sentrin-specific protease 2 catalytic domain |
SIM | = | SUMO-interacting motif |
SUMO | = | small ubiquitin-like modifier |
Topo IIα | = | topoisomerase IIα |
UFB | = | ultrafine DNA bridge |
XEE | = | Xenopus egg extract |
Disclosure of potential conflicts of interest
The authors declare no competing financial interests.
1191713_Supplemental_Material.zip
Download Zip (6.4 MB)Acknowledgments
We thank H. Funabiki of Rockefeller University for sharing the pTGFC70 plasmid. We also thank M.Yoshida of University of Kansas for critical reading of the manuscript and comments on this project.
Funding
This project was supported by Strategic Initiative Grant (#INS0073115) and in part by a general research fund (#2301743) from the University of Kansas.
References
- Nigg EA. Mitotic kinases as regulators of cell division and its checkpoints. Nat Rev Mol Cell Biol 2001; 2:21-32; PMID:11413462; http://dx.doi.org/10.1038/35048096
- Barr FA, Elliott PR, Gruneberg U. Protein phosphatases and the regulation of mitosis. J Cell Sci 2011; 124:2323-34; PMID:21709074; http://dx.doi.org/10.1242/jcs.087106
- Wrighton KH. Mitosis: microtubules protect spindle assembly factors. Nat Rev Mol Cell Biol 2014; 15:150-1; PMID:24518368
- Krebs JE, Fry CJ, Samuels ML, Peterson CL. Global role for chromatin remodeling enzymes in mitotic gene expression. Cell 2000; 102:587-98; PMID:11007477; http://dx.doi.org/10.1016/S0092-8674(00)00081-7
- Baumann C, Korner R, Hofmann K, Nigg EA. PICH, a centromere-associated SNF2 family ATPase, is regulated by Plk1 and required for the spindle checkpoint. Cell 2007; 128:101-14; PMID:17218258; http://dx.doi.org/10.1016/j.cell.2006.11.041
- Chan KL, North PS, Hickson ID. BLM is required for faithful chromosome segregation and its localization defines a class of ultrafine anaphase bridges. EMBO J 2007; 26:3397-409; PMID:17599064; http://dx.doi.org/10.1038/sj.emboj.7601777
- Leng M, Bessuso D, Jung SY, Wang Y, Qin J. Targeting Plk1 to chromosome arms and regulating chromosome compaction by the PICH ATPase. Cell Cycle 2008; 7:1480-9; PMID:18418076; http://dx.doi.org/10.4161/cc.7.10.5951
- Kaulich M, Cubizolles F, Nigg EA. On the regulation, function, and localization of the DNA-dependent ATPase PICH. Chromosoma 2012; 121:395-408; PMID:22527115; http://dx.doi.org/10.1007/s00412-012-0370-0
- Nielsen CF, Huttner D, Bizard AH, Hirano S, Li TN, Palmai-Pallag T, Bjerregaard VA, Liu Y, Nigg EA, Wang LH, et al. PICH promotes sister chromatid disjunction and co-operates with topoisomerase II in mitosis. Nat Commun 2015; 6:8962; PMID:26643143; http://dx.doi.org/10.1038/ncomms9962
- Sridharan V, Park H, Ryu H, Azuma Y. SUMOylation regulates polo-like kinase 1-interacting checkpoint helicase (PICH) during mitosis. J Biol Chem 2015; 290:3269-76; PMID:25564610; http://dx.doi.org/10.1074/jbc.C114.601906
- Hong Y, Rogers R, Matunis MJ, Mayhew CN, Goodson ML, Park-Sarge OK, Sarge KD. Regulation of heat shock transcription factor 1 by stress-induced SUMO-1 modification. J Biol Chem 2001; 276:40263-7; PMID:11514557; http://dx.doi.org/10.1074/jbc.M104714200
- Matunis MJ, Wu J, Blobel G. SUMO-1 modification and its role in targeting the Ran GTPase-activating protein, RanGAP1, to the nuclear pore complex. J Cell Biol 1998; 140:499-509; PMID:9456312; http://dx.doi.org/10.1083/jcb.140.3.499
- Hardeland U, Steinacher R, Jiricny J, Schar P. Modification of the human thymine-DNA glycosylase by ubiquitin-like proteins facilitates enzymatic turnover. EMBO J 2002; 21:1456-64; PMID:11889051; http://dx.doi.org/10.1093/emboj/21.6.1456
- Kerscher O. SUMO junction-what's your function? New insights through SUMO-interacting motifs. EMBO Rep 2007; 8:550-5; PMID:17545995; http://dx.doi.org/10.1038/sj.embor.7400980
- Minty A, Dumont X, Kaghad M, Caput D. Covalent modification of p73alpha by SUMO-1. Two-hybrid screening with p73 identifies novel SUMO-1-interacting proteins and a SUMO-1 interaction motif. J Biol Chem 2000; 275:36316-23; PMID:10961991; http://dx.doi.org/10.1074/jbc.M004293200
- Song J, Durrin LK, Wilkinson TA, Krontiris TG, Chen Y. Identification of a SUMO-binding motif that recognizes SUMO-modified proteins. Proc Natl Acad Sci U S A 2004; 101:14373-8; PMID:15388847; http://dx.doi.org/10.1073/pnas.0403498101
- Hoege C, Pfander B, Moldovan GL, Pyrowolakis G, Jentsch S. RAD6-dependent DNA repair is linked to modification of PCNA by ubiquitin and SUMO. Nature 2002; 419:135-41; PMID:12226657; http://dx.doi.org/10.1038/nature00991
- Shen TH, Lin HK, Scaglioni PP, Yung TM, Pandolfi PP. The mechanisms of PML-nuclear body formation. Mol Cell 2006; 24:331-9; PMID:17081985; http://dx.doi.org/10.1016/j.molcel.2006.09.013
- Ryu H, Yoshida MM, Sridharan V, Kumagai A, Dunphy WG, Dasso M, Azuma Y. SUMOylation of the C-terminal domain of DNA topoisomerase IIalpha regulates the centromeric localization of Claspin. Cell Cycle 2015; 14:2777-84; PMID:26131587; http://dx.doi.org/10.1080/15384101.2015.1066537
- Wan J, Subramonian D, Zhang XD. SUMOylation in control of accurate chromosome segregation during mitosis. Curr Protein Pept Sci 2012; 13:467-81; PMID:22812528; http://dx.doi.org/10.2174/138920312802430563
- Cubenas-Potts C, Goeres JD, Matunis MJ. SENP1 and SENP2 affect spatial and temporal control of sumoylation in mitosis. Molecular biology of the cell 2013; 24:3483-95; PMID:24048451; http://dx.doi.org/10.1091/mbc.E13-05-0230
- Zhang XD, Goeres J, Zhang H, Yen TJ, Porter AC, Matunis MJ. SUMO-2/3 modification and binding regulate the association of CENP-E with kinetochores and progression through mitosis. Molecular cell 2008; 29:729-41; PMID:18374647; http://dx.doi.org/10.1016/j.molcel.2008.01.013
- Diaz-Martinez LA, Gimenez-Abian JF, Azuma Y, Guacci V, Gimenez-Martin G, Lanier LM, Clarke DJ. PIASgamma is required for faithful chromosome segregation in human cells. PLoS One 2006; 1:e53; PMID:17183683; http://dx.doi.org/10.1371/journal.pone.0000053
- Zhao Q, Xie Y, Zheng Y, Jiang S, Liu W, Mu W, Liu Z, Zhao Y, Xue Y, Ren J. GPS-SUMO: a tool for the prediction of sumoylation sites and SUMO-interaction motifs. Nucleic Acids Res 2014; 42:W325-30; PMID:24880689; http://dx.doi.org/10.1093/nar/gku383
- Biebricher A, Hirano S, Enzlin JH, Wiechens N, Streicher WW, Huttner D, Wang LH, Nigg EA, Owen-Hughes T, Liu Y, et al. PICH: a DNA translocase specially adapted for processing anaphase bridge DNA. Mol Cell 2013; 51:691-701; PMID:23973328; http://dx.doi.org/10.1016/j.molcel.2013.07.016
- Ke Y, Huh JW, Warrington R, Li B, Wu N, Leng M, Zhang J, Ball HL, Li B, Yu H. PICH and BLM limit histone association with anaphase centromeric DNA threads and promote their resolution. EMBO J 2011; 30:3309-21; PMID:21743438; http://dx.doi.org/10.1038/emboj.2011.226
- Hubner NC, Wang LH, Kaulich M, Descombes P, Poser I, Nigg EA. Re-examination of siRNA specificity questions role of PICH and Tao1 in the spindle checkpoint and identifies Mad2 as a sensitive target for small RNAs. Chromosoma 2010; 119:149-65; PMID:19904549; http://dx.doi.org/10.1007/s00412-009-0244-2
- Nathan D, Ingvarsdottir K, Sterner DE, Bylebyl GR, Dokmanovic M, Dorsey JA, Whelan KA, Krsmanovic M, Lane WS, Meluh PB, et al. Histone sumoylation is a negative regulator in Saccharomyces cerevisiae and shows dynamic interplay with positive-acting histone modifications. Genes Dev 2006; 20:966-76; PMID:16598039; http://dx.doi.org/10.1101/gad.1404206
- Merai Z, Chumak N, Garcia-Aguilar M, Hsieh TF, Nishimura T, Schoft VK, Bindics J, Slusarz L, Arnoux S, Opravil S, et al. The AAA-ATPase molecular chaperone Cdc48/p97 disassembles sumoylated centromeres, decondenses heterochromatin, and activates ribosomal RNA genes. Proc Natl Acad Sci U S A 2014; 111:16166-71; PMID:25344531; http://dx.doi.org/10.1073/pnas.1418564111
- Azuma Y, Arnaoutov A, Dasso M. SUMO-2/3 regulates topoisomerase II in mitosis. J Cell Biol 2003; 163:477-87; PMID:14597774; http://dx.doi.org/10.1083/jcb.200304088
- Bachant J, Alcasabas A, Blat Y, Kleckner N, Elledge SJ. The SUMO-1 isopeptidase Smt4 is linked to centromeric cohesion through SUMO-1 modification of DNA topoisomerase II. Mol Cell 2002; 9:1169-82; PMID:12086615; http://dx.doi.org/10.1016/S1097-2765(02)00543-9
- Spence JM, Phua HH, Mills W, Carpenter AJ, Porter AC, Farr CJ. Depletion of topoisomerase IIalpha leads to shortening of the metaphase interkinetochore distance and abnormal persistence of PICH-coated anaphase threads. J Cell Sci 2007; 120:3952-64; PMID:17956945; http://dx.doi.org/10.1242/jcs.013730
- Wang LH, Schwarzbraun T, Speicher MR, Nigg EA. Persistence of DNA threads in human anaphase cells suggests late completion of sister chromatid decatenation. Chromosoma 2008; 117:123-35; PMID:17989990; http://dx.doi.org/10.1007/s00412-007-0131-7
- Eladad S, Ye TZ, Hu P, Leversha M, Beresten S, Matunis MJ, Ellis NA. Intra-nuclear trafficking of the BLM helicase to DNA damage-induced foci is regulated by SUMO modification. Hum Mol Genet 2005; 14:1351-65; PMID:15829507; http://dx.doi.org/10.1093/hmg/ddi145
- Rouzeau S, Cordelieres FP, Buhagiar-Labarchede G, Hurbain I, Onclercq-Delic R, Gemble S, Magnaghi-Jaulin L, Jaulin C, Amor-Gueret M. Bloom's syndrome and PICH helicases cooperate with topoisomerase IIalpha in centromere disjunction before anaphase. PLoS One 2012; 7:e33905; PMID:22563370; http://dx.doi.org/10.1371/journal.pone.0033905
- Kelly AE, Ghenoiu C, Xue JZ, Zierhut C, Kimura H, Funabiki H. Survivin reads phosphorylated histone H3 threonine 3 to activate the mitotic kinase Aurora B. Science 2010; 330:235-9; PMID:20705815; http://dx.doi.org/10.1126/science.1189505
- Ryu H, Furuta M, Kirkpatrick D, Gygi SP, Azuma Y. PIASy-dependent SUMOylation regulates DNA topoisomerase IIalpha activity. J Cell Biol 2010; 191:783-94; PMID:21079245; http://dx.doi.org/10.1083/jcb.201004033