ABSTRACT
Kaposi's sarcoma (KS) is a highly angiogenic and inflammatory neoplasia. The angiogenic and inflammatory cytokine angiopoietin-2 (Ang-2) is strongly expressed in KS due to Kaposi's sarcoma-associated herpesvirus (KSHV) infection. In the present study, we determined how Ang-2 contributes to development of KS by using telomerase-immortalized human umbilical vein endothelial cells (TIVE) as a model, which become malignantly transformed and express increased levels of Ang-2 following KSHV infection. Ang-2 released from TIVE-KSHV cells induces tyrosine phosphorylation of Tie-2 receptor from both human and mouse endothelial cells and promotes angiogenesis in nude mice. Functional inhibition or expressional “knock-down” of Ang-2 in these cells blocks angiogenesis and inhibits tumor growth. Ang-2 suppression also reduces the numbers of infiltrating monocytes/macrophages in tumors. In transwell-based cell migration assays, Ang-2 indeed enhances migration of human monocytes in a dose-dependent manner. These results underscore a pivotal role of KSHV-induced Ang-2 in KS tumor development by promoting both angiogenesis and inflammation. Our data also suggest that selective drug targeting of Ang-2 may be used for treatment of KS.
Introduction
Kaposi's sarcoma (KS) is a vascular malignancy of endothelial cell origin that is etiologically associated with Kaposi's sarcoma-associated herpesvirus (KSHV).Citation1 Hallmarks of KS include “spindle-shaped” tumor cells that express KSHV latent nuclear antigen (LANA), extensive “slit-like” blood vessels, and large amounts of infiltrating inflammatory cells.Citation2,3 It has been suggested that KSHV deregulation of angiogenesis and inflammation plays a key role in the development of KS.Citation3
KSHV infection induces a variety of angiogenic and inflammatory cytokines and chemokines such as VEGF, b-FGF, IL-6, IL-8, and Gro-α, etc.Citation4-10 The virus also encodes several virokines, which are homologues of human cytokines and play important roles in KS tumor development as well.Citation11 While all KSHV-induced cytokines and virokines likely contribute to development of KS, some of these factors may be essential and thus are the preferred targets for drug inhibition and treatment of KS.
The endothelial cell specific tyrosine kinase receptor Tie-2 plays an essential role in both pre-natal vasculature development and post-natal angiogenesis.Citation12-15 Angiopoietins are ligands of Tie-2 receptor. Angiopoietin-1 (Ang-1), an agonist of Tie-2 that induces tyrosine phosphorylation of its intracellular kinase domain to trigger downstream signaling, plays an essential role in the maturation and stabilization of newly formed blood vessels during late stage angiogenesis.Citation16 Ang-1 “knock-out” in mice is embryonically lethal due to a vascular defect with unsealed blood vessels.Citation12 Under normal conditions, Ang-1 is constitutively expressed at relatively high levels to promote maturation and stabilization of blood vessels. In contrast, Angiopoietin-2 (Ang-2) is an antagonist of Tie-2 and destabilizes existing blood vessels for the generation of new blood vessels.Citation17 Ang-2 deficient mice die soon after birth due to failure in angiogenesis.Citation17 Ang-2 expression is highly restricted under normal conditions but is rapidly induced in response to wound healing or tumor development. As an antagonist, Ang-2 inhibits Ang-1 induction of tyrosine phosphorylation of Tie-2, and in the absence of Ang-1, Ang-2 can also induce tyrosine phosphorylation of Tie-2.Citation18 In the context of tumor Ang-2 actually acts as an agonist of Tie-2.Citation19 Interestingly, Ang-2 “knockout” mice also display a defect in inflammatory response against bacterial infection, and multiple studies have shown that Ang-2 is indeed an inflammatory cytokine.Citation20,21
Ang-2 is highly expressed by various tumor cells. In cancer patients, the levels of Ang-1 decrease while the levels of Ang-2 increase significantly, and higher levels of Ang-2 are associated with poor prognosis of cancers.Citation22,23 To selectively target Ang-2 for cancer treatment, Amgen, Inc. developed two Ang-2 inhibitors named AMG-386 and L1-10, which are small peptides that bind with high affinity to Ang-1 or Ang-2 to prevent their interactions with Tie-2.Citation24 AMG-386 has an affinity for both Ang-1 and Ang-2 while L1-10 only binds to Ang-2. Both AMG-386 and L1-10 inhibit tumor growth by effectively blocking tumor angiogenesis.Citation24-28
Ang-2, but not Ang-1, is strongly expressed in KS,Citation29 which may be attributable to KSHV infection. Indeed, previous studies showed that KSHV acute infection of primary endothelial cells not only up-regulates expression of Ang-2 but also induces its rapid release.Citation30-32 In the present study, we investigated how Ang-2 contributes to KS tumor development by using telomerase-immortalized human umbilical vein endothelial cells (TIVE), which become malignantly transformed upon KSHV infectionCitation33 and strongly express Ang-2. We demonstrated that suppression of KSHV-induced Ang-2 not only inhibits angiogenesis and tumor growth in nude mice but also reduces the numbers of infiltrating monocytes/macrophages. To our knowledge, this is the first study to report a pivotal role of Ang-2 in KS tumor development and the effectiveness of Ang-2 inhibitors for the treatment of KS in a mouse model.
Results
Ang-2 is strongly expressed in KSHV infected TIVE-KSHV cells
TIVE cells have been proven to be a good cell model for KSHV infection and KS tumor development.Citation33 A cell line, TIVE-KSHV, was generated after acute KSHV infection of TIVE cells and long-term culture. These cells stably maintain the viral genomes and display characteristics of malignant transformation as they grow “KS-like” tumors in nude mice.Citation33 Like most KS tumor cells,Citation2,3 the majority of TIVE-KSHV cells have latent KSHV infection, which can be induced for lytic replication upon stimulation with 12-O-Tetradecanoylphorbol-13-acetate (TPA).Citation33 We re-infected these cells with the recombinant KSHV, BAC16,Citation34 resulting in TIVE-KSHV cells that not only express the viral latent protein LANA but also the GFP marker (). By immunofluorescence antibody (IFA) staining, we found 1.3% and 9.6% of TIVE-KSHV cells expressing KSHV lytic protein ORF59 before and after 48 hours stimulation with TPA, respectively (). By Western blot analysis, ORF59 protein is hardly detectable before TPA treatment and a weak protein band is detected by the anti-ORF59 antibody at 48 hours post TPA stimulation (). These data are consistent with the previous report that most TIVE-KSHV cells have latent KSHV infection under normal conditions, which can be induced to undergo lytic replication with TPA stimulation.Citation33
Figure 1. Ang-2 is strongly expressed in TIVE-KSHV cells. A, B, and C, IFA staining of TIVE and TIVE-KSHV cells that were re-infected with the recombinant KSHV BAC16 with a rat monoclonal antibody to KSHV latent protein LANA (A), a monoclonal antibody to KSHV lytic protein ORF59 (B), and a rabbit antibody to Ang-2 (C), which were revealed with an Alexa Fluor®-594 conjugated goat anti-rat secondary antibody, an Alexa Fluor®-647 conjugated goat anti-mouse secondary antibody, and an Alexa Fluor®-633 conjugated goat anti-rabbit secondary antibody respectively. Rat, mouse, or rabbit IgG was used as negative controls, and DAPI was used for nuclear staining of all cells. Expression of LANA, Ang-2, and GFP was imaged and analyzed under a confocal microscope with a 40× oil objective. D, Western blot detection of Ang-1, Ang-2, Tie-2, LANA, and β-tubulin in cell lysates or supernatants from TIVE and TIVE-KSHV cells. Recombinant Ang-1 (rAng-1) and Ang-2 (rAng-2) were used as references. E, Ang-2 concentrations in the supernatants of TIVE or TIVE-KSHV cells, which were measured by ELISA. F, Western blot detection of ORF59, LANA, Ang-2, and β-tubulin in cell lysates of TIVE-KSHV cells before and after TPA treatment for 48 hours. G, average percentage of ORF59-positive cells from 16 microscopic fields before and after TPA treatment for 48 hours.
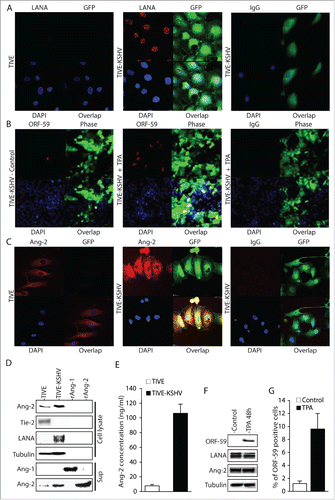
We next examined Ang-2 expression in TIVE-KSHV cells. In healthy endothelial cells, low level of pre-made Ang-2 protein is stored in the Weibel-Palade body in the cytoplasm of endothelial cells and is rapidly released upon stimulation.Citation32,35 As shown in , TIVE cells express low level of Ang-2. In contrast, TIVE-KSHV cells express significantly higher levels of Ang-2. Detectable levels of Ang-2 are also present in the culture supernatant from TIVE-KSHV cells (). By ELISA, we measured 106.4 ng/ml and 7.4 ng/ml Ang-2 in the supernatants of TIVE-KSHV and TIVE cells respectively (Figure E). These results suggest that TIVE-KSHV cells not only express high levels of Ang-2 but also secrete this angiogenic and inflammatory cytokine.
In contrast, we could not detect Ang-1 mRNA from TIVE nor TIVE-KSHV cells (data not shown). As shown in , no Ang-1 protein is detected in the culture supernatants, suggesting that these cells do not express Ang-1. In addition, Tie-2 protein is detected in TIVE cells but not in TIVE-KSHV cells (), indicating that the latter have lost Tie-2 expression and the ability to form blood vessels upon transformation into malignant tumor cells.
Ang-2 from TIVE-KSHV cells interacts with Tie-2 from both human and mouse endothelial cells
We first attempted to determine the functionality of Ang-2 from TIVE-KSHV cells by conducting Matrigel-based in vivo angiogenesis assays in nude mice, with the expectation that Ang-2 from TIVE-KSHV cells can act on mouse endothelial cells in vivo to promote angiogenesis. Human Ang-2 and its mouse counterpart are highly homologous, and multiple studies have demonstrated that Ang-2 from human tumor cells enhances angiogenesis in mice.Citation19,36 To confirm that human Ang-2 acts on mouse endothelial cells through Ang-2/Tie-2 signaling, we cultured human umbilical vein endothelial cells (HUVECs) and mouse dermal microvascular endothelial cells (MDMVECs) in their corresponding basic medium without growth factors and with 0.5% FBS for 12 hours, followed by stimulation of the cells with recombinant human Ang-2 for 30 minutes at 37°C and subsequent protein extraction and Western blot analysis. As shown in , the recombinant human Ang-2 induces tyrosine phosphorylation of Tie-2 from both HUVECs and MDMVECs.
Figure 2. Ang-2 from TIVE-KSHV cells induces tyrosine phosphorylation of Tie-2 in both human and mouse endothelial cells. A, Western blot detection of Tie-2 and phosphorylated (Tyr992) Tie-2 (P-Tie-2) in human and mouse endothelial cells. HUVECs and MDMVECs were cultured in their corresponding medium without growth factors and with 0.5% FBS for 12 hours, followed by stimulation with PBS (control) or recombinant human Ang-2 (rAng-2, 100 ng/ml) at 37°C for 30 minutes and subsequent Western blot detection with antibodies to Tie-2, P-Tie-2, and β-tubulin. B, Immunoprecipitation (IP) of Tie-2 from total protein lysates of HUVECs that were stimulated as described in A with a mouse monoclonal anti-Tie-2 antibody or IgG as control. The IP products were then analyzed by SDS-PAGE and Western blot detection (WB) with the same antibody to Tie-2 and a mouse monoclonal antibody to phosphorylated tyrosine (4G10). Mouse IgG heavy (IgG HC) and light chain (IgG LC) bands are indicated. C, Western blot detection of Tie-2, P-Tie-2, and β-tubulin from HUVECs that were stimulated with PBS (control), recombinant Ang-1 (rAng-1, 100 ng/ml), rAng-2 (100 ng/ml), or supernatant of TIVE-KSHV cells (Sup), in the presence or absence of AMG-386 or L1-10 at 1 µg/ml for 30 minutes respectively.
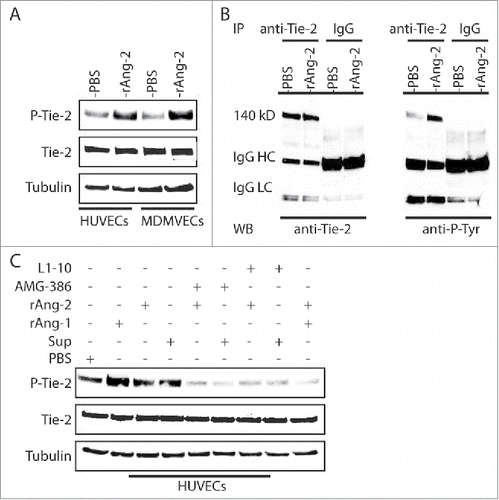
To further confirm Ang-2 induction of Tie-2 phosphorylation, we conducted immunoprecipitation (IP) to pull down Tie-2 protein from cell lysates of HUVECs that were stimulated with PBS and recombinant Ang-2, using a monoclonal antibody to Tie-2 and control IgG. The IP products were then analyzed by SDS-PAGE and Western blots with the same anti-Tie-2 antibody and a monoclonal antibody (4G10) to phosphorylated tyrosine respectively. As shown in , the precipitated Tie-2 protein from Ang-2 stimulated HUVECs displays significantly higher level of tyrosine phosphorylation.
To test if Ang-2 released by TIVE-KSHV cells interacts with Tie-2, in a parallel experiment, we stimulated HUVECs with recombinant Ang-1 (as a positive control for inducing Tie-2 phosphorylation), recombinant Ang-2, and culture supernatant from TIVE-KSHV cells in the absence or presence of Ang-2 inhibitors AMG-386 and L1-10. As shown in , Ang-1 very strongly induces Tie-2 phosphorylation. In contrast, Ang-2 and supernatant from TIVE-KSHV cells modestly induce Tie-2 phosphorylation. Both AMG-386 and L1-10 effectively block Ang-2 induced Tie-2 phosphorylation, which is consistent with previous report that these molecules bind to Ang-2 to prevent its interaction with Tie-2.Citation24
Ang-2 from TIVE-KSHV cells promotes angiogenesis in matrigel-based angiogenesis assays
To conduct in vivo angiogenesis assays, we mixed Matrigel solution with equal numbers of TIVE and TIVE-KSHV cells in the absence or presence of Ang-2 inhibitors AMG-386 and L1-10 respectively, followed by subcutaneous injection into nude mice. Two weeks upon inoculation, we collected the Matrigel pellets. As shown in , Matrigel blocks containing TIVE-KSHV cells are substantially more vascularized than those containing TIVE cells, suggesting that Ang-2 from TIVE-KSHV cells promotes angiogenesis. Notably, both Ang-2 inhibitors strongly inhibit blood vessel development in Matrigel blocks containing TIVE-KSHV cells.
Figure 3. Ang-2 from TIVE-KSHV cells promotes angiogenesis in vivo in nude mice. A, images of Matrigel blocks containing equal numbers (1 × 106) of TIVE or TIVE-KSHV cells in the presence of PBS (placebo), AMG-386, and L1-10 at 1 µg/ml each, which were collected 2 weeks post inoculation into nude mice. B, IFA staining of frozen sections of Matrigel blocks shown in A with a rat monoclonal antibody to mouse endothelial cell marker CD31 and an Alexa Fluor®-647 conjugated goat anti-rat IgG secondary antibody. DAPI was used for nuclear staining of all cells. Images were taken under 10× magnification with the EVOS™ FL cell imaging system (Thermo-Fisher Scientific). Examples of enlarged blood vessels are indicated with an arrow. C, average numbers of vessels per field (10× magnification) from 16 fields per Matrigel block and 2 blocks per group. Unpaired student t test was conducted to assess the statistical significance of differences between the different Matrigel blocks, with all P values smaller than 0.05. D, average concentrations of hemoglobin from 2 Matrigel blocks per group and 3 readings per block.
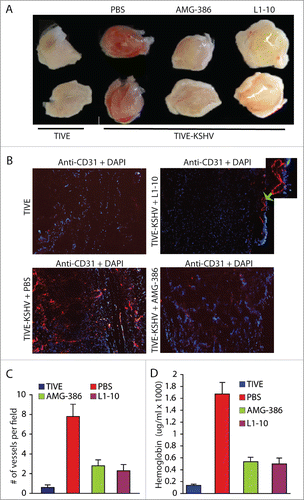
To quantitatively analyze blood vessels in the 4 different groups of Matrigel blocks, we performed IFA staining on Matrigel sections with an antibody to mouse endothelial cell marker CD31. As shown in , Matrigel blocks containing TIVE-KSHV cells display 13.3 times more blood vessels than those containing TIVE cells. In the presence of AMG-386 and L1-10, the numbers of blood vessels in Matrigel blocks containing TIVE-KSHV cells are reduced to 4.7 and 4.0 times that in Matrigel blocks containing TIVE cells respectively. Consistent with differences in the numbers of blood vessels, the relative hemoglobin levels in Matrigel blocks containing TIVE-KSHV cells are substantially higher than those containing TIVE cells, which are also reduced by both Ang-2 inhibitors ().
To further confirm the role of KSHV-induced Ang-2 in promoting angiogenesis, we knocked down expression of Ang-2 in TIVE-KSHV cells with Ang-2 specific shRNA (). By consequence, the supernatant of TIVE-KSHV cells expressing Ang-2 shRNA contains 21 ng/ml Ang-2, which is 5.1 times lower than that in the supernatant of TIVE-KSHV expressing control shRNA (). We then conducted similar Matrigel-based in vivo angiogenesis assays in nude mice by using identical numbers of TIVE-KSHV cells expressing Ang-2 shRNA or control shRNA. As shown in , Matrigel blocks containing TIVE-KSHV cells with Ang-2 “knock-down” display significantly fewer blood vessels and lower levels of hemoglobin than Matrigel blocks containing TIVE-KSHV cells expressing control shRNA. Collectively, these results demonstrate that Ang-2 from TIVE-KSHV cells promotes angiogenesis in nude mice by acting on mouse endothelial cells, which is inhibited when its activity is blocked by the 2 Ang-2 inhibitors or its expression is suppressed by Ang-2 shRNA.
Figure 4. Ang-2 “knock-down” in TIVE-KSHV cells reduces angiogenesis. A, real-time RT-PCR measurement of Ang-2 mRNA in TIVE-KSHV cells stably expressing control or Ang-2 specific shRNA. B, Western blot detection of Ang-2 protein in lysates of TIVE-KSHV cells stably expressing control or Ang-2 specific shRNA. C, Ang-2 concentrations in the supernatants of TIVE-KSHV cells stably expressing control or Ang-2 specific shRNA, which were measured by ELISA. D, Matrigel blocks containing identical numbers (1 × 106) of TIVE and TIVE-KSHV cells stably expressing control or Ang-2 specific shRNA, which were collected at 2 weeks post inoculation into nude mice. E and F, numbers of blood vessels per field and relative concentration of hemoglobin in Matrigel blocks measured and analyzed as described in .
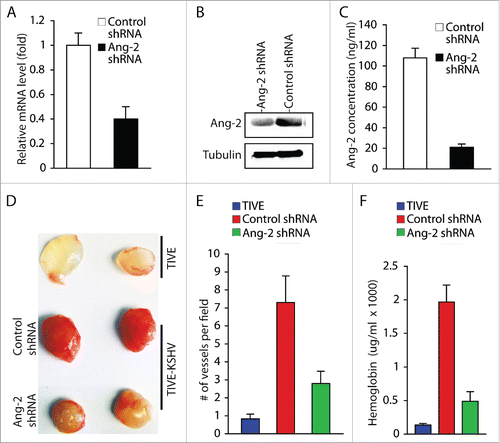
Ang-2 from TIVE-KSHV cells contributes to tumor growth
To determine the role of KSHV-induced Ang-2 in tumor development, we subcutaneously injected equal numbers (5 × 106 per injection site) of TIVE-KSHV cells into nude mice. One week after inoculation, we treated the mice with PBS (placebo), AMG-386, and L1-10 twice a week at 2.5 mg/kg body mass respectively. As shown in , both AMG-386 and L1-10 inhibit tumor growth. The average tumor weights from mice treated with AMG-386 and L1-10 at the end of experiment were 55.7% and 62.2% that of mice treated with PBS respectively (). It is well established that Ang-2 does not possess any mitogenic activity. To rule out the possibility that inhibition of tumor growth by the 2 Ang-2 inhibitors results from cytotoxicity, we performed cell proliferation assays by culturing TIVE-KSHV cells in the presence of various concentrations of AMG-386 and L1-10, respectively. As shown in , no obvious cytotoxicity by either one of these molecules is seen even at high dose (5 µg/ml).
Figure 5. AMG-386 and L1-10 inhibit tumor growth in nude mice. Equal numbers (5 × 106 per injection site, 2 sites per mouse) of TIVE-KSHV cells were subcutaneously injected into 4 week old female nude mice. One week after inoculation, the mice were treated with placebo (PBS), AMG-386, and L1-10 twice a week at 2.5 mg/kg body mass respectively by subcutaneous injection. A, representative tumors in 3 mice from each group at 8 weeks post inoculation. B, average tumor volumes (length x width x height) with standard error of the mean (SEM) values from 24 tumors in 12 mice per group, which were measured once a week and over a period of 8 weeks. Unpaired student t test was conducted to determine the statistical significance of differences between the control and treated mice. Differences with P < 0.05 are indicated with a star. C, average weights of 24 tumors from each group of mice, which were collected at the end of experiment. Unpaired student t test was conducted to compare the different groups of mice. Differences between the control mice and those treated with AMG-386 or L1-10 are statistically significant with P values smaller than 0.05. No significant (ns) difference is seen between treatment with AMG-386 and L1-10. D and E, proliferation rates of TIVE-KSHV cells in culture in the presence of different doses (0, 1.0 µg/ml, 2.5 µg/ml, and 5.0 µg/ml) of AMG-386 and L1-10, respectively. Equal numbers (2 × 104/well) of TIVE-KSHV cells were seeded in each well of 12-well plates on day 0, and counted every 24 hours with a hemocytometer. The average number from 2 readings per well and 3 well per dose was calculated and used for the comparison.
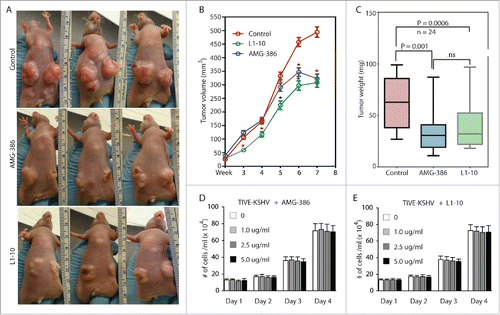
To further confirm the contribution of Ang-2 to tumor growth, we inoculated equal numbers (5 × 106 per injection site) of TIVE-KSHV cells expressing control or Ang-2 specific shRNA into nude mice. As shown in , the inhibitory effect of Ang-2 “knock-down” on tumor growth is even more pronounced than that of the Ang-2 inhibitors. The average tumor weight of 10 tumors from TIVE-KSHV cells with Ang-2 “knock-down” is 31.5% that of 10 tumors from TIVE-KSHV cells expressing control shRNA at the end of the experiment (). To examine if the reduced tumor growth by TIVE-KSHV cells expressing Ang-2 shRNA results from lower cell proliferation, we performed a cell proliferation assay in culture. As shown in , no obvious difference in cell proliferation rate is seen between cells expressing control shRNA and cells expressing Ang-2 specific shRNA, suggesting that Ang-2 “knock down” has no effect on proliferation.
Figure 6. Ang-2 “knock-down” in TIVE-KSHV cells strongly inhibits tumor growth. A, representative tumors from mice inoculated with equal numbers (5 × 106 per injection site, 2 sites per mouse) of TIVE-KSHV cells stably expressing control or Ang-2 specific shRNA at 8 weeks post inoculation. B, tumor volume (length x width x height) with SEM values from 5 mice and 10 tumors per group over a period of 8 weeks. C, average weights of 10 tumors from each group of mice collected at 8 weeks post inoculation. Differences in tumor weight between the 2 groups of mice are statistically significant with a P value smaller than 0.05. D, proliferation rates of TIVE-KSHV cells expressing control or Ang-2-specific shRNA in culture. Equal numbers (2 × 104/well) of cells were loaded into 12-well plates on day 0, and the numbers of cells per well were determined daily for 5 days with a hemocytometer. The average number from 2 readings per well and 3 well per cell line was calculated and used for the comparison.
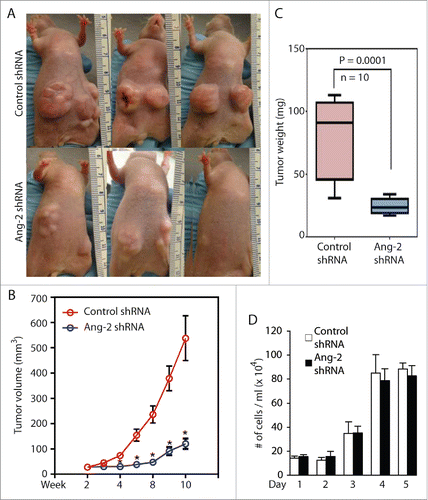
Ang-2 from TIVE-KSHV cells contributes to tumor growth by promoting angiogenesis and infiltration of monocytes/macrophages
Since neither functional inhibition nor expressional “knock-down” of Ang-2 displays any effect on proliferation of TIVE-KSHV cells, we reasoned that Ang-2 contributes to tumor growth mainly by promoting angiogenesis. To test this hypothesis, we conducted IFA staining on tumor sections with an antibody to mouse blood vessel endothelial cell marker CD31, and then counted the number of vessels per field under a fluorescence microscope. As shown in , the average numbers of vessels in tumors from mice treated with AMG-386 and L1-10 are substantially reduced, representing 30.5% and 27.3% that of tumors from mice treated with PBS, respectively. These results, along with data from the in vivo Matrigel-based angiogenesis assays, confirm a pivotal role of KSHV-induced Ang-2 in promoting tumor angiogenesis.
Figure 7. AMG-386 and L1-10 inhibit tumor angiogenesis. A, IFA staining of tumor sections from mice treated with placebo (PBS), AMG-386, and L1-10 with an antibody to mouse CD31 as described in . DAPI was used for nuclear staining of all cells. Enlarged representative blood vessels were indicated with an arrow. B, average numbers of vessels per field (10× magnification) from 4 fields per tumor and 8 tumors per group. Significant differences are seen between tumors from mice treated with PBS and those treated with AMG-386 or L1-10, with P values smaller than 0.05.
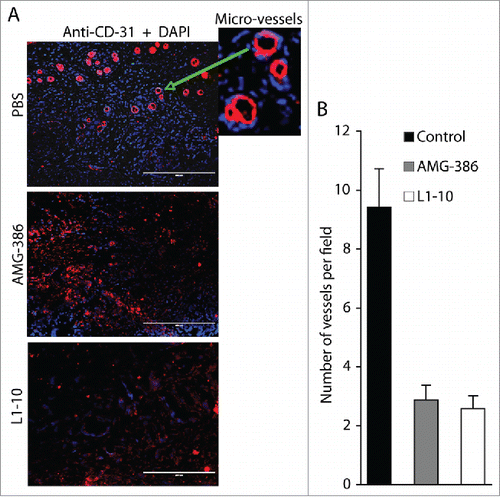
The presence of large amounts of inflammatory cells including monocytes/macrophages is an important feature of KS tumors.Citation3 To determine if Ang-2 plays a role in promoting infiltration of monocytes/macrophages into tumors, we prepared single cell suspensions from the different groups of tumors and stained the cells with mouse monocyte/macrophage markers CD16 and F4/80, followed by flow cytometry analysis of the cells expressing both markers. As shown in , the average percentages of monocytes/macrophages in 6 tumors from mice treated with PBS, AMG-386, and L1-10 are 20.6% ± 4.1%, 8.7% ± 2.7%, and 9.8% ± 2.5%, respectively. By IFA staining of tumor sections with anti-mouse F4/80 antibody, we also observed a significant reduction of monocytes/macrophages in tumors treated with AMG-386 (). These results suggest that Ang-2 from TIVE-KSHV cells not only promotes tumor angiogenesis but also contributes to infiltration of monocytes/macrophages into tumors.
Figure 8. AMG-386 and L1-10 reduce the numbers of infiltrating monocytes/macrophages in tumors. A, representatives of flow cytometry analysis of monocyte/macrophages in single cell suspensions of tumors from mice treated with placebo (PBS), AMG-386, and L1-10 respectively. Mouse monocytes/macrophages were stained with an Alexa Fluor® 647-coupled anti-CD16 antibody and a Pacific Blue®-coupled anti-F4/80 antibody. B, average percentages of cells expressing F4/80 and CD16 in single cell suspensions from 6 tumors per group. Significant differences are seen between tumors from mice treated with PBS and those treated with AMG-386 or L1-10, with P values smaller than 0.05. C, IFA staining of tumor sections from mice treated with PBS and AMG-386, with a rat monoclonal antibody to F4/80 and an Alexa Fluor® 647-coupled goat anti-rat secondary antibody. DAPI was used for nuclear staining.
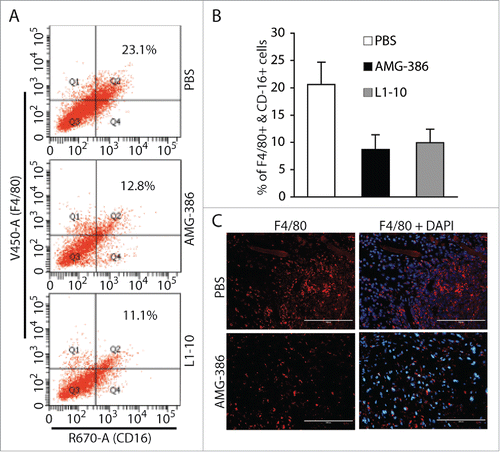
Ang-2 from TIVE-KSHV cells promotes migration of monocytes
To demonstrate that Ang-2 indeed promotes migration and infiltration of monocytes, we performed transwell-based in vitro cell migration assays by using the human monocyte cell line U937. We loaded equal numbers (2 × 105) of U937 cells into the upper chambers and 600 µl of fresh medium or culture supernatant from TIVE-KSHV cells in the absence or presence of AMG-386 and L1-10 into the lower wells of the transwell plates. Upon incubation at 37°C for 8 hours, we counted the numbers of cells that traveled through the transwell membrane into the lower wells. As shown in , compared to fresh medium, culture supernatant from TIVE-KSHV cells gives rise to a 3.04-fold increase in the number of cells that migrate to the lower wells. However, in the presence of AMG-386 and L1-10, the increase drops to 1.53 and 1.68 folds respectively. In a parallel cell migration assay, supernatant from TIVE-KSHV cells expressing control shRNA gives rise to 2.06 times more cells that migrate to the lower wells than supernatant from TIVE-KSHV cells expressing Ang-2 specific shRNA (). Furthermore, when recombinant Ang-2 was added to fresh medium, an Ang-2 dose-dependent effect is seen on cell migration (). Collectively, these results demonstrate a significant role of Ang-2 in promoting migration of monocytes.
Figure 9. Ang-2 promotes migration of monocytes. A and B, transwell-based cell migration assays using human monocytes U937. Equal numbers (2 × 105) of monocytes were loaded into the upper chamber, and 600 μl of RPMI 1640 medium (Med), supernatant (Sup) from TIVE-KSHV cells in the presence of placebo (PBS), AMG-386 (1 µg/ml), or L1-10 (1 µg/ml), and supernatant from TIVE-KSHV cells expressing control or Ang-2 specific shRNA, were loaded to the lower wells of 24-well plate. Upon incubation at 37°C for 8 hours, the average number of cells in the lower wells from 4 repeats was calculated and used for the comparison. C, cell migration assay using U937 cells and RPMI 1640 medium in the presence of various concentrations (0 to 100 ng/ml) of recombinant Ang-2 (rAng-2).
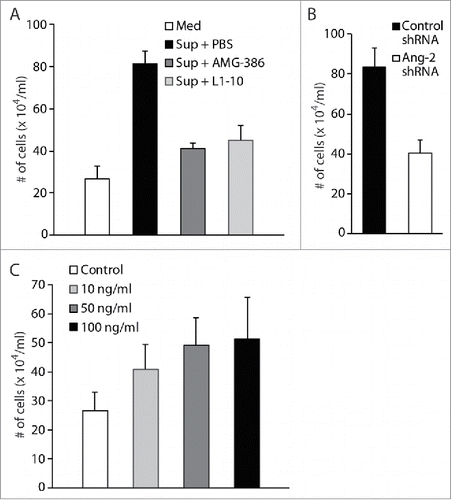
Discussion
The involvement of Ang-2 in tumor development has been well documented in various types of cancer.Citation37,38 Ang-2 is also highly present in KS tumors.Citation29,31 Previous studies demonstrated that acute KSHV infection of endothelial cells not only up-regulates Ang-2 expression but also induces its rapid release from endothelial cells.Citation4,30-32 In the present study, we found that Ang-2 is also strongly expressed in TIVE-KSHV cells, which, like most KS tumor cells, have latent KSHV infection.Citation3,39 Therefore, both acute and latent KSHV infection of endothelial cells up-regulates Ang-2 expression, which may account for the high level of Ang-2 in KS tumors.Citation29,31
By using TIVE-KSHV cells as a KS tumor model, we found that Ang-2 secreted from TIVE-KSHV cells induces tyrosine phosphorylation of Tie-2 in both human and mouse endothelial cells. In KS patients, Ang-2 secreted from KSHV-infected KS tumor cells may behave similarly and act on healthy endothelial cells to promote tumor angiogenesis. We also demonstrate that both functional inhibition and expressional “knock-down” of Ang-2 from TIVE-KSHV cells inhibit tumor growth in nude mice. Since suppression of Ang-2 has little effect on proliferation of TIVE-KSHV cells in culture, we conclude that Ang-2 contributes to tumor growth mainly by promoting angiogenesis. Data from both Matrigel-based angiogenesis assays and IFA staining on tumor sections with anti-mouse blood endothelial cell marker CD31 consistently support a pivotal role of Ang-2 in promoting tumor angiogenesis. While both functional inhibition and expressional “knock-down” of Ang-2 inhibit angiogenesis and tumor growth, the effect of Ang-2 “knock-down” is more pronounced than its functional inhibition by AMG-386 and L1-10. This is likely because angiogenesis is restricted right after inoculation of TIVE-KSHV cells with Ang-2 “knock-down” into nude mice.
Although Ang-2/Tie-2 signaling plays an essential role in angiogenesis, multiple recent studies demonstrated that Ang-2 also regulates angiogenesis through integrin signaling.Citation40,41 A subpopulation of endothelial cells does not express Tie-2 during angiogenesis. Ang-2 binds to integrins in these cells, resulting in phosphorylation of integrins and RAC1 activation, cell migration, and blood vessel sprouting.Citation40 Therefore, Ang-2 from TIVE-KSHV cells may also contribute to tumor angiogenesis through integrin signaling.
An important feature of KS tumors is the presence of large amounts of infiltrating inflammatory cells including monocytes and macrophages.Citation3 Monocytes also express Tie-2,Citation42,43 and a previous study showed that Ang-2 stimulates Tie-2-expressing monocytes to suppress T cell activation and induce expansion of regulatory T cells, resulting in suppression of anti-tumor immunity.Citation44 Ang-2 has also been shown to promote migration of myeloid cells through interaction with integrin.Citation45 We demonstrated that Ang-2 from TIVE-KSHV cells enhances migration of monocytes in transwell-based in vitro cell migration assays. Suppression of Ang-2 not only inhibits migration of monocytes in the in vitro cell migration assays but also significantly reduces the numbers of infiltrating monocytes/macrophages into tumors in mice. Thus, these results reveal a new role of Ang-2 in promoting migration and infiltration of monocytes into tumors. The infiltrating monocytes may differentiate and polarize into tumor-associated macrophages (TAMs), which are well known to promote angiogenesis, anti-tumor innate immunity, and tumor invasion and metastasis.Citation46,47 Therefore, promoting migration and infiltration of monocytes/macrophages is likely another important mechanism by which KSHV-induced Ang-2 contributes to tumor growth.
In summary, our results underscore a pivotal role of KSHV-induced Ang-2 in the development of KS by promoting angiogenesis and infiltration of monocytes/macrophages. Our study also provides the first pre-clinical evidence that Ang-2 is a promising target for chemotherapy of KS. In this regard, the 2 Ang-2 inhibitors AMG-386 and L1-10 are particularly useful and promising. Both AMG-386 and L1-10 effectively inhibit tumor angiogenesis and reduce the numbers of infiltrating monocytes/macrophages. Neither inhibitor completely blocks tumor growth in our model, which might be the result of multiple reasons. Anti-angiogenesis therapy alone has been proven insufficient to cure cancer in general. A combination of these inhibitors with other anti-cancer therapies might be necessary to completely block tumor growth.Citation48 We only treated the mice twice a week for a period of 7 weeks. With more frequent and longer treatment, the effectiveness of these inhibitors may be significantly improved. In addition, Ang-2 may promote angiogenesis through integrin signaling. A combination of AMG-386 and L1-10 with integrin antagonists may be needed to completely block tumor angiogenesis. It is important to note that both AMG-386 and L1-10 have successfully passed early phases of human trials for the treatment of multiple cancers.Citation27,28,49-51 These molecules may offer new and promising treatments for KS.
Materials and methods
Cells, culture media, and reagents
TIVE and human umbilical vein endothelial cells (HUVECs) were cultured in endothelial cells medium EBM2 supplemented with growth factors and 2% fetal bovine serum (FBS) (Lonza, Allendale, New Jersey, USA). The original TIVE-KSHV cells are infected with native KSHV.Citation33 We re-infected these cells with the recombinant KSHV, BAC16, which constitutively expresses a green fluorescence protein (GFP) marker.Citation34 The re-established TIVE-KSHV cells and human monocyte cell line U937 were grown in 1640 RPMI medium plus 10% FBS. Ang-2 inhibitors AMG-386 and L1-10 were provided by Amgen, Inc. (Thousand Oaks, California, USA). C57BL/6 mouse primary dermal microvascular endothelial cells (MDMVECs) were purchased from Cell Biologics, Inc. (Chicago, Illinois, USA) and cultured with mouse endothelial cells culture medium provided by the manufacturer. Antibodies to Tie-2 and phosphorylated Tie-2 (Tyr992) with specificity for both human and mouse Tie-2 were purchased from Cell Signaling (Danvers, Massachusetts, USA). A mouse monoclonal antibody specific for both human and mouse Tie-2 and a mouse monoclonal antibody (clone 4G10) specific for phosphorylated tyrosine were purchased from EMD Millipore (Billerica, Massachusetts, USA). Recombinant Ang-1, Ang-2, antibodies to mouse CD31 and monocyte/macrophage marker F4/80, a rabbit polyclonal antibody to human Ang-2, and control rabbit IgG were purchased from R&D Systems, Inc. (Minneapolis, Minnesota, USA). Antibodies to KSHV latent nuclear antigen (LANA) and lytic protein ORF59 were purchased from Biocompare (San Francisco, California, USA) and Advanced Biotechnologies, Inc. (Columbia, Maryland, USA), respectively.
Ang-2 “Knock-down” and real-time PCR detection of Ang-1 and Ang-2 mRNAs
Lentiviruses expressing Ang-2 specific or control shRNA were purchased from Santa Cruz Biotechnologies (Dallas, Texas, USA). Two days after lentiviral transduction, cells stably expressing Ang-2 specific or control shRNA were selected with puromycin at 5 μg/ml. Ang-2 “knock-down” was verified by real-time RT-PCR measurement of Ang-2 mRNA as described previously Citation52 and Western blot analysis of Ang-2 protein.
The levels of Ang-1 mRNA in TIVE and TIVE-KSHV cells were measured by real-time RT-PCR using the primers 5′GCCTACACTTTCATTCTTCCAGA3′ (forward) and 5′ TCTTCCTTGTGTTTTCCTTCCAT3′ (reverse). The levels of GAPDH mRNA were measured by using the primers 5′GGTGAAGGTCGGAGTCAACG3′ (forward) and 5′CAAAGTTGTCATGGATGACC3′ (reverse), which were used as a reference for normalization and calculation of the relative levels of Ang-1 and Ang-2 mRNAs.
Immunoprecipitation (IP) and Western blot detection of Tie-2 and phosphorylated Tie-2 (P-Tie-2)
HUVECs were stimulated with PBS (control) or recombinant Ang-2 (100 ng/ml) at 37°C for 30 minutes. Total protein lysates from these cells were prepared in protein lysis buffer containing 150 mM NaCl, 100 mM Tris (pH 7.4), 1 mM EDTA, I mM EGTA, 0.5% NP-40, and a cocktail of proteinase and phosphatase inhibitors. A standard procedure for IP was followed, using 5 µg/ml mouse monoclonal antibody to Tie-2 or control IgG. The IP products were then separated in polyacrylamide gel by SDS-PAGE, transferred onto nitrocellulose membrane, and analyzed by Western blot detection with the same antibody to Tie-2 or a monoclonal antibody to phosphorylated tyrosine (4G10).
ELISA measurement of Ang-2 in culture supernatants
The concentrations of Ang-2 in culture supernatants were determined by following a standard procedure for “double-sandwich” ELISA, using colorimetric ELISA substrates for horseradish peroxidase (HRP) from Thermo-Fisher Scientific. Serial dilutions of recombinant Ang-2 were made and used in the ELISA to establish a concentration vs. optimal density standard curve for each protein, and the concentration of Ang-2 in a given sample was calculated by using the corresponding standard curve. Each measurement consisted of 6 replicates, and the concentration of a given sample was the average of the 6 replicates.
Matrigel-based in vivo angiogenesis assay
Matrigel cell matrix solution with low level of growth factor supplements was purchased from BD Biosciences (San Jose, California, USA). The Matrigel solution was mixed with equal numbers (1 × 106) of TIVE or TIVE-KSHV cells in phosphate buffered saline (PBS, placebo), with or without AMG-386 and L1-10 at 1 µg/ml each. The mixtures were then subcutaneously injected into nude mice. Two weeks after inoculation, the solidified Matrigel blocks were surgically removed from the mice. Half of each Matrigel block was frozen sectioned and immunochemically stained with a rat monoclonal antibody to mouse blood vessel endothelial cell marker CD31 (BD Bioscience). The other half of each Matrigel block was weighed and homogenized in 50 mM EDTA at 1 mg Matrigel per ml, and the supernatant was used for determination of the relative hemoglobin level. A colorimetric assay for measurement of hemoglobin concentration was carried out by using Drabkin's reagent (Sigma Aldrich, St. Louis, Missouri, USA) and following the manufacturer's instruction. Serial dilutions of human hemoglobin (Sigma Aldrich) were made and used to establish a standard curve. Hemoglobin concentrations of the samples were calculated by using the standard curve.
Tumor growth and measurement in nude mice
Equal numbers (5 × 106) of TIVE-KSHV or TIVE-KSHV cells stably expressing Ang-2 specific or control shRNA were subcutaneously injected into 4 to 6 week old nude mice. One week after inoculation of TIVE-KSHV cells, the mice were treated by subcutaneous injection of placebo (PBS), AMG-386, and L1-10 twice a week at 2.5mg/kg body mass, respectively. Tumor volumes (length × width × height) were measured once a week with a caliper. At the end of experiments, all tumors were surgically removed from the mice, weighed individually, and imaged together. All procedures were carried out in strict accordance with the recommendations in the Guide for the Care and Use of Laboratory Animals of the National Institutes of Health and following a protocol that was approved by the Institutional Animal Care and Use Committee (IACUC) at Case Western Reserve University.
Determination of infiltrating monocytes/macrophages in tumors
Single cell suspension from tumors was prepared by using collagenase as reported previously.Citation53 Each tumor was minced and incubated with 0.2% collagenase-D (Sigma-Aldrich) in PBS for 1 hour at 37°C. The cell suspension was then passed through a 70µm cell strainer and centrifuged at 1500 rpm for 5 minutes. The supernatant was discarded and the pellet was washed twice with PBS and re-suspended in 1 ml PBS. The single cell suspension from each tumor was then incubated with an Alexa Fluor® 647-coupled anti-CD16 monoclonal antibody (BD Biosciences) at room temperature for 20 minutes, followed by fixation with 4% paraformaldehyde in PBS for 20 minutes. The cells were washed once with PBS and incubated with a Pacific Blue®-coupled anti-mouse F4/80 monoclonal antibody (Bio-Rad, Hercules, California, USA) in PBS for 30 min at room temperature. After being washed 3 times with PBS, the numbers of cells expressing CD16 and F4/80 were determined by flow cytometry analysis.
Transwell-based in vitro cell migration assay
Transwell® permeable support with 5.0 μM polyester membrane and 24-well culture plates (Corning Inc., Corning, New York, USA) were used for cell migration assays, using the human monocyte cell line U937 (ATCC, Manassas, Virginia, USA). Briefly, equal numbers (2 × 105) of U937 cells were loaded into the upper chamber, which was then placed into the 24-well plates with the lower wells filled with 600 μl of culture supernatant from TIVE-KSHV cells in the presence of PBS, AMG-386 (1 µg/ml), and L1-10 (1 µg/ml) respectively, or with 600 μl fresh RPMI 1640 in the presence of various concentrations of recombinant Ang-2. Upon culture at 37°C for 8 hours, cells that traveled through the membrane to the lower wells were harvested and enriched in 100 μl RPMI 1640 medium, followed by cell counting with a hemocytometer. Each cell migration assay consisted of 4 replicates.
Immuno-fluorescence antibody (IFA) staining and confocal imaging
Cells were first incubated with a rat antibody to KSHV latent protein LANA and a rabbit antibody to Ang-2, respectively, followed by staining with an Alexa Fluor®-594 conjugated goat anti-rat antibody and an Alexa Fluor®-633 conjugated goat anti-rabbit antibody to detect LANA and Ang-2. DAPI was used for nuclear staining of all cells. Confocal images were collected using gated HyD detectors on a TCS SP8 X WLL system (Leica Microsystems, Wetzler, Germany) with a 40× oil objective (HC PL APO 1.3 NA), pinhole at 1 Airy unit, and driven by LAS AF software. Images were collected in sequential scanning mode (405 nm diode laser for DAPI plus White Light Laser at 488, 593 and 633 nm for GFP and Alexa Fluors) to avoid cross talk. Projections of images (1024 × 1024) were maximal.
Disclosure of potential conflicts of interest
No potential conflicts of interest were disclosed.
Acknowledgements
We thank Amgen, Inc. for providing Ang-2 inhibitors AMG-386 and L1-10 and technical instruction.
Funding
This research was supported in part by the Skin Disease Research Center Grant 5P30AR039750-24, the Office of Research Infrastructure (NIH-ORIP) Shared Instrumentation Grant S10 OD016164, and the Clinical and Translational Science Collaborative of Cleveland pilot grant UL1TR000439 from the National Center for Advancing Translational Science (NCATS) component of the National Institute of Health and NIH Roadmap for Medical Research. Its contents are solely the responsibility of the authors and do not necessarily represent the official views of the NIH.
References
- Chang Y, Cesarman E, Pessin MS, Lee F, Culpepper J, Knowles DM, Moore PS. Identification of herpesvirus-like DNA sequences in AIDS-associated Kaposi's sarcoma. Science 1994; 266:1865-69; PMID:7997879; http://dx.doi.org/10.1126/science.7997879
- Boshoff C, Weiss RA. Epidemiology and pathogenesis of Kaposi's sarcoma-associated herpesvirus. Philos Transa R Soc Lond B Biol Sci 2001; 356:517-34; http://dx.doi.org/10.1098/rstb.2000.0778
- Boshoff C. Kaposi's sarcoma biology. IUBMB Life 2002; 53:259-61; PMID:12121006; http://dx.doi.org/10.1080/15216540212645
- Wang HW, Trotter MW, Lagos D, Bourboulia D, Henderson S, Makinen T, Elliman S, Flanagan AM, Alitalo K, Boshoff C. Kaposi sarcoma herpesvirus-induced cellular reprogramming contributes to the lymphatic endothelial gene expression in Kaposi sarcoma. Nat Genet 2004; 36:687-93; PMID:15220918; http://dx.doi.org/10.1038/ng1384
- Wang L, Wakisaka N, Tomlinson CC, DeWire SM, Krall S, Pagano JS, Damania B. The Kaposi's sarcoma-associated herpesvirus (KSHV/HHV-8) K1 protein induces expression of angiogenic and invasion factors. Cancer Res 2004; 64:2774-81; PMID:15087393; http://dx.doi.org/10.1158/0008-5472.CAN-03-3653
- Hensler HR, Rappocciolo G, Rinaldo CR, Jenkins FJ. Cytokine production by human herpesvirus 8-infected dendritic cells. J Gen Virol 2009; 90:79-83; PMID:19088276; http://dx.doi.org/10.1099/vir.0.006239-0
- Murakami-Mori K, Mori S, Bonavida B. Molecular pathogenesis of AIDS-associated Kaposi's sarcoma: growth and apoptosis. Adv Cancer Res 2000; 78:159-97; PMID:10547670; http://dx.doi.org/10.1016/S0065-230X(08)61025-8
- Sivakumar R, Sharma-Walia N, Raghu H, Veettil MV, Sadagopan S, Bottero V, Varga L, Levine R, Chandran B. Kaposi's sarcoma-associated herpesvirus induces sustained levels of vascular endothelial growth factors A and C early during in vitro infection of human microvascular dermal endothelial cells: biological implications. J Virol 2008; 82:1759-76; PMID:18057235; http://dx.doi.org/10.1128/JVI.00873-07
- Xu Y, Ganem D. Induction of chemokine production by latent Kaposi's sarcoma-associated herpesvirus infection of endothelial cells. J Gen Viol 2007; 88:46-50; http://dx.doi.org/10.1099/vir.0.82375-0
- Bala K, Bosco R, Gramolelli S, Haas DA, Kati S, Pietrek M, Havemeier A, Yakushko Y, Singh VV, Dittrich-Breihdz O, et al. Kaposi's sarcoma herpesvirus K15 protein contributes to virus-induced angiogenesis by recruiting PLCgamma1 and activating NFAT1-dependent RCAN1 expression. PLoS Pathog 2012; 8:e1002927.
- Klouche M, Carruba G, Castagnetta L, Rose-John S. Virokines in the pathogenesis of cancer: focus on human herpesvirus 8. Ann N Y Acad Sci 2004; 1028:329-39; PMID:15650258
- Lindahl P, Hellstrom M, Kalen M, Betsholtz C. Endothelial-perivascular cell signaling in vascular development: lessons from knockout mice. Curr Opin Lipidol 1998; 9:407-11; PMID:9812194; http://dx.doi.org/10.1097/00041433-199810000-00004
- Patan S. TIE1 and TIE2 receptor tyrosine kinases inversely regulate embryonic angiogenesis by the mechanism of intussusceptive microvascular growth. Microvasc Res 1998; 56:1-21; PMID:9683559; http://dx.doi.org/10.1006/mvre.1998.2081
- Sato TN, Qin Y, Kozak CA, Audus KL. Tie-1 and tie-2 define another class of putative receptor tyrosine kinase genes expressed in early embryonic vascular system. Proc Natl Acad Sci U S A 1993; 90:9355-58; PMID:8415706; http://dx.doi.org/10.1073/pnas.90.20.9355
- Schnurch H, Risau W. Expression of tie-2, a member of a novel family of receptor tyrosine kinases, in the endothelial cell lineage. Development 1993; 119:957-68; PMID:8187650
- Davis S, Aldrich TH, Jones PF, Acheson A, Compton DL, Jain V, Ryan TE, Bruno J, Radziejewski C, Maisonpierre PC, Yancopoulos GD. Isolation of angiopoietin-1, a ligand for the TIE2 receptor, by secretion-trap expression cloning. Cell 1996; 87:1161-69; PMID:8980223; http://dx.doi.org/10.1016/S0092-8674(00)81812-7
- Maisonpierre PC, Suri C, Jones PF, Bartunkova S, Wiegand SJ, Radziejewski C, Compton D, McClain J, Aldrich J, Papadopoulos N, et al. Angiopoietin-2, a natural antagonist for Tie2 that disrupts in vivo angiogenesis. Science 1997; 277:55-60; PMID:9204896; http://dx.doi.org/10.1126/science.277.5322.55
- Yuan HT, Khankin EV, Karumanchi SA, Parikh SM. Angiopoietin 2 is a partial agonist/antagonist of Tie2 signaling in the endothelium. Mol Cell Biol 2009; 29:2011-22; PMID:19223473; http://dx.doi.org/10.1128/MCB.01472-08
- Daly C, Eichten A, Castanaro C, Pasnikowski E, Adler A, Lalani AS, Papadopoulos N, Kyle AH, Minchinton AI, Yancopoulos GD, et al. Angiopoietin-2 functions as a Tie2 agonist in tumor models, where it limits the effects of VEGF inhibition. Cancer Res 2013; 73:108-118; PMID:23149917; http://dx.doi.org/10.1158/0008-5472.CAN-12-2064
- Fiedler U, Reiss Y, Scharpfenecker M, Grunow V, Koidl S, Thurston G, Gale NW, Witzenrath M, Rosseau S, Suttorp N, et al. Angiopoietin-2 sensitizes endothelial cells to TNF-α and has a crucial role in the induction of inflammation. Nat Med 2006; 12:235-39; PMID:16462802; http://dx.doi.org/10.1038/nm1351
- Scholz A, Plate KH, Reiss Y. Angiopoietin-2: a multifaceted cytokine that functions in both angiogenesis and inflammation. Ann N Y Acad Sci 2015; 1347:45-51; PMID:25773744; http://dx.doi.org/10.1111/nyas.12726
- Kawaguchi M, Sugaya M, Suga H, Miyagaki T, Ohmatsu H, Fujita H, Asano Y, Tada Y, Kadono T, Sato S. Serum levels of angiopoietin-2, but not angiopoietin-1, are elevated in patients with erythrodermic cutaneous T-cell lymphoma. Acta Derm Venereol 2014; 94:9-13; PMID:23817541; http://dx.doi.org/10.2340/00015555-1633
- Park JH, Choi H, Kim YB, Kim YS, Sheen SS, Choi JH, Lee HL, Lee KS, Chung WY, Lee S, et al. Serum angiopoietin-1 as a prognostic marker in resected early stage lung cancer. Lung Cancer 2009; 66:359-64; PMID:19339077; http://dx.doi.org/10.1016/j.lungcan.2009.03.002
- Oliner J, Min H, Leal J, Yu D, Rao S, You E, Tang X, Kim H, Meyer S, Han SJ, et al. Suppression of angiogenesis and tumor growth by selective inhibition of angiopoietin-2. Cancer Cell 2004; 6:507-16; PMID:15542434; http://dx.doi.org/10.1016/j.ccr.2004.09.030
- Coutelle O, Schiffmann LM, Liwschitz M, Brunold M, Goede V, Hallek M, Kashkar H, Hacker UT. Dual targeting of Angiopoetin-2 and VEGF potentiates effective vascular normalisation without inducing empty basement membrane sleeves in xenograft tumours. Br J Cancer 2015; 112:495-503; PMID:25562438; http://dx.doi.org/10.1038/bjc.2014.629
- Liontos M, Lykka M, Dimopoulos MA, Bamias A. Profile of trebananib (AMG386) and its potential in the treatment of ovarian cancer. Onco Targets Ther 2014; 7:1837-45; PMID:25336975
- Robson EJ, Ghatage P. AMG 386: profile of a novel angiopoietin antagonist in patients with ovarian cancer. Expert Opin Investig Drugs 2011; 20:297-304; PMID:21210755; http://dx.doi.org/10.1517/13543784.2011.549125
- Suzuki R, Yamamoto H, Ngan CY, Ohtsuka M, Kitani K, Uemura M, Nishimura J, Takemasa I, Mizushima T, Sekimoto M, S et al. Inhibition of angiopoietin 2 attenuates lumen formation of tumour-associated vessels in vivo. Int J Oncol 2013; 43:1447-55; PMID:23982687
- Brown LF, Dezube BJ, Tognazzi K, Dvorak HF, Yancopoulos GD. Expression of Tie1, Tie2, and angiopoietins 1, 2, and 4 in Kaposi's sarcoma and cutaneous angiosarcoma. Am J Pathol 2000; 156:2179-83; PMID:10854238; http://dx.doi.org/10.1016/S0002-9440(10)65088-2
- Vart RJ, Nikitenko LL, Lagos D, Trotter MW, Cannon M, Bourboulia D, Gratrix F, Takeuchi Y, Boshoff C. Kaposi's sarcoma-associated herpesvirus-encoded interleukin-6 and G-protein-coupled receptor regulate angiopoietin-2 expression in lymphatic endothelial cells. Cancer Res 2007; 67:4042-51; PMID:17483315; http://dx.doi.org/10.1158/0008-5472.CAN-06-3321
- Ye FC, Blackbourn DJ, Mengel M, Xie JP, Qian LW, Greene W, Yeh IT, Graham D, Gao SJ. Kaposi's sarcoma-associated herpesvirus promotes angiogenesis by inducing angiopoietin-2 expression via AP-1 and Ets1. J Virol 2007; 81:3980-91; PMID:17287278; http://dx.doi.org/10.1128/JVI.02089-06
- Ye FC, Zhou FC, Nithianantham S, Chandran B, Yu XL, Weinberg A, Gao SJ. Kaposi's sarcoma-associated herpesvirus induces rapid release of angiopoietin-2 from endothelial cells. J Virol 2013; 87:6326-35; PMID:23536671; http://dx.doi.org/10.1128/JVI.03303-12
- An FQ, Folarin HM, Compitello N, Roth J, Gerson SL, McCrae KR, Fakhari FD, Dittmer DP, Renne R. Long-term-infected telomerase-immortalized endothelial cells: a model for Kaposi's sarcoma-associated herpesvirus latency in vitro and in vivo. J Virol 2006; 80:4833-46; PMID:16641275; http://dx.doi.org/10.1128/JVI.80.10.4833-4846.2006
- Brulois KF, Chang H, Lee AS, Ensser A, Wong LY, Toth Z, Lee SH, Lee HR, Myoung J, Ganem D, et al. Construction and manipulation of a new Kaposi's sarcoma-associated herpesvirus Bacterial artificial chromosome clone. J Virol 2012; 86:9708-20; PMID:22740391; http://dx.doi.org/10.1128/JVI.01019-12
- Fiedler U, Scharpfenecker M, Koidl S, Hegen A, Grunow V, Schmidt JM, Kriz W, Thurston G, Augustin HG. The Tie-2 ligand angiopoietin-2 is stored in and rapidly released upon stimulation from endothelial cell Weibel-Palade bodies. Blood 2004; 103:4150-56; PMID:14976056; http://dx.doi.org/10.1182/blood-2003-10-3685
- Etoh T, Inoue H, Tanaka S, Barnard GF, Kitano S, Mori M. Angiopoietin-2 is related to tumor angiogenesis in gastric carcinoma: possible in vivo regulation via induction of proteases. Cancer Res 2001; 61:2145-53; PMID:11280779
- Biel NM, Siemann DW. Targeting the Angiopoietin-2/Tie-2 axis in conjunction with VEGF signal interference. Cancer Lett 2014; S0304-3835(14):00576-X.
- Gerald D, Chintharlapalli S, Augustin HG, Benjamin LE. Angiopoietin-2: an attractive target for improved antiangiogenic tumor therapy. Cancer Res 2013; 73:1649-57; PMID:23467610; http://dx.doi.org/10.1158/0008-5472.CAN-12-4697
- Boshoff C, Chang Y. Kaposi's sarcoma-associated herpesvirus: a new DNA tumor virus. Annu Rev Med 2001; 52:453-70; PMID:11160789; http://dx.doi.org/10.1146/annurev.med.52.1.453
- Felcht M, Luck R, Schering A, Seidel P, Srivastava K, Hu JH, Bartol A, Kienast Y, Vettel C, Loos EK, et al. Angiopoietin-2 differentially regulates angiogenesis through Tie-2 and integrin signaling. J Clin Invest 2012; 122:1991-2005; PMID:22585576; http://dx.doi.org/10.1172/JCI58832
- Hakanpaa L, Sipila T, Leppanen VM, Gautam P, Nurmi H, Jacquemet G, Eklund L, Ivaska J, Alitalo K, Saharinen P. Endothelial destabilization by angiopoietin-2 via integrin β1 activation. Nat Commun 2015; 6:5962; PMID:25635707; http://dx.doi.org/10.1038/ncomms6962
- Murdoch C, Tazzyman S, Webster S, Lewis CE. Expression of Tie-2 by human monocytes and their responses to angiopoietin-2. J Immunol 2007; 178:7405-11; PMID:17513791; http://dx.doi.org/10.4049/jimmunol.178.11.7405
- Venneri MA, De Palma M, Ponzoni M, Pucci F, Scielzo C, Zonari E, Mazzieri R, Doglioni C, Naldini L. Identification of proangiogenic TIE2-expressing monocytes (TEMs) in human peripheral blood and cancer. Blood 2007; 109:5276-85; PMID:17327411; http://dx.doi.org/10.1182/blood-2006-10-053504
- Coffelt SB, Chen YY, Muthana M, Welford AF, Tal AO, Scholz A, Plate KH, Reiss Y, Murdoch C, De Palma M, et al. Angiopoietin 2 stimulates TIE2-expressing monocytes to suppress T cell activation and to promote regulatory T cell expansion. J Immunol 2011; 186:4183-90; PMID:21368233; http://dx.doi.org/10.4049/jimmunol.1002802
- Scholz A, Lang V, Henschler R, Czabanka M, Vajkoczy P, Chavakis E, Drynski J, Harter PN, Mittelbronn M, Dumont DJ, et al. Angiopoietin-2 promotes myeloid cell infiltration in a β(2)-integrin-dependent manner. Blood 2011; 118:5050-59; PMID:21868579; http://dx.doi.org/10.1182/blood-2011-03-343293
- Corliss BA, Azimi MS, Munson J, Peirce SM, Murfee WL. Macrophages: An Inflammatory Link between Angiogenesis and Lymphangiogenesis. Microcirculation 2015; 23:95-121; http://dx.doi.org/10.1111/micc.12259
- Komohara Y, Niino D, Ohnishi K, Ohshima K, Takeya M. Role of tumor-associated macrophages in hematological malignancies. Pathol Int 2015; 65:170-76; PMID:25707506; http://dx.doi.org/10.1111/pin.12259
- Atkins MB, Gravis G, Drosik K, Demkow T, Tomczak P, Wong SS, Michaelson MD, Choueiri TK, Wu B, Navale L, et al. Trebananib (AMG 386) in Combination With Sunitinib in Patients With Metastatic Renal Cell Cancer: An Open-Label, Multicenter, Phase II Study. J Clin Oncol 2015; 33:3431-38; PMID:26304872; http://dx.doi.org/10.1200/JCO.2014.60.6012
- Eroglu Z, Stein CA, Pal SK. Targeting angiopoietin-2 signaling in cancer therapy. Expert Opin Investig Drugs 2013; 22:813-25; PMID:23621441; http://dx.doi.org/10.1517/13543784.2013.793306
- Gaitskell K, Martinek I, Bryant A, Kehoe S, Nicum S, Morrison J. Angiogenesis inhibitors for the treatment of ovarian cancer. Cochrane Database Syst Rev 2011; 9:CD007930.
- Neal J, Wakelee H. AMG-386, a selective angiopoietin-1/-2-neutralizing peptibody for the potential treatment of cancer. Curr Opin Mol Ther 2010; 12:487-95; PMID:20677100
- Ye FC, Lattif AA, Xie JP, Weinberg A, Gao SJ. Nutlin-3 induces apoptosis, disrupts viral latency and inhibits expression of angiopoietin-2 in Kaposi's sarcoma tumor cells. Cell Cycle 2012; 11:1393-99; PMID:22421142; http://dx.doi.org/10.4161/cc.19756
- Ensley JF, Maciorowski Z, Pietraszkiewicz H, Klemic G, KuKuruga M, Sapareto S, Corbett T, Crissman J. Solid tumor preparation for flow cytometry using a standard murine model. Cytometry 1987; 8:479-87; PMID:2444398; http://dx.doi.org/10.1002/cyto.990080508