ABSTRACT
EWSR1, participating in transcription and splicing, has been identified as a translocation partner for various transcription factors, resulting in translocation, which in turn plays crucial roles in tumorigenesis. Recent studies have investigated the role of EWSR1 in mitosis. However, the effect of EWSR1 on mitosis is poorly understood. Here, we observed that depletion of EWSR1 resulted in cell cycle arrest in the mitotic phase, mainly due to an increase in the time from nuclear envelope breakdown to metaphase, resulting in a high percentage of unaligned chromosomes and multipolar spindles. We also demonstrated that EWSR1 is a spindle-associated protein that interacts with α-tubulin during mitosis. EWSR1 depletion increased the cold-sensitivity of spindle microtubules, and decreased the rate of spindle assembly. EWSR1 regulated the level of microtubule acetylation in the mitotic spindle; microtubule acetylation was rescued in EWSR1-depleted mitotic cells following suppression of HDAC6 activity by its specific inhibitor or siRNA treatment. In summary, these results suggest that EWSR1 regulates the acetylation of microtubules in a cell cycle-dependent manner through its dynamic location on spindle MTs, and may be a novel regulator for mitosis progress independent of its translocation.
Introduction
Ewing sarcoma breakpoint region 1 protein (EWSR1), also called Ewing's sarcoma protein, is an RNA-binding protein and a member of the TLS/EWS/TAF15 family, which was initially recognized in Ewing sarcoma as forming a fusion protein through t(11;22)(q24;q12) chromosome translocation.Citation1,2 During the past decades, EWSR1 has been identified as a translocation partner for many transcription factors including FLI-1, ATF-1, NR4A3, and CHOP in diverse tumors.Citation3-7 Recently, an increasing number of studies have focused on the characterization of EWSR1 itself. EWSR1 is ubiquitously expressed in most human cell types, except for cardiac muscle cells and melanocytes.Citation8 Alternative splicing of EWSR1 in the brain results in a special isoform that is associated with neural differentiation.Citation9 EWSR1 is a multi-domain protein containing an N-terminal transcription activation domain (TAD) with several conserved serine-tyrosine-glycine-glutamine (SYGQ) repeats, and a C-terminal nucleic acid binding domain (RBD) with an RNA recognition motif, a zinc finger motif, and 3 Arginine-Glycine-Glycine- (RGG-) repeat boxes, which play critical roles in various biological processes through their interactions with other proteins.Citation10 For example, several studies reported the participation of EWSR1 in transcriptional regulation through its association with the general transcription factor TFIID and the tissue-specific transcription factors Oct4 and Brn3a.Citation11-13 The association of EWSR1 with YB-1, a shuttling splicing activator, demonstrates the involvement of EWSR1 in splicing through its C-terminal domain.Citation14 Some lines of evidence, such as the interaction of EWSR1 with Drosha, also suggest a relationship between EWSR1 and microRNA processing.Citation15 Moreover, EWSR1 might regulate the DNA recombination and repair process through the coupling of its NH2 terminus with BRAD1, a partner of the breast cancer susceptibility gene BRCA1.Citation16 Recently, Park et al. demonstrated that EWSR1 interacts with Aurora B kinase, a component of the chromosome passenger complex (CPC) which is critical for checkpoint control in mitosis, through its RGG3 domain, recruiting Aurora B to the midzone.Citation17
EWS-deficient mice were born at normal Mendelian ratios; however, the size of neonates was smaller than those of the wild type, and a high frequency of postnatal mortality was observed.Citation18 Detailed analysis of surviving mice showed that loss of EWSR1 led to a defect in pre-B lymphocyte development, significantly reduced cellularity in major haematopoietic organs, and deficient gametogenesis in both sexes. Ews-null mouse embryonic fibroblasts (MEFs) exhibited premature cellular senescence and hypersensitivity to DNA damage. Moreover, knockdown of EWSR1 in zebrafish using specific morpholinos resulted in morphological abnormalities in the central nervous system.Citation19 In EWSR1 knockdown embryos, an increased number of aberrant spindles were observed, along with mislocalization of Aurora B. Similarly, knockdown of EWSR1 in HeLa cells caused mitotic defects and p53-dependent apoptosis.Citation19
It has been reported that EWSR1 exhibits variant subcellular localization at different cellular stages. Under basal conditions, EWSR1 is located mainly in the nucleus through 3 independent nuclear import sequences, and removal of the last 18 amino acids in the C-terminus resulted in its cytoplasmic retention.Citation10 In various phases of the cell cycle, cytoplasmic localization has been observed using immunofluorescence in HeLa and HEK293T cells.Citation20 Moreover, the cell-surface distribution of EWSR1 in peripheral blood mononuclear cells (PBMCs) and tumor cells was found to be methylation-dependent, which might serve as a mediator for signal transduction.Citation21 Recently, an analysis of 817 ALS cases and 1082 matched healthy controls identified 3 missense variants in EWSR1 in ALS patients, which affect its localization in motor neurons.Citation22 These findings suggest that differences in subcellular location might be involved in EWSR1-dependent biological events; however, this has not been conclusively demonstrated.
In this study, we examined the subcellular localization of EWSR1 in greater detail by immunostaining and co-immunoprecipitation. The effect of EWSR1 on mitosis was observed by means of time-lapse analysis, and microtubule (MT) spindles were detected based on the quantity of α-tubulin intensity. In addition, we explored the mechanism of MT acetylation using RNAi-mediated knockdown and HDAC6 inhibitor treatment. We determined that EWSR1 was a spindle-associated protein through its association with MTs in metaphase. Knocking down EWSR1 in HeLa cells led to cell cycle arrest in the G2/M phase due to a defect in the formation of the bipolar spindle. EWSR1 regulates the dynamics of mitotic spindle assembly by affecting MT acetylation through HDAC6. Our study provides new insights into the mechanism by which EWSR1 is involved in mitotic progression, as well as potential new directions for the development of drugs targeting mitosis disorders for tumor treatment.
Results
Knockdown of EWSR1 leads to prolonged mitosis exit
Recently, a few studies have reported that knockdown of EWSR1 caused mitotic defects; however, the details remained to be elucidated. Because genes involved in mitosis are likely to be induced in the G2/M phase, we first examined the protein levels of EWSR1 through the cell cycle. HeLa cells were arrested at the G1/S phase by thymidine treatment for 18 h and then released into taxol to accumulate mitotic cells. As shown in , the cell cycle phase was determined based on the level of the mitotic markers Cyclin E1, Cyclin D1, Cyclin B1, and phospho-histone H3. The levels of EWSR1 were relatively low in G1/S cells but slowly increased in G2/M cells. Consistently, the fluorescence intensity of EWSR1, which was confirmed by immunofluorescence assay, also increased with the duration of taxol treatment (Fig. S1A). Interestingly, EWSR1 appeared to be diffusely distributed in the cytoplasm at the mitotic stage, indicating that it may play a special role in mitosis. To rule out non-specific antibody recognition, the specificity of the antibody against EWSR1 was confirmed by western-blot and by immunostaining (Fig. S1, B and C). Next, we examined the effect of knockdown of EWSR1 on cell-cycle progression. HeLa cells were transfected with EWSR1-specific siRNA oligos for 24 h, followed by thymidine-nocodazole treatment to arrest the cells in mitotic phase. The cells were harvested for cell cycle analysis at different time points after nocodazole release. As shown in , more than 70% of control cells completed mitosis within 2 h after nocodazole release, whereas knockdown of EWSR1 induced a significant increase in cell populations in the G2/M phase. Moreover, we counted the numbers of cells in each phase by observing the shape of chromosomes following immunostaining, in order to distinguish the cause of mitosis arrest. As shown in , at 2 h after nocodazole removal, ∼95% of control cells had left mitosis, whereas ∼25% of cells treated with RNAi against EWSR1 were still in the M phase. Until 6 h after nocodazole release, a smaller percentage of EWSR1-deficient cells had exited the M phase compared to that in control cells. However, 8 h after synchronous release, the percentages of mitotic phase cells were similar between EWSR1-deficient cells and control cells. To gain further insight into the mitotic exit delay caused by EWSR1 depletion, mitotic progression was characterized in detail. As shown in , more than 90% of control cells had progressed through mitosis into telophase and interphase at 2 h after nocodazole release, while ∼20% of the EWSR1-depleted cells remained in prometaphase. Until 6 h after nocodazole release, the percentage of cells in prometaphase-metaphase were much higher and cells in anaphase-telophase and interphase were relatively few in EWSR1-depleted cells, compared to control cells.
Figure 1. EWSR1 regulates cell cycle progression. (A) HeLa cells were treated with 2.5 mM thymidine for 18 h, and then released into 100 nM taxol for the indicated times. Cells were harvested and analyzed by western blot with anti-Cyclin E1, anti-Cyclin D1, anti-Cyclin B1, anti- phospho-histone H3, and anti-EWSR1 antibodies. (B-D) HeLa cells were transfected with negative control siRNA or EWSR1-specific siRNA oligos. Twenty-four hours after transfection, the cells were treated with 2.5 mM thymidine for 18 h, released in fresh culture for 3 h, and then treated with nocodazole for 12 h and released in fresh culture. The cells were harvested at various time points following nocodazole release as indicated and analyzed for DNA content by flow cytometry (B). Eight hours after nocodazole release, the cells were treated again with nocodazole to arrest cells in the next mitosis. The percentage of mitotic cells (n = 200 cells) at different time points after nocodazole release was determined by DNA staining (C). The percentage of cells in each mitotic stage was determined by DNA staining (D). All results are represented as the mean ± SD. of triplicate experiments. Student t test was used to compare the mean relative values between groups (*p < 0.05, **p < 0.01, ***p < 0.001).
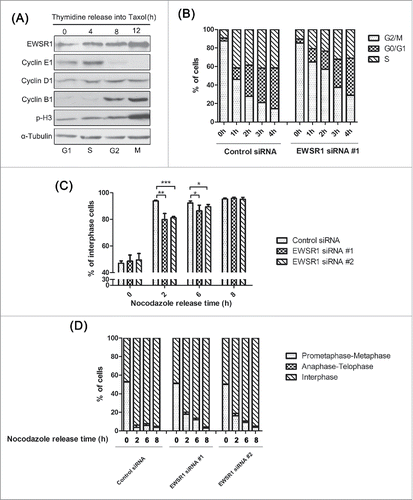
In addition, mitotic progression was monitored by time-lapse imaging. HeLa cells stably expressing RFP-tagged histone H2B were transfected with siRNA for EWSR1. As shown in , EWSR1 knockdown caused a significant increase in the length of the mitotic phase, compared with control cells. Meanwhile, the average time from NEB to metaphase increased from 17 min in control cells to 49 min (siRNA #1) or 30 min (siRNA #2) in EWSR1-depleted cells (n = 180; ). However, the duration of metaphase to anaphase onset was almost unaltered after silencing EWSR1 (n = 180, P>0.05; ). Moreover, the percentage of cells with unaligned chromosomes and multipolar spindles was significantly increased in EWSR1 knockdown, compared with control cells ().
Figure 2. EWSR1 influences the progression of mitosis. (A) HeLa/RFP-H2B cells were transfected with negative control siRNA or EWSR1-specific siRNA oligos for 48 h. Images were observed over a period of 10 h and collected every 5 minutes by time-lapse microscopy (Scale bar: 10 µm). NEB: nuclear envelope breakdown; M: metaphase; A: anaphase. (B-D) Scatter plots (indicating the median and interquartile range) show the times from NEB to anaphase onset, from NEB to metaphase, and from metaphase to anaphase onset. Data were obtained from at least 180 cells in each group. (E & F) Cells with unaligned chromosomes and multipolar spindles in control (n = 100 cells) and EWSR1-knockdown cells (n = 100 cells) were quantified. The graph shows the percentage of cells with unaligned chromosomes or multipolar spindles (Scale bar: 10 μm). (G) HeLa cells were transfected with siRNA-resistant GFP-EWSR1 or GFP for 12 h and then transfected with negative control siRNA or EWSR1-specific siRNA oligos. Twenty-four hours after transfection, the cells were treated with 2.5 mM thymidine for 18 h, released in fresh culture for 3 h, treated with nocodazole for 12 h, and then released in fresh culture. The cells were harvested at various time points following nocodazole release as indicated, and analyzed for DNA content by flow cytometry. (H-J) HeLa/RFP-H2B cells were transfected with siRNA-resistant GFP-EWSR1 or GFP for 12 h and then transfected with control or EWSR1 siRNAs for 48 h. Images were observed over a period of 10 h and collected every 5 minutes by time-lapse microscopy (Scale bar: 10 μm) (H). Scatter plots (indicating the median and interquartile range) showed the times from NEB to metaphase. Data were obtained from at least 180 cells in each group (I). EWSR1 expression levels were assessed in HeLa cells transfected with EWSR1 siRNA or siRNA-resistant EWSR1 by protein gel blotting, and GAPDH was used as an internal reference (J). All the results are represented as the mean ± SD of triplicate experiments. Student t test was used to compare mean relative values between groups (*p < 0.05, **p < 0.01, ***p < 0.001).
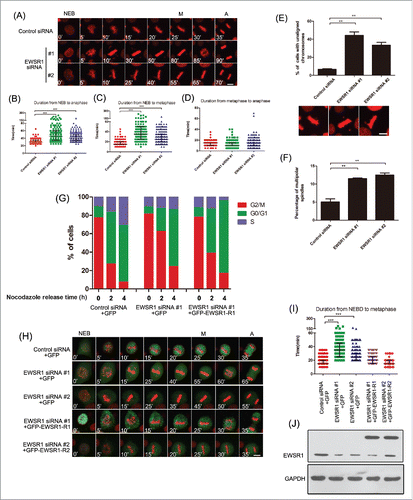
To rule out any off-target effect of EWSR1 siRNA, HeLa cells were transfected with siRNA-resistant EWSR1 before siRNA transfection. As expected, expression of the siRNA-resistant EWSR1 rescued the G2/M arrest phenotype (), and the prolonged time from NEB to metaphase induced by EWSR1 knockdown was also reversed (). Taken together, these results indicate that EWSR1 functions in the cell cycle by affecting metaphase progression and chromosome congression.
EWSR1 localizes to the mitotic spindle
It has been reported that EWSR1 has a different subcellular location in the cell cycle and may interact with microtubulin. In order to better understand the mechanism of regulation of mitosis by EWSR1, we next examined the localization of endogenous EWSR1 during mitosis. As shown in Fig. S2A, EWSR1 was mainly localized in the nucleus during interphase. At prometaphase, EWSR1 spread into the cytoplasm, and from metaphase to telophase, a discernible enrichment of EWSR1 was observed in the spindle MT region, and small areas of EWSR1 signals emerged on chromosomes. Furthermore, when cells were treated with PEM buffer under MT-stabilizing conditions before being fixed, the localization of EWSR1 in spindle appeared much clearer (). To validate this phenomenon, GFP-EWSR1 was overexpressed in HeLa cells and GFP signals were also identified on mitotic spindles during mitosis with or without PEM buffer treatment (Fig. S2B).
Figure 3. EWSR1 localizes on the mitotic spindle. (A) HeLa cells were treated with PEM buffer before being fixed with paraformaldehyde, and stained with anti-EWSR1 and anti-α-tubulin antibodies. DNA was stained with Hoechst 33342 (blue). The location of EWSR1 was observed through confocal imaging during the mitotic phase. (Scale bar: 10 μm). (B) HeLa cells were treated with double thymidine and released for 10 h. Whole-cell lysates were extracted and subjected to immunoprecipitation with anti-α-tubulin antibody or mouse IgG and immunoblotted with both anti-α-tubulin and anti-EWSR1 antibodies. (C) Asynchronous HeLa cell lysates were immunoprecipitated with anti-α-tubulin antibody and portions of the immunoprecipitates and cell lysates were analyzed by immunoblotting with anti-α-tubulin and anti-EWSR1 antibodies. (D) HeLa/RFP-H2B cells were transfected with siRNA-resistant EWSR1ΔNLS for 12 h and then transfected with control or EWSR1 siRNAs for 48 h. (E) HeLa/RFP-H2B cells were transfected with control or EWSR1 siRNAs for 48 h. The synchronous cells were incubated with 2 μg/ml α-amanitin for 5 h. For (D) and (E), Images were observed over a period of 10 h and collected every 5 minutes by time-lapse microscopy (Scale bar: 10 μm). Scatter plots (indicating the median and interquartile range) show the time from NEB to metaphase. Data were obtained from at least 180 cells in each group. All the results are represented as the mean ± SD of triplicate experiments. Student t test was used to compare the mean relative values between groups (***p < 0.001).
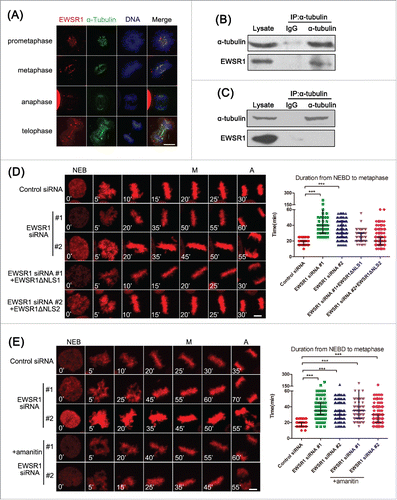
Due to the localization of EWSR1 on spindle MTs, we speculate that there are interactions between EWSR1 and spindle proteins. HeLa cells were synchronized in mitotic phase by double thymidine treatment and release, and then the cell lysates were subjected to immunoprecipitation with anti-α-tubulin antibody. As results, EWSR1 co-precipitated with α-tubulin in lysates from mitotic cells (), but did not co-precipitate with α-tubulin in lysates from asynchronous cells (). Similar results were also obtained in L02 cells (Fig. S2C and D). To further confirm this association, we performed a GST-pull down assay using GST-EWSR1 fusion protein purified from bacteria and HeLa cell extracts. GST-EWSR1, but not GST alone, was observed to be specifically bound to α-tubulin (Fig. S3). Moreover, when RNAase A was used to remove RNA prior to GST-pull down, similar results were observed, suggesting that the localization of EWSR1 in spindle MTs is not mediated by RNA.
To explore the role of MT-binding of EWSR1 in the regulation of mitosis, we constructed the EWSR1ΔNLS mutant in which the last 18 amino acids at the C-terminus were deleted to prevent the nuclear translocation of the protein, in order to exclude the effect of nuclear-localized EWSR1. As shown in , expression of this EWSR1ΔNLS mutant rescued the prolonged time from NEB to metaphase induced by EWSR1 knockdown. The localization of this EWSR1ΔNLS mutant during cell cycle transitions is shown in Fig. S4. We further investigated the effect of EWSR1 on mitosis using α-amanitin, an inhibitor of RNA polymerase II, to rule out its transcriptional activity. As shown in , no obvious changes were found in the inhibitory effects of EWSR1-siRNA on mitosis after treatment with α-amanitin. These results indicate that EWSR1 is localized on the mitotic spindle during mitosis through an interaction with α-tubulin to play a role in the cell cycle in a transcription-independent manner.
Knockdown of EWSR1 leads to mitotic spindle abnormalities
The spindle is an important cell machine that is formed in mitosis by the extension of microtubules from the opposite poles of the cell. Due to the important role played by spindle MTs in chromosome congression, we first examined the effect of knockdown EWSR1 on spindle formation. The regrowth rate of the spindle was analyzed kinetically following complete MT depolymerization induced by 1 μg/ml nocodazole. As shown in , in control cells, the mitotic spindle was reformed within 5 min and most of the chromosomes were arranged on the metaphase plate. In contrast, EWSR1-depleted cells had disorganized spindles, and chromosomes in these cells were not congressed, which suggested that depletion of EWSR1 reduces the rate of spindle assembly. Next, we examined the stability of MTs. As shown in , in control cells, the spindle structure was relatively intact, whereas spindle MTs in EWSR1-depleted cells appeared as unbundled microtubules and chromosomes were not fully aligned along the metaphase plate. MT density was also significantly decreased in EWSR1-depleted cells, compared with that in control cells, in response to cold treatment, suggesting that the knockdown of EWSR1 causes instability of spindle microtubules, owing to a lack of kinetochore-microtubule attachment.
Figure 4. EWSR1 regulates the formation of the mitotic spindle. HeLa cells were transfected with negative control siRNA or EWSR1-specific siRNA oligos. (A) Forty-eight hours after transfection, the cells were treated with 1 μg/ml nocodazole for 10 min and re-incubated at 37°C for different times, as indicated. The cells were fixed and stained for α-tubulin and EWSR1. (B) Twenty-four hours after transfection, the cells were treated with 2.5 mM thymidine for 18 h, released in fresh culture for 8 h, and subsequently synchronized to metaphase with 10 μM MG132 for 2 h. Before fixing, the cells were incubated on ice for 10 min, and then stained for α-tubulin and EWSR1. MT fluorescence intensity of metaphase cells in control (n = 15 cells) or EWSR1-depleted cells (n = 15 cells) was quantified and the results were graphed as fold change compared with control. DNA was stained with Hoechst 33342 (blue) and images were obtained through an inverted fluorescence microscope (Scale bar: 10 μm). All the results are represented as the mean ± SD of triplicate experiments. Student t test was used to compare the mean relative values between groups (*p < 0.05, **p < 0.01). (C & D) The cells were treated as in (B), fixed with 1% paraformaldehyde, stained with anti-CREST and anti-Mad2 (C) or anti-BubR1 (D) antibodies and Hoechst 33342 (blue), and visualized using an inverted fluorescence microscope. Scale bar = 10 μm.
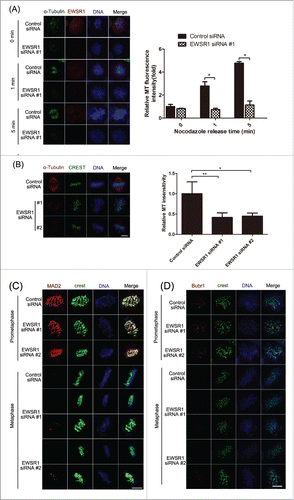
To determine whether the lack of kinetochore-MT interaction was due to a kinetochore structural defect, we examined the effect of EWSR1 knockdown on the locations of checkpoint proteins Mad2 and BubR1, which supervise the residence of MTs on kinetochores. As shown in , representative fluorescence staining of Mad2 and BubR1 was observed on kinetochores for both control and EWSR1-depleted cells at prometaphase. As expected, these signals disappeared from kinetochores in control metaphase cells, consistent with previously reports. In contrast, knockdown of EWSR1 did not inhibit the decrease in fluorescence intensity of checkpoint proteins in metaphase cells upon chromosome congression to the metaphase plate. However, we also observed these fluorescence signals on kinetochores in metaphase cells with unaligned chromosomes, suggesting that EWSR1 depletion generates a weakened checkpoint at individual unattached kinetochores, even though anaphase onset is normal, as has been shown in the above results (). Others have reported that EWSR1 regulated midzone formation during anaphase by recruiting Aurora B.Citation17 However Aurora B is also involved in many other processes in mitosis, such as chromatin modification and microtubule-kinetochore attachment, but not in cytokinesis.Citation23 Therefore, we examined the location of Aurora B, which appeared to be dynamically localized throughout the mitosis (Fig. S5). In agreement with previous reports, from prometaphase to metaphase, Aurora B remains localized in centromeres and EWSR1 depletion did not affect its location, indicating that depletion of EWSR1 does not influence the kinetochore assembly of Aurora B during prometaphase/metaphase. During anaphase, Aurora B relocated to the midzone in control cells, whereas in EWSR1-depleted cells, fluorescence staining appeared in the area between the chromosomes and the central spindle, consistent with other reports. Taken together, these results indicate that EWSR1 may affect mitosis spindle assembly in the progression of spindle dynamics, thereby influencing chromosome alignment.
Knockdown of EWSR1 decreases α-tubulin acetylation
It has been reported that the acetylation of α-tubulin at residue Lys-40 correlated with the stability of MTs.Citation24 Thus, we examined the effects of EWSR1 on α-tubulin acetylation. As shown in , cells transfected with control vector or Myc-EWSR1 were synchronized in mitosis with thymidine-MG132 treatment. The levels of acetylated α-tubulin were obviously higher in cells with EWSR1 overexpression than in control cells. Consistently, compared with synchronized cells transfected with the control siRNA, acetylated α-tubulin was notably decreased in synchronized cells transfected with EWSR1 siRNAs (). Furthermore, EWSR1 siRNA also had no effects on the expression of Ac-tubulin and α-tubulin in asynchronous cells (). Furthermore, the effects of EWSR1 on the acetylation of α-tubulin were also confirmed by immunofluorescence. To observe the level of Ac-tubulin in spindle MTs, MG132 was used to synchronize cells in metaphase. Compared with control cells, the fluorescence intensity of tubulin acetylation was obviously decreased in prometaphase and metaphase cells transfected with EWSR1 siRNAs, resulting in unaligned chromosomes (). Quantitative analyses revealed that the relative ratio of Ac-tubulin to α-tubulin was also significantly decreased in prometaphase and metaphase EWSR1-depleted cells.
Figure 5. EWSR1 affects MT acetylation in mitotic spindles. (A) HeLa cells were transfected with control Myc or Myc-EWSR1 vectors for 12 h. The synchronous cells were analyzed by immunoblotting with the indicated antibodies. (B & C) HeLa cells were transfected with negative control siRNA or EWSR1-specific siRNAs for 24 h. Synchronized cells were subjected to SDS-PAGE, followed by immunoblotting with antibodies as indicated (B). Asynchronous HeLa cell lysates were immunoprecipitated with anti-α-tubulin antibody, and portions of the immunoprecipitates and cell lysates were analyzed by immunoblotting with antibodies as indicated (C). For (A) and (B), the cells were treated by 2.5 mM thymidine for 18 h, released in fresh culture for 8 h, and synchronized at the metaphase with 10 μM MG132 for a further 2 h. (D-F) HeLa cells were transfected with negative control siRNA or EWSR1-specific siRNAs for 24 h. The cells were treated by 2.5 mM thymidine for 18 h, and released in fresh culture for 8 h. To observe the level of Ac-tubulin in spindle MTs, 10 μM MG132 was used for further 2 h to synchronize cells in metaphase. Cells were fixed and stained with anti-Ac-tubulin and anti-α-tubulin antibodies. DNA was stained with Hoechst 33342 (blue) and images were obtained through an inverted fluorescence microscope at the indicated stages of the cell cycle (Scale bar: 10 μm). Quantitative analysis of the relative ratio of Ac-tubulin to α-tubulin in synchronized cells (n = 50) at the indicated stages of the cell cycle. Student t test was used to compare the mean relative values between groups (*p < 0.05, **p < 0.01) (D). The synchronous HeLa cells were incubated with 2 μg/ml α-amanitin for 5 h and subjected to immunoblot with antibodies as indicated (E). The synchronous HeLa cells were subjected to immunoblot with antibodies as indicated (F).
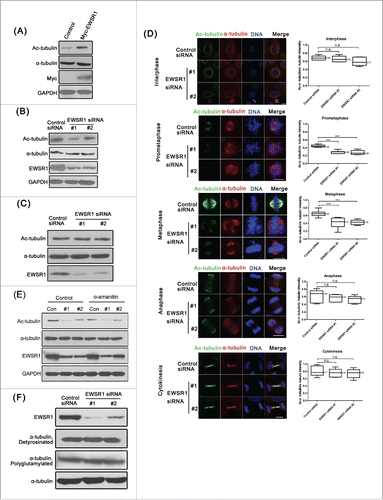
Defects in chromosome alignment may also affect MT acetylation by altering MT stability. To distinguish the cause of MT acetylation, we observed cells in which the chromosomes were fully aligned. As shown in Fig. S6, silencing EWSR1 significantly decreased the level of spindle MT acetylation in cells with properly aligned chromosome, suggesting that chromosome misalignment is the result of reduced MT acetylation induced by EWSR1 deficiency.
Furthermore, to avoid the side effects of MG132 on MT acetylation, HeLa cells were synchronized by thymidine for 18 h and released for 10 h. As shown in Fig. S7, the level of spindle MT acetylation remained significantly lower in mitotic cells transfected with EWSR1 siRNA, which suggests that MG132 treatment was not the reason for the decreased MT acetylation in EWSR1-depleted cells.
Additionally, we also investigated whether the effect of EWSR1 on α-tubulin acetylation was dependent on its transcriptional activity. After treatment with α-amanitin, no obvious changes were found in the inhibition effects of EWSR1-siRNA on Ac-tubulin in mitotic cells (), which suggests that EWSR1 regulates α-tubulin acetylation in a transcription-independent manner.
The occurrence of other post-translational modifications in the mitotic spindle, such as detyrosination and polyglutamylation, has been reported.Citation25 However, knockdown of EWSR1 did not change the detyrosination and polyglutamylation levels of tubulin in mitotic cells (). Taken together, these results indicate a specific role of EWSR1 in the acetylation of spindle MTs in mitotic cells.
EWSR1 regulates acetylation of spindle MTs via HDAC6
Since the acetylation of MT is regulated by histone deacetylase 6 (HDAC6) and Sirtuin 2 (SIRT2)Citation26,27, we investigated whether the EWSR1-mediated acetylation of spindle MTs was associated with these proteins. Tubacin is a specific inhibitor of HDAC6. As shown in , in control cells, treatment with tubacin significantly enhanced MT acetylation in a dose-dependent manner. EWSR1-siRNA apparently inhibited the acetylation of α-tubulin in cells in the absence of tubacin treatment, whereas the inhibition of Ac-tubulin induced by EWSR1 knockdown was obviously weakened in cells treated with 1 μM tubacin, and almost disappeared in cells treated with 2 μM tubacin. Moreover, similar results were also observed in mitotic cells transfected with HDAC6-specific siRNA (). However, SIRT2-siRNA did not appear to exert a significant effect on α-tubulin acetylation in EWSR1-depleted mitotic cells ().
Figure 6. HDAC6 is involved in EWSR1 affection on acetylation of spindle MTs. HeLa cells were transfected with the indicated siRNA for 24 h, and were then were treated by 2.5 mM thymidine for 18 h, released in fresh culture for 8 h and synchronized at the metaphase with 10 μM MG132 for a further 2 h. (A) The synchronous HeLa cells were treated with 1 μM or 2 μM tubacin for 4 h and subjected to immunoblot with antibodies as indicated. (B & C) Cell lysates were prepared and subjected to immunoblot with antibodies as indicated. (D & E) The synchronous HeLa cells were incubated with 2 μg/ml α-amanitin for 5 h. Cells with unaligned chromosomes and multipolar spindles in control (n = 100 cells) and EWSR1-knockdown cells (n = 100 cells) were quantified. (F & G) Synchronous HeLa cells with unaligned chromosomes and multipolar spindles in control (n = 100 cells) and EWSR1-knockdown cells (n = 100 cells) were quantified. The graph shows the percentage of cells with unaligned chromosomes or multipolar spindles. Images were obtained through an inverted fluorescence microscope (Scale bar: 10 μm). (H) EWSR1 and HDAC6 expression levels were assessed by western blotting, and GAPDH was used as the internal reference. The results were represented as the mean ± SD of triplicate experiments. Student t test was used to compare the mean relative values between groups (*p < 0.05, **p < 0.01).
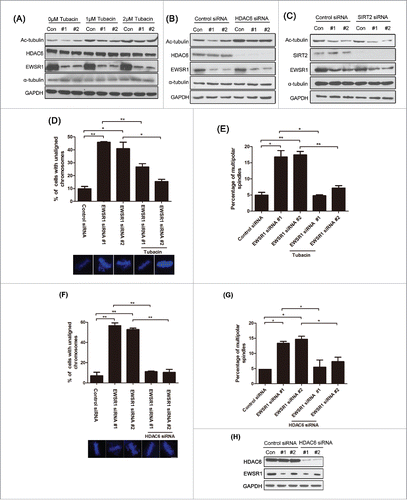
To further confirm the role of HDAC6 in EWSR1-mediated MT acetylation, we examined whether exposure to tubacin could restore the defect in mitosis spindle formation induced by EWSR1-depletion. HeLa cells were synchronized at metaphase and treated with tubacin. As shown in , the percentage of cells with unaligned chromosomes and multipolar spindles were significantly increased after silencing EWSR1, as compared with control cells, until chromosome segregation. Furthermore, the misalignment of chromosomes induced by EWSR1 knockdown was obviously ameliorated in cells treated with tubacin. Similar results were also observed in mitotic cells transfected with HDAC6-specific siRNA ().
Discussion
Most of the earlier studies in EWSR1 were concentrated in the field of cancer biology because its translocations appeared in tumors.Citation28 However, heterozygous EWSR1 loss, resulting in a low level of the EWSR1 protein itself, may also contribute to the development of cancers, and this has not been explored fully. Here we report that EWSR1 plays an important role in mitotic progression independent of its nuclear role. During mitosis, EWSR1 partially localizes on mitotic spindles. Biochemical analyses further demonstrate that EWSR1 can bind to the α-tubulin protein. Reduction of EWRS1 significantly reduces the level of acetyl-α-tubulin on mitotic spindles, indicating that less stable spindle microtubules are assembled. This leads to abnormal spindle formation and results in mitotic arrest.
The EWSR1 protein is mainly located in the nucleus, but it can also translocate to cytoplasm and cell surface.Citation10,29, 30 Leemann-Zakaryan et al. reported a dynamic subcellular distribution of recombinant EWS-yellow fluorescent protein (YFP) fusion protein in different cell lines depending on cell stage, with a predominantly nuclear localization in interphase cells, perichromosomal localization in prometaphase cells, and cytoplasmic localization in metaphase cells.Citation20 However, the subcellular locations of endogenous EWSR1 during mitosis have not been confirmed by fluorescence assays. Our results demonstrated that EWSR1 was distributed throughout the whole cell and was mainly enriched in spindle region during mitotic phase, which was further confirmed by an improved experimental method by which MTs and MT-associated proteins were found to be more stable. Recently, RNA in centrosomes and MT-associated RNA have been identified to play roles in the dynamics of the mitotic spindle.Citation31 Therefore, some researchers have speculated that the RNA-binding feature of EWSR1 might contribute to its location in the centrosome, and that EWSR1 might be involved in centrosome-associated functions by interacting with centrosomal RNA and MTs.Citation20 However, Leemann-Zakaryan et al. reported a direct interaction between EWSR1 and α-tubulin by GST-pull down, following the removal of RNA using RNase A.Citation20 We have also observed this in the present study. Moreover, our immunoprecipitation experiments have also identified the interaction between EWSR1 and α-tubulin, and this interaction was confirmed not only in HeLa cells, but also in L02 cells. Consistent with the lack of colocalization between EWSR1 and MTs in interphase, almost no interaction between EWSR1 and α-tubulin was found in asynchronous cells, suggesting that these proteins interact during mitosis, and that this plays a role in cell cycle regulation.
Mitosis is a dynamic process that mainly depends on the mitotic spindle, a molecular machine assembled from microtubules.Citation32 In mitotic cells, MTs are composed of heterodimers of α/β-tubulin subunits arranged head-to-tail.Citation33 Defective structure and altered dynamics of MTs result in abnormal spindle function, and in turn lead to chromosome alignment errors and cell cycle arrest.Citation34 Depletion of EWSR1 reduced the regrowth ability of spindle MTs, indicating a role for EWSR1 in spindle assembly. Consistent with this, depletion of EWSR1 led to a significant delay in M phase progression, and the expression of either GFP-EWSR1 or GFP-EWSR1ΔNLS rescued the prolonged time from NEB to metaphase resulting from EWSR1 knockdown, suggesting that the cell cycle function of EWSR1 mainly depends on its role outside the nucleus. Moreover, spindle MTs in EWSR1-depleted cells are more sensitive to cold treatment, indicating the role of EWSR1 in kinetochore-microtubule attachment. Kinetochore-microtubule detachment could active the mitotic checkpoint to delay anaphase onset to prevent single chromosomes from being missegregated.Citation35 Knockdown of EWSR1 did not influence the structural integrity of kinetochores, as indicated by the similar fluorescence intensity of checkpoint proteins in these cells compared with control prometaphase cells. It has been reported that the weakening of the checkpoint due to individual unattached kinetochores does not block anaphase onset but lead to increased frequency of aneuploidy.Citation36 In our research, the levels of Mad2 and BubR1 were reduced in EWSR1-depleted cells, but not eliminated, due to the presence of some unaligned chromosomes. We also observed a high ratio of multipolar spindles in EWSR1-depleted cells, which might be the result of weak signal generation at individual unattached kinetochores. It has been reported that tumors and tumor cell lines, including those with chromosomally instability, have a weakened checkpoint signal that is sufficient for maintaining a viable population of cells but allows them to missegregate small numbers of chromosomes per division, resulting in aneuploidy and chromosomal instability.Citation37,38 Therefore, we believe that knockdown of EWSR1 may impair spindle MT assembly and stability; causing tumorigenesis due to the missegregation of a few chromosomes.
As the building block of MTs, tubulin plays a pivotal role in regulating the properties of MTs, such as structure and stability, and MT-based functions, including cell division, ciliary beating, and intracellular trafficking.Citation39 Several factors have been postulated to affect the dynamics of the microtubule system. Tubulin is subjected to a number of post-translational modifications, such as phosphorylation, acetylation, tyrosination, polyglycylation, and polyglutamylation,Citation25 which influence the stability and structure of MT assemblies and thereby alter the dynamic properties and cellular functions of MTs.Citation40 Acetylation of the Lys40 residue in α-tubulin is a well-noted MT modification, and acetylated α-tubulin (Ac-tubulin) is enriched at interpolar and kinetochore MTs in mitotic cells.Citation24 In our research, we showed that depletion of EWSR1 reduced the level of MT acetylation but did not affect the levels of MT polyglutamylation and detyrosination in mitotic cells, indicating that EWSR1 has a specific role in the regulation of MT acetylation. Moreover, EWSR1 is distributed in the nucleus during interphase but is localized to spindle MTs during mitosis. In according with this, depletion of EWSR1 had no effect on MT acetylation in interphase cells. Many microtubule-associated proteins (MAPs), such as Mdp3, Dysferlin, Furry, and MST2, are reported to increase microtubule stability by regulating MT acetylation.Citation40-43 Therefore, we propose that EWSR1 is a new MAP that can regulate MT acetylation in spindles, along with MT stability and density.
Acetylation of polymerized α-tubulin at Lys40 is negatively regulated by the activity of HDAC6.Citation26,42, 44 HDAC6 is a member of the class II histone deacetylase family, which is expressed in a wide range of cells. HDAC6 overexpression leads to the complete deacetylation of microtubules, whereas inhibition of HDAC6 by specific inhibitors, RNAi-mediated protein depletion, or genetic knockout increases microtubule acetylation.Citation26,45, 46 Our results revealed that HDAC6 inhibition by tubacin or specific RNAi was able to efficiently rescue the decrease in α-tubulin acetylation induced by silencing EWSR1 in mitotic cells, which suggests the involvement of EWSR1 in microtubule dynamics via HDAC6. However, the molecular mechanisms are not yet explored. We propose that EWSR1 might competitively bind to spindle MTs, leading to the decreased binding of HDAC6 to the spindle, as has been partially observed in our immunoprecipitation studies (Fig. S8). Overexpression EWSR1 reduced tubulin-HDAC6 interaction and knockdown of EWSR1 increased their association. A possible inhibiting effect on HDAC6 activity by EWSR1 might be excluded, as the association between HDAC6 and EWSR1 was not examined by our immunoprecipitation and GST-pull down studies, which suggest the deficiency of the basis of destruction of deacetylase enzyme activity (Figs. S9 & S10). However the molecular mechanisms are not yet explored fully. For example, the protein-protein interaction domain between EWSR1 and α-tubulin merits further study to distinguish whether EWSR1 and HDAC6 bind the same site of α-tubulin or EWSR1 acts as a scaffold protein to recruit other HDAC6 inhibitors. Besides, among numerous HDACs, HDAC3 is localized on spindle microtubules, and reduction of HDAC3 results in defective kinetochore-microtubule attachment,Citation47 which was also observed in EWSR1-depleted cells in our research, whereas HDAC3 does not alter the acetylation of α-tubulin. Moreover, HDAC6 inhibition by tubacin could rescue the increased percentage of unaligned chromosomes and multipolar spindles in EWSR1-deficient cells, suggesting that the deacetylation of α-tubulin could be the main reason for the irregularities in the mitotic spindle caused by EWSR1 depletion. SIRT2 has also been described as a deacetylase enzyme of α-tubulin in previous studies,Citation48 but SIRT2 depletion had no effects on the reduction of α-tubulin acetylation in EWSR1-deficient cells, indicating that the effect of EWSR1 on spindle MT acetylation is independent on SIRT2 activity. Furthermore, the acetylation of α-tubulin is also controlled by the enzymatic activity of multiple acetyltransferases, including α-TAT1 and the Elongator protein complex;Citation49-51 further studies on the regulatory effects of these proteins on EWSR1 are needed.
EWSR1 plays important roles in the cell cycle through the transcriptional regulation of gene expression, such as CCDC6.Citation52 To distinguish the effect of EWSR1 in mitosis, we examined the changes in α-tubulin acetylation in mitotic cells treated with the polymerase II inhibitor α-amanitin, and observed that α-amanitin treatment did not alter the level of α-tubulin acetylation caused by EWSR1 depletion. These findings suggest that EWSR1 regulates spindle MT acetylation in a transcription-independent manner, but we could not exclude the possibility that the effects of EWSR1 on mitosis might also occur via indirect mechanisms involving the expression of cell cycle-regulating genes or signaling processes.
In conclusion, our study suggests that EWSR1 plays a crucial role in mitotic progression and spindle dynamics, and that EWSR1 promote MT acetylation in the mitotic spindle by inhibiting the activity of HDAC6. These observations may extend our understanding of the mechanism of EWSR1 in mitotic arrest and provide new ideas for developing drugs that target mitotic disorders for tumor treatment.
Materials and methods
Chemical reagents and antibodies
Thymidine (T1895), nocodazole (M1404), MG-132 (C2211), tubacin (SML0065) and α-amanitin (A2263) were purchased from Sigma-Aldrich; paclitaxel (Taxol) (H20130359) was purchased from Bristol-Myers Squibb.
Mouse monoclonal anti-EWSR1 (sc-48404) and anti-Myc (sc-40) were from Santa Cruz Biotechnology; mouse monoclonal anti-acetylation (K40) α-tubulin (T6793) and anti-flag (M2) (F3165) were from Sigma; rabbit polyclonal anti-α-tubulin (AC003), anti-HDAC6 (A1732) and anti-SIRT2 (A0273) were from ABclonal; mouse monoclonal anti-α-tubulin (ZS-8035) was from ZSGB-BIO; mouse monoclonal anti-GAPDH (60004-1-Ig) was from Proteintech; mouse anti-polyglutamylation (AG-20B-0020) was from AdipoGen; rabbit polyclonal anti-BubR1 (A300-386A-T) and anti-Mad2 (A300-300A) was from Bethly; mouse monoclonal anti-Aurora B (611082) was from BD Biosciences; rabbit polyclonal anti-tubulin, detyrosinated (AB3201) was from Millipore; and human anti-centromere (15-234) was from Antibodies Incorporated.
Plasmids and siRNA oligonucleotides
Human full-length EWSR1 was amplified by polymerase chain reaction (PCR) from human liver cDNA, and cloned into the pFLAG-CMV2 (Sigma), pCMV-Myc (Clontech), pEGFP-N1 (Clontech), and pGEX-4T-2 (Clontech) vectors. The siRNA oligos were purchased from GenePharma; their sequences were as follows: EWSR1 siRNA #1: GGG CAA CAA AGC UAU GGA A; EWSR1 siRNA #2: CGC CUA UGC AAC UUC UUA U; HDAC6 siRNA: CAU CCA AGU CCA UCG CAG A; control siRNA: UUC UCC GAA CGU GUC ACG U. The SIRT2 siRNA pool (sc-40988) and control siRNA-A (sc-37007) were purchased from Santa Cruz Biotechnology.
To generate the RNAi-insensitive plasmid for EWSR1, the wobble codons corresponding to the siRNA oligos of EWSR1 were mutated for 4 sites using site-directed mutagenesis in pEGFP-N1-EWSR1 or Myc-EWSR1ΔNLS according to the standard methods (Sbsgene). Two mutation experiments were carried out, and 2 sites were mutated each time. The first pair of primers, corresponding to EWSR1 siRNA #1. were primer #1-1F (5′-GGCATATGGGCAACAGTGCTATGGAACCTATG-3′) and primer #1-1R (5′-CATAGGTTCCATAGCACTGTTGCCCATATGCC-3′). The second pair of primers corresponding to EWSR1 siRNA #1 were primer #1-2F (5′-ATATGGGCAACAGTCGTATGGAACCTATGG-3′) and primer #1-2R (5′-CCATAGGTTCCATACGACTGTTGCCCATAT-3′). The first pair of primers corresponding to EWSR1 siRNA #2 were primer #2-1F (5′-GACCGCCTATGCAACCACTTATGGACAGCCTC-3′) and primer #2-1R (5′-GAGGCTGTCCATAAGTGGTTGCATAGGCGGTC-3′). The second pair of primers corresponding to EWSR1 siRNA #2 were primer #2-2F (5′-CCGCCTATGCAACCAGCTATGGACAGCCTCCC-3′) and primer #2-2R (5′-GGGAGGCTGTCCATAGCTGGTTGCATAGGCGG-3′). Primer sequences for Myc-EWSR1ΔNLS were as follows: EWSR1ΔNLS-F (5′-GGAATTCGGATGGCGTCCACGGATTACAG-3′), and EWSR1ΔNLS-R (5′-CCGCTCGAGCTATCCTCCACGTCCTCCTCTTC-3′).
Cell culture and transfection
HeLa cells were cultured in H-DMEM (Gibco) medium with 10% fetal bovine serum (Gibco) and L02 cells were cultured in RPMI1640 (Gibco) medium with 10% fetal bovine serum. The HeLa/RFP-H2B stable cell line was kindly provided by Dr. Li Teng (China National Center of Biomedical Analysis, Beijing, China).
Transfections with recombinant plasmids were performed using jetPRIME (Polyplus transfection) and transfections with duplexed siRNAs were performed using Lipofectamine RNAiMAX (Invitrogen), according to the manufacturer's instructions.
Cell synchronization
Double thymidine, thymidine-nocodazole or thymidine-MG132 was used to arrest cells in mitotic phase. For the double thymidine arrest experiment, cells were cultured in 2.5 mM thymidine-containing medium for 16 h, and then released into fresh medium for 8 h, subsequently cultured in medium containing 2.5 mM thymidine for 18 h and then released into fresh medium for 10 h. For the thymidine-nocodazole arrest experiment, cells were cultured in 2.5 mM thymidine-containing medium for 18 h, and then released into fresh medium for 3 h, followed by treated with 100 ng/ml nocodazole for 12 h. For the thymidine-MG132 arrest experiment, cells were cultured in 2.5 mM thymidine-containing medium for 18 h, released into fresh medium for 8 h and treated with 10 μM MG-132 for 2 h.
Time-lapse imaging
HeLa/RFP-H2B stable cell lines were seeded in an 8-chambered cover glass (Lab-Tek Chambered Coverglass, Thermo Fisher), and transfected with the indicated siRNA oligos or recombinant plasmids. The cover glass was placed into a sealed growth chamber at 37°C with 5% CO2. Images were obtained every 5 minutes for 12 h using the inverted fluorescence microscope (Nikon Eclipse Ti-E; magnification: 200×) with an UltraView spinning-disc confocal scanner unit (Perkin Elmer). The images were analyzed and processed using Volocity software (Improvision).
Immunofluorescence
Immunofluorescence was performed as previously described with a little change. Briefly, the cells were seeded on glass coverslips in 24-well plate at a density of 1 × 105 cells/well. After specific treatments, the cells were fixed in 4% paraformaldehyde for 10 min, the cells were permeated with 0.3% Triton X-100 for 5 min, and blotted with 3% bovine serum albumin (BSA) for 3 h at room temperature. Then the cells were incubated with the primary antibodies at 4°C overnight and followed by FITC- or TRITC- conjugated secondary antibodies in the dark for 45 min. For the detection of BubR1 and Mad2 localization, cells were fixed with 1% paraformaldehyde for 10 min.Citation53 To better visualize the co-localization of EWSR1-MTs, cells were incubated in PEM buffer (100 mM PIPES, pH 6.8, 5 mM EGTA, 2 mM MgCl2, 5 nM taxol, and 0.05% Triton X-100) for 20–30 s at room temperature before fixing as previously described.Citation54 DNA was stained with Hoechst 33342 (KenGEN) in the dark for 15 min. Confocal imaging was performed using a fluorescence microscope (Nikon Eclipse Ti-E; magnification: 600×). Green fluorescence was observed following excitation at 488 nm with a 505–530 nm barrier filter, and red fluorescence was simultaneously observed by excitation at 543 nm with 560 nm barrier filter. The images were analyzed using Image J 1.49m (National Institutes of Health).
Immunoprecipitation and western blotting
Immunoprecipitation and western blotting were performed as previously described.Citation55
FACS analysis
For cell cycle analysis, cells were fixed in 70% ethanol overnight, treated with 200 mg/ml DNase-free RNase A (Sigma) at 37°C for 30 min and stained with 10 mg/ml propidium iodide (Sigma). DNA contents were analyzed using a BD FACSCalibur flow cytometer and CellQuest software (BD Biosciences).
Statistical analysis
All data were expressed as the mean ± standard deviation (SD) and analyzed using SPSS 19.0 (SPSS Inc., Chicago, IL). Student's t test was performed for comparisons between 2 groups and one-way ANOVA followed by the LSD test was conducted to evaluate differences among multiple groups. A value of P < 0.05 was considered statistically significant.
Disclosure of potential conflicts of interest
No potential conflicts of interest were disclosed.
KCCY_A_1200774_supplement.zip
Download Zip (7 MB)Funding
This work was supported by grants from the China National Basic Research Program (2013CB910801), the State Key Laboratory of Proteomics (SKLP-K201404).
References
- Bertolotti A, Lutz Y, J.Heard D, Chambon P, Toral L. hTAF(II)68, a novel RNA-ssDNA-binding protein with homology to the pro-oncoproteins TLS-FUS and EWS is associated with both TFIID and RNA polymerase II. EMBO J 1996; 15:5022-31; PMID:8890175
- Aurias A, Rimbaut C, Buffe D, Zucker J-M, Mazabraud A. Translocation involving chromosome 22 in Ewing's Sarcoma. Cancer Genetics and Cytogenetics 1984; 12:21-5; PMID:6713357; http://dx.doi.org/10.1016/0165-4608(84)90003-7
- Thompson AD, Teitell MA, Arvand A, Denny CT. Divergent Ewing's sarcoma EWS/ETS fusions confer a common tumorigenic phenotype on NIH3T3 cells. Oncogene 1999; 18:5506-13; PMID:10523827; http://dx.doi.org/10.1038/sj.onc.1202928
- Zucman J, Delattre O, Desmaze C, Epstein AL, Stenman G, Speleman F, Fletchers CDM, Aurias A, Thomas G. EWS and ATF-1 gene fusion induced by t(12;22) translocation in malignant melanoma of soft parts. Nat Genet 1993; 4:341-5; PMID:8401579; http://dx.doi.org/10.1038/ng0893-341
- Gerald WL, Rosai J, Ladanyi M. Characterization of the genomic breakpoint and chimeric transcripts in the EWS-WT1 gene fusion of desmoplastic small round cell tumor. Proc Natl Acad Sci 1995; 92:1028-32; PMID:7862627; http://dx.doi.org/10.1073/pnas.92.4.1028
- Filion C, Labelle Y. Identification of genes regulated by the EWS/NR4A3 fusion protein in extraskeletal myxoid chondrosarcoma. Tumor Biol 2012; 33:1599-605; http://dx.doi.org/10.1007/s13277-012-0415-2
- Cin PD, Sciot R, Panagopoulos I, Aman P, Samson I, Mandahl N, Mitelman F, Van den Berghe H, M.Fletcher CD. Additional evidence of a variant translocation t(12;22) with EWS/CHOP fusion in myxoid liposarcoma: clinicopathological features. Pathol 1997; 182:437-41; http://dx.doi.org/10.1002/(SICI)1096-9896(199708)182:4%3c437::AID-PATH882%3e3.0.CO;2-X
- Andersson MK, Stahlberg A, Arvidsson Y, Olofsson A, Semb H, Stenman G, Nilsson O, Aman P. The multifunctional FUS, EWS and TAF15 proto-oncoproteins show cell type-specific expression patterns and involvement in cell spreading and stress response. BMC Cell Biol 2008; 9:37; PMID:18620564; http://dx.doi.org/10.1186/1471-2121-9-37
- Melot T, Dauphinot L, Sévenet N, Radvanyi F, Delattre O. Characterization of a new brain-pecific isoform of the EWS oncoprotein. Eur J Biochem 2001; 268:3483-9; PMID:11422378; http://dx.doi.org/10.1046/j.1432-1327.2001.02251.x
- Zakaryan RP, Gehring H. Identification and characterization of the nuclear localization/retention signal in the EWS proto-oncoprotein. J Mol Biol 2006; 363:27-38; PMID:16965792; http://dx.doi.org/10.1016/j.jmb.2006.08.018
- Bertolotti A, Melot T, Acker J, Vigneron M, Delattre O, Tora L. EWS, but not EWS-FLI-1, is associated with both TFIID and RNA polymerase II interactions between two members of the TET family, EWS and hTAFII68, and subunits of TFIID and RNA polymerase II complexes. Mol Cell Biol 1998; 18:1489-97; PMID:9488465; http://dx.doi.org/10.1128/MCB.18.3.1489
- Lee J, Rhee BK, Bae GY, Han YM, Kim J. Stimulation of Oct-4 activity by Ewing's sarcoma protein. Stem Cells 2005; 23:738-51; PMID:15917470; http://dx.doi.org/10.1634/stemcells.2004-0375
- Gascoyne DM, Thomas GR, Latchman DS. The effects of Brn-3a on neuronal differentiation and apoptosis are differentially modulated by EWS and its oncogenic derivative EWS/Fli-1. Oncogene 2004; 23:3830-40; PMID:15021903; http://dx.doi.org/10.1038/sj.onc.1207497
- Chansky HA, Hu M, Hickstein DD, Yang L. Oncogenic TLS/ERG and EWS/Fli-1 fusion proteins inhibit RNA splicing mediated by YB-1 protein. Cancer Res 2001; 61:3586-90; PMID:11325824
- Sohna EJ, Park J, Kang S-I, Wu Y-P. Accumulation of pre-let-7g and downregulation of mature let-7g with the depletion of EWS. Biochem Biophys Res Commun 2012; 426:89-93; PMID:22910415; http://dx.doi.org/10.1016/j.bbrc.2012.08.041
- Spahn L, Petermann R, Siligan C, Schmid JA, Aryee DNT, Kovar H. Interaction of the EWS NH2 terminus with BARD1 links the Ewing's Sarcoma gene to a common tumor suppressor pathway. Cancer Res 2002; 62:4583-7; PMID:12183411
- Park H, Turkalo TK, Nelson K, Folmsbee SS, Robb C, Roper B, Azuma M. Ewing sarcoma EWS protein regulates midzone formation by recruiting Aurora B kinase to the midzone. Cell Cycle 2014; 13:2391-9; PMID:25483190; http://dx.doi.org/10.4161/cc.29337
- Li H, Watford W, Li C, Parmelee A, Bryant MA, Chuxia Deng, O'Shea J, Lee SB. Ewing sarcoma gene EWS is essential for meiosis and B lymphocyte development. J Clin Investig 2007; 117:1314-23; PMID:17415412; http://dx.doi.org/10.1172/JCI31222
- Azuma M, Embree LJ, Sabaawy H, Hickstein DD. Ewing sarcoma protein Ewsr1 maintains mitotic integrity and proneural cell survival in the Zebrafish embryo. PloS one 2007; 2:e979; PMID:17912356; http://dx.doi.org/10.1371/journal.pone.0000979
- Leemann-Zakaryan RP, Pahlich S, Sedda MJ, Quero L, Grossenbacher D, Gehring H. Dynamic subcellular localization of the Ewing sarcoma proto-oncoprotein and its association with and stabilization of microtubules. J Mol Biol 2009; 386:1-13; PMID:19133275; http://dx.doi.org/10.1016/j.jmb.2008.12.039
- Belyanskaya LL, Gehrig PM, Gehring H. Exposure on Cell Surface and Extensive Arginine Methylation of Ewing Sarcoma (EWS) Protein. J Biol Chem 2001; 276:18681-7; PMID:11278906; http://dx.doi.org/10.1074/jbc.M011446200
- Couthouis J, Hart MP, Erion R, King OD, Diaz Z, Nakaya T, Ibrahim F, Kim HJ, Mojsilovic-Petrovic J, Panossian S, et al. Evaluating the role of the FUS/TLS-related gene EWSR1 in amyotrophic lateral sclerosis. Hum Mol Genet 2012; 21:2899-911; PMID:22454397; http://dx.doi.org/10.1093/hmg/dds116
- Fu J, Bian M, Jiang Q, Zhang C. Roles of Aurora Kinases in Mitosis and Tumorigenesis. Mol Cancer Res 2007; 5:1-10; PMID:17259342; http://dx.doi.org/10.1158/1541-7786.MCR-06-0208
- Soppina V, Herbstman JF, Skiniotis G, Verhey KJ. Luminal localization of alpha-tubulin K40 acetylation by cryo-EM analysis of fab-labeled microtubules. PloS one 2012; 7:e48204; PMID:23110214; http://dx.doi.org/10.1371/journal.pone.0048204
- Hammond JW, Cai D, Verhey KJ. Tubulin modifications and their cellular functions. Curr Opin Cell Biol 2008; 20:71-6; PMID:18226514; http://dx.doi.org/10.1016/j.ceb.2007.11.010
- Hubbert C, Guardiola A, Shao R, Kawaguchi Y, Ito A, Nixon A, Yoshida M, Wang X-F, Yao T-P. HDAC6 is a microtubule-associated deacetylase. Nature 2002; 417:455-8; PMID:12024216; http://dx.doi.org/10.1038/417455a
- Dryden SC, Nahhas FA, Nowak JE, Goustin AS, Tainsky MA. Role for human SIRT2 NAD-dependent deacetylase activity in control of mitotic exit in the cell cycle. Mol Cell Biol 2003; 23:3173-85; PMID:12697818; http://dx.doi.org/10.1128/MCB.23.9.3173-3185.2003
- Fisher C. The diversity of soft tissue tumours with EWSR1 rearrangements: a review. Histopathology 2014; 64:134-50; PMID:24320889; http://dx.doi.org/10.1111/his.12269
- Felsch JS, Lane WS, Peralta EG. Tyrosine kinase Pyk2 mediates G-protein-coupled receptor regulation of the Ewing sarcoma RNAbinding protein EWS. Curr Biol 1999; 9:485-8; PMID:10322114; http://dx.doi.org/10.1016/S0960-9822(99)80214-0
- Belyanskaya LL, Delattre O, Gehring H. Expression and subcellular localization of Ewing sarcoma (EWS) protein is affected by the methylation process. Exp Cell Res 2003; 288:374-81; PMID:12915128; http://dx.doi.org/10.1016/S0014-4827(03)00221-0
- Oeffinger M, Fatica A, Rout MP, Tollervey D. Yeast Rrp14p is required for ribosomal subunit synthesis and for correct positioning of the mitotic spindle during mitosis. Nucleic Acids Res 2007; 35:1354-66; PMID:17272295; http://dx.doi.org/10.1093/nar/gkl824
- Hyman AA, Karsenti E. Morphogenetic Properties of Microtubules and Mitotic Spindle Assembly. Cell 1996; 84:401-10; PMID:8608594; http://dx.doi.org/10.1016/S0092-8674(00)81285-4
- Kline-Smith SL, Walczak CE. Mitotic spindle assembly and chromosome segregation: refocusing on microtubule dynamics. Mol Cell 2004; 15:317-27; PMID:15304213; http://dx.doi.org/10.1016/j.molcel.2004.07.012
- Wu G, Lin Y-T, Wei R, Chen Y, Shan Z, Lee W-H. Hice1, a novel microtubule-associated protein required for maintenance of spindle integrity and chromosomal stability in human cells. Mol Cell Biol 2008; 28:3652-62; PMID:18362163; http://dx.doi.org/10.1128/MCB.01923-07
- Petsalaki E, Zachos G. Chk2 prevents mitotic exit when the majority of kinetochores are unattached. J Cell Biol 2014; 205:339-56; PMID:24798733; http://dx.doi.org/10.1083/jcb.201310071
- Ryan SD, Britigan EM, Zasadil LM, Witte K, Audhya A, Roopra A, Weaver BA. Up-regulation of the mitotic checkpoint component Mad1 causes chromosomal instability and resistance to microtubule poisons. Proc Natl Acad Sci U S A 2012; 109:E2205-14; PMID:22778409; http://dx.doi.org/10.1073/pnas.1201911109
- Kops GJ, Weaver BA, Cleveland DW. On the road to cancer: aneuploidy and the mitotic checkpoint. Nat Rev Cancer 2005; 5:773-85; PMID:16195750; http://dx.doi.org/10.1038/nrc1714
- Weaver BAA, Cleveland DW. Decoding the links between mitosis, cancer, and chemotherapy: The mitotic checkpoint, adaptation, and cell death. Cancer Cell 2005; 8:7-12
- Hammond JW, Cai D, Verhey KJ. Tubulin modifications and their cellular functions. Curr Opin Cell Biol 2008; 20:71-6; PMID:16023594; http://dx.doi.org/10.1016/j.ccr.2005.06.011
- Nagai T, Ikeda M, Chiba S, Kanno S, Mizuno K. Furry promotes acetylation of microtubules in the mitotic spindle by inhibition of SIRT2 tubulin deacetylase. J Cell Sci 2013; 126:4369-80; PMID:23886946; http://dx.doi.org/10.1242/jcs.127209
- Sun X, Shi X, Liu M, Li D, Zhang L, Liu X, Zhou J. Mdp3 is a novel microtubule-binding protein that regulates microtubule assembly and stability. Cell Cycle 2014; 10:3929-37; http://dx.doi.org/10.4161/cc.10.22.18106
- Di Fulvio S, Azakir BA, Therrien C, Sinnreich M. Dysferlin interacts with histone deacetylase 6 and increases alpha-tubulin acetylation. PloS one 2011; 6:e28563; PMID:22174839; http://dx.doi.org/10.1371/journal.pone.0028563
- Chiba S, Ikeda M, Katsunuma K, Ohashi K, Mizuno K. MST2- and Furry-mediated activation of NDR1 kinase is critical for precise alignment of mitotic chromosomes. Curr Biol 2009; 19:675-81; PMID:19327996; http://dx.doi.org/10.1016/j.cub.2009.02.054
- Tokesi N, Lehotzky A, Horvath I, Szabo B, Olah J, Lau P, Ovadi J. TPPP/p25 promotes tubulin acetylation by inhibiting histone deacetylase 6. J Biol Chem 2010; 285:17896-906; PMID:20308065; http://dx.doi.org/10.1074/jbc.M109.096578
- Deakin NO, Turner CE. Paxillin inhibits HDAC6 to regulate microtubule acetylation, Golgi structure, and polarized migration. J Cell Biol 2014; 206:395-413; PMID:25070956; http://dx.doi.org/10.1083/jcb.201403039
- Bobrowska A, Paganetti P, Matthias P, Bates GP. Hdac6 knock-out increases tubulin acetylation but does not modify disease progression in the R6/2 mouse model of Huntington's disease. PloS one 2011; 6:e20696; PMID:21677773; http://dx.doi.org/10.1371/journal.pone.0020696
- Ishii S, Kurasawa Y, Wong J, Yu-Lee LY. Histone deacetylase 3 localizes to the mitotic spindle and is required for kinetochore-microtubule attachment. Proc Natl Acad Sci U S A 2008; 105:4179-84; PMID:18326024; http://dx.doi.org/10.1073/pnas.0710140105
- North BJ, Denu JM, Marshall BL, Verdin E. The Human Sir2 Ortholog, SIRT2, Is an NAD+-Dependent Tubulin Deacetylase. Mol Cell 2003; 11: 437-44; PMID:12620231; http://dx.doi.org/10.1016/S1097-2765(03)00038-8
- Castro-Castro A, Janke C, Montagnac G, Paul-Gilloteaux P, Chavrier P. ATAT1/MEC-17 acetyltransferase and HDAC6 deacetylase control a balance of acetylation of alpha-tubulin and cortactin and regulate MT1-MMP trafficking and breast tumor cell invasion. Eur J Cell Biol 2012; 91:950-60; PMID:22902175; http://dx.doi.org/10.1016/j.ejcb.2012.07.001
- Akella JS, Wloga D, Kim J, Starostina NG, Abbott SL, Morrissette NS, Dougan ST, Kipreos ET, Gaertig J. MEC-17 is an α-tubulin acetyltransferase. Nature 2010; 467:218-22; PMID:20829795; http://dx.doi.org/10.1038/nature09324
- Solinger JA, Paolinelli R, Kloss H, Scorza FB, Marchesi S, Sauder U, Mitsushima D, Capuani F, Sturzenbaum SR, Cassata G. The Caenorhabditis elegans Elongator complex regulates neuronal alpha-tubulin acetylation. PLoS Genet 2010; 6:e1000820; PMID:20107598; http://dx.doi.org/10.1371/journal.pgen.1000820
- Duggimpudi S, Larsson E, Nabhani S, Borkhardt A, Hoell JI. The cell cycle regulator CCDC6 is a key target of RNA-binding protein EWS. PloS one 2015; 10:e0119066; PMID:25751255; http://dx.doi.org/10.1371/journal.pone.0119066
- Gao Y-F, Li T, Chang Y, Wang Y-B, Zhang W-N, Li W-H, He K, Mu R, Zhen C, Man J-H, et al. Cdk1-phosphorylated CUEDC2 promotes spindle checkpoint inactivation and chromosomal instability. Nat Cell Biol 2011; 13:924-33; PMID:21743465; http://dx.doi.org/10.1038/ncb2287
- Jiang H, He X, Wang S, Jia J, Wan Y, Wang Y, Zeng R, YatesIII J, Zhu X, Zheng Y. A microtubule-associated zinc finger protein, BuGZ, regulates mitotic chromosome alignment by ensuring Bub3 stability and kinetochore targeting. Dev Cell 2014; 28:268-81; PMID:24462186; http://dx.doi.org/10.1016/j.devcel.2013.12.013
- Zhang J, Cao M, Dong J, Li C, Xu W, Zhan Y, Wang X, Yu M, Ge C, Ge Z, et al. ABRO1 suppresses tumourigenesis and regulates the DNA damage response by stabilizing p53. Nat Commun 2014; 5:5059; PMID:25283148; http://dx.doi.org/10.1038/ncomms6059