ABSTRACT
Polo-like kinase 1 (Plk1) is an important mitotic kinase that is crucial for entry into mitosis after recovery from DNA damage-induced cell cycle arrest. Plk1 activation is promoted by the conserved protein Bora (SPAT-1 in C. elegans), which stimulates the phosphorylation of a conserved residue in the activation loop by the Aurora A kinase. In a recent article published in Cell Reports, we show that the master mitotic kinase Cdk1 contributes to Plk1 activation through SPAT-1/Bora phosphorylation. We identified 3 conserved Sp/Tp residues that are located in the N-terminal, most conserved part, of SPAT-1/Bora. Phosphorylation of these sites by Cdk1 is essential for Plk1 function in mitotic entry in C. elegans embryos and during DNA damage checkpoint recovery in mammalian cells. Here, using an untargeted Förster Resonance Energy Transfer (FRET) biosensor to monitor Plk1 activation, we provide additional experimental evidence supporting the importance of these phosphorylation sites for Plk1 activation and subsequent mitotic entry after DNA damage. We also briefly discuss the mechanism of Plk1 activation and the potential role of Bora phosphorylation by Cdk1 in this process. As Plk1 is overexpressed in cancer cells and this correlates with poor prognosis, understanding how Bora contributes to Plk1 activation is paramount for the development of innovative therapeutical approaches.
KEYWORDS:
Plk1 function and regulation
The serine threonine kinase Plk1, originally discovered in Drosophila melanogaster almost 30 y ago,Citation1 is an important regulator of mitotic entry and progression during a normal cell cycle and after cell cycle arrest induced by DNA damage.Citation2,3
Mitotic entry is controlled through the activation of the Cyclin-dependent kinase Cdk1-Cyclin B, whose activity is regulated by phosphorylation. Wee1 and Myt1 kinases phosphorylate Cdk1 to inactivate it,Citation4,5 whereas Cdc25 phosphatases dephosphorylate these sites to activate it.Citation6,7 Plk1 is part of the feedback loop that activates Cdk1 by promoting Cdc25 activationCitation8 and targeting Wee1 and Myt1 for ubiquitin-mediated degradation.Citation9,10 In addition to its role in Cdk1 activation, Plk1 controls centrioles and centrosome biogenesis, chromosome condensation, spindle assembly and cytokinesis.Citation2 Plk1 plays also a prominent role in checkpoint recovery from DNA damage where it inactivates the checkpoint, promotes DNA repair and mitotic entry.Citation11,12
Plk1 is overexpressed in a wide range of human tumors where its high levels correlate with aggressiveness and poor prognosis.Citation13 How Plk1 contributes to tumorigenesis is currently unclear but it might force cells with DNA damage to enter into mitosis and to escape the G2-M arrest induced by the DNA damage checkpoint.Citation11,14,15 Whereas Plk1 function has been extensively studied, its activation mechanism is less well understood.
Plk1 is composed of 2 structural elements, a N-terminal serine-threonine kinase domain and a C-terminal PBD that contains 2 polo-boxes PB1 and PB2. The PBD is a phospho-binding domain that binds phosphorylated substrates.Citation16 Cdk1 generally achieves priming phosphorylation of the substrate and thereby regulates the subcellular localization of Plk1 by targeting it to its substrates.
Plk1 kinase activation depends on phosphorylation of a conserved residue (T210) in the activation segment (T-loop)Citation17 by the Aurora A kinase.Citation18,19 This process requires the conserved protein Bora, originally discovered in Drosophila,Citation20 which stimulates Plk1 phosphorylation by Aurora A.Citation18,19
Bora is an intrinsically disordered protein subjected to post-translational modifications, particularly phosphorylation by several protein kinases including Cdk1,Citation20,21 Aurora A,Citation20 Gsk3β,Citation22 Ataxia telangiectasia and Rad3 relatedCitation23 and Plk1 itself.Citation21,24 Cdk1 phosphorylates Bora on multiple sites distributed along the entire protein, some of which have specific well-characterized functions. Phosphorylation of Serine-252 by Cdk1 primes Bora interaction with the Plk1 PBD. This interaction triggers Plk1-dependent Bora phosphorylation on a degron for the Skp1-Cullin-F box (SCF) β−TrCP E3-Ligase (496-DSGYNT-501), which then targets Bora for proteasomal degradation.Citation21,24 By contrast, phosphorylation of Threonine-52 by Cdk1 prevents Bora degradation in mitosis,Citation25 which might be required to maintain sufficient Bora levels to sustain Plk1 activity during mitosis.Citation26 Cdk1 thus can control the time and the levels of Bora degradation by phosphorylating different residues on Bora. In addition to this role, our results indicate that Cdk1 phosphorylates Bora on yet other residues to promote Plk1 activation by Aurora A.Citation27,28 Here we present additional data, obtained using a Plk1 FRET probe, supporting our finding that phosphorylation of Bora is crucial for Plk1 activation and we discuss the role of Cdk1 in this process.
Multisite phosphorylation of Bora NT by Cdk1 is required for Plk1 activation
In vitro, phosphorylation of Bora by Cdk1 stimulates Plk1 phosphorylation on its activation loop by the Aurora A kinase. Identical results were obtained using SPAT-1, the C. elegans homolog of Bora.Citation27 Whereas there are 26 potential Cdk1 phosphorylation sites in Bora, there are only 14 in SPAT-1, making the C. elegans embryo an attractive model to study the functional relevance of Cdk1-dependent SPAT-1 phosphorylation for mitotic entry.
Division of the early embryo is asymmetric and generates 2 blastomeres of different sizes and developmental potentials that enter into mitosis asynchronously.Citation29,30 The anterior AB blastomere enters mitosis 2 min before the posterior P1 blastomere. The length of AB and P1 cell cycles is highly reproducible from embryo to embryo and can easily be measured by DIC microscopy. plk-1 or spat-1 inactivation by RNA interference delays mitotic entry in both blastomeres.Citation31 An RNAi-resistant GFP::SPAT-1 transgene restores normal cell cycle length in SPAT-1 depleted embryos, allowing to investigate the contribution of SPAT-1 phosphorylation sites for mitotic entry. Using this system, we showed that serines and threonines located in the N-terminal half of SPAT-1 and phosphorylated by Cdk1 in vitro are required for its function in mitotic entry, whereas C-terminally located residues are dispensable for SPAT-1-dependent cell cycle control.Citation27
Consistent with these observations, we recently showed that the N-terminal part of Bora (residues 1-225a), when phosphorylated by Cdk1, is sufficient to support Plk1 phosphorylation by Aurora A in vitro. These results are also fully consistent with previous findings showing that the N-terminal domain of Bora interacts with Aurora ACitation20 and prompted us to search for N-terminally located Sp/Tp sites conserved between SPAT-1 and Bora proteins that might play a role in Plk1 activation in human cells.
Multiple protein alignments revealed the presence of 3 conserved Sp/Tp sites in this region, and 2 conserved Cy (or RXL) motifs,Citation28 which are Cyclin docking sites,Citation32,33 consistent with the notion that Cyclin B1/Cdk1 efficiently phosphorylates SPAT-1/Bora. Mutation of the Cy motif strongly reduced Bora phosphorylation by Cyclin B/Cdk1 and abolished Cyclin B-Bora binding in vitro. Concomitantly, Bora-carrying mutations of the 3 conserved phosphorylation sites abrogated Aurora A-dependent Plk1 phosphorylation on the T-loop in vitro.Citation28
In vivo, mutation of these 3 conserved residues affected SPAT-1 function in mitotic entry in the early C. elegans embryo. Likewise, a Bora transgene mutated on the corresponding 3 sites failed to rescue the mitotic entry defect observed in HeLa cells that were depleted for endogenous Bora in a DNA damage checkpoint recovery assay.Citation28 This is in agreement with the deficient Cdk1-dependent Plk1 activation that we observed in vitro with a non-phosphorylatable version of Bora.Citation28
Monitoring Plk1 activation during checkpoint recovery using a FRET probe
To monitor Plk1 activity in our rescue experiments we have measured the phosphorylation on the T-loop of Plk1. Expression of a Bora siRNA-resistant transgene (BoraR) but not a mutated BoraR3A was accompanied by an increase in the phosphorylation of Plk1-T210. Although phosphorylation of T210 is associated with activation of Plk1, it is not a direct readout of Plk1 activity itself and it does not allow monitoring other possible mechanisms of Plk1 activation independent of Plk1-pT210.Citation28
To address this issue and measure Plk1 activity in real time, we developed a HeLa cell line stably expressing an untargeted fluorescence resonance energy transfer (FRET) sensor to track Plk1 activity changes in time. The probe consists of 2 fluorophores, a donor fluorophore (cyan fluorescent protein, CFP) and an acceptor fluorophore (yellow fluorescent protein, YFP), which are joined in the linker region between donor and acceptor by a consensus Plk1 motif shown to be phosphorylated specifically by Plk1 in mitosis.Citation34 Phosphorylation of the probe by Plk1 is expected to induce a conformational change within the sensor that leads to a low resonance energy transfer between YFP and CFP (low FRET; high CFP/YFP ratio),Citation35,36 whereas dephosphorylation results in high-energy transfer (high FRET; low CFP/YFP ratio). Following the experimental set up shown in , the same percentage of interphase cyclin B1 positive cells was seen in all conditions (not shown), indicating that cells were similarly arrested in G2 at the time of caffeine addition and FRET analysis. Depletion of Bora resulted in decreased CFP/YFP ratio compared to control cells, consistent with a decrease of Plk1 activity (). Cells transfected with wild type siRNA-resistant Bora (mCherry-BoraR) displayed similar FRET levels to those seen in control cells (high CFP/YFP ratio). In contrast, in BoraR3A expressing cells, the sensor was dephosphorylated resulting in higher FRET (low CFP/YFP ratio), indicating that Plk1 activity was reduced (). Therefore, we confirm with a different in vivo assay that phosphorylation on the 3 conserved N-terminal sites of Bora is required for Plk1 activity when cells recover from a G2 DNA damage induced arrest.
Figure 1. (A) Scheme of the assay used to test the effect of Cdk1-dependent sites on Bora for Plk1 activation in living cells and for pS10-H3 staining assessment in fixed cells. (B) Histogram showing CFP/YFP emission ratios averaged over multiple cells (n ≥ 15 per condition) expressing an untargeted Plk1 phosphorylation sensor after G2 checkpoint recovery of control or Bora-depleted cells transfected with mCherry-BoraR and mCherry-BoraR3A constructs. N = 4 independent experiments. Mean ± standard deviation is shown. Statistical significance was determined using Student's t test: ns = not significant, *** = p < 0.001. (C) Representative images showing the mCherry channel (top panels) and the false-colored coded CFP/YFP emission ratios (bottom panels). Scale bar, 10 μM. (D) Histogram showing the percentage of pS10-H3 positive cells after G2-checkpoint recovery of control or Bora-depleted cells transfected with Cherry-Bora constructs after 9 hours and 18 hours treated with nocodazole as in (A), N = 3 independent experiments. Statistical significance was determined using Student's t test: ** = p < 0.01 for Bora-depleted cells or mCherry-BoraR3A –rescued cells compared to mCherry-BoraR–rescued cells.
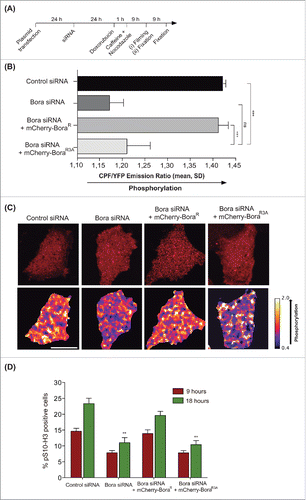
To assess whether non-phosphorylatable Bora was abrogating Plk1 activity leading to impaired entry into mitosis or it was simply delaying mitotic entry, we monitored the percentage of cells in mitosis by means of phosphorylated Histone 3 (pS10-H3) staining in fixed samples and by counting the percentage of mitotic transfected cells (mCherry positive) in our live FRET set up at 9 h and 18 h of nocodazole treatment. We observed accumulation of mitotic cells after 18 h of nocodazole treatment in control cells and BoraR rescued cells, but no significant increment was seen upon Bora depletion or in cells transfected with the BoraR3A version (), indicating that either there is no residual Plk1 activity when Bora is not phosphorylated in the 3 abovementioned Cdk1 sites or that any residual activity is not sufficient to drive mitotic entry.
These experiments further confirm that phosphorylation of Bora on these 3 sites is required in vivo for mitotic entry during DNA damage checkpoint recovery.
Discussion
Our data support a model in which phosphorylation of SPAT-1/Bora by Cdk1 is crucial to activate Plk1. What are the cyclins involved in this process and how does phosphorylation of Bora and SPAT-1 impact on Plk1 activation?
Bora and SPAT-1 contain Cyclin-docking sites whose mutation affects their phosphorylation by Cyclin B/Cdk1 in vitro. Furthermore, Cyclin B was recovered in Bora immunoprecipitates from mitotic cells. Cdk1/Cyclin B might thus act in a feedback loop keeping Plk1 active via Bora phosphorylation to sustain Plk1 activity during mitosis.Citation26 Alternatively, Cdk1/Cyclin A, which is known to trigger Cdk1/Cyclin B activation in G2, might phosphorylate Bora in G2 and thereby contribute to Plk1 activation, which occurs prior CyclinB/Cdk1 activation.Citation18 Although we did not recover Cyclin A in our immunoprecipitations, Cyclin A/Cdk1 was active at the time point where Bora is maximally phosphorylated. Determining when and where Bora becomes phosphorylated on the critical Sp/Tp sites with respect to CyclinA/Cdk1 and CyclinB/Cdk1 kinase activity will be required to address this issue.
Phosphorylation of Bora is crucial for Plk1 activation. How Bora promotes Plk1 phosphorylation by Aurora A, and its subsequent activation is still an open question. It has been proposed that Bora might induce Plk1 activation by promoting a drastic conformational change that relieves the auto-inhibition by the PBD on the kinase domain thereby separating the N and C-terminal regions and exposing the activation loop for phosphorylation by Aurora A.Citation18,19 However, recent cross-linking experiments rather suggest that Plk1 keeps a closed conformation within the Plk1-Aurora A-Bora complex.Citation37 Although these observations strongly suggest that Plk1 does not undergo massive conformational changes separating the N and C-terminal region, they do not exclude the possibility that Bora induces more subtle conformational changes, not detectable by this method. A possibility is that these subtle conformation changes would generate partially active Plk1 states with different degree of catalytic activity.Citation38 Indeed, conformational plasticity and flexibility is crucial for the activation of protein kinases. Bora might thus induce subtle conformational changes of Plk1 exposing the T-loop to Aurora A. Alternatively, Bora could be required to activate Aurora A, as originally proposed in Drosophila.Citation20 However, it has been reported that human Bora does not modify Aurora A activity per se and does not significantly increase Aurora activity toward substrates other than Plk1.Citation18,19 The function of Bora during Plk1 activation could be required for the establishment of a transient but stable Aurora A-Bora-Plk1 complex.
The exact contribution of the 3 conserved Cdk1-dependent phosphorylation sites in Bora-SPAT-1 for Plk1 activation is currently unclear. It is tempting to speculate that these phosphorylated sites have independent functions in modulating the interaction of Bora with Plk1 or with Aurora A. The amino-acid sequence surrounding the 3 residues is different, supporting a model in which all these residues engage a unique set of interactions. Single mutants in these sites have delayed cell cycle timing in the C. elegans embryo, indicating that each site contributes to the phenotype observed in triple mutants.
A better understanding of the role of Bora and SPAT-1 in Plk1 activation and the contribution of Cdk1 phosphorylation to this process will require further biochemical, genetic and structural analysis, a challenging task for the future, as Bora is a highly unstructured protein. Structural analysis of Plk1 from Danio rerio in complex with the PBD-binding motif of Drosophila melanogaster Map205 shed light onto the activation mechanisms of Plk1 and might offer a good framework in the future to understand the role of Bora in Plk1 activation.Citation39
Methods
Förster resonance energy transfer (FRET)
A Hela S3 cell line stably expressing an untargeted Plk1 phosphorylation sensor (plasmid was a kind gift from Michael Lampson, University of Pennsylvania, Philadelphia, US) was generated and the experimental set up shown in was followed. Cells were grown on LabTek II 8-well chamber and were transiently transfected with the indicated mCherry-Bora constructs and depleted of Bora or control siRNA. Live imaging of the Plk1 sensor was performed at the Advanced Light Microscopy Unit at the Center for Genomic Regulation (CRG, Barcelona), on a Leica TCS SP5 II CW-STED microscopy using a 40 x 1.25 NA objective and a charge-coupled device camera (ORCA-AG; Hamamatsu Photonics). CFP was excited with a CFP excitation filter, and CFP and YFP emissions were acquired sequentially by switching between CFP and YFP emission filters using a filter wheel (Ludl Electronic Products). The CFP/YFP emission ratio in each image was calculated after background subtraction and averaged over multiple cells.
Disclosure of potential conflicts of interest
No potential conflicts of interest were disclosed.
Acknowledgments
We thank Mike Lampson (University of Pennsylvania, Philadelphia, US) for providing the FRET construct, Isabelle Vernos, Nuria Mallol and Raquel Garcia (Center for Genomic Regulation, Barcelona) for technical help. We thank Erich A. Nigg for his support.
Funding
AS is supported by the Miguel Servet Program (CP13/00158) from the Instituto Carlos III co-funded by the European Regional Development Fund (ERDF). AP is supported by a VHIR PhD fellowship. YT was supported by a Post-doctoral fellowship from the Foundation for Medical Research. Work in the laboratory of LP is supported by the French National Research Agency under grant no. ANR-2012-BSV2-0001-01 and by the Foundation for Medical Research “Equipe FRM DEQ20140329538.” Work in the laboratory of MG is supported by the University of Geneva, by the Swiss National Science Foundation (Grant number 31003A_156013) and by the NCCR Chemical Biology. Work in the laboratory of AS is supported by the Instituto Carlos III (Grants number PI15/02238 and RTC-2015-3821-1).
References
- Sunkel CE, Glover DM. polo, a mitotic mutant of Drosophila displaying abnormal spindle poles. J Cell Sci 1988; 89:25-38; PMID:3417791
- Zitouni S, Nabais C, Jana SC, Guerrero A, Bettencourt-Dias M. Polo-like kinases: structural variations lead to multiple functions. Nat Rev Mol Cell Biol 2014; 15:433-52; PMID:24954208; http://dx.doi.org/10.1038/nrm3819
- Archambault V, Lépine G, Kachaner D. Understanding the Polo Kinase machine. Oncogene 2015; 34:4799-807; PMID:25619835; http://dx.doi.org/10.1038/onc.2014.451
- Mueller PR, Coleman TR, Kumagai A, Dunphy WG. Myt1: a membrane-associated inhibitory kinase that phosphorylates Cdc2 on both threonine-14 and tyrosine-15. Science 1995; 270:86-90; PMID:7569953; http://dx.doi.org/10.1126/science.270.5233.86
- McGowan CH, Russell P. Human Wee1 kinase inhibits cell division by phosphorylating p34cdc2 exclusively on Tyr15. EMBO J 1993; 12:75-85; PMID:8428596
- Kumagai A, Dunphy WG. The cdc25 protein controls tyrosine dephosphorylation of the cdc2 protein in a cell-free system. Cell 1991; 64:903-14; PMID:1825803; http://dx.doi.org/10.1016/0092-8674(91)90315-P
- Strausfeld U, Labbé JC, Fesquet D, Cavadore JC, Picard A, Sadhu K, Russell P, Dorée M. Dephosphorylation and activation of a p34cdc2/cyclin B complex in vitro by human CDC25 protein. Nature 1991; 351:242-5; PMID:1828290; http://dx.doi.org/10.1038/351242a0
- Kumagai A, Dunphy WG. Purification and molecular cloning of Plx1, a Cdc25-regulatory kinase from Xenopus egg extracts. Science 1996; 273:1377-80; PMID:8703070; http://dx.doi.org/10.1126/science.273.5280.1377
- Watanabe N, Arai H, Nishihara Y, Taniguchi M, Watanabe N, Hunter T, Osada H. M-phase kinases induce phospho-dependent ubiquitination of somatic Wee1 by SCFbeta-TrCP. Proc Natl Acad Sci U S A 2004; 101:4419-24; PMID:15070733; http://dx.doi.org/10.1073/pnas.0307700101
- Inoue D, Sagata N. The Polo-like kinase Plx1 interacts with and inhibits Myt1 after fertilization of Xenopus eggs. EMBO J 2005; 24:1057-67; PMID:15692562; http://dx.doi.org/10.1038/sj.emboj.7600567
- van Vugt MA, Brás A, Medema RH. Polo-like kinase-1 controls recovery from a G2 DNA damage-induced arrest in mammalian cells. Mol Cell 2004; 15:799-811; PMID:15350223; http://dx.doi.org/10.1016/j.molcel.2004.07.015
- Medema RH, Macurek L. Checkpoint recovery in cells: how a molecular understanding can help in the fight against cancer. F1000 Biol Rep 2011; 3:10; PMID:21655336
- Kumar S, Sharma AR, Sharma G, Chakraborty C, Kim J. PLK-1: Angel or devil for cell cycle progression. Biochim Biophys Acta 2016; 1865:190-203; PMID:26899266
- Cholewa BD, Liu X, Ahmad N. The role of polo-like kinase 1 in carcinogenesis: cause or consequence. Cancer Res 2013; 73:6848-55; PMID:24265276; http://dx.doi.org/10.1158/0008-5472.CAN-13-2197
- Bakhoum SF, Kabeche L, Murnane JP, Zaki BI, Compton DA. DNA-damage response during mitosis induces whole-chromosome missegregation. Cancer Discov 2014; 4:1281-9; PMID:25107667; http://dx.doi.org/10.1158/2159-8290.CD-14-0403
- Elia AE, Rellos P, Haire LF, Chao JW, Ivins FJ, Hoepker K, Mohammad D, Cantley LC, Smerdon SJ, Yaffe MB. The molecular basis for phosphodependent substrate targeting and regulation of Plks by the Polo-box domain. Cell 2003; 115:83-95; PMID:14532005; http://dx.doi.org/10.1016/S0092-8674(03)00725-6
- Jang YJ, Ma S, Terada Y, Erikson RL. Phosphorylation of threonine 210 and the role of serine 137 in the regulation of mammalian polo-like kinase. J Biol Chem 2002; 277:44115-20; PMID:12207013; http://dx.doi.org/10.1074/jbc.M202172200
- Macurek L, Lindqvist A, Lim D, Lampson MA, Klompmaker R, Freire R, Clouin C, Taylor SS, Yaffe MB, Medema RH. Polo-like kinase-1 is activated by aurora A to promote checkpoint recovery. Nature 2008; 455:119-23; PMID:18615013; http://dx.doi.org/10.1038/nature07185
- Seki A, Coppinger JA, Jang CY, Yates JR, Fang G. Bora and the kinase Aurora a cooperatively activate the kinase Plk1 and control mitotic entry. Science 2008; 320:1655-8; PMID:18566290; http://dx.doi.org/10.1126/science.1157425
- Hutterer A, Berdnik D, Wirtz-Peitz F, Zigman M, Schleiffer A, Knoblich JA. Mitotic activation of the kinase Aurora-A requires its binding partner Bora. Dev Cell 2006; 11:147-57; PMID:16890155; http://dx.doi.org/10.1016/j.devcel.2006.06.002
- Chan EH, Santamaria A, Sillje HH, Nigg EA. Plk1 regulates mitotic Aurora A function through betaTrCP-dependent degradation of hBora. Chromosoma 2008; 117:457-69; PMID:18521620; http://dx.doi.org/10.1007/s00412-008-0165-5
- Lee YC, Liao PC, Liou YC, Hsiao M, Huang CY, Lu PJ. Glycogen synthase kinase 3 β activity is required for hBora/Aurora A-mediated mitotic entry. Cell Cycle 2013; 12:953-60; PMID:23442801; http://dx.doi.org/10.4161/cc.23945
- Qin B, Gao B, Yu J, Yuan J, Lou Z. Ataxia telangiectasia-mutated- and Rad3-related protein regulates the DNA damage-induced G2/M checkpoint through the Aurora A cofactor Bora protein. J Biol Chem 2013; 288:16139-44; PMID:23592782; http://dx.doi.org/10.1074/jbc.M113.456780
- Seki A, Coppinger JA, Du H, Jang CY, Yates JR, Fang G. Plk1- and β-TrCP-dependent degradation of Bora controls mitotic progression. J Cell Biol 2008; 181:65-78; PMID:18378770; http://dx.doi.org/10.1083/jcb.200712027
- Feine O, Hukasova E, Bruinsma W, Freire R, Fainsod A, Gannon J, Mahbubani HM, Lindqvist A, Brandeis M. Phosphorylation-mediated stabilization of Bora in mitosis coordinates Plx1/Plk1 and Cdk1 oscillations. Cell Cycle 2014; 13:1727-36; PMID:24675888; http://dx.doi.org/10.4161/cc.28630
- Bruinsma W, Macurek L, Freire R, Lindqvist A, Medema RH. Bora and Aurora-A continue to activate Plk1 in mitosis. J Cell Sci 2014; 127:801-11; PMID:24338364; http://dx.doi.org/10.1242/jcs.137216
- Tavernier N, Noatynska A, Panbianco C, Martino L, Van Hove L, Schwager F, Léger T, Gotta M, Pintard L. Cdk1 phosphorylates SPAT-1/Bora to trigger PLK-1 activation and drive mitotic entry in C. elegans embryos. J Cell Biol 2015; 208:661-9; PMID:25753036; http://dx.doi.org/10.1083/jcb.201408064
- Thomas Y, Cirillo L, Panbianco C, Martino L, Tavernier N, Schwager F, Van Hove L, Joly N, Santamaria A, Pintard L, Gotta M. Cdk1 phosphorylates SPAT-1/Bora to promote Plk1 activation in C. elegans and human cells. Cell Rep 2016; 15:510-8; PMID:27068477; http://dx.doi.org/10.1016/j.celrep.2016.03.049
- Deppe U, Schierenberg E, Cole T, Krieg C, Schmitt D, Yoder B, von Ehrenstein G. Cell lineages of the embryo of the nematode Caenorhabditis elegans. Proc Natl Acad Sci U S A 1978; 75:376-80; PMID:272653; http://dx.doi.org/10.1073/pnas.75.1.376
- Sulston JE, Schierenberg E, White JG, Thomson JN. The embryonic cell lineage of the nematode Caenorhabditis elegans. Dev Biol 1983; 100:64-119; PMID:6684600; http://dx.doi.org/10.1016/0012-1606(83)90201-4
- Noatynska A, Panbianco C, Gotta M. SPAT-1/Bora acts with Polo-like kinase 1 to regulate PAR polarity and cell cycle progression. Development 2010; 137:3315-25; PMID:20823068; http://dx.doi.org/10.1242/dev.055293
- Adams PD, Sellers WR, Sharma SK, Wu AD, Nalin CM, Kaelin WGJ. Identification of a cyclin-cdk2 recognition motif present in substrates and p21-like cyclin-dependent kinase inhibitors. Mol Cell Biol 1996; 16:6623-33; PMID:8943316; http://dx.doi.org/10.1128/MCB.16.12.6623
- Chen J, Saha P, Kornbluth S, Dynlacht BD, Dutta A. Cyclin-binding motifs are essential for the function of p21CIP1. Mol Cell Biol 1996; 16:4673-82; PMID:8756624; http://dx.doi.org/10.1128/MCB.16.9.4673
- Liu D, Davydenko O, Lampson MA. Polo-like kinase-1 regulates kinetochore-microtubule dynamics and spindle checkpoint silencing. J Cell Biol 2012; 198:491-9; PMID:22908307; http://dx.doi.org/10.1083/jcb.201205090
- Tramier M, Zahid M, Mevel JC, Masse MJ, Coppey-Moisan M. Sensitivity of CFP/YFP and GFP/mCherry pairs to donor photobleaching on FRET determination by fluorescence lifetime imaging microscopy in living cells. Microsc Res Tech 2006; 69:933-9; PMID:16941642; http://dx.doi.org/10.1002/jemt.20370
- Sahoo H, Schwille P. FRET and FCS–friends or foes. Chemphyschem 2011; 12:532-41; PMID:21308943; http://dx.doi.org/10.1002/cphc.201000776
- Lössl P, Brunner AM, Liu F, Leney AC, Yamashita M, Scheltema RA, Heck AJ. Deciphering the interplay among multisite phosphorylation, interaction dynamics, and conformational transitions in a tripartite protein system. ACS Cent Sci 2016; 2:445-55; PMID:27504491; http://dx.doi.org/10.1021/acscentsci.6b00053
- Bayliss R, Fry A, Haq T, Yeoh S. On the molecular mechanisms of mitotic kinase activation. Open Biol 2012; 2:120136; PMID:23226601; http://dx.doi.org/10.1098/rsob.120136
- Xu J, Shen C, Wang T, Quan J. Structural basis for the inhibition of Polo-like kinase 1. Nat Struct Mol Biol 2013; 20:1047-53; PMID:23893132; http://dx.doi.org/10.1038/nsmb.2623