ABSTRACT
The centrosome is the major microtubule-organizing center in animal cells but is dispensable for proper microtubule spindle formation in many biological contexts and is thus thought to fulfill additional functions. Recent observations suggest that the centrosome acts as a scaffold for proteasomal degradation in the cell to regulate a variety of biological processes including cell fate acquisition, cell cycle control, stress response, and cell morphogenesis. Here, we review the body of studies indicating a role for the centrosome in promoting proteasomal degradation of ubiquitin-proteasome substrates and explore the functional relevance of this system in different biological contexts. We discuss a potential role for the centrosome in coordinating local degradation of proteasomal substrates, allowing cells to achieve stringent spatiotemporal control over various signaling processes.
Introduction
Biological processes rely on a vast array of signaling and information-processing networks operating at the cellular and molecular level. Though a multitude of signaling strategies have been identified and well-characterized over the past half-century, it remains unclear how they unfold in space and time Centrosomes are well-known for their role in promoting timely separation of mitotic chromosomes, but are also emerging as potential hubs for cell signaling components, particularly those that rely on the ubiquitin-proteasome system (UPS).Citation1,2 Consistent with as yet unidentified roles in signaling and proteostasis, we recently found that a C. elegans transcriptional co-activator, the β-catenin SYS-1, is negatively regulated in a cell-cycle dependent manner by localizing to centrosomes during mitosis.Citation3 Here, we review additional studies that suggest an intriguing role for the centrosome as a physical scaffold for concentrating protein degradation and associated regulatory events during cell signaling. The centrosome is the major microtubule-organizing center (MTOC) in animal cells. It is composed of 2 centrioles –bilaterally symmetric cylindrical appendages made of short microtubule triplets - surrounded by a pericentriolar material (PCM) – a proteinaceous matrix composed of microtubule-anchoring γ-tubulin ring complexes (γ-TuRCs), filamentous scaffolds, and various mitotic regulators.Citation1,4 Centrosomes are highly dynamic non-membrane-bound organelles whose composition, activity, and behavior can be ultimately controlled by a number of different upstream intracellular cues such as cell cycle status and cell stress.Citation5,6 Given the centrosome's long-standing, canonical role as a microtubule-organizing center, proteomic analysis of purified centrosomes identify a surprisingly broad list of centrosomal proteins whose functional characterization resist – with even the most generous speculation - placement into any category even tangentially related to microtubule behavior.Citation7,8 These data, if assumed biologically relevant, suggest centrosomes may confer additional functional roles in biology.
Centrosome activity and behavior changes with the cell cycle
The composition and activity of the centrosome is dynamic and ultimately controlled by cell cycle regulatory factors. A newly divided cell inherits one centrosome. During G1 to S-phase transition, new daughter centrioles are formed orthogonally to each mother centriole to produce 2 centrosomes, each with its own PCM and hybrid mother/daughter centriole pair. Throughout interphase, the centrosomes remain tethered via proteinaceous linkers.Citation9 Cell cycle regulators that trigger mitotic entry promote cleavage of these linkers, allowing microtubule-based motors to drive the 2 centrosomes to opposite poles of the cell.Citation10,11 Mitotic entry also results in a dynamic elaboration of centrosome structure during a process called centrosome maturation in which a physical expansion of the PCM and associated factors triggers an increase in microtubule-nucleating activity.Citation12,13 Surrounding the centrioles, the interphase PCM is supported by a structured series of concentrically-arranged toroidal rings rich in coiled-coil domain scaffold proteins such as Pericentrin, Cep192, and CDK5RAP2.Citation14 While the substructure of the mitotic PCM is less clear, it has been demonstrated that its maturation is cued by phosphorylation of Pericentrin by the mitotic kinase Plk1 to allow further recruitment of Cep192, γ-tubulin, and other core components.Citation12 Properly separated mature centrosomes organize the bipolar mitotic spindle to ensure proper chromosome segregation and successful cell division.Citation2 In post-mitotic cells, the centrosome can be targeted to the cell cortex, and components of the mother centriole may dock at the plasma membrane to form the primary cilium.Citation15 As centrosome activity dynamically changes according to the cell cycle status of the cell, it seems plausible that proteasomes or substrates associated with the centrosome may also retain this property, a possibility we address in this manuscript.
The ubiquitin-proteasome system in eukaryotes
The ubiquitin-proteasome system is a major protein degradation pathway in eukaryotic cells and is largely responsible for clearance of undesired protein molecules. For a protein fated for degradation, passage through the ubiquitin-proteasome pathway begins with covalent attachment of the small protein tag ubiquitin. A monomer of ubiquitin enters into the UPS pathway upon forming an ATP-dependent isopeptide bond with an E1 activating enzyme before being covalently attached to an E2 conjugating enzyme. An E3 ligase complex then catalyzes the transfer of ubiquitin from the E2 enzyme to a lysine residue on the target substrate. In this manner, additional ubiquitin monomers can be linked to the substrate's original conjugate to from polyubiquitin chains.Citation16 Polyubiquitinated proteins are subject to proteolysis by the 26S proteasome, a large 2.5Mda macromolecular assembly.Citation17 The 26S proteasome is composed of 2 complexes: (1) the 20S catalytic subunit, a cylindrical complex with an internal core equipped with various forms of peptidase activity and (2) the 19S regulatory subunit, a ringed amalgamation of scaffolds, ubiquitin-receptors, and ATPases that cooperate to recognize, unfold, and ultimately translocate substrates into the 20S core.Citation18
Early reports of centrosome-associated degradation
Preliminary evidence of centrosome-associated degradation emerged when indirect immunofluorescence experiments using antibodies against proteasomal antigens stained spindle poles in dividing cells and detergent-insoluble perinuclear structures in interphase cells.Citation19 This pattern was further characterized when Wigley et al first showed in 1999 that the 20S and 19S proteasomal complexes both displayed punctate enrichment at γ-tubulin-labeled centrosomes in HEK293 and HeLa cells.Citation20 The fact that a number of well-characterized proteasomal substrates shared this localization pattern suggested that degradation of substrates by active proteasomes is concentrated at the centrosomes.Citation21-23 Consistent with this idea, Fabunmi et al, 2000 showed that purified centrosomes from HeLa cells were able to degrade ubiquitinated substrates and proteasome-specific peptides in a manner that was ATP-dependent yet sensitive to proteasome inhibitors. Purified centrosomes also retained the ability to cleave single ubiquitins from a polyubiquitin chain linked to a substrate, a general feature of 19S proteasome subunits.Citation24 Thus, centrosome fractions display the same enzymatic profile as intact, active proteasomes. Intriguingly, many of these early studies showed that inhibition of proteasome activity led to redistribution of cytosolic proteasomes to the centrosome, suggesting a mechanism to concentrate degradation specifically at the centrosome when proteasome substrates were in excess.Citation20,24, 25
Concurrent with these key discoveries were reports demonstrating that large centrosome-associated protein aggregates could form in response to either proteasome inhibition or overexpression of misfolded proteins. Overexpression of inefficiently-folded alleles of cystic fibrosis transmembrane conductance regulator (CFTR) or presenilin-1 in HEK293 cells leads to CFTR accumulation in large inclusion bodies at the centrosome, an effect exacerbated upon proteasomal inhibition.Citation22 These structures, dubbed 'aggresomes', are composed of electron-dense protein aggregates that depend on minus-end-directed microtubule trafficking for accumulation at centrosomes.Citation26 Aggresome formation depends on the histone deacetylase HDAC6 which simultaneously binds polyubiquitinated unfolded proteins and dynein motors to participate in active trafficking of aggregate particles to the centrosome.Citation27 Aggresomes are rich in ubiquitin, 19S and 20S proteasome subunits, and the chaperones Hsp70 and Hsp90.Citation28 Additionally, aggresome formation triggers redistribution of vimentin intermediate filaments from cytoplasmic networks to a cage-like structure surrounding the aggresome core.Citation22 Thus, when the capacity of the proteasome is exceeded, as in cases where toxic proteins are overexpressed,Citation29 a dramatic cellular response involving cytoskeletal reorganization and trafficking events ensures that misfolded/damaged proteins are sequestered along with ubiquitin-proteasome and other quality-control components specifically at the centrosome.
Aggregated proteins that bind to the 19S regulatory complex but resist entry into the 20S core cannot be subject to proteolysis and may inhibit the proteasome.Citation30 Therefore, aggresomes are largely thought to be cleared by autophagy rather than proteasome-mediated degradation.Citation31 Since proteasomes are unable to process aggregates, the fact that they are actively targeted to aggresomes presents a paradox. It was recently demonstrated that proteasomes play a role in aggresome clearance not by catalyzing substrate degradation but by removing ubiquitin chains from polyubiquitinated protein aggregates.Citation32 Freed from their aggregated substrates, accumulation of unconjugated polyubiquitin chains triggers activation of the autophagy pathway. Thus, centrosomal proteasomes may act as substrate sensors, using their non-degradative enzymatic activities to influence the ubiquitin profile of the cell, provoking an alternative degradation strategy. Since many neurodegenerative disorders involve aggregation of cytotoxic proteins, it is not surprising that aggresomes and aggresome-like inclusions appear in several disease states such as Parkinson,Citation27 Alzheimer,Citation33 Huntington's,Citation34 and multiple myeloma.Citation35 Future studies elucidating the explicit role of the centrosome in coordinating cellular response to unfolded protein stress should provide important insights into the pathophysiology of diseases vulnerable to defects in protein degradation.
Less clear is the capacity of centrosome-associated proteasomes to degrade substrates in the absence of proteotoxic stress, although several reviews suggest such a mechanism may be operational during basal physiological conditions.Citation28,36,37 Indeed, isolated examples of centrosomally-degraded substrates involved in a host of biological processes continue to emerge, which we discuss below (Individual examples covered in this review are shown in ).
Figure 1. Components of the ubiquitin-proteasome system and substrates localize to the centrosome (composed of the marked PCM and centrioles) in a conserved manner. Representative examples. of ubiquitin-proteasome components (shown above the dashed line) and substrates (shown below the dashed line) are color-coded according to the organism in which centrosomal localization was observed. Citations for individual reports are provided in brackets.
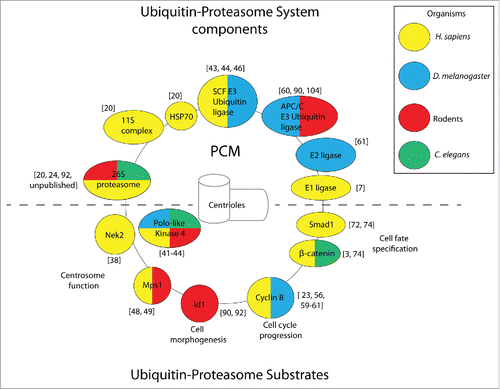
Centrosome function depends on the proteasome
Virtually all aspects of centrosome behavior and organization are under stringent control by the proteasome (). In HeLa cells, inhibition of the proteasome leads to overaccumulation of a number of different centrosomal proteins such as γ-tubulin, ninein, pericentrin, dynactin and PCM1.Citation38 As a consequence of misregulation of core centrosomal factors, cells lacking proteasome activity are severely deficient in microtubule growth and organization, harboring ectopic sites of microtubule nucleation and shrunken microtubule asters around centrosomes. Transmission electron micrographs of wild type cells exhibit a characteristic anchoring of microtubules at the PCM; however, discernable sites of microtubule anchorage within the PCM are lost with proteasome inhibition, suggesting that the proteasome limits the dosage of critical centrosomal factors necessary for normal MTOC function.Citation38
The cell-cycle regulated centrosomal kinase Nek2 is responsible for regulating centrosome separation at G2/M transition. Nek2 exhibits a dynamic pattern of recruitment to the centrosome with upwards of 70% of centrosome-bound Nek2 subject to rapid turnover in fluorescence recovery after photobleaching experiments. Interestingly, dynamic recruitment of new Nek2 molecules depends on its degradation since inhibition of the proteasome or observing a non-degradable Nek2 allele both lead to an attenuated pattern of recovery.Citation39 This experiment strongly suggests that the centrosomal pool of Nek2 is subject to active degradation by the proteasome. Since Nek2 activity at the centrosome is primarily exerted at G2/M transition, it is possible that degradation of excess Nek2 at centrosomes at other cell cycle stages limits this activity to prevent untimely centrosome splitting.
More extensively characterized is the highly conserved role of proteasomal degradation in limiting centriole duplication to a single event at G1/S transition in eukaryotic cells. Centriole duplication is initiated by kinase cascades under the regime of cell cycle regulators.Citation40 Polo-like-kinase 4 (Plk4) is a serine/threonine kinase that promotes centriole duplication during S-phase.Citation40,41 Plk4 is rapidly turned over and its expression is tightly regulated by a Skip/Cullin/F-box (SCF)-class E3 ubiquitin ligase and the 26S proteasome.Citation42-44 The substrate adaptor component of SCF ligase in flies, Slimb, binds to a Plk4 phosodegron to target the protein for degradation. Slimb localizes to centrosomes throughout the cell cycle and restricts levels of centrosomal Plk4 at S-phase. This pattern of precise spatiotemporal regulation of Plk4 dosage prevents any residual Plk4 activity from triggering an undesired reduplication of centrioles.Citation43,44 Given that Plk4 is overexpressed in over a quarter of human tumors, it is rapidly emerging as a potential cancer target, and suppression of Plk4 reduces cell proliferation in a number of breast cancer cell lines.Citation45 A more comprehensive understanding of centrosome-specific degradation pathways may allow for novel therapeutic strategies that modulate degradation of substrates specifically at the centrosome without disrupting the bulk of total cellular ubiquitin-proteasomal degradation.
Consistent with regulation of Plk4 by centrosome-bound Slimb in flies, localization of core SCF components to centrosomes has also been reported in multiple human cell lines, revealing the possibility that centrosomal targeting of this essential ubiquitin ligase complex is a conserved and functionally relevant feature of cycling cells.Citation46 Given the aforementioned reports of active, centrosome-bound 26S proteasomes, it seems likely that the centrosome harbors its own “in-house” degradation system equipped to limit its own protein content, especially in the case of proteins that may perturb centrosome functionality when in excess. An “in-house” centrosomal degradation system would presumably allow cells to specify an optimal centrosome composition without having to fine tune overall cellular levels of every individual centrosomal protein to fulfill a stoichiometric demand. Since centrosomal factors may be multifunctional, locally-acting mechanisms restrict their accumulation specifically at centrosomes without affecting their abundance in other subcellular locales. This phenomenon has been demonstrated in principle by observations of the centrosomal kinase monopolar spindle 1 (Mps1). Mps1, a target of the proteasome, localizes to centrosomes, nuclear pores and to kinetochores, where, in the latter, it mediates the spindle assembly checkpoint during mitosis.Citation47-49 During G1/S transition, centrosomal Cyclin A-Cdk2 stabilizes Mps1 to promote centriole duplication. Overexpression of Cyclin A or inhibition of the proteasome result in over-accumulation of Mps1 specifically at centrosomes. Conversely, depletion of Cyclin A results in loss of centrosomal Mps1 while having very little effect on the nuclear pore-associated pool or whole cell levels.Citation48 Thus, centrosome-associated proteasomes may allow cells to confine degradation to the centrosomal subpopulation of a multifunctional protein, leaving other subcellular pools and associated processes intact. It is also worth noting that centrosomal Mps1 is degraded by an ubiquitin-independent proteasomal degradation mechanism mediated by Antizyme, a highly conserved protein that localizes to the centrosome, binds non-ubiquitinated substrates and targets them to the proteasome for degradation.Citation50,51 Loss of Antizyme-mediated Mps1 degradation results in centrosome amplification and contributes to aberrant MTOC activity and subsequent aneuploidy in human melanomas.Citation52 Antizyme regulation of centrosome duplication via Mps1 degradation suggests diverse modes of proteasome-mediated degradation, including ubiquitin-independent proteolysis, are utilized by centrosome-associated proteasomes.Citation53
Centrosome-association of rapidly-degraded cell cycle regulatory factors
Progression through mitosis requires thorough spatiotemporal regulation of mitotic cyclins by the ubiquitin-proteasome system. A well-characterized prerequisite for metaphase to anaphase transition (MAT) is the ubiquitination of cyclin B by the multi-subunit E3 ligase anaphase promoting complex/cyclosome (APC/C) and degradation by the proteasome.Citation54,55 Cyclin B has been reported to localize to mitotic centrosomes in multiple systems, raising the possibility that centrosome-associated proteasomal degradation may play a role in regulating cyclin B levels during MAT.Citation56,57 This notion is well-supported by several experiments. One preliminary hint emerged from elegant cell fusion experiments that generated cells containing 2 mitotic spindles. Upon fusion, spindles at different mitotic stages initiated anaphase asynchronously, invoking the possibility that each spindle, despite sharing a common cytoplasm, exerts local, autonomous control over cyclin B degradation to cue its own mitotic exit.Citation58 This idea was reinforced by subsequent in vivo studies in flies and human cells, revealing that cyclin B follows a dynamic spatiotemporal pattern of regulation during mitosis. Cyclin B::GFP is concentrated at the centrosomes and spindle until late metaphase. Prior to anaphase entry, cyclin B degradation progresses in a stepwise pattern, disappearing along the spindle in a pattern that begins at the centrosomes and ends at the metaphase plate.Citation23,59 In this way, cyclin B initially disappears from centrosomes and is gradually restricted to the spindle equator (). Consistent with degradation of cyclin B at centrosomes is the observation that key components of APC/C also localize to the centrosomes and spindle throughout mitosis. A non-degradable allele of cyclin B obtained by mutating its APC/C binding sites persists on centrosomes throughout mitosis, suggesting cyclin B removal from metaphase centrosomes involves its polyubiquitination and subsequent degradation.Citation60
Figure 2. Centrosome-associated degradation pathways. A) Centrosome and spindle-associated degradation of Cyclin B. Top panel: At metaphase, degradation of cyclin B begins at centrosomes in a step-wise pattern that propagates to the mitotic spindle in a direction toward the spindle equator and is effectively cleared prior to anaphase entry. Bottom panel: Centrosome-bound cyclin B is polyubiquitinated (small red circles represent ubiquitin tags) by the E3 ubiquitin ligase APC/C and the E2 ubiquitin conjugating enzyme Vihar, marking it for degradation by the proteasome. Vihar is polyubiquitinated and marked for degradation by APC/C, resulting in a negative feedback loop on APC/C ubiquitination of cyclin B. (B) Centrosome-associated degradation of SYS-1 during C. elegans asymmetric cell division. Top panel: SYS-1 localizes to centrosomes, where it is subject to dynamic turnover via the proteasome during division and is asymmetrically distributed between daughter cell nuclei after division. Middle panel: When SYS-1 centrosomal localization is disrupted by rsa-2 (RNAi), its degradation is attenuated during division, resulting in elevated SYS-1 levels in both daughter cell nuclei. Bottom panel: When the proteasome is inhibited, SYS-1 accumulation at centrosomes is increased and its turnover is decreased. In the absence of the proteasome, SYS-1 protein avoids negative regulation during asymmetric division and is severely overaccumulated in both daughter cells. (C) Centrosomal degradation pathways control dendrite growth in neurons. CaMKIIβCitation104 binds centrosomes by interaction with PCM1 and phosphorylates the APC/C component Cdc20, causing it to dissociate from centrosomes. Unphosphorylated Cdc20 localizes to centrosomes and polyubiquitinates the helix-loop-helix protein Id1 targeting it for degradation by the centrosomal proteasome. In the absence of negative regulation by Cdc20 and the proteasome, Id1 retracts dendrites. Id1 negatively regulates proteasomes by preventing association of S5a with the 19S complex, potentially autoregulating its own degradation.
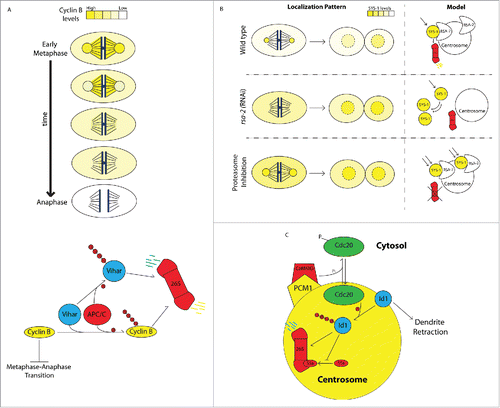
APC/C-mediated cyclin B degradation requires Vihar, an E2 ubiquitin-conjugating enzyme that also localizes to centrosomes during metaphase before disappearing as mitosis progresses (). Loss of Vihar results in increased levels of cyclin B and severe overaccumulation at mitotic centrosomes. Overexpression of Vihar, on the other hand, leads to loss of centrosomal cyclin B. These two lines of evidence indicate that this E2 ligase is responsible for concentrating initial cyclin B degradation at centrosomes.Citation61 Vihar, itself, is targeted for degradation via polyubiquitination by APC/C and disappears from centrosomes upon entry into anaphase. APC/C, therefore, autoregulates its ability to polyubiquitinate centrosomal cyclin B by promoting degradation of its cofactor Vihar. It is thought that this feedback mechanism terminates the first ‘wave’ of cyclin B degradation at the centrosomes before degradation of cyclin B progresses to the spindle, presumably mediated by other E2 ligases.Citation23 That cyclin B and several of its negative regulators transiently associate with the centrosome immediately preceding initiation of cyclin B degradation strongly suggests that both ubiquitination and degradation are actively concentrated at centrosomes. It is tempting to speculate that localization of cyclin B and its regulatory factors to the mitotic apparatus brings them in close physical proximity to spindle components to allow cells to rapidly pause mitosis in response to spindle defects by quickly inhibiting cyclin B degradation. In fact, the centrosome may play a role in coordinating degradation of spindle-associated cyclin B, a possibility that was raised by observations of an intriguing mutant isolated in Drosophila called centrosome fall off (cfo). Centrosomes in cfo mutants become physically detached from the mitotic spindle. In cfo mutants, initial cyclin B degradation at centrosomes proceeds as usual but fails to propagate to the mitotic spindle.Citation62 These data confirm that there are 2 discreet ‘waves’ of cyclin B degradation, and that progression from the first wave to the second requires physical contact between the spindle and centrosomes.
Another function of this centrosome-associated degradation pathway may be to ensure timely and efficient clearance of cyclin B. Indeed, thorough clearance of cyclin B is absolutely required for irreversible commitment to MAT.Citation63 To ensure all chromosomes are tightly bound to kinetochore microtubules before allowing chromatid separation, cyclin B degradation can be inhibited by a spindle assembly checkpoint (SAC) pathway that monitors kinetochore-microtubule attachment and tension.Citation64 This pathway must be disabled upon entry into anaphase, so as not to mistake loosely-secured kinetochores in metaphase chromosomes for those in actively-separating anaphase chromatids, in which tension has been released due to chromatid separation.Citation65 Desensitizing the cell to this surveillance mechanism is accomplished, at least in part, through complete removal of cyclin B by late anaphase. This was shown by a recent study that relied on small cyclin B deletions to experimentally reduce its degradation efficiency. The results showed that even a modest amount of residual cyclin B in anaphase can trigger SAC reactivation, in turn, stabilizing any leftover cyclin B and shutting down mitotic progression.Citation66 Clearly, mitotic cells must guarantee thorough and timely cyclin B clearance to irreversibly shut down SAC, a task that may be ensured by actively concentrating cytoplasmic cyclin B to the centrosomes just prior to the initiation of degradation. Probing the mechanisms by which cyclin B is targeted to the centrosome would allow further studies to test the functional relevance of cyclin B centrosomal enrichment on both its rate of degradation and its dynamic spatiotemporal regulation during mitosis.
Centrosome-associated degradation of cell fate determinants
Cell differentiation during development relies on extensive transcriptional reprogramming, in which the activity of downstream transcription factors is controlled by networks of cell signaling pathways. Under default conditions, terminal effectors of many cell signaling pathways experience both high production and degradation rates when at steady state, such that even slight modulation of either production or degradation can trigger rapid increase or decrease in intracellular concentration, respectively. Key transcriptional regulators of cell fate exhibit half-life values on the scale of minutes to a few hours. These include p53Citation67 (<20 minutes), β-cateninCitation68 (∼1hr), and the Hippo pathway transcription factor Yap (1hr-2.5hr).Citation69,70 This pattern of constitutive production and degradation may represent a strategy to optimize signaling pathway response time by allowing for maximum responsiveness at the time of signal acquisition, but not long periods after signaling events have ceased.Citation71 Timely and efficient degradation of signaling effectors is critical for cell fate specification, and the regulation of cell fate-determining factors by centrosomal degradation pathways has been reported in several studies.
Smad1 is a transcription factor that mediates TGFβ signaling events utilized during key vertebrate cell fate specification processes such as dorsal-ventral axis determination by BMP. Phosphorylation by the kinase GSK3 targets Smad1 for polyubiquitination and degradation by the proteasome. In Cos7 cells, phosphospecific antibodies against GSK3-phosphorylated Smad1 show enriched staining at centrosomes, a pattern dramatically enhanced by inhibition of the proteasome. This visualization of the degradation-fated pool of Smad1 indicates that its degradation is rate-limiting and likely concentrated at centrosomes. The latter notion is further confirmed by localization of the proteasome subunit S20α5 and polyubiquitin to the centrosomes in this cell line.Citation72 Subsequent experiments revealed that phospho-Smad1 localized asymmetrically to mitotic centrosomes in human embryonic stem cells (hESCs). Phospho-Smad1 also colocalized with centrosomal microtubules. Interestingly, depolymerization of microtubules by nocodazole treatment led to increased phospho-Smad1 levels, a preliminary indication that proper degradation of pSmad1 requires microtubule-dependent transport to the centrosome. GSK3-phosphorylated β-catenin (another substrate marked for degradationCitation73) and polyubiquitinated proteins showed the same localization pattern during hESC division, suggesting that association with mitotic centrosomes may be a general feature of various proteasome-fated substrates.Citation74
We recently showed that C. elegans embryos use such a strategy to limit levels of SYS-1/β-catenin during asymmetric cell division.Citation3 SYS-1/β-catenin is a transcription factor acting downstream of the Wnt signaling pathway to activate target genes necessary for cell fate specification.Citation75-82 During asymmetric division, SYS-1 is targeted to mitotic centrosomes by its interaction with the centrosomal protein RSA-2.Citation83 Disrupting SYS-1 centrosomal localization by depletion of RSA-2 leads to increased SYS-1 retention by daughter cells after division, indicating the presence of a negative regulatory mechanism involving centrosomal targeting. Photobleaching experiments show that the entire centrosomal SYS-1 population turns over on the scale of few minutes, a common feature of centrosomal proteins.Citation39,84 Interestingly, inhibition of the proteasome not only causes a severe increase in SYS-1 specifically at centrosomes but also attenuates its turnover rate, suggesting that centrosomal SYS-1 is actively degraded (). This centrosome-associated degradation mechanism may represent a strategy to efficiently degrade SYS-1 during division, thereby limiting its retention by daughter cells.Citation3 Thus, regulation of SYS-1 may be coupled to the cell cycle by localization to mitotic centrosomes and subsequent degradation.
Can centrosome-associated degradation broadly link cellular processes such as the cell cycle and cell signaling? We have found that, in addition to enriched centrosomal polyubiquitin, Rpt4/S10b, an ATPase subunit of the proteasome lid, also localizes to mitotic centrosomes during C. elegans embryogenesis, indicating conservation of proteasome targeting to centrosomes from humans to nematodes (unpublished data). This temporally and spatially restricted Rpt4 pattern suggests that a local increase of proteasome function at the mitotic centrosome may directly link the cell cycle to enhanced substrate degradation through PCM accumulation, and that additional targets of this mechanism await discovery. Like vertebrate β-catenin,Citation73 SYS-1 protein is likely subject to high turnover rate and is tightly regulated by Wnt signaling to allow precise control over cellular concentration. Recent in vivo observations demonstrate that a range of relative concentrations of various Wnt signaling components including SYS-1 and its cofactor TCF can produce a continuum of signaling strengths throughout C. elegans embryogenesis.Citation85 The explicit role of centrosome-associated degradation in modulating the dosage of SYS-1 and other clients in various developmental contexts remains a challenge for future studies.
Since the size of the centrosome increases dramatically during PCM maturation, it is possible that centrosome-associated degradation mechanisms can be used as a strategy to synchronize protein degradation with the cell division. A recent mass spectrometry-based characterization of S-phase and M-phase centrosomes showed centrosomal accumulation of polyubiquitinated proteins specifically during mitosis. Depletion of the endogenous proteasome inhibitor Ecm29Citation86 led to a significant reduction in polyubiquitinated proteins at the centrosome, supporting the view that these substrates may be actively degraded by centrosomal proteasomes.Citation87 While proteasomal activity is roughly the same between S-phase and M-phase centrosomes, centrosomal targeting of polyubiquitinated proteins increases during maturation, demonstrating that other substrates may share this mode of division-coupled regulation. Together, these studies suggest that centrosome-associated degradation strategies control various signaling factors during mitotic divisions, linking pathway activity to the cell cycle.
Local degradation by centrosomal proteasomes controls neuronal morphology
Neurons are morphologically elaborate post-mitotic cells that can dynamically control the behavior of cellular appendages to establish and reorganize cell-cell contacts. This remarkable property is thought to be achieved by confining basic biological processes like translation, vesicular trafficking, and protein degradation to local subcellular domains.Citation88,89 Several studies have revealed a role for centrosome-associated degradation in neuronal dendrite elaboration. In rat cerebellar granule neurons, the APC/C subunit Cdc20 promotes dendrite growth and arborization by marking the centrosomal helix-loop-helix protein Id1 for degradation.Citation90 Cdc20, itself, binds centrosomes through a 19-amino acid centrosomal localization domain, a region that is necessary for its function in dendrite arborization. Importantly, Cdc20 lacking its native centrosomal localization domain can be driven to centrosomes upon fusion with the conserved centrosome-targeting domain PACT, which rescues its function. These experiments elegantly demonstrate that Cdc20s functional contribution to promoting proper dendrite elaboration depends explicitly on its local activity at the centrosome.Citation90,91 More recently, it was shown that the proteasomal subunit S5a/Rpn10, an ubiquitin receptor that binds substrates to the 19S proteasome complex, stimulates local degradation by centrosomal proteasomes in order to promote dendrite growth. Id1 negatively regulates centrosomal proteasomes by preventing the association of S5a to the 19S complex, demonstrating that centrosome-associated degradation mechanisms in cerebellar neurons can be subject to negative regulation to modulate dendrite extension/retraction.Citation92 That Id1, itself, is both a target and a regulator of the centrosomal proteasome suggests that dendrite growth may be controlled by an autoregulatory feedback loop that modulates local proteasome activity. These studies compellingly demonstrate that local proteasomal degradation imposed on as-yet-unidentified substrates contributes to growth dynamics of certain subcellular appendages (). These studies also raise implications about the potential of centrosome-associated degradation in controlling subcellular organization in other contexts if additional, well-established observations of centrosome positioning are considered. Given that centrosomes have been shown to act as polarity cues in multiple systemsCitation93,94 and tend to assume stereotyped subcellular positions in a number of differentiated cell types (epithelial cells, migrating fibroblasts),Citation95 it could be imagined that centrosome-associated degradation may be used as a strategy to locally deplete polarity-determining factors from certain subcellular domains in order to contribute to intracellular organization.
Centrosome-associated degradation in cells of the immune system
In addition to neurons, there are reports of centrosome-associated degradation mechanisms in various cells of the immune system. Centrosomes are known to participate in molecular mechanisms underlying immune system function, most notably in the formation of cytotoxic immunological synapses. Activation of cytotoxic T lymphocytes upon binding of an antigen presenting cell is followed by centrosome migration and physical docking at the site of cell-cell contact.Citation96 This dynamic polarization event is required for the formation of the immunological synapse (IS), an integrin-rich cell-cell adhesion that allows the T cell to secrete lytic granules exclusively to its target. Thus, docking of the centrosome to the contact site allows not only specification of the IS site but also active, microtubule-dependent trafficking of secretory materials.Citation94
It was recently shown that leukocytes from patients with elevated body temperature harbor damaged centrosomes with decreased γ-tubulin levels.Citation97 Cells in culture also display this feature after heat stress.Citation97,98 Centrosomes in these cells are deficient in microtubule nucleation, cilia assembly, and fail to retain proper levels of core components such as γ-tubulin, pericentrin, PCM1, and centrin. Heat-stress induced centrosome inactivation is dependent on degradation of centrosomal proteins by the centrosomal proteasome since a centrosomally-targeted dominant negative Rpn10 allele rescues centrosomes upon expression in heat-stressed cells. This mechanism of centrosome inactivation is likely to be physiologically relevant to the immune system because both centrosome docking and immunological synapse formation are compromised Jurkat cells after heat-stress.Citation97
Earlier studies using in vitro models of immune response suggest that centrosomes may act as sites of antigen processing. Proteins from invading pathogens are processed by modified proteasomes into short peptides to be displayed on the cell surface for presentation to cytotoxic T cells via MHC class I molecules.Citation99 During immune response, signaling via the cytokine IFNγ induces expression of PA28, an alternative regulatory complex that associates with a modified 20S proteasome core to form the immunoproteasome.Citation100 Interestingly, components of the PA28 complex have previously been localized to the centrosome.Citation20 A few examples of centrosome-associated degradation have been elucidated in the context of antigen processing. The influenza virus nucleoprotein, from which antigens are derived following infection, is a highly unstable, rapidly-degraded substrate that localizes to centrosomes upon proteasome inhibition in human osteosarcoma cells. Interestingly, restoring proteasome activity while inhibiting protein synthesis in these cells results in the rapid clearance of centrosome-associated nucleoprotein, strongly suggesting that nucleoprotein proteolysis is concentrated at centrosomes.Citation101
Expression of an unstable form of the HIV protein Nef in antigen-presenting cells results in rapid degradation and evokes a cytotoxic T lymphocyte response. This form of Nef localizes to centrosomes in a microtubule-dependent manner in Cos7 cells. Depolymerizing microtubules by nocodazole disrupts Nef centrosomal localization and also leads to decreased antigen presentation of an epitope tagged form of Nef, suggesting that microtubule-based trafficking of Nef to the centrosome plays a role in its antigen processing.Citation102
The most compelling evidence of centrosome-associated antigen processing comes from a study using the human papilloma virus protein E7, a model tumor antigen. When E7 is driven to centrosomes by fusion with γ-tubulin, dendritic cells display increased MHC class I antigen presentation. Furthermore, vaccination of mice with γ-tubulin::E7 or E7 alone demonstrated that fusion to γ tubulin enhances cytotoxic T-cell activation during immune response. Using an E7-expressing tumor model, mice vaccinated with the γ-tubulin::E7 fusion display significantly less pulmonary tumor metastases than mice vaccinated with E7 alone. Together, these studies reveal the centrosome as an essential site for proteasome-dependent antigen processing events using a variety of cell types and models and raise therapeutic implications.Citation103
Concluding remarks
That centrosomal proteasomes regulate the localization and expression of clients involved in a broad range of processes such as centrosome organization, cell cycle progression, cell fate assignment, subcellular morphology, and immune response suggests that centrosome-associated degradation may represent a fundamental biological mechanism key to cell function. The majority of tumor cells display centrosome abnormalities with respect to either copy number or morphology and therefore may misregulate protein stability in addition to protein inheritance after cell division. Thus, it would be of importance to gain an understanding of the functional relevance of centrosomes in regulating the activity of the ubiquitin-proteasome system. In particular, future studies should test whether presence or absence of functional centrosomes influences the activity of the ubiquitin-proteasome system and its clients. Further exploration into this phenomenon will allow movement beyond characterization of the participants and interactions involved in signaling networks toward fostering an understanding of how individual signaling events are coordinated in space and time.
Disclosure of potential conflicts of interest
No potential conflicts of interest were disclosed.
Funding
This research was supported by grants from the American Cancer Society (RSG-11-140-01-DC), the Roy J. Carver Charitable Trust (13-4131), and the National Science Foundation (IOS-1456941).
References
- Bettencourt-Dias M, Glover DM. Centrosome biogenesis and function: centrosomics brings new understanding. Nat Rev Mol Cell Biol 2007; 8:451-63; PMID:17505520
- Badano JL, Teslovich TM, Katsanis N. The centrosome in human genetic disease. Nat Rev Genet 2005; 6:194-205; PMID:15738963
- Vora S, Phillips BT. Centrosome-Associated Degradation Limits beta-Catenin Inheritance by Daughter Cells after Asymmetric Division. Curr Biol 2015; 25:1005-16; PMID:25819561
- Oakley BR, Oakley CE, Yoon Y, Jung MK. Γ-tubulin is a component of the spindle pole body that is essential for microtubule function in Aspergillus nidulans. Cell 1990; 61:1289-301; PMID:2194669
- Sluder G. Two-way traffic: centrosomes and the cell cycle. Nat Rev Mol Cell Biol 2005; 6:743-8; PMID:16231423
- Lange BM. Integration of the centrosome in cell cycle control, stress response and signal transduction pathways. Curr Opin Cell Biol 2002; 14:35-43; PMID:11792542
- Andersen JS, Wilkinson CJ, Mayor T, Mortensen P, Nigg EA, Mann M. Proteomic characterization of the human centrosome by protein correlation profiling. Nature 2003; 426:570-4; PMID:14654843
- Nogales-Cadenas R, Abascal F, Diez-Perez J, Carazo JM, Pascual-Montano A. CentrosomeDB: a human centrosomal proteins database. Nucleic acids research 2009; 37:D175-80; PMID:18971254
- Nigg EA, Stearns T. The centrosome cycle: Centriole biogenesis, duplication and inherent asymmetries. Nat Cell Biol 2011; 13:1154-60; PMID:21968988
- Fry AM. The Nek2 protein kinase: a novel regulator of centrosome structure. Oncogene 2002; 21:6184-94; PMID:12214248
- Smith E, Hegarat N, Vesely C, Roseboom I, Larch C, Streicher H, Straatman K, Flynn H, Skehel M, Hirota T, et al. Differential control of Eg5-dependent centrosome separation by Plk1 and Cdk1. EMBO J 2011; 30:2233-45; PMID:21522128
- Lee K, Rhee K. PLK1 phosphorylation of pericentrin initiates centrosome maturation at the onset of mitosis. J Cell Biol 2011; 195:1093-101; PMID:22184200
- Piehl M, Tulu US, Wadsworth P, Cassimeris L. Centrosome maturation: measurement of microtubule nucleation throughout the cell cycle by using GFP-tagged EB1. Proc Natl Acad Sci U S A 2004; 101:1584-8; PMID:14747658
- Lawo S, Hasegan M, Gupta GD, Pelletier L. Subdiffraction imaging of centrosomes reveals higher-order organizational features of pericentriolar material. Nat Cell Biol 2012; 14:1148-58; PMID:23086237
- Kobayashi T, Dynlacht BD. Regulating the transition from centriole to basal body. J Cell Biol 2011; 193:435-44; PMID:21536747
- Berndsen CE, Wolberger C. New insights into ubiquitin E3 ligase mechanism. Nat Struct Mol Biol 2014; 21:301-7; PMID:24699078; http://dx.doi.org/10.1038/nsmb.2780
- Ravid T, Hochstrasser M. Diversity of degradation signals in the ubiquitin-proteasome system. Nat Rev Mol Cell Biol 2008; 9:679-90; PMID:18698327; http://dx.doi.org/10.1038/nrm2468
- Bhattacharyya S, Yu H, Mim C, Matouschek A. Regulated protein turnover: snapshots of the proteasome in action. Nat Rev Mol Cell Biol 2014; 15:122-33; PMID:24452470; http://dx.doi.org/10.1038/nrm3741
- Wojcik C, Paweletz N, Schroeter D. Localization of proteasomal antigens during different phases of the cell cycle in HeLa cells. Eur J Cell Biol 1995; 68:191-8; PMID:8575465
- Wigley WC, Fabunmi RP, Lee MG, Marino CR, Muallem S, DeMartino GN, Thomas PJ. Dynamic association of proteasomal machinery with the centrosome. J Cell Biol 1999; 145:481-90; PMID:10225950; http://dx.doi.org/10.1083/jcb.145.3.481
- Brown CR, Doxsey SJ, White E, Welch WJ. Both viral (adenovirus E1B) and cellular (hsp 70, p53) components interact with centrosomes. J Cellular Physiol 1994; 160:47-60; PMID:8021299; http://dx.doi.org/10.1002/jcp.1041600107
- Johnston JA, Ward CL, Kopito RR. Aggresomes: a cellular response to misfolded proteins. J Cell Biol 1998; 143:1883-98; PMID:9864362; http://dx.doi.org/10.1083/jcb.143.7.1883
- Huang J, Raff JW. The disappearance of cyclin B at the end of mitosis is regulated spatially in Drosophila cells. EMBO J 1999; 18:2184-95; PMID:10205172; http://dx.doi.org/10.1093/emboj/18.8.2184
- Fabunmi RP, Wigley WC, Thomas PJ, DeMartino GN. Activity and regulation of the centrosome-associated proteasome. J Biol Chem 2000; 275:409-13; PMID:10617632; http://dx.doi.org/10.1074/jbc.275.1.409
- Wojcik C, Schroeter D, Wilk S, Lamprecht J, Paweletz N. Ubiquitin-mediated proteolysis centers in HeLa cells: indication from studies of an inhibitor of the chymotrypsin-like activity of the proteasome. Eur J Cell Biol 1996; 71:311-8; PMID:8929570
- Johnston JA, Illing ME, Kopito RR. Cytoplasmic dynein/dynactin mediates the assembly of aggresomes. Cell Motil Cytoskeleton 2002; 53:26-38; PMID:12211113; http://dx.doi.org/10.1002/cm.10057
- Kawaguchi Y, Kovacs JJ, McLaurin A, Vance JM, Ito A, Yao TP. The deacetylase HDAC6 regulates aggresome formation and cell viability in response to misfolded protein stress. Cell 2003; 115:727-38; PMID:14675537; http://dx.doi.org/10.1016/S0092-8674(03)00939-5
- Corboy MJ, Thomas PJ, Wigley WC. Aggresome formation. Methods Mol Biol 2005; 301:305-27; PMID:15917642
- Bence NF, Sampat RM, Kopito RR. Impairment of the ubiquitin-proteasome system by protein aggregation. Science 2001; 292:1552-5; PMID:11375494; http://dx.doi.org/10.1126/science.292.5521.1552
- Snyder H, Mensah K, Theisler C, Lee J, Matouschek A, Wolozin B. Aggregated and monomeric alpha-synuclein bind to the S6′ proteasomal protein and inhibit proteasomal function. J Biol Chem 2003; 278:11753-9; PMID:12551928; http://dx.doi.org/10.1074/jbc.M208641200
- Chin LS, Olzmann JA, Li L. Parkin-mediated ubiquitin signalling in aggresome formation and autophagy. Biochem Soc Trans 2010; 38:144-9; PMID:20074049; http://dx.doi.org/10.1042/BST0380144
- Hao R, Nanduri P, Rao Y, Panichelli RS, Ito A, Yoshida M, Yao TP. Proteasomes activate aggresome disassembly and clearance by producing unanchored ubiquitin chains. Molecular cell 2013; 51:819-28; PMID:24035499; http://dx.doi.org/10.1016/j.molcel.2013.08.016
- Viswanathan J, Haapasalo A, Bottcher C, Miettinen R, Kurkinen KM, Lu A, Thomas A, Maynard CJ, Romano D, Hyman BT, et al. Alzheimer's disease-associated ubiquilin-1 regulates presenilin-1 accumulation and aggresome formation. Traffic 2011; 12:330-48; PMID:21143716; http://dx.doi.org/10.1111/j.1600-0854.2010.01149.x
- Waelter S, Boeddrich A, Lurz R, Scherzinger E, Lueder G, Lehrach H, Wanker EE. Accumulation of mutant huntingtin fragments in aggresome-like inclusion bodies as a result of insufficient protein degradation. Mol Biol Cell 2001; 12:1393-407; PMID:11359930; http://dx.doi.org/10.1091/mbc.12.5.1393
- Sukhdeo K, Mani M, Hideshima T, Takada K, Pena-Cruz V, Mendez G, Ito S, Anderson KC, Carrasco DR. beta-catenin is dynamically stored and cleared in multiple myeloma by the proteasome-aggresome-autophagosome-lysosome pathway. Leukemia 2012; 26:1116-9; PMID:22051532; http://dx.doi.org/10.1038/leu.2011.303
- Wojcik C, DeMartino GN. Intracellular localization of proteasomes. Int J Biochem Cell Biol 2003; 35:579-89; PMID:12672451; http://dx.doi.org/10.1016/S1357-2725(02)00380-1
- Fisk HA. Many Pathways to Destruction: Many pathways to destruction: the centrosome and its control by and role in regulated proteolysis. In: Schatten H, ed. The Centrosome: Cell and Molecular Mechanisms of Functions and Dysfunctions in Disease. Humana Press, 2012:133-55.
- Didier C, Merdes A, Gairin JE, Jabrane-Ferrat N. Inhibition of proteasome activity impairs centrosome-dependent microtubule nucleation and organization. Mol Biol Cell 2008; 19:1220-9; PMID:18094058; http://dx.doi.org/10.1091/mbc.E06-12-1140
- Hames RS, Crookes RE, Straatman KR, Merdes A, Hayes MJ, Faragher AJ, Fry AM. Dynamic recruitment of Nek2 kinase to the centrosome involves microtubules, PCM-1, and localized proteasomal degradation. Mol Biol Cell 2005; 16:1711-24; PMID:15659651; http://dx.doi.org/10.1091/mbc.E04-08-0688
- Habedanck R, Stierhof YD, Wilkinson CJ, Nigg EA. The Polo kinase Plk4 functions in centriole duplication. Nat Cell Biol 2005; 7:1140-6; PMID:16244668; http://dx.doi.org/10.1038/ncb1320
- Hudson JW, Kozarova A, Cheung P, Macmillan JC, Swallow CJ, Cross JC, Dennis JW. Late mitotic failure in mice lacking Sak, a polo-like kinase. Curr Biol 2001; 11:441-6; PMID:11301255; http://dx.doi.org/10.1016/S0960-9822(01)00117-8
- Peel N, Dougherty M, Goeres J, Liu Y, O'Connell KF. The C. elegans F-box proteins LIN-23 and SEL-10 antagonize centrosome duplication by regulating ZYG-1 levels. J Cell Sci 2012; 125:3535-44; PMID:22623721; http://dx.doi.org/10.1242/jcs.097105
- Cunha-Ferreira I, Rodrigues-Martins A, Bento I, Riparbelli M, Zhang W, Laue E, Callaini G, Glover DM, Bettencourt-Dias M. The SCF/Slimb ubiquitin ligase limits centrosome amplification through degradation of SAK/PLK4. Curr Biol 2009; 19:43-9; PMID:19084407; http://dx.doi.org/10.1016/j.cub.2008.11.037
- Rogers GC, Rusan NM, Roberts DM, Peifer M, Rogers SL. The SCF Slimb ubiquitin ligase regulates Plk4/Sak levels to block centriole reduplication. J Cell Biol 2009; 184:225-39; PMID:19171756; http://dx.doi.org/10.1083/jcb.200808049
- Mason JM, Lin DC, Wei X, Che Y, Yao Y, Kiarash R, Cescon DW, Fletcher GC, Awrey DE, Bray MR, et al. Functional characterization of CFI-400945, a Polo-like kinase 4 inhibitor, as a potential anticancer agent. Cancer Cell 2014; 26:163-76; PMID:25043604; http://dx.doi.org/10.1016/j.ccr.2014.05.006
- Freed E, Lacey KR, Huie P, Lyapina SA, Deshaies RJ, Stearns T, Jackson PK. Components of an SCF ubiquitin ligase localize to the centrosome and regulate the centrosome duplication cycle. Gen Dev 1999; 13:2242-57; PMID:10485847; http://dx.doi.org/10.1101/gad.13.17.2242
- Dou Z, Liu X, Wang W, Zhu T, Wang X, Xu L, Abrieu A, Fu C, Hill DL, Yao X. Dynamic localization of Mps1 kinase to kinetochores is essential for accurate spindle microtubule attachment. Proc Natl Acad Sci U S A 2015; 112:E4546-55; PMID:26240331; http://dx.doi.org/10.1073/pnas.1508791112
- Kasbek C, Yang CH, Yusof AM, Chapman HM, Winey M, Fisk HA. Preventing the degradation of mps1 at centrosomes is sufficient to cause centrosome reduplication in human cells. Mol Biol Cell 2007; 18:4457-69; PMID:17804818; http://dx.doi.org/10.1091/mbc.E07-03-0283
- Fisk HA, Winey M. The mouse Mps1p-like kinase regulates centrosome duplication. Cell 2001; 106:95-104; PMID:11461705; http://dx.doi.org/10.1016/S0092-8674(01)00411-1
- Kasbek C, Yang CH, Fisk HA. Antizyme restrains centrosome amplification by regulating the accumulation of Mps1 at centrosomes. Mol Biol Cell 2010; 21:3878-89; PMID:20861309; http://dx.doi.org/10.1091/mbc.E10-04-0281
- Mangold U, Hayakawa H, Coughlin M, Munger K, Zetter BR. Antizyme, a mediator of ubiquitin-independent proteasomal degradation and its inhibitor localize to centrosomes and modulate centriole amplification. Oncogene 2008; 27:604-13; PMID:17667942; http://dx.doi.org/10.1038/sj.onc.1210685
- Liu J, Cheng X, Zhang Y, Li S, Cui H, Zhang L, Shi R, Zhao Z, He C, Wang C, et al. Phosphorylation of Mps1 by BRAFV600E prevents Mps1 degradation and contributes to chromosome instability in melanoma. Oncogene 2013; 32:713-23; PMID:22430208; http://dx.doi.org/10.1038/onc.2012.94
- Ben-Nissan G, Sharon M. Regulating the 20S proteasome ubiquitin-independent degradation pathway. Biomolecules 2014; 4:862-84; PMID:25250704; http://dx.doi.org/10.3390/biom4030862
- Yu H, King RW, Peters JM, Kirschner MW. Identification of a novel ubiquitin-conjugating enzyme involved in mitotic cyclin degradation. Curr Biol 1996; 6:455-66; PMID:8723350; http://dx.doi.org/10.1016/S0960-9822(02)00513-4
- Sudakin V, Ganoth D, Dahan A, Heller H, Hershko J, Luca FC, Ruderman JV, Hershko A. The cyclosome, a large complex containing cyclin-selective ubiquitin ligase activity, targets cyclins for destruction at the end of mitosis. Mol Biol Cell 1995; 6:185-97; PMID:7787245; http://dx.doi.org/10.1091/mbc.6.2.185
- Debec A, Montmory C. Cyclin B is associated with centrosomes in Drosophila mitotic cells. Biol Cell 1992; 75:121-6; PMID:1393148; http://dx.doi.org/10.1016/0248-4900(92)90131-J
- Jackman M, Lindon C, Nigg EA, Pines J. Active cyclin B1-Cdk1 first appears on centrosomes in prophase. Nat Cell Biol 2003; 5:143-8; PMID:12524548; http://dx.doi.org/10.1038/ncb918
- Rieder CL, Khodjakov A, Paliulis LV, Fortier TM, Cole RW, Sluder G. Mitosis in vertebrate somatic cells with two spindles: implications for the metaphase/anaphase transition checkpoint and cleavage. Proc Natl Acad Sci U S A 1997; 94:5107-12; PMID:9144198; http://dx.doi.org/10.1073/pnas.94.10.5107
- Clute P, Pines J. Temporal and spatial control of cyclin B1 destruction in metaphase. Nat Cell Biol 1999; 1:82-7; PMID:10559878; http://dx.doi.org/10.1038/10049
- Raff JW, Jeffers K, Huang JY. The roles of Fzy/Cdc20 and Fzr/Cdh1 in regulating the destruction of cyclin B in space and time. J Cell Biol 2002; 157:1139-49; PMID:12082076; http://dx.doi.org/10.1083/jcb.200203035
- Mathe E, Kraft C, Giet R, Deak P, Peters JM, Glover DM. The E2-C vihar is required for the correct spatiotemporal proteolysis of cyclin B and itself undergoes cyclical degradation. Curr Biol 2004; 14:1723-33; PMID:15458643; http://dx.doi.org/10.1016/j.cub.2004.09.023
- Wakefield JG, Huang JY, Raff JW. Centrosomes have a role in regulating the destruction of cyclin B in early Drosophila embryos. Curr Biol 2000; 10:1367-70; PMID:11084336; http://dx.doi.org/10.1016/S0960-9822(00)00776-4
- Chang DC, Xu N, Luo KQ. Degradation of cyclin B is required for the onset of anaphase in Mammalian cells. J Biol Chem 2003; 278:37865-73; PMID:12865421; http://dx.doi.org/10.1074/jbc.M306376200
- Lara-Gonzalez P, Westhorpe FG, Taylor SS. The spindle assembly checkpoint. Curr Biol 2012; 22:R966-80; PMID:23174302; http://dx.doi.org/10.1016/j.cub.2012.10.006
- Vazquez-Novelle MD, Mirchenko L, Uhlmann F, Petronczki M. The 'anaphase problem': how to disable the mitotic checkpoint when sisters split. Biochem Soc Trans 2010; 38:1660-6; PMID:21118144; http://dx.doi.org/10.1042/BST0381660
- Clijsters L, van Zon W, Riet BT, Voets E, Boekhout M, Ogink J, Rumpf-Kienzl C, Wolthuis RM. Inefficient degradation of cyclin B1 re-activates the spindle checkpoint right after sister chromatid disjunction. Cell Cycle 2014; 13:2370-8; PMID:25483188; http://dx.doi.org/10.4161/cc.29336
- Pant V, Lozano G. Limiting the power of p53 through the ubiquitin proteasome pathway. Gen Dev 2014; 28:1739-51; PMID:25128494; http://dx.doi.org/10.1101/gad.247452.114
- Salic A, Lee E, Mayer L, Kirschner MW. Control of beta-catenin stability: reconstitution of the cytoplasmic steps of the wnt pathway in Xenopus egg extracts. Molecular cell 2000; 5:523-32; PMID:10882137; http://dx.doi.org/10.1016/S1097-2765(00)80446-3
- Vigneron AM, Ludwig RL, Vousden KH. Cytoplasmic ASPP1 inhibits apoptosis through the control of YAP. Gen Dev 2010; 24:2430-9; PMID:21041411; http://dx.doi.org/10.1101/gad.1954310
- Moroishi T, Park HW, Qin B, Chen Q, Meng Z, Plouffe SW, Taniguchi K, Yu FX, Karin M, Pan D, et al. A YAP/TAZ-induced feedback mechanism regulates Hippo pathway homeostasis. Gen Dev 2015; 29:1271-84; PMID:26109050; http://dx.doi.org/10.1101/gad.262816.115
- Alon U. An Introduction to Systems Biology: Design Principles of Biological Circuits. CRC Press, 2006: 19-21.
- Fuentealba LC, Eivers E, Ikeda A, Hurtado C, Kuroda H, Pera EM, De Robertis EM. Integrating patterning signals: Wnt/GSK3 regulates the duration of the BMP/Smad1 signal. Cell 2007; 131:980-93; PMID:18045539; http://dx.doi.org/10.1016/j.cell.2007.09.027
- MacDonald BT, Tamai K, He X. Wnt/beta-catenin signaling: components, mechanisms, and diseases. Dev Cell 2009; 17:9-26; PMID:19619488; http://dx.doi.org/10.1016/j.devcel.2009.06.016
- Fuentealba LC, Eivers E, Geissert D, Taelman V, De Robertis EM. Asymmetric mitosis: Unequal segregation of proteins destined for degradation. Proc Natl Acad Sci U S A 2008; 105:7732-7; PMID:18511557; http://dx.doi.org/10.1073/pnas.0803027105
- Kidd AR, III, Miskowski JA, Siegfried KR, Sawa H, Kimble J. A â-catenin identified by functional rather than sequence criteria and its role in Wnt/MAPK signaling. Cell 2005; 121:761-72; PMID:15935762; http://dx.doi.org/10.1016/j.cell.2005.03.029
- Baldwin AT, Phillips BT. The tumor suppressor APC differentially regulates multiple beta-catenins through the function of axin and CKIalpha during C. elegans asymmetric stem cell divisions. J Cell Sci 2014; 127:2771-81; PMID:24762815; http://dx.doi.org/10.1242/jcs.146514
- Phillips BT, Kimble J. A new look at TCF and beta-catenin through the lens of a divergent C. elegans Wnt pathway. Dev Cell 2009; 17:27-34; PMID:19619489; http://dx.doi.org/10.1016/j.devcel.2009.07.002
- Phillips BT, Kidd AR, 3rd, King R, Hardin J, Kimble J. Reciprocal asymmetry of SYS-1/beta-catenin and POP-1/TCF controls asymmetric divisions in Caenorhabditis elegans. Proc Natl Acad Sci U S A 2007; 104:3231-6; PMID:17296929; http://dx.doi.org/10.1073/pnas.0611507104
- Baldwin AT, Clemons AM, Phillips BT. Unique and redundant beta-catenin regulatory roles of two Dishevelled paralogs during C. elegans asymmetric cell division. J Cell Sci 2016; 129:983-93; PMID:26795562; http://dx.doi.org/10.1242/jcs.175802
- Mila D, Calderon A, Baldwin AT, Moore KM, Watson M, Phillips BT, Putzke AP. Asymmetric Wnt Pathway Signaling Facilitates Stem Cell-Like Divisions via the Nonreceptor Tyrosine Kinase FRK-1 in Caenorhabditis elegans. Genetics 2015; 201:1047-60; PMID:26358719; http://dx.doi.org/10.1534/genetics.115.181412
- Chesney MA, Lam N, Morgan DE, Phillips BT, Kimble J. C. elegans HLH-2/E/Daughterless controls key regulatory cells during gonadogenesis. Dev Biol 2009; 331:14-25; PMID:19376107; http://dx.doi.org/10.1016/j.ydbio.2009.04.015
- Liu J, Phillips BT, Amaya MF, Kimble J, Xu W. The C. elegans SYS-1 protein is a bona fide beta-catenin. Dev Cell 2008; 14:751-61; PMID:18477457; http://dx.doi.org/10.1016/j.devcel.2008.02.015
- Schlaitz AL, Srayko M, Dammermann A, Quintin S, Wielsch N, MacLeod I, de Robillard Q, Zinke A, Yates JR, 3rd, Muller-Reichert T, et al. The C. elegans RSA complex localizes protein phosphatase 2A to centrosomes and regulates mitotic spindle assembly. Cell 2007; 128:115-27; PMID:17218259; http://dx.doi.org/10.1016/j.cell.2006.10.050
- Mahen R, Jeyasekharan AD, Barry NP, Venkitaraman AR. Continuous polo-like kinase 1 activity regulates diffusion to maintain centrosome self-organization during mitosis. Proc Natl Acad Sci U S A 2011; 108:9310-5; PMID:21576470; http://dx.doi.org/10.1073/pnas.1101112108
- Zacharias AL, Walton T, Preston E, Murray JI. Quantitative Differences in Nuclear beta-catenin and TCF Pattern Embryonic Cells in C. elegans. PLoS genetics 2015; 11:e1005585; PMID:26488501; http://dx.doi.org/10.1371/journal.pgen.1005585
- De La Mota-Peynado A, Lee SY, Pierce BM, Wani P, Singh CR, Roelofs J. The proteasome-associated protein Ecm29 inhibits proteasomal ATPase activity and in vivo protein degradation by the proteasome. J Biol Chem 2013; 288:29467-81; PMID:23995839; http://dx.doi.org/10.1074/jbc.M113.491662
- Kimura H, Miki Y, Nakanishi A. Centrosomes at M phase act as a scaffold for the accumulation of intracellular ubiquitinated proteins. Cell Cycle 2014; 13:1928-37; PMID:24743317; http://dx.doi.org/10.4161/cc.28896
- Holt CE, Schuman EM. The central dogma decentralized: new perspectives on RNA function and local translation in neurons. Neuron 2013; 80:648-57; PMID:24183017; http://dx.doi.org/10.1016/j.neuron.2013.10.036
- Segref A, Hoppe T. Think locally: control of ubiquitin-dependent protein degradation in neurons. EMBO Rep 2009; 10:44-50; PMID:19079132; http://dx.doi.org/10.1038/embor.2008.229
- Kim AH, Puram SV, Bilimoria PM, Ikeuchi Y, Keough S, Wong M, Rowitch D, Bonni A. A centrosomal Cdc20-APC pathway controls dendrite morphogenesis in postmitotic neurons. Cell 2009; 136:322-36; PMID:19167333; http://dx.doi.org/10.1016/j.cell.2008.11.050
- Puram SV, Kim AH, Bonni A. An old dog learns new tricks: a novel function for Cdc20-APC in dendrite morphogenesis in neurons. Cell Cycle 2010; 9:482-5; PMID:20195072; http://dx.doi.org/10.4161/cc.9.3.10558
- Puram SV, Kim AH, Park HY, Anckar J, Bonni A. The ubiquitin receptor S5a/Rpn10 links centrosomal proteasomes with dendrite development in the mammalian brain. Cell Rep 2013; 4:19-30; PMID:23831032; http://dx.doi.org/10.1016/j.celrep.2013.06.006
- Cowan CR, Hyman AA. Centrosomes direct cell polarity independently of microtubule assembly in C. elegans embryos. Nature 2004; 431:92-6; PMID:15343338; http://dx.doi.org/10.1038/nature02825
- Tsun A, Qureshi I, Stinchcombe JC, Jenkins MR, de la Roche M, Kleczkowska J, Zamoyska R, Griffiths GM. Centrosome docking at the immunological synapse is controlled by Lck signaling. J Cell Biol 2011; 192:663-74; PMID:21339332; http://dx.doi.org/10.1083/jcb.201008140
- Tang N, Marshall WF. Centrosome positioning in vertebrate development. J Cell Sci 2012; 125:4951-61; PMID:23277534; http://dx.doi.org/10.1242/jcs.038083
- Stinchcombe JC, Griffiths GM. Communication, the centrosome and the immunological synapse. Philos Trans R Soc Lond B Biol Sci 2014; 369; http://dx.doi.org/10.1098/rstb.2013.0463
- Vertii A, Zimmerman W, Ivshina M, Doxsey S. Centrosome-intrinsic mechanisms modulate centrosome integrity during fever. Mol Biol Cell 2015; 26:3451-63; PMID:26269579; http://dx.doi.org/10.1091/mbc.E15-03-0158
- Vidair CA, Doxsey SJ, Dewey WC. Heat shock alters centrosome organization leading to mitotic dysfunction and cell death. J Cellular Physiol 1993; 154:443-55; PMID:8436595; http://dx.doi.org/10.1002/jcp.1041540302
- Rivett AJ, Hearn AR. Proteasome function in antigen presentation: immunoproteasome complexes, Peptide production, and interactions with viral proteins. Curr Protein Pept Sci 2004; 5:153-61; PMID:15180520; http://dx.doi.org/10.2174/1389203043379774
- Ferrington DA, Gregerson DS. Immunoproteasomes: structure, function, and antigen presentation. Prog Mol Biol Transl Sci 2012; 109:75-112; PMID:22727420; http://dx.doi.org/10.1016/B978-0-12-397863-9.00003-1
- Anton LC, Schubert U, Bacik I, Princiotta MF, Wearsch PA, Gibbs J, Day PM, Realini C, Rechsteiner MC, Bennink JR, et al. Intracellular localization of proteasomal degradation of a viral antigen. J Cell Biol 1999; 146:113-24; PMID:10402464; http://dx.doi.org/10.1083/jcb.146.1.113
- Lacaille VG, Androlewicz MJ. Targeting of HIV-1 Nef to the centrosome: implications for antigen processing. Traffic 2000; 1:884-91; PMID:11208077; http://dx.doi.org/10.1034/j.1600-0854.2000.011107.x
- Hung CF, Cheng WF, He L, Ling M, Juang J, Lin CT, Wu TC. Enhancing major histocompatibility complex class I antigen presentation by targeting antigen to centrosomes. Cancer Res 2003; 63:2393-8; PMID:12750257
- Puram SV, Kim AH, Ikeuchi Y, Wilson-Grady JT, Merdes A, Gygi SP, Bonni A. A CaMKIIbeta signaling pathway at the centrosome regulates dendrite patterning in the brain. Nat Neurosci 2011; 14:973-83; PMID:21725312; http://dx.doi.org/10.1038/nn.2857