ABSTRACT
Fidgetin-like 1 (FIGL-1) is a homolog of fidgetin, an AAA protein that was identified as the protein encoded by the gene mutated in fidget mice. Because the phenotypes of fidget mice are reminiscent of the phenotypes of ciliopathy diseases, and because fidgetin has microtubule-severing activity, we hypothesize that these proteins participate in cilia-related processes. Indeed, overexpression of FIGL-1 interfered with ciliogenesis in cultured cells. In particular, overexpressed FIGL-1 strongly accumulated at the centrosome, and, when highly expressed, perturbed the localization of centrosomal proteins such as pericentrin, CP110, and centrin. Using a polyclonal antibody against human FIGL-1, we found that endogenous FIGL-1 localized preferentially around the mother centriole. Consistently, depletion of FIGL-1 by shRNA treatment enhanced ciliogenesis in HEK293T cells. By checking the integrity of the cytoplasmic microtubule network in FIGL-1-overexpressing cells, we found that FIGL-1 probably has microtubule-severing activity, as suggested by its sequence homology with other microtubule-severing proteins. Furthermore, we showed that overexpression of FIGL-1 in zebrafish embryo decreased the length of cilia and perturbed the heart laterality. Taken together, these results demonstrate that FIGL-1 is a new centrosomal protein and inhibits ciliogenesis. These results extend the already long list of centrosomal proteins and provide new insights into the regulation of ciliogenesis.
Introduction
AAA (ATPase associated with various cellular activities) proteins are a huge family of various proteins containing one or more conserved AAA domains. With the energy derived from ATP hydrolysis in their AAA domains, AAA proteins play important roles in many fundamental cellular events, including protein unfolding and degradation, membrane fusion, nucleosome remodeling, and microtubule severing.Citation1,2 Therefore, dysfunction of some AAA proteins leads to abnormal physiological conditions and diseases. Some AAA proteins were indeed identified by cloning genes mutated in disease conditions. One such AAA protein is fidgetin, whose gene was cloned from fidget mutant mice.Citation3 These mutant mice exhibit a series of developmental phenotypes, such as polydactyly, small eyes, and reduced or absent semicircular canals.Citation4,5 It is interesting to note that these phenotypes of fidget mutant mice resemble the typical phenotypes of ciliopathy.Citation6 On the other hand, based on sequence similarity, fidgetin was classified into subfamily 7 of the AAA superfamily, which includes the microtubule-severing proteins spastin and katanin.Citation7 Fidgetin has microtubule-severing activity in vitroCitation8 and in vivo,Citation9 and localizes at the centrosome in cells.Citation8 Given that the centrosome functions as the basal body for ciliogenesis and cilia are microtubule-based organelles,Citation10 it seems likely that fidgetin is involved in ciliogenesis and the phenotypes of fidget mice are caused by an abnormality in ciliogenesis.
Fidgetin-like 1 (FIGL-1) is a homologous protein of fidgetin,Citation3 but is less well-studied. While Caenorhabditis elegans FIGL-1 is a nuclear protein and controls the mitotic progression in the germ line,Citation11,12 mouse FIGL-1 is more cytoplasmic at least when overexpressedCitation13 and is probably involved in the control of male meiosis.Citation14 These discrepancies are likely related with their different biochemical properties.Citation15 Despite these studies, the molecular function of FIGL-1 is far from clear yet. In particular, its potential function in ciliogenesis is totally uncharacterized.
In this study, we focused on the molecular function of human FIGL-1. We first confirmed that the overexpressed FIGL-1 markedly disturbs ciliogenesis and the centrosome structure. Then, we found that endogenous FIGL-1 localizes to the centrosome in cells, preferentially to the mother centriole. Depletion of FIGL-1 enhances ciliogenesis and slows cilia disassembly. This negative regulation of ciliogenesis by FIGL-1 is probably due to its microtubule-disassembly activity and correlates with its expression level. Furthermore, in zebrafish embryos, overexpressed FIGL-1 compromises the length of cilia and interferes with the heart laterality. These results establish that human FIGL-1 is a centrosome protein involved in ciliogenesis.
Results
Overexpressed FIGL-1 has inhibitory effects on ciliogenesis
To quickly examine the effects of FIGL-1 on ciliogenesis, this protein was tagged with GFP at the N-terminus and overexpressed in the NIH-3T3 and HEK293T cell lines. Consistent with a previous study,Citation13 overexpressed FIGL-1 was widely localized throughout the entire cell. However, unlike in control cells expressing GFP alone, cells expressing a high level of the GFP-FIGL-1 fusion protein often exhibited a bright green dot close to the nucleus, suggesting a preferred localization of FIGL-1 protein ( and Fig. S1). When the formation of primary cilia was induced by serum starvation for 24 h, overexpression of FIGL-1 strongly inhibited ciliogenesis, based on immunostaining with an antibody against acetylated tubulin ( and Fig. S1). While about 50% of untransfected cells and GFP-expressing cells developed primary cilia, overexpression of FIGL-1 decreased this percentage to less than 20% ( and Fig. S1). This result suggests that FIGL-1 protein may be related with ciliogenesis.
Figure 1. The effects of FIGL-1 overexpression. (A) NIH-3T3 cells were transfected with plasmids to overexpress GFP or GFP-FIGL-1 for 24 h, serum-starved for 24 h to induce the formation of primary cilia, and stained for acetylated tubulin (Ac-tub, magenta), pericentrin (PCNT, red) and DNA (blue). Scale bar: 7.5 μm. (B) The percentage of ciliated NIH-3T3 cells is reduced by overexpression of FIGL-1. Error bars represent the standard deviation. (C) HEK293T cells overexpressing GFP were immunostained for pericentrin (PCNT, red) and DNA (blue). Scale bar: 10 μm. (D and E) HEK293T cells were transfected with a GFP-FIGL-1-expressing vector for 24 h and immunostained for pericentrin (PCNT, red) and DNA (blue). FIGL-1 at a low expression level colocalizes with pericentrin (panel D, scale bar: 5 μm), but no pericentrin staining is observed in cells with a high expression level of FIGL-1 (panel E, scale bar: 10 μm). (F) HEK293T cells overexpressing GFP-FIGL-1 were immunostained for centrin (red) and DNA (blue). Scale bar: 7.5 μm. (G) HEK293T cells overexpressing GFP-FIGL-1 were immunostained for CP110 (red) and DNA (blue). Scale bar: 10 μm.
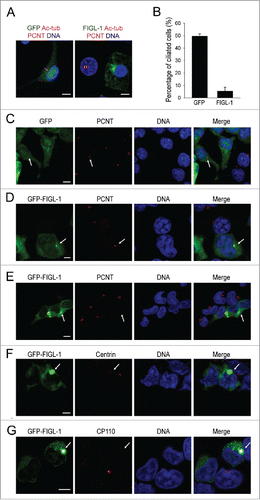
Overexpressed FIGL-1 damages the centrosome
To determine the cause of decreased primary cilia formation in cells overexpressing FIGL-1, we examined the status of the centrosome, which functions as the basal body during ciliogenesis, by checking the localization of the centrosome protein pericentrin. In GFP-overexpressing control cells, pericentrin staining showed a normal single dot indicating the position of the centrosome (). To our surprise, when FIGL-1 was overexpressed such that a green dot was visible, this dot colocalized with pericentrin, suggesting that FIGL-1 preferably localizes in the centrosome (). However, when the expression level of FIGL-1 was very high such that a bright green dot was visible, staining of pericentrin was often lost (), implying that overdose of FIGL-1 protein interferes with the normal positioning of pericentrin. Furthermore, staining of 2 other centrosome proteins, centrin and CP110, also disappeared in cells highly overexpressing FIGL-1 protein (). These results strongly indicate that overexpression of FIGL-1 severely damages the centrosome.
Endogenous FIGL-1 localizes to the mother centriole of the centrosome
To clarify the localization of endogenous FIGL-1, we generated a rabbit polyclonal antibody against human FIGL-1 protein. This antibody recognized a single band of a molecular weight consistent with the theoretical size of human FIGL-1 protein (74 kD) in western blotting of both ARPE19 and HEK293T cell lysates, but no band was detected with mouse NIH-3T3 cell lysate (). Since the sequence of the epitope for antibody generation is not conserved in mouse FIGL-1, this western blotting result suggested the satisfactory specificity of this polyclonal antibody. In fixed ARPE19 and HEK293 cells, immunostaining with this antibody revealed that FIGL-1 colocalized well with pericentrin, confirming that endogenous FIGL-1 is indeed a centrosome protein ( and Fig. S2).
Figure 2. Subcellular localization of endogenous FIGL-1. (A) Western blotting with a polyclonal antibody against human FIGL-1 detected endogenous FIGL-1 protein from ARPE19 and HEK293T cell lysate, but not from mouse NIH-3T3 cell lysate. (B) ARPE19 cells were immunostained for endogenous FIGL-1 (red) and pericentrin (PCNT, green), which clearly colocalize. Scale bar: 5 μm. The lower small panels are high magnification images of the staining area indicated by the arrow. (C) The colocalization of FIGL-1 with centrin at different stages in ARPE19 cells was visualized by immunofluorescence. Scale bar: 5 μm. (D) ARPE19 cells were serum-starved for 24 h to induce the formation of primary cilia, and then immunostained for endogenous FIGL-1 (red), acetylated tubulin (Ac-tub, green), and DNA (blue), showing that FIGL-1 is enriched at the mother centriole. Scale bar: 5 μm. (E) Left: SIM image of G2 phase ARPE19 cells stained for FIGL-1 (red) and centrin (green). Scale bar: 5 μm. Right top: a high magnification image of the staining area in the left panel indicated by the arrow. Bar: 0.5 μm. Right bottom: the orthogonal view of the top panel sectioned at the position of the arrow.
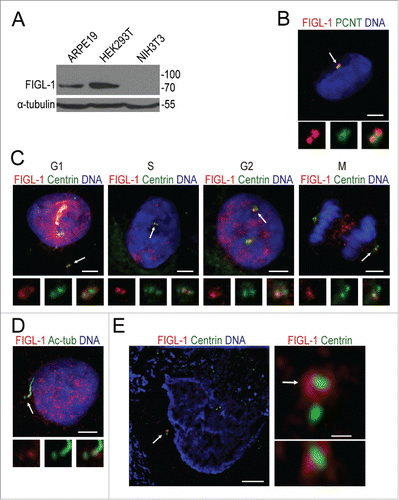
Next, we detected the localization of FIGL-1 at different stages of the cell cycle in ARPE19 cells () using the canonical centriolar marker centrin as a reference. In G1 phase cells, anti-FIGL-1 antibody staining exhibited 2 separate dots that overlapped substantially with centrin. However, from S phase to M phase, although the number of centrin dots doubled due to the duplication of centrioles, there were always 2 FIGL-1 foci in each cell, which overlapped with only one centrin dot in each pair. In particular, when the 2 pairs of centrioles were still engaged in S phase cells, the FIGL-1 foci were close to the central 2, but not the outer 2, of the 4 centrin dots, suggesting that FIGL-1 preferentially localizes on the mother centriole. Indeed, when primary cilia formed after serum withdrawal, FIGL-1 accumulated around the centriole at the base of the cilium, i.e., the mother centriole (). These results establish that FIGL-1 is a mother centriole-preferred centrosome protein.
To determine the precise localization of FIGL-1, we sought to observe the localization of endogenous FIGL-1 protein using super-resolution structured illumination microscopy (SIM). In G2 phase ARPE19 cells, which had 2 separate pairs of centrin dots, SIM imaging confirmed that FIGL-1 localized to only one centriole of each pair (). In addition, SIM images demonstrated that FIGL-1 formed a ring around the centriole marker protein centrin. We notice that the diameter of FIGL-1 ring (about 500 nm) is comparable to that of a typical pericentriolar material (PCM) protein pericentrin.Citation16,17 Since PCM surrounds mainly around the mother centriole in S and G2 phases when centriole duplication is occurring,Citation16 FIGL-1 is probably a PCM protein.
Depletion of FIGL-1 promotes ciliogenesis
The preferred positioning of FIGL-1 strongly suggests it has a mother centriole-related cellular function. To explore the function of FIGL-1, we depleted this protein using shRNA targeting the coding region of FIGL-1 (). Under normal culture conditions, this shRNA treatment did not cause any abnormality in pericentrin or CP110 (Fig. S3A and S3B). Furthermore, the organization of the 9 microtubule triplets in the centriole of FIGL-1-depleted cells was indistinguishable from that in control cells, implying that FIGL-1 is not an essential protein for maintenance of the centrosome (Fig. S3C). Consistently, the cell cycle was not affected by FIGL-1 depletion (Fig. S3D).
Figure 3. RNA interference of FIGL-1 promotes ciliogenesis. (A) HEK293T cells were transfected with control shRNA, FIGL-1-targeted shRNA1, or FIGL-1-targeted shRNA2 for 72 h, and then examined by western blotting for FIGL-1. (B) HEK293T cells were transfected with control shRNA or FIGL-1-targeted shRNA for 72 h, serum-starved for 24 h to induce the formation of primary cilia, and immunostained for acetylated tubulin (Ac-tub, red). Scale bars: 10 μm. (C) The percentage of ciliated HEK293T cells is increased by FIGL-1-targeted shRNA treatment. Error bars represent the standard deviation. (D) HEK293T cells were transfected with control shRNA or FIGL-1-targeted shRNA for 72 h, and then serum-starved for 24 h to induce the formation of primary cilia. Thereafter, serum was added to the medium to induce the absorption of cilia. At the indicated amount of time after serum re-addition, cells were fixed and immunostained for acetylated tubulin (Ac-tub, red). Scale bar: 10 μm. (E) Time course of cilia disassembly after serum addition.
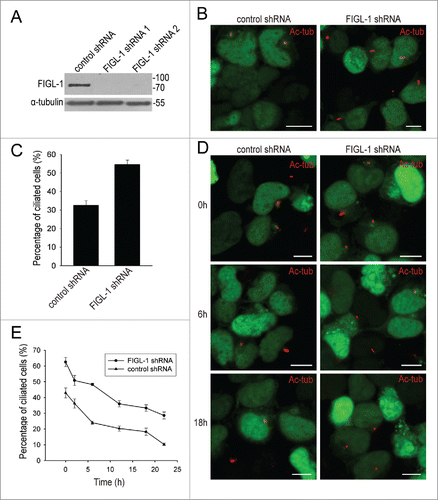
However, when the formation of primary cilia was induced by serum starvation, FIGL-1 depletion enhanced ciliogenesis. While about 35% of cells transfected with control shRNA developed cilia, more than 50% of cells transfected with FIGL-1-targeted shRNA were ciliated (). This suggests that the presence of endogenous FIGL-1 on the mother centriole somehow inhibits the assembly of primary cilia. To further test whether the disassembly of cilia is affected by FIGL-1, we added serum to serum-starved cells in order to induce cilia absorption and re-entry into the cell cycle. In comparison with control cells, cells transfected with FIGL-1-targeted shRNA exhibited slower cilia resorption (). Together, these results demonstrate that FIGL-1 protein disfavors ciliogenesis by inhibiting cilia assembly and assisting cilia disassembly.
The centrosome localization signal resides in the N-terminal part of FIGL-1
To understand the mechanism underlying the functions of FIGL-1, we investigated its localization mechanism. The only predictable domain of FIGL-1 is the conserved AAA domain in the C-terminal half of this protein; therefore, we cloned its N-terminal part (residues 1–374, FIGL-1-Nter) and its AAA domain (residues 375–674, FIGL-1-AAA) separately, and checked the localization of these GFP fusion proteins expressed in HEK293T cells. While FIGL-1-AAA was diffusely distributed throughout the entire cell, different localizations were observed for overexpressed FIGL-1-Nter (Fig. S4). In some cells, FIGL-1-Nter showed no distinct localization, being spread throughout the entire cell. However, in other cells, it exhibited definite centrosome localization, demonstrating that the centrosome localization signal of the FIGL-1 protein resides in its N-terminal part.
FIGL-1 functions in ciliogenesis partly through its microtubule-severing activity
FIGL-1 was initially discovered due to its homology with the microtubule-severing protein fidgetin. However, it remains unknown whether FIGL-1 is also a microtubule-severing protein. To further investigate the possible mechanism by which FIGL-1 inhibits ciliogenesis, we examined first whether it has any microtubule-severing activity in cells. By using an antibody against acetylated tubulin to stain the microtubule network as well as the centrosome, we found that when overexpressed FIGL-1 was strongly accumulated in the centrosome, rather than being spread throughout the cell, the cytoplasmic microtubule network largely disappeared (). By contrast, overexpression of FIGL-1-Nter, which is devoid of the AAA domain and thus of enzymatic activity, had no effect on the cytoplasmic microtubule network () or the centrosome (). The inhibitory effect of FIGL-1-Nter on ciliogenesis was also weaker than that of full-length FIGL-1 (). On the other hand, we prepared recombinant FIGL-1 protein from E. coli to verify the biochemical activity directly in vitro. Unfortunately, the microtubule-severing event was not observed with FIGL-1, which is in clear contrast with spastin, a well-established microtubule-severing protein tested in parallel (data not shown). One possible reason is that functional FIGL-1 would need some essential cofactor or post-translational modification, which is absent in the in vitro circumstance. However, FIGL-1 cosedimented with microtubules (Fig. S5), strongly indicating their direct interaction. Taken together, these results suggest that FIGL-1 is highly likely a microtubule-severing protein in cells.
Figure 4. The mechanism underlying FIGL-1 function in ciliogenesis. (A) ARPE19 cells were transfected with the GFP-FIGL-1-expressing plasmid for 24 h and then immunostained for acetylated tubulin (Ac-tub, red) and DNA (blue). For staining in (A) and (B), cells were fixed with cold methanol for only 3 min so that both cytoplasmic microtubules and centrosomes could be stained. Scale bar: 10 μm. (B) ARPE19 cells were transfected with the GFP-FIGL-1-Nter-expressing plasmid for 24 h and then immunostained for acetylated tubulin (Ac-tub, red) and DNA (blue). Scale bar: 10 μm. (C) HEK293T cells were transfected with the GFP-FIGL-1-Nter-expressing plasmid for 24 h, serum-starved for 24 h to induce the formation of primary cilia, and then immunostained for acetylated tubulin (Ac-tub, red) and DNA (blue). Scale bar: 7.5 μm. (D) Percentages of ciliated HEK293T cells overexpressing GFP, GFP-FIGL-1, or GFP-FIGL-1-Nter after serum starvation for 24 h. (E) The relative amount of endogenous FIGL-1 protein of ARPE19 cells at different stages was detected by protein gel blotting. G0 stage cells were obtained by serum starvation for 24 h. Cells were synchronized in G1, S, G2, and M phase cells as described in the Methods. Control cells were not treated. The normalized relative amount of FIGL-1 protein is given below the gel.
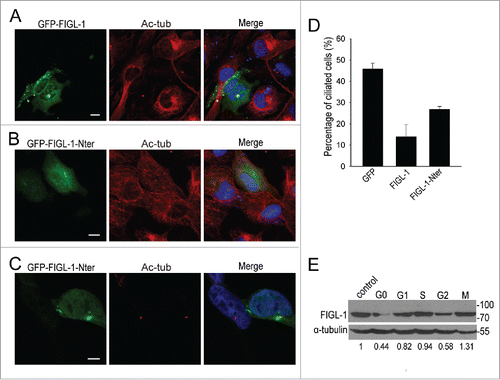
The protein level of FIGL-1 is regulated along with the cell cycle
Given that endogenous FIGL-1 localized to the centrosome and functioned in ciliogenesis, both of which are tightly controlled during the cell cycle, we hypothesized that the endogenous FIGL-1 protein level should be regulated in a cell cycle-dependent manner. By examining cells synchronized at different stages, we found that the amount of endogenous FIGL-1 varied during the duplicating cell cycle, with the amount of FIGL-1 protein lowest in G2 phase (). In addition, when cells were serum-starved to exit the cell cycle and enter G0 phase, the protein level of FIGL-1 significantly decreased. Given that ciliogenesis occurs in G0 phase, this change seems to be consistent with the notion that the FIGL-1 protein inhibits ciliogenesis.
Overexpression of FIGL-1 affects cilia length in zebrafish
To further explore the physiological role of FIGL-1, we overexpressed FIGL-1 in zebrafish embryos by injecting GFP-FIGL-1 mRNA, and checked cilia length in Kupffer's Vesicle (KV). In agreement of ciliogenesis-inhibiting effect observed in cultured cells, the average cilium length in KV at 8-somite stage of GFP-FIGL-1-overexpressing embryos was significantly reduced than that of control embryos (). As cilia-driven flow makes the earliest break for left-right symmetry,Citation18 we next checked heart laterality at 30 hpf (hours post fertilization) in zebrafish. Indeed, while heart was positioned to left in all the GFP-overexpressing control embryos, heart was positioned to middle or right in a significant percentage of GFP-FIGL-1-overexpressing embryos (). Furthermore, we analyzed the expression pattern of southpaw, a Nodal homolog, which is normally expressed in the left lateral plate in embryos at the 20-somite stage.Citation19 In a significant percentage of GFP-FIGL-1-overexpressing embryos, southpaw was bilaterally or right-sided expressed, in comparison with the control group (). Together, these results in zebrafish demonstrate that overexpression of FIGL-1 also interferes with ciliogenesis, as well as a cilia-related developmental event, in a physiological circumstance.
Figure 5. The effects of FIGL-1 overexpression in zebrafish embryos. (A) Representative fluorescent images of Kupffer's Vesicle (KV) from GFP- or GFP-FIGL-1-overexpressing embryos, stained with an antibody against acetylated tubulin (Ac-tub, red). Scale Bar: 10 μm. Bar graph shows the average cilium length in GFP- or GFP-FIGL-1-overexpressing embryos. ‘n’ indicates the number of embryos pooled from 2 independent experiments. (B) Representative bright-field images showing the heart position in zebrafish embryos at 30 hpf. The embryos are shown in ventral view, showing typical left-, middle- or right-positioned heart as indicated. Bar graph shows the distribution of heart position in GFP- or GFP-FIGL-1-overexpressing embryos. ‘n’ indicates the number of embryos pooled from 3 independent experiments. (C) Representative bright-field images showing the expression pattern of southpaw in the left, bilateral or right lateral plate as indicated. Embryos are shown in dorsal view. Bar graph shows the distribution of southpaw in GFP- or GFP-FIGL-1-overexpressing embryos. ‘n’ indicates the number of embryos pooled from 3 independent experiments. In this figure, *: 0.01≤p<0. 05, **: 0.005≤p<0.01, ***: p<0.005.
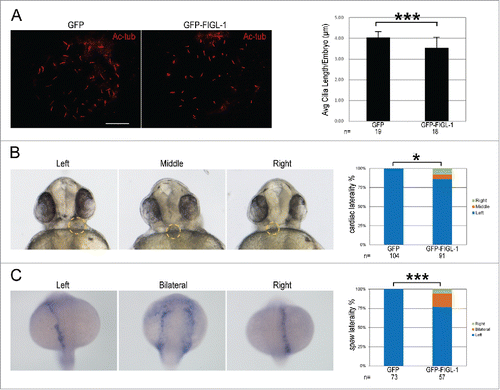
Discussion
Although the primary cilium was first described more than 300 y ago,Citation20 its physiological importance has emerged only in the recent 2 decades. Primary cilia are the organelles that sense the extracellular environment and also serve as the compartments for many important signaling events, including the hedgehog and Wnt signaling pathways.Citation21 As a consequence, an abnormality in ciliogenesis is the cause of various developmental defects and diseases.Citation6 Modern imaging techniques and proteomics techniques have allowed the identification of the detailed building structure of cilia and the existence of many structural (e.g., tubulin and SAS-6) and signaling (e.g., plk4) proteins in the cilia.Citation10,22-24 However, the regulation of ciliogenesis is still not fully understood.
Given that cilia are microtubule-based protrusions from the mother centriole attached beneath the cell surface,Citation10 it is not surprising that different microtubule-regulating proteins participate in ciliogenesis. Among the 5 essential components for centriole assembly identified in C. elegans, the SAS-4-related protein CPAP (also known as CENPJ) has a tubulin-sequestering motif and a microtubule elongation-promoting effect, although the full function of this protein remains elusive.Citation22,25,26 Recently, 2 microtubule-depolymerizing proteins, Kif24 and Kif2A, were found to regulate ciliogenesis by remodeling microtubules.Citation27,28 In this study, we established that FIGL-1 is a new centrosome protein. This protein preferentially localizes to the mother centriole and has an inhibitory effect on ciliogenesis.
FIGL-1 is an AAA ATPase protein. Because the AAA domain in the C-terminal half of FIGL-1 is conserved among all members of the AAA superfamily, which have various cellular functions,Citation2 it is the N-terminal part of FIGL-1 that drives this protein to the centrosome (Fig. S4). Through an unknown mechanism, FIGL-1 localized preferentially to the distal part of the mother centriole (), where it prevented the assembly of cilia probably by severing microtubules (). Therefore, when primary cilia were formed in G0 phase, the protein level of FIGL-1 accordingly decreased (). Given that FIGL-1 has a ubiquitination site,Citation29 it is likely that FIGL-1 level is regulated via the ubiquitination-proteasome pathway, which regulates the levels of some other centrosome proteins, such as CP110.Citation30,31 We also noticed that the FIGL-1 level was lower in G2 phase than in other phases (). This is probably related to the fact that daughter centrioles grow in G2 phase.Citation32 Taken together, as a microtubule-severing protein, the level of FIGL-1 is negatively correlated with the assembly of cilia/centrioles.
It is worth noting that FIGL-1 is not the first microtubule-severing protein identified in the centrosome. Katanin also localizes to the centrosomeCitation33 and regulates the biogenesis of the centrosome and motile cilia.Citation34,35 Fidgetin localizes to the centrosome, too.Citation8 Super-resolution SIM imaging revealed that endogenous fidgetin, similar to FIGL-1 (), was mainly enriched around the mother centriole (Fig. S6A). We checked that overexpressed fidgetin protein has similar inhibitory effect on ciliogenesis (Fig. S6B and S6C). It is therefore likely that fidgetin is also involved in the regulation of ciliogenesis, which might perfectly explain the ciliopathy-like phenotypes of fidget mice.Citation4,5 The presence of these microtubule-severing proteins in the centrosome suggests that microtubule severing is a necessary regulatory mechanism for centrosome duplication and ciliogenesis. However, these proteins were not identified in proteomic studies of the centrosome,Citation23,29 highlighting the importance of studying proteins individually.
Intriguingly, FIGL-1 was found to participate in DNA repair in the nucleus, a function that is totally unrelated with microtubule severing and ciliogenesis.Citation36,37 Nevertheless, we also observed the nuclear localization of endogenous FIGL-1 protein in interphase and its spindle equator localization in mitosis (), supportive of a DNA-related function. Further studies are required to better understand how FIGL-1 plays these 2 different functions in cells.
Materials and methods
Cell culture and plasmids
HEK293T and NIH-3T3 cells (ATCC) were grown in DMEM (Hyclone, SH30022.01B) supplemented with 10% fetal bovine serum (FBS; Gibco, 10099–141). ARPE19 cells (a generous gift from Dr. Guotong Xu, Tongji University) were grown in F12 medium (Hyclone, SH30026.01B) supplemented with 10% FBS. The formation of primary cilia was induced by serum starvation for 24 h.
Overexpression plasmids of GFP-tagged fidgetin and FIGL-1 were constructed with the pEGFP-C1 vector (Clontech, 6084-1). GFP-expressing shRNA plasmids of control shRNA and FIGL-1-targeted shRNAs were ordered from Genechem (Shanghai) Ltd. The sequences of shRNAs against FIGL-1 were as follows: 1#, 5′-CTGTAATACTGCTCTCTTT-3′ and 2#, 5′-GGAGAGCAATCGTTTGAAA-3′. All of these plasmids were transfected with Gene Expression Max Transfection Reagent (Excellgen, EG-1086).
Zebrafish husbandry and experiments
Wild-type zebrafish of Tubingen were maintained according to standard protocol.Citation38 Embryos were collected from natural mating and kept at 28.5°C in E3 solution. Zebrafish care and experiments were approved by the Institutional Animal Care and Use Committee of Tongji University.
Antibodies
The commercial antibodies used were rabbit polyclonal anti-pericentrin (Covance, PRB-432C), mouse monoclonal anti-acetylated tubulin (Sigma, T7451), rabbit polyclonal anti-CP110 (Proteintech, 12780-1-AP), and mouse monoclonal anti-centrin (Merck Millipore, 04-1624-20H5). Alexa Fluor 488- and Alexa Fluor 568-conjugated secondary antibodies were from Life Technologies. A polyclonal antibody against human FIGL-1 was generated in rabbits against a specific peptide sequence (SLPNSAHDRDRTQDFPE). A rabbit polyclonal anti-fidgetin antibody was prepared as described.Citation8
Western blotting
Cells were lysed in lysis buffer (8.85% SDS, 50 mM Tris-HCl pH 7.4, 1 mM PMSF, and 50 mM DTT) containing a protease inhibitor cocktail (Roche, 4693159001), electrophoresed on 10% SDS polyacrylamide gels, and then transferred to Immobilon-P PVDF transfer membrane (Merck Millipore, IPVH00010). The membranes were blocked in TBST (0.5% Tween-20 prepared in 1× TBS buffer pH 7.4) containing 5% nonfat milk powder for 3 h and incubated with primary antibodies diluted 1:1000 in TBST for 3 h. Bound primary antibodies were detected using secondary antibodies conjugated to horseradish peroxidase and chemiluminescence reagents (Pierce, 34080).
Immunofluorescence microscopy
HEK293T and ARPE19 cells were cultured on coverslips. For staining of the centrosome with antibodies against centrosome proteins, cells were fixed with cold methanol for 20 min. For staining of the cytoplasmic microtubule network and the centrosome simultaneously with an anti-acetylated tubulin antibody, cells were fixed with cold methanol for 3 min. For staining of primary cilia with an anti-acetylated tubulin antibody, cells were fixed with 4% paraformaldehyde for 30 min.
Thereafter, slides were permeabilized with 0.2% Triton X-100 prepared in phosphate-buffered saline (PBS) for 10 min and blocked with 1% bovine serum albumin prepared in PBS for 1 h. Samples were incubated with primary and secondary antibodies at room temperature for 2 h and 1 h, respectively. DNA was stained with 4,6-diamidino-2-phenylindole (DAPI) for 10 min at room temperature. Confocal microscopy images were taken on a Leica TCS SP5 system with a ×63/1.40–0.60 NA oil objective. 3D-SIM images were obtained on a NIKON NSIM system equipped with a ×100/1.50 NA oil-immersion objective (Nikon) and 3 laser beams (405, 488, and 561 nm).
Zebrafish embryos were fixed in Dent's fixative (80% methanol: 20% dimethyl sulfoxide) at −20°C overnight or in 4% formalin overnight at 4°C. Before antibody staining, the fixed embryos were rehydrated, washed with 0.5% Tween-20 prepared in PBS (PBST), and blocked with 10% goat serum (GS) in PBST. Images were taken with a Nikon eclipse 80i fluorescent microscope and cilia length were analyzed with NIS-elements software (Nikon).
Electron microscopy
HEK293T cells were digested, centrifuged, and fixed with 2.5% glutaraldehyde prepared in 0.1 M sodium phosphate buffer (pH 7.4) for 1.5 h at 4°C. After washing with 0.1 M sodium phosphate buffer (pH 7.4), samples were postfixed with 2% OsO4 prepared in the same buffer for 1.5 h. After washing with 0.1 M sodium phosphate buffer (pH 7.4), samples were dehydrated with ethanol and acetone, and embedded in Epon 812 agent. Embedded blocks were sliced at a thickness of 70 nm by ultra-thin microtome sectioning, doubly stained with uranyl acetate and Reynolds's lead citrate, and viewed with a transmission electron microscope (FEI Tecnai G2 Spirit).
Recombinant protein expression and microtubule interaction analysis
The coding region of full-length human FIGL-1 (residues 1–674) or FIGL-1-Δ183 (residues 184–674) was cloned into pGEX-6p-1 vector through BamH I and EcoRI restriction sites, and 2 proteins with different length were expressed in E. coli BL21 StarTM strain (Life Technologies), and purified with GST affinity column, as previously described for recombinant spastin protein.Citation39 The full-length FIGL-1 protein was not soluble, so only FIGL-1-Δ183 was purified and used for microtubule-interacting analysis.
Microtubules were prepared as previously described; the severing of fluorescence-labeled microtubules was observed as in the study of spastin.Citation39 To check the direct binding between FIGL-1-Δ183 and microtubules, 1 μM Taxotere-stabilized microtubules were incubated with 1 μM GST-FIGL-1-Δ183 fusion protein in various nucleotide conditions for 15 min, and then ultracentrifuged at 80,000 rpm (Beckman TLA100.3 rotor) for 15 min at 25°C. Equal fraction of supernatant and pellet were analyzed by SDS-PAGE.
Cell cycle synchronization and FACS analysis
APRE19 cells were synchronized by drug treatment. G1, S, and G2 phase cells were obtained by treating the cells with 0.4 mM mimosine for 14 h, 2 mM thymidine for 24 h, and 9 μM RO-3306 for 19 h, respectively. M phase cells were obtained by treating the cells with 80 ng/ml nocodazole for 14 h and then culturing in normal cell culture medium for 2 h. The cell cycle distribution of HEK293T cells transfected with shRNA plasmids for 72 h was analyzed by flow cytometry after they were fixed with cold 70% ethanol for 2 h and stained with 50 μg/ml propidium iodide for 30 min.
mRNA injection
GFP-FIGL-1-encoding fragment was PCR amplified and subcloned into pCS2 vector. mRNA was transcribed in vitro with the mMESSAGE mMACHINE SP6 kit (Ambion) using linearized DNA construct as template.
In situ hybridization
Whole-mount in situ hybridization was performed as previously described.Citation40 Full-length of southpaw was PCR amplified from a homemade zebrafish cDNA library and cloned into T-easy vector. Antisense probes for southpaw was made with digoxigenin-labeled UTPs (Roche) using linearized DNA construct as template for RNA synthesis. The stained embryos were photographed with a Nikon SMZ1500 stereomicroscope.
Disclosure of potential conflicts of interest
No potential conflicts of interest were disclosed.
1204059_Supplemental_Material.docx
Download MS Word (16.4 MB)Acknowledgments
The authors are very grateful to Dr. Guotong Xu (Tongji University) for kindly providing the ARPE19 cells and Dr. Zhiyong Mao (Tongji University) for sharing reagents. The authors are grateful to the Confocal and FACS Facility of Tongji University for their technical help. The authors would like to thank the National Center for Protein Science Shanghai for the 3D-SIM imaging.
Funding
This work was supported by grants from National Science Foundation of China (Grants no. 31370771 and 31171412) and a Specialized Research Fund for the Doctoral Program of Ministry of Education of China (SRFDP grant 20130072110031).
References
- Hanson PI, Whiteheart SW. AAA+ proteins: have engine, will work. Nat Rev Mol Cell Biol 2005; 6:519-29; PMID:16072036; http://dx.doi.org/10.1038/nrm1684
- Snider J, Houry WA. AAA+ proteins: diversity in function, similarity in structure. Biochem Soc Trans 2008; 36:72-7; PMID:18208389; http://dx.doi.org/10.1042/BST0360072
- Cox GA, Mahaffey CL, Nystuen A, Letts VA, Frankel WN. The mouse fidgetin gene defines a new role for AAA family proteins in mammalian development. Nature genetics 2000; 26:198-202; PMID:11017077; http://dx.doi.org/10.1038/79923
- Truslove MG. The anatomy and development of the fidget mouse. J Genet 1956; 54:64-8; http://dx.doi.org/10.1007/BF02981704
- Gruneberg H. Two new mutant genes in the house mouse. J Genet 1943; 45:22-8; http://dx.doi.org/10.1007/BF02982771
- Waters AM, Beales PL. Ciliopathies: an expanding disease spectrum. Pediatr Nephrol 2011; 26:1039-56; PMID:21210154; http://dx.doi.org/10.1007/s00467-010-1731-7
- Frickey T, Lupas AN. Phylogenetic analysis of AAA proteins. J Struct Biol 2004; 146:2-10; PMID:15037233; http://dx.doi.org/10.1016/j.jsb.2003.11.020
- Mukherjee S, Diaz Valencia JD, Stewman S, Metz J, Monnier S, Rath U, Asenjo AB, Charafeddine RA, Sosa HJ, Ross JL, et al. Human Fidgetin is a microtubule severing the enzyme and minus-end depolymerase that regulates mitosis. Cell Cycle 2012; 11:2359-66; PMID:22672901; http://dx.doi.org/10.4161/cc.20849
- Leo L, Yu W, D'Rozario M, Waddell EA, Marenda DR, Baird MA, Davidson MW, Zhou B, Wu B, Baker L, et al. Vertebrate Fidgetin Restrains Axonal Growth by Severing Labile Domains of Microtubules. Cell Rep 2015; 12:1723-30; PMID:26344772; http://dx.doi.org/10.1016/j.celrep.2015.08.017
- Reiter JF, Blacque OE, Leroux MR. The base of the cilium: roles for transition fibres and the transition zone in ciliary formation, maintenance and compartmentalization. EMBO Rep 2012; 13:608-18; PMID:22653444; http://dx.doi.org/10.1038/embor.2012.73
- Luke-Glaser S, Pintard L, Tyers M, Peter M. The AAA-ATPase FIGL-1 controls mitotic progression, and its levels are regulated by the CUL-3MEL-26 E3 ligase in the C. elegans germ line. J Cell Sci 2007; 120:3179-87; PMID:17878235; http://dx.doi.org/10.1242/jcs.015883
- Onitake A, Yamanaka K, Esaki M, Ogura T. Caenorhabditis elegans fidgetin homolog FIGL-1, a nuclear-localized AAA ATPase, binds to SUMO. J Struct Biol 2012; 179:143-51; PMID:22575764; http://dx.doi.org/10.1016/j.jsb.2012.04.022
- Yang Y, Mahaffey CL, Berube N, Nystuen A, Frankel WN. Functional characterization of fidgetin, an AAA-family protein mutated in fidget mice. Exp Cell Res 2005; 304:50-8; PMID:15707573; http://dx.doi.org/10.1016/j.yexcr.2004.11.014
- L'Hote D, Vatin M, Auer J, Castille J, Passet B, Montagutelli X, Serres C, Vaiman D. Fidgetin-like1 is a strong candidate for a dynamic impairment of male meiosis leading to reduced testis weight in mice. PLoS One 2011; 6:e27582; PMID:22110678; http://dx.doi.org/10.1371/journal.pone.0027582
- Peng W, Lin Z, Li W, Lu J, Shen Y, Wang C. Structural insights into the unusually strong ATPase activity of the AAA domain of the Caenorhabditis elegans fidgetin-like 1 (FIGL-1) protein. J Biol Chem 2013; 288:29305-12; PMID:23979136; http://dx.doi.org/10.1074/jbc.M113.502559
- Mennella V, Agard DA, Huang B, Pelletier L. Amorphous no more: subdiffraction view of the pericentriolar material architecture. Trends Cell Biol 2014; 24:188-97; PMID:24268653; http://dx.doi.org/10.1016/j.tcb.2013.10.001
- Sonnen KF, Schermelleh L, Leonhardt H, Nigg EA. 3D-structured illumination microscopy provides novel insight into architecture of human centrosomes. Biol Open 2012; 1:965-76; PMID:23213374; http://dx.doi.org/10.1242/bio.20122337
- Hirokawa N, Tanaka Y, Okada Y, Takeda S. Nodal flow and the generation of left-right asymmetry. Cell 2006; 125:33-45; PMID:16615888; http://dx.doi.org/10.1016/j.cell.2006.03.002
- Long S, Ahmad N, Rebagliati M. The zebrafish nodal-related gene southpaw is required for visceral and diencephalic left-right asymmetry. Development 2003; 130:2303-16; PMID:12702646; http://dx.doi.org/10.1242/dev.00436
- Satir P. Landmarks in cilia research from Leeuwenhoek to us. Cell Motil Cytoskeleton 1995; 32:90-4; PMID:8681400; http://dx.doi.org/10.1002/cm.970320203
- Goetz SC, Anderson KV. The primary cilium: a signalling centre during vertebrate development. Nat Rev Genet 2010; 11:331-44; PMID:20395968; http://dx.doi.org/10.1038/nrg2774
- Gonczy P. Towards a molecular architecture of centriole assembly. Nat Rev Mol Cell Biol 2012; 13:425-35; PMID:22691849; http://dx.doi.org/10.1038/nrm3373
- Andersen JS, Wilkinson CJ, Mayor T, Mortensen P, Nigg EA, Mann M. Proteomic characterization of the human centrosome by protein correlation profiling. Nature 2003; 426:570-4; PMID:14654843; http://dx.doi.org/10.1038/nature02166
- Jakobsen L, Vanselow K, Skogs M, Toyoda Y, Lundberg E, Poser I, Falkenby LG, Bennetzen M, Westendorf J, Nigg EA, et al. Novel asymmetrically localizing components of human centrosomes identified by complementary proteomics methods. EMBO J 2011; 30:1520-35; PMID:21399614; http://dx.doi.org/10.1038/emboj.2011.63
- Kirkham M, Muller-Reichert T, Oegema K, Grill S, Hyman AA. SAS-4 is a C. elegans centriolar protein that controls centrosome size. Cell 2003; 112:575-87; PMID:12600319; http://dx.doi.org/10.1016/S0092-8674(03)00117-X
- Cormier A, Clement MJ, Knossow M, Lachkar S, Savarin P, Toma F, Sobel A, Gigant B, Curmi PA. The PN2-3 domain of centrosomal P4.1-associated protein implements a novel mechanism for tubulin sequestration. J Biol Chem 2009; 284:6909-17; PMID:19131341; http://dx.doi.org/10.1074/jbc.M808249200
- Kobayashi T, Tsang WY, Li J, Lane W, Dynlacht BD. Centriolar kinesin Kif24 interacts with CP110 to remodel microtubules and regulate ciliogenesis. Cell 2011; 145:914-25; PMID:21620453; http://dx.doi.org/10.1016/j.cell.2011.04.028
- Miyamoto T, Hosoba K, Ochiai H, Royba E, Izumi H, Sakuma T, Yamamoto T, Dynlacht BD, Matsuura S. The Microtubule-Depolymerizing Activity of a Mitotic Kinesin Protein KIF2A Drives Primary Cilia Disassembly Coupled with Cell Proliferation. Cell Rep 2015; 10:664-673.
- Wagner SA, Beli P, Weinert BT, Nielsen ML, Cox J, Mann M, Choudhary C. A proteome-wide, quantitative survey of in vivo ubiquitylation sites reveals widespread regulatory roles. Mol Cell Proteomics 2011; 10:M111 013284; http://dx.doi.org/10.1074/mcp.M111.013284
- Li J, D'Angiolella V, Seeley ES, Kim S, Kobayashi T, Fu W, Campos EI, Pagano M, Dynlacht BD. USP33 regulates centrosome biogenesis via deubiquitination of the centriolar protein CP110. Nature 2013; 495:255-9; PMID:23486064; http://dx.doi.org/10.1038/nature11941
- D'Angiolella V, Donato V, Vijayakumar S, Saraf A, Florens L, Washburn MP, Dynlacht B, Pagano M. SCF(Cyclin F) controls centrosome homeostasis and mitotic fidelity through CP110 degradation. Nature 2010; 466:138-42; PMID:20596027; http://dx.doi.org/10.1038/nature09140
- Nigg EA, Stearns T. The centrosome cycle: Centriole biogenesis, duplication and inherent asymmetries. Nat Cell Biol 2011; 13:1154-60; PMID:21968988; http://dx.doi.org/10.1038/ncb2345
- McNally FJ, Okawa K, Iwamatsu A, Vale RD. Katanin, the microtubule-severing ATPase, is concentrated at centrosomes. J Cell Sci 1996; 109 ( Pt 3):561-7; PMID:8907702
- Hu WF, Pomp O, Ben-Omran T, Kodani A, Henke K, Mochida GH, Yu TW, Woodworth MB, Bonnard C, Raj GS, et al. Katanin p80 regulates human cortical development by limiting centriole and cilia number. Neuron 2014; 84:1240-57; PMID:25521379; http://dx.doi.org/10.1016/j.neuron.2014.12.017
- Sharma N, Bryant J, Wloga D, Donaldson R, Davis RC, Jerka-Dziadosz M, Gaertig J. Katanin regulates dynamics of microtubules and biogenesis of motile cilia. J Cell Biol 2007; 178:1065-79; PMID:17846175; http://dx.doi.org/10.1083/jcb.200704021
- Yuan J, Chen J. FIGNL1-containing protein complex is required for efficient homologous recombination repair. Proc Natl Acad Sci U S A 2013; 110:10640-5; PMID:23754376; http://dx.doi.org/10.1073/pnas.1220662110
- Girard C, Chelysheva L, Choinard S, Froger N, Macaisne N, Lemhemdi A, Mazel J, Crismani W, Mercier R. AAA-ATPase FIDGETIN-LIKE 1 and Helicase FANCM Antagonize Meiotic Crossovers by Distinct Mechanisms. PLoS Genet 2015; 11:e1005369; PMID:26161528; http://dx.doi.org/10.1371/journal.pgen.1005369
- Westerfield M. The zebrafish book. A guide for the laboratory use of zebrafish (Danio rerio). 4th ed. Eugene: Univ. of Oregon Press, 2000.
- Wen M, Wang C. The nucleotide cycle of spastin correlates with its microtubule-binding properties. FEBS J 2013; 280:3868-77; PMID:23745751; http://dx.doi.org/10.1111/febs.12385
- Thisse B, Heyer V, Lux A, Alunni V, Degrave A, Seiliez I, Kirchner J, Parkhill JP, Thisse C. Spatial and temporal expression of the zebrafish genome by large-scale in situ hybridization screening. Method Cell Biol 2004; 77:505-519