ABSTRACT
Sterile α motif and HD domain-containing protein 1 (SAMHD1) is a mammalian dNTP hydrolase (dNTPase) that regulates intracellular dNTP balance. We have previously reported that SAMHD1 mRNA and protein levels are significantly downregulated in CD4+ T-cells of patients with cutaneous T-cell lymphoma (CTCL), a disease characterized by infiltration of neoplastic CD4+ T-lymphocytes into the skin. However, functional significance of SAMHD1 in CTCL development and progression remains unknown. Here we investigate the mechanism by which SAMHD1 induces apoptosis in CTCL-derived CD4+ T-cells. We stably expressed exogenous SAMHD1 in the CTCL-derived HuT78 T-cell line containing a very low level of endogenous SAMHD1 protein. We found that low-level exogenous expression of SAMHD1 led to a significant reduction in HuT78 cell growth, proliferation, and colony formation. Exogenous SAMHD1 expression in HuT78 cells also resulted in increased spontaneous and Fas ligand (Fas-L)-induced apoptosis levels via activation of the extrinsic pathway, including caspase-8, −3 and −7. Additionally, increased SAMHD1 significantly reduced the protein and mRNA expression of the short isoform of cFLIP (cFLIPS), an important negative regulator of Fas-L-mediated apoptotic signaling. Our results indicate that exogenous SAMHD1 expression inhibits HuT78 cell growth and proliferation in part by increasing apoptosis. These findings implicate that SAMHD1 acts as an inhibitor in CTCL cell growth, suggesting that downregulation of SAMHD1 expression in neoplastic T-cells can facilitate uncontrolled cell proliferation.
Introduction
SAMHD1 is the first identified mammalian dNTPase,Citation1-4 which plays a key role in regulation of dNTP homeostasis through hydrolysis of intracellular dNTPs.Citation5 SAMHD1 has been identified as an important host restriction factor that inhibits the infection of several retroviruses and DNA viruses by diminishing the intracellular dNTP pool needed for their propagation.Citation1,6-9 SAMHD1 has also been implicated in regulating immune responses.Citation10 Homozygous mutations in SAMHD1 have been identified in 17% of patients with Aicardi-Goutières syndrome (AGS), an autoimmune disorder that is attributed to excessive intracellular nucleic acids. Additionally, homozygous deletion in the SAMHD1 gene was also identified in atypical AGS patients.Citation11
Despite few studies (reviewed inCitation12), significance of SAMHD1 function in development and progression of cancer remains unknown. SAMHD1 somatic mutations have been identified in several cancers, including solid cancers such as glioblastoma,Citation13 colorectal,Citation14-16 breast,Citation15 lung,Citation17 pancreaticCitation18 cancers and blood-related malignancies such as chronic lymphocytic leukemia (CLL)Citation19,20 and myeloma .21 Additionally, SAMHD1 mRNA and/or protein expression is also significantly downregulated in CLL,Citation19 breastCitation19 and lungCitation22 cancers. Our recent studies using the monocytic leukemia cell line (THP-1) showed that SAMHD1 gene silencing significantly increases cell proliferation, reduces apoptosis, and redistributes the cells into G1/G0 phase of the cell cycle.Citation23 These results collectively suggest an important role of SAMHD1 in cancer development and progression.
Cutaneous T-cell lymphoma (CTCL) is a heterogeneous group of non-Hodgkin's lymphomas that is primarily characterized by infiltration of proliferative CD4+ T-lymphocytes into the skin.Citation24,25 The two most studied subtypes of CTCL are the indolent form mycosis fungoides (MF) and the more aggressive, leukemic variant, Sézary syndrome (SS) that is characterized by presence of malignant CD4+ T-cells in the blood.Citation25-27 Resistance to apoptosis is a major hallmark of several cancers including CTCL.Citation28,29 Several studies suggest that the enhanced proliferation of malignant T-cells in CTCL is largely due to the increased resistance to apoptosis via defective apoptotic signaling.Citation28-31 Dysregulation of Fas-L-mediated apoptotic signaling and its mediators including Fas-L, Fas, caspase-8 and cellular FLICE-like inhibitory protein (cFLIP) is a major factor in CTCL development.Citation30,32-41 cFLIP inhibits the Fas-L signaling by preventing Fas-mediated activation of caspase-8 and caspase-10.Citation36,37 While Fas-L and Fas are significantly downregulated in CTCL-derived primary CD4+ T-cells and cell lines, cFLIP is highly overexpressed.Citation28,29,32,36,37,39,40 Additionally, several other regulators of apoptosis are aberrantly expressed or inactivated, leading to reduced sensitivity of CTCL-derived cells to apoptotic stimuli.Citation41,42 Recent genome sequencing studies have improved the understanding of altered gene expression in CTCL patientsCitation43-48; however, the pathogenesis and progression of CTCL is not completely understood and therefore there is a clear lack of effective targeted treatment strategies.
We have previously demonstrated that SAMHD1 mRNA and protein levels are significantly downregulated in peripheral blood mononuclear cells (PBMC) and CD4+ T-cells of CTCL (SS and MF) patients relative to cells from healthy donors.Citation49 Our previous studies also show that SAMHD1 expression is lower in lymphoma and leukemia derived CD4+ T-cell lines relative to primary CD4+ T-cells from healthy donors.Citation50 Additionally, SAMHD1 downregulation in these cell lines and in SS patient cells highly correlated with significant increase in SAMHD1 promoter DNA methylation status.Citation49,50 SAMHD1 expression was induced by treatment of these CD4+ T-cell lines with methyl-transferase and histone deacetylase inhibitors, suggesting that SAMHD1 expression is regulated by epigenetic modulations.Citation49,50 However, the functional significance of SAMHD1 downregulation in CTCL pathophysiology remains unknown.
Herein, we report a novel growth inhibitory function of SAMHD1 in CTCL-derived cells. Exogenous expression of SAMHD1 in a CTCL-derived CD4+ T-cell line (HuT78) resulted in significant inhibition of cell growth and proliferation relative to control cells. SAMHD1 expression also reduced the ability of HuT78 cells to form colonies. Moreover, SAMHD1 expression in HuT78 cells induced spontaneous apoptosis via increased activation of extrinsic apoptotic signaling mediators, and sensitizes these cells to Fas-L-stimulated apoptosis. Mechanistically, SAMHD1 expression in HuT78 cells led to a significant reduction in expression of cFLIPS, a key anti-apoptotic regulator that is commonly overexpressed in CTCL patients.Citation32,41 Our findings suggest that SAMHD1 can inhibit uncontrolled proliferation of neoplastic T-cells in CTCL in part by inducing apoptosis, and thus may function as a potential tumor suppressor in CTCL.
Results
Exogenous SAMHD1 expression in CTCL-derived HuT78 cells
We have reported that SAMHD1 mRNA and protein level expression is significantly downregulated in CD4+ T-cells from SS patients and several lymphoma and leukemia derived CD4+ T-cell lines relative to cells from healthy donors.Citation49,50 To determine the functional significance of downregulated SAMHD1 expression in CTCL patients, we utilized SS patient-derived HuT78 CD4+ T-lymphocytic cell line as a model, which expresses very low, but detectable endogenous SAMHD1 protein.Citation50 Using lentiviral vector-mediated transduction, we stably expressed HA-tagged SAMHD1 in these cells. Exogenous expression of SAMHD1 in HuT78 cells was validated by immunoblotting (anti-HA) () and qPCR analyses ().
Figure 1. Exogenous SAMHD1 expression inhibits HuT78 cell growth and proliferation. HuT78 cells expressing hemagglutinin (HA)-tagged SAMHD1 were generated via lentiviral vector-mediated transduction. (A) Exogenous expression of SAMHD1 was validated by immunoblotting analysis using antibodies to HA (SAMHD1) and GAPDH (loading control). (B) Total RNA was isolated from HuT78 vector or SAMHD1-expressing cells and qPCR analysis was performed to quantify the relative SAMHD1 mRNA levels. GAPDH mRNA levels were quantified as internal control. (C) HuT78 vector control and SAMHD1 expressing cells were seeded in triplicate in 24-well plates at a density of 1 × 104 cells/well in 1 ml culture media on day 0 and live cells were counted on indicated days via trypan-blue exclusion method. (D) Cell lines were seeded on day 0 in 4 replicates in 96-well plates at density of 1 × 103 cells/well in 100 µl and cell proliferation was determined on indicated days utilizing an MTS-based proliferation assay. All the data presented are representative of 3 or more independent experiments. (C-D) ***, p < 0.0001.
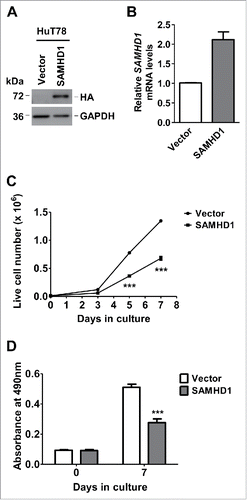
Exogenous SAMHD1 expression inhibits HuT78 cell proliferation and clonogenic potential
To determine whether increased SAMHD1 expression alters the HuT78 cell growth and proliferation, we performed cell growth assay and MTS-based cell proliferation assay using SAMHD1-expressing and vector control cells. As indicated in , using live cell counting via trypan blue exclusion method on 3, 5 and 7 d post-seeding, we noticed a significant reduction (49% and 53% reduction on days 5 and 7, respectively, P ≤ 0.0001) in growth rate of SAMHD1-expressing cells relative to control cells on day 5 and 7. Further, we measured the cell proliferation in these cell lines using the MTS-based cell proliferation assay. Consistent with the changes in cell growth, cells with ectopic SAMHD1 expression showed a significant reduction (46%, P < 0.0001) in cell proliferation and viability on day 7 (). These results indicate that exogenous expression SAMHD1 inhibits HuT78 cell growth and proliferation.
We next assessed the effect of SAMHD1 on the tumorigenicity of CTCL cells in vitro by measuring their clonogenic potential via methylcellulose media-based colony formation assay. HuT78 cells with SAMHD1 expression showed a significant reduction (41%) in total number of colonies formed relative to empty-vector control cells (). Furthermore, we observed that the SAMHD1 cells formed relatively smaller colonies than the control cells (). These data suggest that SAMHD1 expression impairs clonogenic potential in CTCL cells.
Figure 2. Exogenous SAMHD1 expression in HuT78 cells results in reduced colony formation. HuT78 vector control, and SAMHD1-expressing cells were plated in duplicate in 6-well plates at a density of 1 × 103 cells/well in 1 ml methyl cellulose medium and were left in culture for 10 d. (A) Numbers of colonies were counted after 10 d following standard counting criteria (all colonies with >25 cells were counted). Data is presented as % of colony yield relative to vector control cells (left panel). **, p < 0.005, Representative images (20 × magnification) of colonies formed after 10 d (right panel). (B) Numbers of colonies of different sizes were counted after 10 d based on following standard counting criteria (small: <25 cells; medium: 25–50 cells, and large: >50 cells). Data is presented as percentages of colony yield relative to vector control cells. All the data presented are representative of 3 independent experiments.
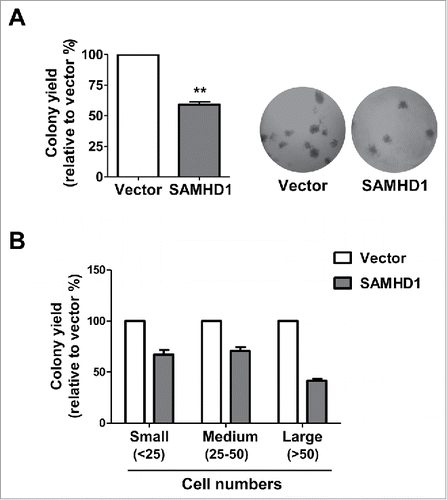
SAMHD1 expression in HuT78 cells leads to increased levels of sub-G1 population and apoptosis
Previous studies have reported that altered SAMHD1 expression can influence DNA replication and cell cycle progression.23,51-53 SAMHD1 associates with several proteins that are involved in cell cycle regulation, such as CDK1, CDK2, and cyclin A2.Citation51,53 Because altered cell cycle progression can result in significant changes in the rate of cell growth and proliferation, we next assessed whether SAMHD1-induced growth inhibition was due to altered cell cycle status in these cells. In this regard, we performed a cell-cycle analysis with SAMHD1-expressing HuT78 cells to identify the percentage of cells in specific (G1, S, G2/M and Sub-G1) phases of cell cycle. Although we did not notice significant changes in the G1, S, and G2/M phases of cell cycle between control cells and SAMHD1-expressing cells (), there was a significant increase (∼2.2-fold, P = 0.0001) in the Sub-G1 population of SAMHD1-expressing cells relative to vector control cells ().
Figure 3. Exogenous SAMHD1 expression induces apoptosis and caspase-3/7 activity via activation of extrinsic apoptosis signaling. HuT78 vector control and SAMHD1 expressing cells were seeded at density of 2 × 104 cells per 6-cm dish containing 5 ml growth media on day 0 following trypan-blue exclusion method. (A) Seven days post-seeding, cells in triplicate, were stained with propidium iodide (PI) and cell cycle analysis was performed via flow cytometry. Percentages of cells in G1/G0, S, and G2/M phases of cell cycle are presented. (B) Percentage of cells in Sub-G1 phase are presented. (C) HuT78 vector control and SAMHD1-expressing cells were seeded at density of 2 × 104 cells per 6-cm dish containing 5 ml growth media on day 0 following trypan-blue exclusion method. Seven days post-seeding, cells were stained with annexin V-PE and 7-AAD in triplicate and analyzed by flow cytometry. Representative flow cytometric profiles are presented (left panel). The percentages of non-apoptotic cells, early apoptotic cells, and late apoptotic cells were quantified as presented (right panel). (D) On day 7 post-seeding, cell lysates from all cell lines were collected and western analysis was performed using the antibodies to caspase-8, caspase-9, caspase-3, PARP, HA (SAMHD1), and GAPDH (loading control). (E) On day 7 post-seeding, 1 × 104 cells per cell line were collected in 4 replicates and incubated in 100 µl of Caspase-Glo 3/7 reagent at room temperature in dark for 1 hour. Caspase-3/7 activity was then determined by measuring luminescence values. All the data presented are representative of 3 independent experiments. B, C, E, ***, p < 0.001.
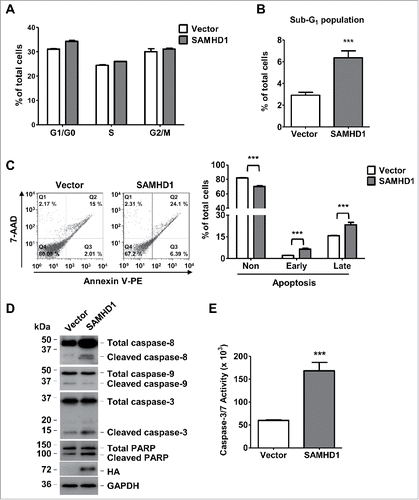
Sub-G1 population comprises of cells that exhibit irregular nuclear DNA fragmentation, a hallmark of cells undergoing apoptosis.Citation54 Therefore, in order to directly assess whether SAMHD1-mediated inhibition of CTCL cell growth is due to increased apoptosis, we performed an apoptosis assay via dual staining of the cells with annexin V and 7-aminoactinomycin D (7-AAD). Indeed, consistent with the increased sub-G1 population, Hu78 cells expressing SAMHD1 showed a significantly higher number cells undergoing early (3.1-fold, positive for annexin V only, P < 0.001) and late (1.6-fold, positive for both annexin V and 7-AAD, P < 0.001) apoptosis compare with vector control cells (). These results suggest that increased SAMHD1 expression does not significantly affect the cell cycle progression, but enhances apoptosis levels in CTCL cells.
SAMHD1 induces apoptosis in HuT78 cells via activation of the extrinsic apoptotic pathway
To investigate the mechanisms of SAMHD1-induced apoptosis in HuT78 cells, we examined the activation status of several apoptotic signaling mediators via immunoblotting. Exogenous expression of SAMHD1 in HuT78 cells resulted in enhanced activation of caspase-8, initiator of caspase cascade mediating the extrinsic apoptosis pathway, compared to control cells (). We also observed a marked increase in activation of caspase-3, a downstream effector caspase in the caspase signaling, in SAMHD1-expressing cells relative to control cells (). Consistently, SAMHD1-expressing HuT78 cells also showed enhanced cleavage of poly (ADP-ribose) polymerase (PARP) (), a direct substrate of activated caspase-3 and a marker of apoptosis.Citation55,56 Interestingly, we did not observe any significant changes in activation of caspase-9 (), which mediates the intrinsic pathway of apoptosis.Citation34 These results suggest that SAMHD1-induced apoptosis is specifically mediated by the extrinsic apoptotic pathway, but not the intrinsic pathway.
Furthermore, to validate the increased apoptosis in exogenous SAMHD1-expressing HuT78 cells, we measured the activity of caspase-3 and −7 (effector caspases) in these cells by performing Caspase-Glo 3/7 assay as described under materials and methods. As expected, we observed a significantly (2.8-fold, P < 0.0001) higher caspase activity in SAMHD1-expressing cells compare with control cells (). Collectively, these results indicate that SAMHD1 leads to increased spontaneous apoptosis in HuT78 cells via activation of extrinsic apoptosis signaling mediated by caspase-3, −7 and −8.
SAMHD1 sensitizes HuT78 cells to Fas-L-induced apoptosis
While intrinsic apoptosis pathway is initiated in response to intracellular stress, extrinsic apoptosis is initiated by activation of cell death surface receptors, such as Fas receptor by Fas-L.Citation34 Since Fas and its downstream signaling mediators are dysregulated in cells from CTCL patients resulting in defective apoptosis,Citation32,33,40 we next assessed whether the effect of SAMHD1 on induced apoptosis is mediated by Fas-L/Fas activated pathway. In this regard, we stimulated the vector control and SAMHD1-expressing HuT78 cells with Fas-L for 48 hours. Post-treatment, we determined the Fas-L induced apoptosis levels in these cells. Interestingly, Fas-L stimulation resulted in a significantly higher percentage of late apoptosis cells (by ∼2.5-fold, P < 0.001) in SAMHD1 cells relative to vector control cells (). These results indicate that SAMHD1-expressing cells are significantly more susceptible to Fas-L-induced apoptosis compare with vector control cells.
Figure 4. Exogenous SAMHD1 expression in HuT78 cells significantly increases Fas-L induced apoptosis and caspase-3/7 activity. (A) HuT78 vector control and SAMHD1-expressing cells were treated with 100 ng/ml Fas-L for 48 hours. After 48 hours of treatment, all the cells in triplicate were stained with annexin V-PE and 7-AminoactinomycinD (7-AAD) followed by flow cytometry. Representative flow cytometric profiles are presented (left panel). The percentage of non-apoptotic cells, early apoptotic cells, and late apoptotic cells were quantified as presented (right panel). (B) HuT78 vector control and SAMHD1-expressing cells were treated with 100 ng/ml Fas-L for 48 hours. Post-treatment, 1 × 104 cells per cell line were collected in triplicate and incubated in 100 µl of Caspase-Glo 3/7 reagent at room temperature in dark for 1 hour. Caspase-3/7 activity was then determined by measuring luminescence values. C, HuT78 vector control and SAMHD1-expressing cells were treated with Fas-L (100 ng/ml) for 48 hours. Post-treatment, cell lysates from all cell lines were collected and immunoblotting was performed using the antibodies to caspase-8, caspase-3, PARP, and GAPDH (loading control). All the data presented are representative of 3 independent experiments. A–B, ***, p < 0.001.
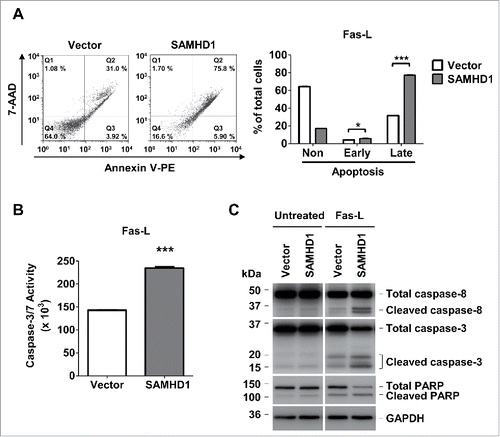
To further validate that SAMHD1 expression sensitizes HuT78 cells to Fas-L stimulation, we performed the caspase-3/7 activity assay in these cells. SAMHD1-expressing cells exhibited significantly higher levels (P < 0.001) of caspase-3/7 activation compare with vector control cells in presence of Fas-L stimulation (). Consistently, immunoblotting analysis clearly indicated that Fas-L induced activation of extrinsic apoptotic mediators (caspase-8, caspase-3 and cleavage of PARP) was markedly higher in SAMHD1 expressing cells than in the vector control cells (). Collectively, these findings suggest the role of SAMHD1 in sensitizing the HuT78 cells to Fas-L-induced cell death.
Exogenous SAMHD1 expression reduces cFLIPS protein and mRNA levels in HuT78 cells
cFLIP is a key negative regulator of death-receptor mediated apoptotic pathways including the Fas-L signaling.Citation36,37 The two major isoforms of cFLIP, cFLIP-long (cFLIPL) and cFLIP-short (cFLIPS), are overexpressed in several cancers including CTCL.Citation32,35,37,57 Since exogenous SAMHD1 expression significantly increased Fas-L sensitivity in HuT78 cells, we next determined the role of cFLIP in SAMHD1-induced apoptosis. In this regard, we measured the protein expression of cFLIPL and cFLIPS in SAMHD1-expressing cells via immunoblotting. We found that cFLIPS protein level was significantly lower in SAMHD1-expressing cells compared with that in the control cells (∼65%; ). Additionally, qPCR analysis demonstrated a slight but significant reduction in cFLIPS mRNA level (∼3-fold; ) in SAMHD1-expressing cells compared with that in the control cells, suggesting that reduced cFLIPS protein expression may in part be due to reduced cFLIPS mRNA levels. In comparison, cFLIPL protein and mRNA levels were not significantly altered in SAMHD1-expressing cells (). Collectively, these results suggest that SAMHD1-induced Fas-L sensitivity in HuT78 cells may in part be due to reduced cFLIPS expression.
Figure 5. Exogenous SAMHD1 expression in HuT78 cells reduces the levels of cFLIPS protein and mRNA. HuT78 vector control and SAMHD1-expressing cells were seeded at density of 2 × 104 cells per 6-cm dish containing 5 ml growth media on day 0 following trypan-blue exclusion method. (A) On day 7 post-seeding, cell lysates were collected and immunoblotting analysis was performed for cFLIP, HA (SAMHD1) and GAPDH (loading control) (left panel). Densitometry was performed using ImageJ to quantify the relative cFLIPL and cFLIPS protein levels (right panel). ***, p < 0.001 (B) On day 7 post-seeding, total RNA was isolated from HuT78 vector or SAMHD1-expressing cells and qPCR analysis was performed to quantify the relative cFLIPL and cFLIPS mRNA levels. GAPDH mRNA levels were quantified as internal control. All the data presented are representative of 3 independent experiments. *, p < 0.05.
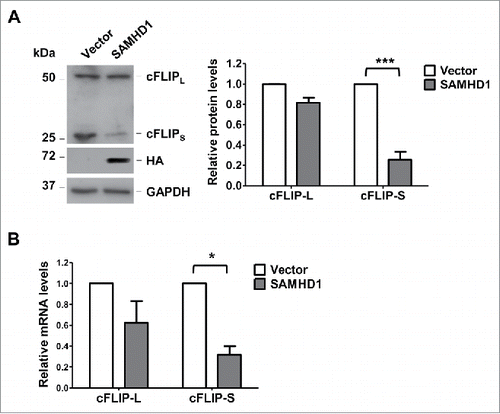
Discussion
In this study, we identify a novel function of SAMHD1 in CTCL-derived HuT78 cells as an anti-proliferative and apoptosis-inducing protein. We demonstrate that exogenous expression of SAMHD1 in HuT78 cells inhibits their growth and proliferation, and significantly reduces colony formation in these cells (). SAMHD1 overexpression has been reported to inhibit cell proliferation in solid tumor derived-cell lines, such as HeLa (cervical cancer)Citation19 and A549 (lung cancer)Citation22 cell lines. Our recent studies showed that SAMHD1 knockout in monocytic leukemia THP-1 cells leads to increased cell proliferation and decreased apoptosis relative to control cells expressing endogenous SAMHD1.Citation23 Our findings here provide the evidence demonstrating the anti-proliferative effect of SAMHD1 in CTCL-derived cells. Furthermore, we provide new evidence for the role of SAMHD1 inhibiting colony formation potential (), an important aspect in cancer development. It remains to be investigated whether this reduced ability to form colonies by cells with SAMHD1 expression is direct result of reduced proliferation. In our previous studies, we have reported that SAMHD1 mRNA and protein expression is significantly downregulated in CTCL patient PBMCs and lymphoma and leukemia-derived CD4+ T-cell lines compare with healthy donor CD4+ T-cells.Citation49,50 Together, these results suggest that normal SAMHD1 expression in CD4+ T-cells can prevent them from uncontrolled proliferation. Thus, SAMHD1 downregulation in cancer cells can contribute to neoplasia and malignancy. To further validate the potential tumor suppressive function of SAMHD1, it is important to determine whether exogenous expression of SAMHD1 can also inhibit CTCL-derived cell proliferation in vivo. It is interesting to note that SAMHD1 knockout mice do not show any spontaneous tumorigenic phenotype under normal conditions.Citation58,59 However, SAMHD1 silencing per se might not be able to cause carcinogenesis. It is possible that additional driver mutations might be required as is the case with several tumor suppressor genes.
Figure 6. Summary of the findings and proposed mechanisms. Our data indicate that increased SAMHD1 expression inhibits growth and proliferation in CTCL-derived CD4+ T-cells by inducing spontaneous and Fas-L stimulated apoptosis. SAMHD1 expression also diminishes colony forming potential in these cells. Furthermore, increased SAMHD1 expression also significantly reduces the mRNA and protein levels of cFLIPS, a key anti-apoptotic protein. Complete mechanisms by which SAMHD1 regulates cFLIPS expression, apoptosis and colony formation in HuT78 cells remain to be understood.
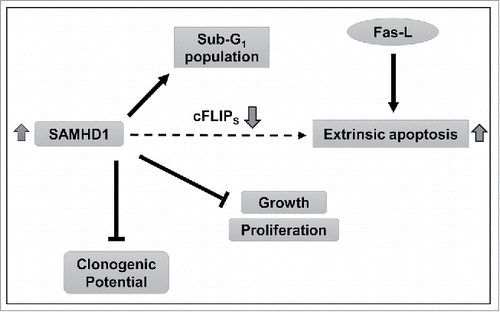
Changes in cell cycle progression can affect cell proliferation and SAMHD1 interacts with several proteins involved in regulation of cell cycle.Citation51,53 While we did not observe significant changes in G1/G0, S, and G2/M phases of cell cycle in HuT78 cells expressing exogenous SAMHD1 relative to control cells, we found an increase in the sub-G1 population (), which comprises of cells undergoing apoptosis.Citation54 SAMHD1 has been implicated in mediating human T-cell leukemia virus type 1-infection induced apoptosis in primary monocytes,Citation60 while its role in apoptosis of cancer cells has not been investigated. We observed that exogenous expression of SAMHD1 results in increased levels of spontaneous and Fas-L induced apoptosis in HuT78 cells. These findings are consistent with our recent studies in THP-1 cells that demonstrate reduced spontaneous apoptosis levels upon SAMHD1 silencing,Citation23 suggesting an important role of SAMHD1 in regulating apoptosis.
Our data also indicate that SAMHD1-induced apoptosis is mediated via increased activation of extrinsic apoptotic signaling. It should be noted that the exogenous SAMHD1 protein level expressed in HuT78 cells was much lower than the endogenous protein in primary CD4+ T-cells from healthy donors (data not shown). Thus, the modest increase in apoptosis upon SAMHD1 expression in HuT78 cells was likely due to low levels of expression. Evasion of apoptosis is one of the major mechanisms through which malignant cells accomplish uncontrolled proliferation in several cancers including CTCL. Indeed, increasing evidence suggests that CTCL development might be primarily due to defective apoptosis rather than enhanced proliferation.Citation28,29,39,40 This is achieved mainly through downregulation or loss of function of pro-apoptotic mediators and simultaneous overexpression or increased activation of anti-apoptotic regulators.
Interestingly, several mediators of Fas-L-induced apoptosis have been reported to be dysregulated in CTCL, resulting in cells developing resistance to Fas-L stimulation.Citation29,32,33,39,40 cFLIP is an important anti-apoptotic protein that inhibits Fas-L signaling by inhibiting the caspase-8/10 activation. The two major splice variants of cFLIP (cFLIPL and cFLIPS) are overexpressed in CTCL and other cancers, leading to increased resistance to death-receptor-mediated apoptosis and cell proliferation.Citation32,36,37,57 We demonstrate that exogenous SAMHD1 expression reduces the cFLIPS protein and mRNA levels in HuT78 cells (). In comparison, we did not observe significant changes in cFLIPL protein and mRNA levels upon SAMHD1 expression. These results suggest that SAMHD1-induced apoptosis and Fas-L sensitivity can be a result of reduced expression of cFLIPS but not cFLIPL. Interestingly, evidence indicates that while cFLIPL can sometimes function to promote Fas-L mediated apoptosis via caspase-8 activation, cFLIPS only functions as an anti-apoptotic regulator.Citation61-63
Further studies using additional cell lines and primary cells derived from CTCL patients are needed to better understand the role of cFLIPS in regulating SAMHD1-induced apoptosis and Fas-L sensitivity, as well as the underlying mechanisms. It is important to determine whether SAMHD1 can directly regulate Fas-L signaling by associating with other Fas-L signaling mediators such as Fas-L, Fas, and caspase-8. Anti-apoptotic proteins belonging to the inhibitors of apoptosis family and the Bcl-2 family, are also aberrantly expressed in CTCL.Citation41,42,64 However, we did not observe any significant changes in expression levels Bcl-2 or X-liked inhibitor of apoptosis (XIAP) upon exogenous expression of SAMHD1 in HuT78 cells (data not shown), suggesting that their involvement in SAMHD1-mediated apoptosis in CTCL is unlikely. Furthermore, the intracellular dNTP levels of SAMHD1-expressing HuT78 cells were not significantly reduced compare with control cells, suggesting that the effects that we observed may not be dependent on dNTP reduction in the cells. It is also interesting to note that our previous studies in diving cells such as HEK293T and HeLa cell lines show that very high levels of exogenous SAMHD1 overexpression can only lead to a modest reduction in intracellular dNTP levels.Citation8
In conclusion, we provide evidence for a novel function of SAMHD1 as an anti-proliferative and apoptosis inducing protein in CTCL-derived cells. We also demonstrate that SAMHD1 inhibits colony forming potential in CTCL-derived cells. Together, these findings implicate SAMHD1 as an important inhibitor in CTCL tumorigenesis (). Further studies identifying the molecular mechanisms of SAMHD1-induced apoptosis will facilitate development of new and effective therapeutic avenues in the treatment of CTCL and other cancers.
Materials and methods
Generation of HuT78 cell lines with stable expression of SAMHD1
Human CD4+ T-lymphocytic cell line HuT78 derived from an Sézary syndrome patient was obtained from the American Type Culture Collection (ATCC, TIB-161). To establish HuT78 cell lines stably expressing human SAMHD1, lentiviral vectors were generated by transfection of human embryonic kidney 293T (HEK293T) cells with empty pLenti-Puro plasmid or with pLenti-Puro plasmid encoding HA-tagged SAMHD1 along with the lentiviral packaging vectors as described.Citation65 Twenty-four hours post-transfection, the supernatants were harvested and passed through a 0.2 μm filter to remove the cell debris. Viral vectors were used to transduce HuT78 cells in the presence of polybrene (8 μg/mL) via spinoculation. Post-transduction, all the transduced HuT78 cells were selected by culturing cells in RPMI-1640 media containing 0.5 μg/mL puromycin.
Cell culture and treatments
HuT78 cells were cultured in RPMI-1640 medium containing 10% fetal bovine serum and 1% penicillin/streptomycin. HuT78 cells transduced with lentiviral vector expressing SAMHD1 were cultured in RPMI-1640 medium additionally containing 0.5 µg/ml of puromycin. HEK293T cells were maintained in complete DMEM supplemented with 10% fetal bovine serum and 1% penicillin/streptomycin. All the cell lines utilized for the presented studies were maintained at 37°C, 5% CO2 and were tested negative for mycoplasma contamination using universal mycoplasma detection kit (ATCC, #30–101–2K). For the Fas-L mediated apoptosis studies, cells were treated with 100 ng/mL Fas-L (Sigma Aldrich, #SRP3036) for 48 hours.
Protein extraction and western analysis
Cells were collected, washed with ice cold PBS and were lysed using 1× cell lysis buffer (Cell signaling, #9803). Collected protein samples were normalized via BCA assay and were resolved by SDS-PAGE followed by immunoblotting as described.Citation65 Immunoblotting was performed using the following antibodies: HA-11 (1:1000, Covance #901501), caspase-8 (1:1000, Cell signaling #9746S), caspase-3 (1:1000, Cell signaling #9662S), PARP (1:1000, Cell Signaling, #9542S), FLIPS/L (1:500, Santa Cruz Biotechnology sc-5276) and GAPDH (1:3000, Bio-Rad #AHP1628).
Quantitative PCR analysis
Cells were collected, washed with PBS and total RNA was isolated using RNeasy minikit (Qiagen). RNA was then converted to cDNA and qPCR was performed using specific cDNA primers to quantify relative mRNA levels as described previously.Citation50 GAPDH mRNA levels were quantified and used as an endogenous reference.
Cell growth assay
HuT78 vector control or SAMHD1-expressing cells were seeded into 24-well plates in triplicate at a density of 1 × 104 cells per well in 1 ml culture media. On days 3, 5 and 7, number of live cells from each well was determined using trypan blue exclusion method. Average of the live cell number from the 3 replicates was determined.
Cell proliferation assay
Vector control or SAMHD1-expressing cells were plated into 96-well plates at a density of 1 × 103 cells per well in 100 µl of culture media (4 replicates). On the indicated time points, 20 µl of CellTiter 96® AQueous One Cell Proliferation assay reagent (Promega, #G3582) was added to each well and plates were incubated for 1 hour at 37°C. After 1 hour, absorbance values were measured at 490 nm and 690 nm (non-specific background). Absorbance values were then blank (culture media only) and non-specific background-corrected and average of data from all the replicates was calculated.
Colony formation assay
Clonogenic potential in HuT78 cells was determined using methyl cellulose-based culture media. Vector control or SAMHD1-expressing cells were plated in 6-well plates (in duplicate) at a density of 1 × 103 cells per well in 1 ml of culture media (MethoCult, STEMCELL technologies, #H4230) following manufacturer's instructions. After 10 d in culture, numbers of colonies were quantified following the standard scoring criteria: (small: <25 cells; medium: 25–50 cells, and large: >50 cells). Representative images of the cell colonies were taken at 20 × magnification.
Cell cycle analysis
Vector control and SAMHD1-expressing cells were plated at a density of 2 × 104 in separate 6-cm culture dishes in 5 ml media. Seven days post-seeding, cells were collected and cell cycle analysis was performed using the Guava Cell Cycle Reagent (EMD Millipore, #4500–0220) following the provided instructions. Briefly, the collected cells were plated in a 96-well plate (in triplicate), washed with ice-cold PBS, fixed with 70% ethanol, and stained with 200 µl of Guava Cell Cycle Reagent. Flow cytometry was performed using Guava flow cytometer and Guava Cytosoft 4.2 software to determine the distribution of cells in G0/G1, S, G2/M, and Sub-G0/G1 phases of cell cycle.
Apoptosis assay
Percentage of cells undergoing early and late apoptosis was quantified by performing Guava Nexin Annexin V Assay (EMD Millipore, #4500–0450) according to the manufacturer's instructions. Seven days post-seeding (as described under cell cycle analysis) or 48 hours post-treatment with 100 ng/mL Fas-L, cells were washed with ice-cold PBS and were resuspended in 1X binding buffer at a density of 1 × 106 cells/ml. Cells were then stained with annexin V-PE and 7-AAD for 15 min at room temperature in dark. Cells were then analyzed for apoptosis via flow cytometry using Guava flow cytometer and Guava Nexin software. Cells negative for both annexin V and 7-AAD were labeled non-apoptotic while cells positive for annexin V only were labeled early apoptotic and double positive cells for annexin V and 7-AAD were labeled late apoptotic.
Caspase-3/7 activity assay
Caspase-3/7 activity in cells was determined using Caspase-Glo 3/7 assay kit (Promega, #G8090). Briefly, 7 d post-seeding or 48 hours post treatment with Fas-L, 1 × 104 cells were counted and seeded (in 4 replicates) per well in a white opaque 96-well plate. Then, 100 µl of Caspase-Glo 3/7 reagent was added to each well and incubated at room temperature for 1 hour in dark. Luminescence readings taken were blank (media only) corrected and average of all replicates were calculated.
Statistical analyses
Error bars displayed on the bar graphs represent standard deviations. P values were calculated based on the non-parametric Student's t-test. P<0.05 is considered as statistically significant.
Disclosure of potential conflicts of interest
No potential conflicts of interest were disclosed.
Authors' contributions
LW conceived the study and designed experiments with KMK. KMK performed experiments and analyzed data with LW. ML and SL generated SAMHD1-expressing HuT78 cell line. KMK and LW wrote the manuscript. All the authors read and approved the manuscript.
Acknowledgments
We thank Rebeca Kohnken and the Wu lab members for valuable discussions, and Giovanna Merchand Reyes for technical assistance.
Funding
This work was supported by a NIH grant (CA181997) to LW. LW is also supported in part by NIH grants (AI104483, AI120209, and AI127667) and the Public Health Preparedness for Infectious Diseases Program of the Ohio State University.
References
- Goldstone DC, Ennis-Adeniran V, Hedden JJ, Groom HC, Rice GI, Christodoulou E, Walker PA, Kelly G, Haire LF, Yap MW, et al. HIV-1 restriction factor SAMHD1 is a deoxynucleoside triphosphate triphosphohydrolase. Nature 2011; 480:379-82; PMID:22056990; http://dx.doi.org/10.1038/nature10623
- Hrecka K, Hao C, Gierszewska M, Swanson SK, Kesik-Brodacka M, Srivastava S, Florens L, Washburn MP, Skowronski J. Vpx relieves inhibition of HIV-1 infection of macrophages mediated by the SAMHD1 protein. Nature 2011; 474:658-61; PMID:21720370; http://dx.doi.org/10.1038/nature10195
- Laguette N, Sobhian B, Casartelli N, Ringeard M, Chable-Bessia C, Ségéral E, Yatim A, Emiliani S, Schwartz O, Benkirane M. SAMHD1 is the dendritic- and myeloid-cell-specific HIV-1 restriction factor counteracted by Vpx. Nature 2011; 474:654-7; PMID:21613998; http://dx.doi.org/10.1038/nature10117
- Powell RD, Holland PJ, Hollis T, Perrino FW. Aicardi-Goutieres syndrome gene and HIV-1 restriction factor SAMHD1 is a dGTP-regulated deoxynucleotide triphosphohydrolase. J Biol Chem 2011; 286:43596-600; PMID:22069334; http://dx.doi.org/10.1074/jbc.C111.317628
- Franzolin E, Pontarin G, Rampazzo C, Miazzi C, Ferraro P, Palumbo E, Reichard P, Bianchi V. The deoxynucleotide triphosphohydrolase SAMHD1 is a major regulator of DNA precursor pools in mammalian cells. Proc Natl Acad Sci U S A 2013; 110:14272-7; PMID:23858451; http://dx.doi.org/10.1073/pnas.1312033110
- Baldauf HM, Pan X, Erikson E, Schmidt S, Daddacha W, Burggraf M, Schenkova K, Ambiel I, Wabnitz G, Gramberg T, et al. SAMHD1 restricts HIV-1 infection in resting CD4(+) T cells. Nat Med 2012; 18:1682-7; PMID:22972397; http://dx.doi.org/10.1038/nm.2964
- Lahouassa H, Daddacha W, Hofmann H, Ayinde D, Logue EC, Dragin L, Bloch N, Maudet C, Bertrand M, Gramberg T, et al. SAMHD1 restricts the replication of human immunodeficiency virus type 1 by depleting the intracellular pool of deoxynucleoside triphosphates. Nat Immunol 2012; 13:223-8; PMID:22327569; http://dx.doi.org/10.1038/ni.2236
- St Gelais C, de Silva S, Amie SM, Coleman CM, Hoy H, Hollenbaugh JA, Kim B, Wu L. SAMHD1 restricts HIV-1 infection in dendritic cells (DCs) by dNTP depletion, but its expression in DCs and primary CD4+ T-lymphocytes cannot be upregulated by interferons. Retrovirology 2012; 9:105; PMID:23231760; http://dx.doi.org/10.1186/1742-4690-9-105
- Antonucci JM, St. Gelais C, de Silva S, Yount JS, Tang C, Ji X, Shepard C, Xiong Y, Kim B, Wu L. SAMHD1-mediated HIV-1 restriction in cells does not involve ribonuclease activity. Nat Med 2016; 22:1072-4; PMID:27711056; http://dx.doi.org/10.1038/nm.4163
- Rice GI, Bond J, Asipu A, Brunette RL, Manfield IW, Carr IM, Fuller JC, Jackson RM, Lamb T, Briggs TA, et al. Mutations involved in Aicardi-Goutieres syndrome implicate SAMHD1 as regulator of the innate immune response. Nat Genet 2009; 41:829-32; PMID:19525956; http://dx.doi.org/10.1038/ng.373
- Leshinsky-Silver E, Malinger G, Ben-Sira L, Kidron D, Cohen S, Inbar S, Bezaleli T, Levine A, Vinkler C, Lev D, et al. A large homozygous deletion in the SAMHD1 gene causes atypical Aicardi-Goutieres syndrome associated with mtDNA deletions. Eur J Hum Genet 2011; 19:287-92; PMID:21102625; http://dx.doi.org/10.1038/ejhg.2010.213
- Kohnken R, Kodigepalli KM, Wu L. Regulation of deoxynucleotide metabolism in cancer: novel mechanisms and therapeutic implications. Mol Cancer 2015; 14:176; PMID:26416562; http://dx.doi.org/10.1186/s12943-015-0446-6
- Parsons DW, Jones S, Zhang X, Lin JC, Leary RJ, Angenendt P, Mankoo P, Carter H, Siu IM, Gallia GL, et al. An integrated genomic analysis of human glioblastoma multiforme. Science 2008; 321:1807-12; PMID:18772396; http://dx.doi.org/10.1126/science.1164382
- Comprehensive molecular characterization of human colon and rectal cancer. Nature 2012; 487:330-7; PMID:22810696; http://dx.doi.org/10.1038/nature11252
- Sjoblom T, Jones S, Wood LD, Parsons DW, Lin J, Barber TD, Mandelker D, Leary RJ, Ptak J, Silliman N, et al. The consensus coding sequences of human breast and colorectal cancers. Science 2006; 314:268-74; PMID:16959974; http://dx.doi.org/10.1126/science.1133427
- Rentoft M, Lindell K, Tran P, Chabes AL, Buckland RJ, Watt DL, Marjavaara L, Nilsson AK, Melin B, Trygg J, et al. Heterozygous colon cancer-associated mutations of SAMHD1 have functional significance. Proc Natl Acad Sci U S A 2016; 113:4723-8; PMID:27071091; http://dx.doi.org/10.1073/pnas.1519128113
- Liu J, Lee W, Jiang Z, Chen Z, Jhunjhunwala S, Haverty PM, Gnad F, Guan Y, Gilbert HN, Stinson J, et al. Genome and transcriptome sequencing of lung cancers reveal diverse mutational and splicing events. Genome Res 2012; 22:2315-27; PMID:23033341; http://dx.doi.org/10.1101/gr.140988.112
- Jones S, Zhang X, Parsons DW, Lin JC, Leary RJ, Angenendt P, Mankoo P, Carter H, Kamiyama H, Jimeno A, et al. Core signaling pathways in human pancreatic cancers revealed by global genomic analyses. Science 2008; 321:1801-6; PMID:18772397; http://dx.doi.org/10.1126/science.1164368
- Clifford R, Louis T, Robbe P, Ackroyd S, Burns A, Timbs AT, Wright Colopy G, Dreau H, Sigaux F, Judde JG, et al. SAMHD1 is mutated recurrently in chronic lymphocytic leukemia and is involved in response to DNA damage. Blood 2014; 123:1021-31; PMID:24335234; http://dx.doi.org/10.1182/blood-2013-04-490847
- Schuh A, Becq J, Humphray S, Alexa A, Burns A, Clifford R, Feller SM, Grocock R, Henderson S, Khrebtukova I, et al. Monitoring chronic lymphocytic leukemia progression by whole genome sequencing reveals heterogeneous clonal evolution patterns. Blood 2012; 120:4191-6; PMID:22915640; http://dx.doi.org/10.1182/blood-2012-05-433540
- Walker BA, Wardell CP, Melchor L, Hulkki S, Potter NE, Johnson DC, Fenwick K, Kozarewa I, Gonzalez D, Lord CJ, et al. Intraclonal heterogeneity and distinct molecular mechanisms characterize the development of t(4;14) and t(11;14) myeloma. Blood 2012; 120:1077-86; PMID:22573403; http://dx.doi.org/10.1182/blood-2012-03-412981
- Wang JL, Lu FZ, Shen XY, Wu Y, Zhao LT. SAMHD1 is down regulated in lung cancer by methylation and inhibits tumor cell proliferation. Biochem Biophys Res Commun 2014; 455:229-33; PMID:25449277; http://dx.doi.org/10.1016/j.bbrc.2014.10.153
- Bonifati S, Daly MB, St Gelais C, Kim SH, Hollenbaugh JA, Shepard C, Kennedy EM, Kim DH, Schinazi RF, Kim B, et al. SAMHD1 controls cell cycle status, apoptosis and HIV-1 infection in monocytic THP-1 cells. Virology 2016; 495:92-100; PMID:27183329; http://dx.doi.org/10.1016/j.virol.2016.05.002
- Scarisbrick JJ. Staging and management of cutaneous T-cell lymphoma. Clin Exp Dermatol 2006; 31:181-6; PMID:16487086; http://dx.doi.org/10.1111/j.1365-2230.2005.02019.x
- Olsen E, Vonderheid E, Pimpinelli N, Willemze R, Kim Y, Knobler R, Zackheim H, Duvic M, Estrach T, Lamberg S, et al. Revisions to the staging and classification of mycosis fungoides and Sezary syndrome: a proposal of the International Society for Cutaneous Lymphomas (ISCL) and the cutaneous lymphoma task force of the European Organization of Research and Treatment of Cancer (EORTC). Blood 2007; 110:1713-22; PMID:17540844; http://dx.doi.org/10.1182/blood-2007-03-055749
- Wong HK, Mishra A, Hake T, Porcu P. Evolving insights in the pathogenesis and therapy of cutaneous T-cell lymphoma (mycosis fungoides and Sezary syndrome). Br J Haematol 2011; 155:150-66; PMID:21883142; http://dx.doi.org/10.1111/j.1365-2141.2011.08852.x
- Siegel RS, Pandolfino T, Guitart J, Rosen S, Kuzel TM. Primary cutaneous T-cell lymphoma: review and current concepts. J Clin Oncol 2000; 18:2908-25; PMID:10920140
- Contassot E, French LE. Targeting apoptosis defects in cutaneous T-cell lymphoma. J Investigat Dermatol 2009; 129:1059-61; PMID:19369931; http://dx.doi.org/10.1038/jid.2009.14
- Meech SJ, Edelson R, Walsh P, Norris DA, Duke RC. Reversible resistance to apoptosis in cutaneous T cell lymphoma. Ann N Y Acad Sci 2001; 941:46-58; PMID:11594582; http://dx.doi.org/10.1111/j.1749-6632.2001.tb03710.x
- Braun FK, Fecker LF, Schwarz C, Walden P, Assaf C, Durkop H, Sterry W, Eberle J. Blockade of death receptor-mediated pathways early in the signaling cascade coincides with distinct apoptosis resistance in cutaneous T-cell lymphoma cells. J Investigat Dermatol 2007; 127:2425-37; PMID:17495957; http://dx.doi.org/10.1038/sj.jid.5700868
- Kacinski BM, Flick M. Apoptosis and cutaneous T cell lymphoma. Ann N Y Acad Sci 2001; 941:194-9; PMID:11594573; http://dx.doi.org/10.1111/j.1749-6632.2001.tb03723.x
- Contassot E, Kerl K, Roques S, Shane R, Gaide O, Dupuis M, Rook AH, French LE. Resistance to FasL and tumor necrosis factor-related apoptosis-inducing ligand-mediated apoptosis in Sezary syndrome T-cells associated with impaired death receptor and FLICE-inhibitory protein expression. Blood 2008; 111:4780-7; PMID:18314443; http://dx.doi.org/10.1182/blood-2007-08-109074
- Esmailzadeh S, Huang Y, Su MW, Zhou Y, Jiang X. BIN1 tumor suppressor regulates Fas/Fas ligand-mediated apoptosis through c-FLIP in cutaneous T-cell lymphoma. Leukemia 2015; 29:1402-13; PMID:25578476; http://dx.doi.org/10.1038/leu.2015.9
- Fulda S, Debatin KM. Extrinsic versus intrinsic apoptosis pathways in anticancer chemotherapy. Oncogene 2006; 25:4798-811; PMID:16892092; http://dx.doi.org/10.1038/sj.onc.1209608
- Safa AR, Day TW, Wu CH. Cellular FLICE-like inhibitory protein (C-FLIP): a novel target for cancer therapy. Curr Cancer Drug Targets 2008; 8:37-46; PMID:18288942; http://dx.doi.org/10.2174/156800908783497087
- Safa AR, Pollok KE. Targeting the anti-apoptotic protein c-FLIP for cancer therapy. Cancers (Basel) 2011; 3:1639-71; PMID:22348197; http://dx.doi.org/10.3390/cancers3021639
- Safa AR. c-FLIP, a master anti-apoptotic regulator. Exp Oncol 2012; 34:176-84; PMID:23070002
- Villa-Morales M, Fernández-Piqueras J. Targeting the Fas/FasL signaling pathway in cancer therapy. Expert Opin Ther Targets 2012; 16:85-101; PMID:22239437; http://dx.doi.org/10.1517/14728222.2011.628937
- Wang Y, Su M, Zhou LL, Tu P, Zhang X, Jiang X, Zhou Y. Deficiency of SATB1 expression in Sezary cells causes apoptosis resistance by regulating FasL/CD95L transcription. Blood 2011; 117:3826-35; PMID:21270445; http://dx.doi.org/10.1182/blood-2010-07-294819
- Wu J, Nihal M, Siddiqui J, Vonderheid EC, Wood GS. Low FAS/CD95 expression by CTCL correlates with reduced sensitivity to apoptosis that can be restored by FAS upregulation. J Investigat Dermatol 2009; 129:1165-73; PMID:18923451; http://dx.doi.org/10.1038/jid.2008.309
- Zhang CL, Kamarashev J, Qin JZ, Burg G, Dummer R, Dobbeling U. Expression of apoptosis regulators in cutaneous T-cell lymphoma (CTCL) cells. J Pathol 2003; 200:249-54; PMID:12754746; http://dx.doi.org/10.1002/path.1341
- Nevala H, Karenko L, Vakeva L, Ranki A. Proapoptotic and antiapoptotic markers in cutaneous T-cell lymphoma skin infiltrates and lymphomatoid papulosis. Brit J Dermatol 2001; 145:928-37; PMID:11899146; http://dx.doi.org/10.1046/j.1365-2133.2001.04523.x
- Choi J, Goh G, Walradt T, Hong BS, Bunick CG, Chen K, Bjornson RD, Maman Y, Wang T, Tordoff J, et al. Genomic landscape of cutaneous T cell lymphoma. Nat Genet 2015; 47:1011-9; PMID:26192916; http://dx.doi.org/10.1038/ng.3356
- da Silva Almeida AC, Abate F, Khiabanian H, Martinez-Escala E, Guitart J, Tensen CP, Vermeer MH, Rabadan R, Ferrando A, Palomero T. The mutational landscape of cutaneous T cell lymphoma and Sézary syndrome. Nat Genet 2015; 47:1465-70; PMID:26551667; http://dx.doi.org/10.1038/ng.3442
- McGirt LY, Jia P, Baerenwald DA, Duszynski RJ, Dahlman KB, Zic JA, Zwerner JP, Hucks D, Dave U, Zhao Z, et al. Whole-genome sequencing reveals oncogenic mutations in mycosis fungoides. Blood 2015; 126:508-19; PMID:26082451; http://dx.doi.org/10.1182/blood-2014-11-611194
- Wang L, Ni X, Covington KR, Yang BY, Shiu J, Zhang X, Xi L, Meng Q, Langridge T, Drummond J, et al. Genomic profiling of Sézary syndrome identifies alterations of key T cell signaling and differentiation genes. Nat Genet 2015; 47:1426-34; PMID:26551670; http://dx.doi.org/10.1038/ng.3444
- Kiel MJ, Sahasrabuddhe AA, Rolland DC, Velusamy T, Chung F, Schaller M, Bailey NG, Betz BL, Miranda RN, Porcu P, et al. Genomic analyses reveal recurrent mutations in epigenetic modifiers and the JAK-STAT pathway in Sézary syndrome. Nat Commun 2015; 6:8470; PMID:26415585; http://dx.doi.org/10.1038/ncomms9470
- Ungewickell A, Bhaduri A, Rios E, Reuter J, Lee CS, Mah A, Zehnder A, Ohgami R, Kulkarni S, Armstrong R, et al. Genomic analysis of mycosis fungoides and Sézary syndrome identifies recurrent alterations in TNFR2. Nat Genet 2015; 47:1056-60; PMID:26258847; http://dx.doi.org/10.1038/ng.3370
- de Silva S, Wang F, Hake TS, Porcu P, Wong HK, Wu L. Downregulation of SAMHD1 expression correlates with promoter DNA methylation in Sezary syndrome patients. J Investigat Dermatol 2014; 134:562-5; PMID:23884314; http://dx.doi.org/10.1038/jid.2013.311
- de Silva S, Hoy H, Hake TS, Wong HK, Porcu P, Wu L. Promoter methylation regulates SAMHD1 gene expression in human CD4+ T cells. J Biol Chem 2013; 288:9284-92; PMID:23426363; http://dx.doi.org/10.1074/jbc.M112.447201
- Cribier A, Descours B, Valadao AL, Laguette N, Benkirane M. Phosphorylation of SAMHD1 by cyclin A2/CDK1 regulates its restriction activity toward HIV-1. Cell Rep 2013; 3:1036-43; PMID:23602554; http://dx.doi.org/10.1016/j.celrep.2013.03.017
- Pauls E, Ruiz A, Badia R, Permanyer M, Gubern A, Riveira-Munoz E, Torres-Torronteras J, Alvarez M, Mothe B, Brander C, et al. Cell cycle control and HIV-1 susceptibility are linked by CDK6-dependent CDK2 phosphorylation of SAMHD1 in myeloid and lymphoid cells. J Immunol 2014; 193:1988-97; PMID:25015816; http://dx.doi.org/10.4049/jimmunol.1400873
- St Gelais C, de Silva S, Hach JC, White TE, Diaz-Griffero F, Yount JS, Wu L. Identification of cellular proteins interacting with the retroviral restriction factor SAMHD1. J Virol 2014; 88:5834-44; PMID:24623419; http://dx.doi.org/10.1128/JVI.00155-14
- Kajstura M, Halicka HD, Pryjma J, Darzynkiewicz Z. Discontinuous fragmentation of nuclear DNA during apoptosis revealed by discrete “sub-G1” peaks on DNA content histograms. Cytometry Part A: J Int Soc Anal Cytol 2007; 71:125-31; PMID:17252584; http://dx.doi.org/10.1002/cyto.a.20357
- Kaufmann SH, Desnoyers S, Ottaviano Y, Davidson NE, Poirier GG. Specific proteolytic cleavage of poly(ADP-ribose) polymerase: an early marker of chemotherapy-induced apoptosis. Cancer Res 1993; 53:3976-85; PMID:8358726
- Kaufmann SH. Induction of endonucleolytic DNA cleavage in human acute myelogenous leukemia cells by etoposide, camptothecin, and other cytotoxic anticancer drugs: a cautionary note. Cancer Res 1989; 49:5870-8; PMID:2790800
- Park SJ, Kim YY, Ju JW, Han BG, Park SI, Park BJ. Alternative splicing variants of c-FLIP transduce the differential signal through the Raf or TRAF2 in TNF-induced cell proliferation. Biochem Biophys Res Commun 2001; 289:1205-10; PMID:11741321; http://dx.doi.org/10.1006/bbrc.2001.6086
- Behrendt R, Schumann T, Gerbaulet A, Nguyen LA, Schubert N, Alexopoulou D, Berka U, Lienenklaus S, Peschke K, Gibbert K, et al. Mouse SAMHD1 has antiretroviral activity and suppresses a spontaneous cell-intrinsic antiviral response. Cell Rep 2013; 4:689-96; PMID:23972988; http://dx.doi.org/10.1016/j.celrep.2013.07.037
- Rehwinkel J, Maelfait J, Bridgeman A, Rigby R, Hayward B, Liberatore RA, Bieniasz PD, Towers GJ, Moita LF, Crow YJ, et al. SAMHD1-dependent retroviral control and escape in mice. EMBO J 2013; 32:2454-62; PMID:23872947; http://dx.doi.org/10.1038/emboj.2013.163
- Sze A, Belgnaoui SM, Olagnier D, Lin R, Hiscott J, van Grevenynghe J. Host restriction factor SAMHD1 limits human T cell leukemia virus type 1 infection of monocytes via STING-mediated apoptosis. Cell Host Microbe 2013; 14:422-34; PMID:24139400; http://dx.doi.org/10.1016/j.chom.2013.09.009
- Chang DW, Xing Z, Pan Y, Algeciras-Schimnich A, Barnhart BC, Yaish-Ohad S, Peter ME, Yang X. c-FLIP(L) is a dual function regulator for caspase-8 activation and CD95-mediated apoptosis. EMBO J 2002; 21:3704-14; PMID:12110583; http://dx.doi.org/10.1093/emboj/cdf356
- Micheau O, Thome M, Schneider P, Holler N, Tschopp J, Nicholson DW, Briand C, Grutter MG. The long form of FLIP is an activator of caspase-8 at the Fas death-inducing signaling complex. J Biol Chem 2002; 277:45162-71; PMID:12215447; http://dx.doi.org/10.1074/jbc.M206882200
- Pop C, Oberst A, Drag M, Van Raam BJ, Riedl SJ, Green DR, Salvesen GS. FLIP(L) induces caspase 8 activity in the absence of interdomain caspase 8 cleavage and alters substrate specificity. Biochem J 2011; 433:447-57; PMID:21235526; http://dx.doi.org/10.1042/BJ20101738
- Dummer R, Michie SA, Kell D, Gould JW, Haeffner AC, Smoller BR, Warnke RA, Wood GS. Expression of bcl-2 protein and Ki-67 nuclear proliferation antigen in benign and malignant cutaneous T-cell infiltrates. J Cutaneous Pathol 1995; 22:11-7; PMID:7751472; http://dx.doi.org/10.1111/j.1600-0560.1995.tb00733.x
- Wang F, St Gelais C, de Silva S, Zhang H, Geng Y, Shepard C, Kim B, Yount JS, Wu L. Phosphorylation of mouse SAMHD1 regulates its restriction of human immunodeficiency virus type 1 infection, but not murine leukemia virus infection. Virology 2016; 487:273-84; PMID:26580513; http://dx.doi.org/10.1016/j.virol.2015.10.024