To take advantage of the different chemical environments made possible by internal membrane bound sub-compartments, Eukaryotic cells heavily utilize active transport. Much of this involves moving distinct cargos along microtubules, perhaps to facilitate cargo-cargo interactions—e.g. to control transfer of lipids from lipid droplets to mitochondria as part of metabolism—or to allow function of extended cells such as neurons. There are 2 major families of microtubule molecular motors: the predominantly plus-end directed kinesin motors, and minus-end directed dynein, and evolutionarily they appear to have taken a different approach to modulating function. There are many kinesin-family members,Citation1 and these appear to have been adapted to specific cellular functions. In contrast, there are typically only one or at most 2 genes for cytoplasmic dynein, and the multiple distinct functions of dynein are altered by functioning with the 2 large cofactor complexes, Dynactin and NudE/Lis1.
Dynactin was originally discovered to increase dynein travel distances,Citation2 and was later reported to also have a domain able to turn off dynein force production,Citation3 suggesting multiple levels of potential regulation that have yet to be explored. The NudE/Lis1 system was identified to play an important role in development and disease, as mutations in the Lis1 protein result in a devastating developmental defect known as Miller-Dieker Syndrome, resulting from impaired neuronal migration due to impaired nuclear migration.Citation4 Later studiesCitation5 in vitro showed that together with NudE, Lis1 modulates dynein force production, allowing the motor to better remain attached to microtubules when under load. By decreasing the motors’ detachment rate when exposed to opposing forces, this in turn allows multiple motors to better add their forces, resulting in improved ensemble function, and thus better ability to move cargos against opposition.
While this in vitro study appeared to potentially explain the in vivo Miller-Dieker lissencephaly phenotype—neuronal migration requires transport of the cells’ nucleus down the axon, a dynein-dependent process likely facing serious opposition, and thus such migration is likely dependent on improved dynein function—a number of key questions remained. First, it was unknown whether cargo transport was relatively constant, and insensitive to local environmental factors, or whether there were in fact some local sensing and response, e.g., to impediments to motion. Second, it remained to be determined whether the NudE/Lis1 system altered dynein the same way in vivo as it did in vitro, given the more complicated in vivo environment. Third, the extent to which the NudE/Lis1 system was important for cargos other than nuclei remained an open question. Finally, whether—and how—the system worked in conjunction with the dynactin complex was unknown.
These questions were answered in a new studyCitation6 entitled “Load-induced enhancement of Dynein force production by LIS1-NudE in vivo and in vitro.” The work started by using an optical trap () to measure force production of a relatively large class of endogenous cargo—the lipid droplet—in cultured mammalian cells. To their surprise, Reddy et al had difficulty making the measurements, because although the moving lipid droplets could initially be captured and stalled, they would eventually escape from the optical trap (). After some further studies, it was discovered that the droplets’ force production was in fact not constant, but rather, increased over time, potentially reflecting adaptation to obstacles.
Figure 1. Cartoon of a lipid droplet in the optical trap. Lower: dark circle: lipid droplet; red parabolic lines: optical trap, exerting a centripetal force (red arrow) on the droplet when displaced from trap center. Horizontal black lines: dynein motors, attached to microtubule (blue). Upper: enlargement of the lower portion of the lipid droplet, showing attached dynein motors in black, with NudE (red) and Lis1 (green) cofactors. Two dyneins on left: pre-adaptation stage, with un-extended NudE (red, short) and Lis1 (green) that is not interacting with the dynein motor domains (ovals). Five dyneins on right: adapted dynein; NudE extended, Lis1 interacting with the dynein motor domains.
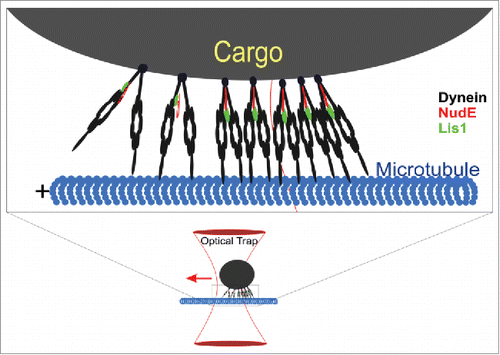
Mechanistically, this adaptation involved increased utilization of the NudE/Lis1 system, and could be blocked by decreasing cellular levels of either the NudE or Lis1 proteins. Importantly, on average it was not ‘all or none’, but rather, reflected gradually increasing utilization of NudE and Lis1: the divergence between function in the wild-type and knockdown backgrounds increased as a function of the number of attempted escapes from the optical trap.
One appealing model for the increasing contribution of the NudE/Lis1 system to the droplet’s force production would be to have load-induced recruitment of these factors to the droplets. However, that was found not to be required. Instead, all factors necessary for adaptation were already present on the cargo, and adaptation could be recapitulated in vitro, in buffer where no cytosolic factors were present to be recruited. Thus, the study discovered a new cargo-level adaptation, demonstrating that at least lipid droplets have the ability to sense when they are stalled, and in response, up-regulate ensemble force production to overcome the obstacle.
This study leaves intriguing questions unanswered. First, since the NudE/Lis1 system is broadly present upon cargos, and its importance has directly been confirmed for organelle traffic,Citation7 it seems likely that such adaptation occurs for other cargos—perhaps to avoid traffic jams—but this remains to be explored. Second, how does the system sense load, and then respond by increasing NudE/Lis1 utilization? Third, the lipid droplet system appears to use both Dynactin and NudE/Lis1 simultaneously, with the dynamic increase in NudE utilization coming as needed. Why is there adaption, rather than always utilizing NudE/Lis1—is there a cost to excessive NudE/Lis1 utilization? Now that we know that it exists, it will be exciting to determine the importance of cargo-level regulation, as opposed to more global cell-level regulation achieved by tuning signaling pathways.
Disclosure of potential conflicts of interest
No potential conflicts of interest were disclosed.
References
- Hirokawa N, Noda Y, Tanaka Y, Niwa S. Kinesin superfamily motor proteins and intracellular transport. Nat Rev Mol Cell Biol 2009; 10:682-96; PMID:19773780; http://dx.doi.org/10.1038/nrm2774
- King SJ, Schroer TA. Dynactin increases the processivity of the cytoplasmic dynein motor. Nat Cell Biol 2000; 2:20-4; PMID:10620802; http://dx.doi.org/10.1038/71338
- Tripathy SK, Weil SJ, Chen C, Anand P, Vallee RB, Gross SP. Autoregulatory mechanism for dynactin control of processive and diffusive dynein transport. Nat Cell Biol 2014; 16:1192-201; PMID:25419851; http://dx.doi.org/10.1038/ncb3063
- Reiner O, Carrozzo R, Shen Y, Wehnert M, Faustinella F, Dobyns WB, Caskey CT, Ledbetter DH. Isolation of a Miller–Dicker lissencephaly gene containing G protein β-subunit-like repeats. Nature 1993; 364:717-21; PMID:8355785; http://dx.doi.org/10.1038/364717a0
- McKenney RJ, Vershinin M, Kunwar A, Vallee RB, Gross SP. LIS1 and NudE Induce a Persistent Dynein Force-Producing State. Cell 2010; 141:304-14; PMID:20403325; http://dx.doi.org/10.1016/j.cell.2010.02.035
- Reddy BJN, Mattson M, Wynne CL, Vadpey O, Durra A, Chapman D, Vallee RB, Gross SP. Load-induced enhancement of Dynein force production by LIS1–NudE in vivo and in vitro. Nat Commun 2016; 7:12259; PMID:27489054; http://dx.doi.org/10.1038/ncomms12259
- Lam C, Vergnolle MAS, Thrope L, Woodman PG, Allan VJ. Functional interplay between LIS1, NDE1 and NDEL1 in dynein-dependent organelle positioning. J Cell Sci 2010; 123:202-12; PMID:20048338; http://dx.doi.org/10.1242/jcs.059337