ABSTRACT
Unlike somatic cells mitosis, germ cell meiosis consists of 2 consecutive rounds of division that segregate homologous chromosomes and sister chromatids, respectively. The meiotic oocyte is characterized by an absence of centrioles and asymmetric division. Centriolin is a relatively novel centriolar protein that functions in mitotic cell cycle progression and cytokinesis. Here, we explored the function of centriolin in meiosis and showed that it is localized to meiotic spindles and concentrated at the spindle poles and midbody during oocyte meiotic maturation. Unexpectedly, knockdown of centriolin in oocytes with either siRNA or Morpholino micro-injection, did not affect meiotic spindle organization, cell cycle progression, or cytokinesis (as indicated by polar body emission), but led to a failure of peripheral meiotic spindle migration, large polar body emission, and 2-cell like oocytes. These data suggest that, unlike in mitotic cells, the centriolar protein centriolin does not regulate cytokinesis, but plays an important role in regulating asymmetric division of meiotic oocytes.
Introduction
Unlike mitosis, meiosis consists of 2 consecutive rounds of chromosome separation with a single round of DNA replication. Errors in chromosome separation leads to the production of aneuploid eggs, which is believed to be the main cause of infertility, abortion and many genetic diseases in humans.Citation1 Oocyte meiotic maturation is characterized by asymmetric division, producing a small polar body and a large mature egg containing most of the ooplasm. Chromosome segregation is driven by the spindle microtubules. For accurate segregation, spindle organization and chromosome separation must be coordinated. Meiotic spindles in mammalian oocytes lack typical centrosomes (and centrioles are absent), and spindle assembly is executed by microtubule organizing centers (MTOCs), which are the functional equivalents of the centrosomes in mitosis. In the mouse oocyte, spindle poles are formed by the asters, or cytasters, which assemble in the second metaphase (MII) oocyte ooplasm at first, and then aggregate at MII spindle poles.Citation2 These cytasters were first described several years agoCitation3 and are seen particularly in rodent species.Citation4 These differences in the reproduction system among different species have been the topic of debate with regard to the terminology of MTOCs versus centrosomes. A consensus on the definition and terminology is still lacking.Citation2 Previous studies have shown that at prophase in mouse oocyte meiosis, over 80% of the MTOCs develop from a cytoplasmic microtubule network. These MTOCs contain the major acentriolar centrosome materials including γ-tubulin and pericentrin, and have microtubule nucleation properties similar to those of centrosomes.Citation2 After germinal vesicle breakdown (GVBD), MTOCs cluster between chromosomes and promote microtubule nucleation to form a centrally located bipolar spindle. After the chromosomes align in the MI spindle, the spindle complex containing chromosome migrates peripherally to a subcortical location,Citation5 then extrude the first polar body. Many regulators of mitosis are expected to play similar roles in meiosis. However, only a few of them have been confirmed. Therefore, the detailed molecular mechanisms of spindle assembly, meiotic cell progression, and asymmetric division in mammalian meiotic oocytes have yet to be determined.
Centrosomes nucleate microtubules and contribute to mitotic spindle organization and function. The main component of the mitosis centrosome is the centrosome material, with centrioles being the central area.Citation6 Centriolin is a relatively novel human protein that localizes to the centriole and functions in mitotic cell cycle progression and cytokinesis. It was shown that centriolin/CEP110 is localized to a specific domain of the centrosome, and both the reformation of interphase centrosomes following mitosis and function of MTOCs require centriolin.,Citation7,8 Depletion of centriolin by small interfering RNA (siRNA) silencing, overexpression, or antibody inhibition leads to G1/G0 arrest and cytokinesis errors.Citation9 Centriolin silencing results in cleavage failure, and the cytoplasm of the daughter cells is still interconnected. The centriolin domain is homologous to Nud1p/Cdc11 and it binds to Bub2p, which is required in cytokinesis.Citation9 Mis-oriented spindles were also found to be associated with disrupted astral microtubules and the loss of a unique set of centrosome proteins from the spindle poles, including ninein, Cep215, and centriolin.Citation10
This study aimed to clarify whether centriolin, as a centriole-appendage protein, regulates the progression of oocyte meiotic maturation, focusing on meiotic spindle organization, cytokinesis, and asymmetric division.
Materials and methods
Antibodies
Rabbit polyclonal anti-centriolin antibodies were purchased from Santa Cruz Biotechnology (Santa Cruz, CA). Rabbit monoclonal anti-α-tubulin antibodies were obtained from Cell Signaling Technology (Beverly, MA). A mouse monoclonal anti-α-tubulin antibody was purchased from Sigma (St. Louis, MO). A rabbit monoclonal GADPH antibody was obtained from Proteintech Group Inc. (Chicago, IL), and a mouse monoclonal anti-β-actin antibody was purchased from Santa Cruz Biotechnology.
Oocyte collection and culture
All animal experiments and handling were conducted according to the guidelines of the Animal Research Committee of Chungbuk National University and Institute of Zoology, Chinese Academy of Sciences. Oocytes with intact germinal vesicles were collected from the ovaries of 6–8-week old ICR mice, and were cultured in M16 medium (Sigma Chemical Co., St. Louis, MO) containing 2.5 mM milrinone under paraffin oil at 37°C and 5% CO2 in air. Oocytes were collected at different time points for microinjection, immunostaining, and polymerase chain reaction (PCR) analysis, and western blot analysis.
Nocodazole treatment of oocytes
For nocodazole treatment, oocytes were incubated in 10 μg/mL nocodazole (20 mg/mL nocodazole stock diluted in M2 medium) for 10 min, washed 3 times in M16, and then used for immunofluorescent staining and confocal microscopy. At least 30 oocytes were observed in 3 replicates.
Microinjection of centriolin siRNA and Morpholino
For knockdown experiments, about 5–10 pL of 60 μM centriolin siRNA was microinjected into the cytoplasm of a fully grown GV-arrested oocyte using a Nikon Diaphot ECLIPSE TE300 inverted microscope (Nikon UK Ltd., Kingston upon Thames, Surrey, UK) equipped with hydraulic 3-dimensional micromanipulators (MM0–202N; Narishige Inc., Sea Cliff, NY) in M16 medium containing 2.5 mM milrinone to inhibit GVBD. The siRNAs used in this study were chemically synthesized by Gene Pharma. The centriolin siRNA sequences were as follows:
Five′-CUGGACGAGAUACUUUGUATT-3′,
Five′-GUACCUUGGAACCAUCAAATT-3′,
and 5′-CUGCGAAAGAGCACUUGAATT-3′. Control oocytes were microinjected with 5–10 pL negative control siRNA and processed in parallel with the centriolin siRNA- injected group. Centriolin-specific Morpholino (Gene Tools) was also microinjected into the cytoplasm to deplete centriolin. The centriolin Morpholino sequences were as follows: centriolin Morpholino-1: GCCTTCGTTCAGAACCTTTCTTCAT; centriolin Morpholino-2: CTTTGCCCCTCTTCTGGGCTTTCAT. The same amount of negative control Morpholino (MO) was used as a control. After microinjection of 1 mM MO, the oocytes were cultured in fresh M16 medium containing 2.5 mM milrinone under paraffin oil at 37°C in an atmosphere of containing 5% CO2 in air for 24 h. Then, the oocytes were washed 5 times in fresh M16 medium for 2 min each. Spindle phenotypes were examined by confocal microscopy. Polar body extrusion was observed with a stereo microscope.
PCR
Total RNA was extracted from 50 oocytes using the Dynabead mRNA DIRECT kit (Invitrogen Dynal AS, Norway), and first strand cDNA was generated with a cDNA synthesis kit (Takara); using Oligo (dT) 12–18 primers (Invitrogen). Centriolin cDNA was amplified using the following primers: forward: 5′-ATT GAG GGC CTG GAG AGG AT-3′; and reverse: 5′-GCT TCT GCT CCA GGA GGT TT-3′. Reverse transcriptase (RT)-PCR products were evaluated by electrophoresis on a 2% agarose gel. The quantitative RT-PCR (qRT-PCR) conditions were as follows: 94°C for 10 min, followed by 39 cycles of 95°C for 15 s, 60°C for 15 s, and 72°C for 45 s, and a final extension of 72°C for 5 min.
Immunofluorescence analysis and confocal microscopy
Oocytes were fixed with 4% paraformaldehyde in phosphate-buffered saline (PBS) for 30 min at room temperature. After permeabilization with 0.5% Triton X-100 for 20 min, the oocytes were then blocked with 1% bovine serum albumin in PBS for 1 h at room temperature. For single staining of α-tubulin, oocytes were incubated with anti-α-tubulin-fluorescein isothiocyanate antibodies (1:500) over night at 4°C, and DNA was stained with Hoechst 33342 for 15 min. Oocytes were mounted onto glass slides and examined using a laser scanning confocal microscope (LSM 710 META; Zeiss, Oberkochen, Germany). At least 30 oocytes were observed in 3 replicates.
Immunoblotting analysis
For the immunoblotting analysis, 180 mouse oocytes were collected in 7 μL of 2× sodium dodecyl sulfate (SDS) buffer. The proteins were separated by SDS- polyacrylamide gel electrophoresis and then transferred to polyvinylidene difluoride membranes. After transfer, the membranes were blocked in Tris-buffered saline with Tween 20 (TBST) containing 5% skimmed milk for 2 h at room temperature, and then incubated overnight at 4°C with a rabbit polyclonal anti-centriolin antibody (1:200) and mouse monoclonal anti-β-actin antibody (1:1,000) over night at 4°C. After 3 washes in TBST buffer (10 minutes each) the membranes were incubated with horseradish peroxidase-conjugated goat anti-mouse IgG (1:1,000), for 1 h at 37°C. Finally, the membranes were processed using the enhanced chemiluminescence-detection system (Bio-Rad, CA).
Statistical analysis
Data (mean ± standard error of mean [SEM]) were generated from replicate experiments that were repeated at least 3 times analyzed by ANOVA using SPSS software (SPSS Inc., Chicago, IL) followed by the Student-Newman-Keuls test. Spindle morphology and distance to membrane was analyzed with SPSS software. All images were rendered 3-dimensional to determine the maximum length of the spindle. Differences with p-values <0.05 were considered statistically significant, and are indicated in figures with different superscript letters.
Results
Localization of centriolin during mouse oocyte meiotic maturation
Centriolin was expressed in oocytes at various stages. Analysis of oocytes at the GV stage showed that it was distributed in both the cytoplasm and nucleus, although more condensed staining was observed in the GV. After GVBD, centriolin was localized to the meiotic spindle, and was more concentrated at the spindle poles. At telophase and before polar body emission, strong staining was observed on the midbody. In MII mature oocytes, centriolin was distributed at the spindle, with condensed staining on the poles. At metaphase of meiosis I and II, sporadic dots of centriolin were observed in the cytoplasm (). When MI and MII oocytes were treated with nocodazole to disrupt spindles, centriolin was no longer localized to the spindles or spindle poles ().
Figure 1. Expression and localization of centriolin during mouse oocyte meiotic maturation. A. mRNA expression of centriolin during oocyte meiotic maturation. Each sample contained 50 oocytes. B. mRNA levels for centriolin during mouse oocyte meiosis. The mRNA levels of centriolin at the Pro-MI, MI, and MII stages were 117 ± 33%, 107 ± 18%, and 314 ± 67%, respectively. C. Centriolin localization during oocyte meiotic maturation. During meiosis I, it was distributed on the spindles, and concentrated on the spindle poles at metaphase, but then relocated to the midbody at telophase. Green, centriolin; red: DNA. Bar = 20 μm.
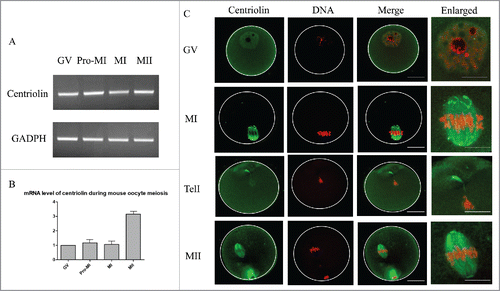
Figure 2. Distribution of centriolin after treatment of metaphase oocytes with nocodazole. After disruption of the meiotic spindle, centriolin disappeared from the spindle and spindle poles. At least 30 oocytes were observed in 3 replicates. Red, centriolin; green, microtubules; blue, DNA. Bar = 20 μm.
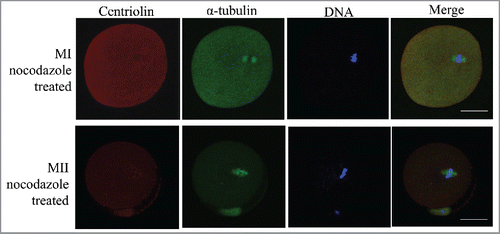
Centriolin depletion by siRNA does not affect first polar body extrusion, but disrupts asymmetric division of mouse oocytes
To explore the functional role of centriolin during oocyte meiotic maturation, we used centriolin MO micro-injection. As shown in and , western blotting and densitometry analysis showed that the centriolin expression was reduced after injection with the centriolin MO (100% vs. [vs.] 37.39 ± 19.72%, p < 0.05). There was no significant difference in the polar body emission rate between the control MO and centriolin MO microinjection groups at 10, 11, and 12 h of culture (33.5 ± 3.5% vs. 37.92 ± 7.68%; 54.5 ± 5.50% vs. 56.67 ± 7.13%; and 74.5 ± 4.15% vs. 66.16 ± 7.95% respectively) (). A unique phenotype evident after centriolin depletion was the failure of asymmetric division in a high proportion of oocytes. As shown in , after 12 h of culture, numerous centriolin MO-injected oocytes underwent symmetric division. The rate of symmetric divisions in the centriolin MO-injected group (22.33 ± 8.39%) was significantly higher than that of the control MO group (4.25 ± 5.90%, p < 0.05) ().
Figure 3. Knock-down of centriolin by Morpholino (MO) microinjection did not affect meiotic oocyte maturation process, but disrupted asymmetric division. A, B: Centriolin was effectively knocked down after microinjection a centriolin-specific MO. A total of 200 oocytes were used in each group. C: Depletion of centriolin did not affect completion of meiosis I. The polar body emission rate in the centriolin MO-injected group was the same as that in the control MO group after 10 -12 h of culture. A total of 166 oocytes were assessed in the MO group and 154 oocytes were assessed in the control group. D, E: A significantly higher proportion of oocytes underwent symmetric division after depletion of centriolin (p < 0.05).
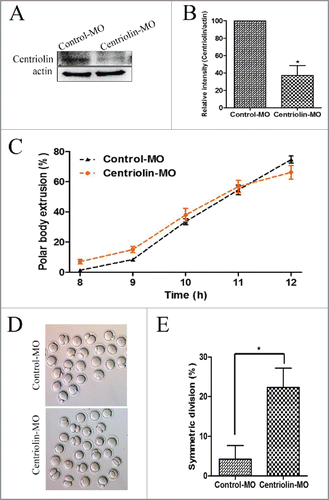
To further confirm this phenotype, we also micro -injected centriolin siRNA into the GV oocytes. As shown in , centriolin was successfully knocked down after the centriolin siRNA microinjection, but meiotic progression and oocyte maturation, as indicated by polar body emission were not affected. However, in the centriolin siRNA group, the proportion of oocytes with a large polar body, or 2-cell like oocytes was significantly higher than that of control group. In the big polar bodies and equal-sized cells, normal spindles were formed.
Figure 4. Knock-down of centriolin by siRNA microinjection did not affect meiotic oocyte maturation process, but led to the production of equal-sized cells or a large polar body with normal MII spindles. A, B: Effective depletion of centriolin after microinjection of siRNA into the oocyte. A total of 200 oocytes were used in centriolin siRNA-injected group, and a total of 200 oocytes were used in the control group. C: Similar percentages of mature oocytes were observed in siRNA group and control siRNA groups. At least 100 oocytes were assessed in each replicate. D. Big polar rate and symmetric division rate after centriolin siRNA knock-down. E. Centriolin knock-down produce equal-sized cells or a large polar body with normal MII spindles.
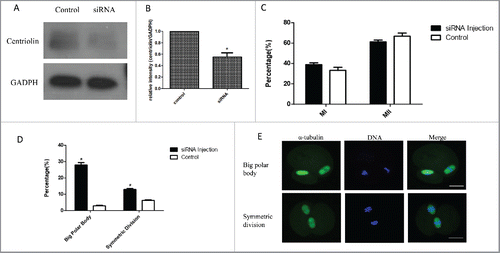
These results demonstrated that downregulation of centriolin did not affect meiotic cell cycle progression or polar body emission, but disrupted asymmetric division of oocytes.
Centriolin depletion affects spindle size and peripheral spindle migration in oocytes
Considering the above-mentioned phenotype of large polar bodies and symmetric divisions, we analyzed the spindle migration and cell polarization during Meiosis I (MI). In the control group, the spindle of most oocytes moved to the cortex at late MI after 9.5 h of culture (). In contrast, some centriolin MO-injected oocytes displayed centrally located spindles. As shown in , the distance between the spindle and the plasma membrane in the centriolin MO-injected group was significantly greater than that in the control MO-injected groups (18.52 ± 1.62 μM vs. 26.17 ± 2.62 μM, p < 0.05). The length of the MI spindles in the centriolin MO-injected groups was significantly longer than in the corresponding control MO-injected groups (27.30 ± 2.83 μM vs. 22.10 ± 2.13 μM, p < 0.05) (). Centriolin-depleted oocytes via siRNA microinjection also showed centrally localized MI spindles (), and these oocytes did not establish polarity, as indicated by the lack of an actin cap ().
Figure 5. Centriolin depletion by Morpholino (MO) injection affects spindle size and peripheral spindle migration in oocytes. A: In control oocytes, most spindles moved to the cortex at late MI, whereas in centriolin MO-injected oocytes, spindles were centrally located. Bar = 20 μm B: The distance between the spindle and plasma membrane in the control MO-injected group was significantly shorter than that in the centriolin MO-injected group (p < 0.05). C: The length of the MI spindles in the centriolin MO-injected group was significantly longer than that of spindles in the control MO-injected group.
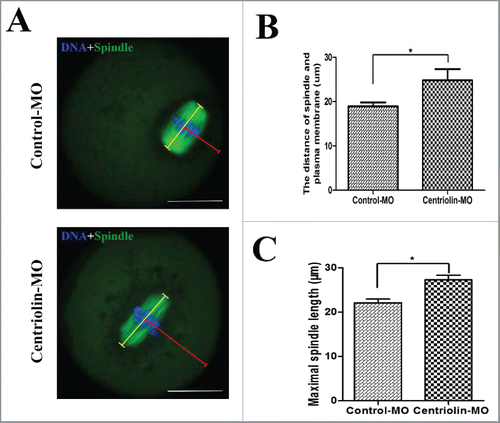
Figure 6. Centriolin siRNA microinjection inhibited peripheral spindle localization and polarity establishment in oocytes. A: Centrally localized MI spindles in centriolin depleted oocytes. At least 100 oocytes were assessed in each replicate. B: Centriolin siRNA microinjection led to a failure of polarity establishment, as indicated by the loss of an actin-cap. At least 50 oocytes were assessed in each replicate. Green, spindle; blue, DNA; red, actin. Bar = 20 μm.
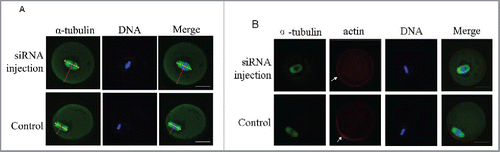
Subsequently, we examined spindle location and morphology in MII oocytes. As shown in , compared with control oocytes, the centriolin MO-micro-injected oocytes, showed elongated spindles as the major defects. The length of the abnormal spindles in the centriolin MO-injected group was significantly longer than that in the corresponding control MO-injected group (33.88 ± 4.47 μM vs. 22.77 ± 1.94 μM, p < 0.05) ().
Discussion
Oocyte meiosis differs from somatic cell mitosis in several ways: 1) Oocytes do not have typical centrosomes and the meiotic spindle is organized by MTOCs in the absence of centrioles; and 2) oocytes undergo 2 rounds of asymmetric divisions, producing small polar bodies and a large egg. In the present study, using siRNA and MO microinjection, we showed that a relatively new centriole component, centriolin, does not regulate meiotic cell cycle progression and cytokinesis (in contrast to its role in mitosis), but is important for peripheral spindle migration and asymmetric division in mouse oocytes, providing evidence that this protein may play different roles in somatic cell mitosis and oocyte meiosis.
Mammalian oocytes are arrested at the GV stage for months or years depending on the species.Citation11 After puberty, a limited number of fully-grown oocytes resume meiosis, as indicated by GVBD, and then, the meiotic spindle is organized. During oogenesis, an oocyte loses its centrioles, and the meiotic spindle is organized in the absence of typical centrosomes.Citation12 In mouse oocytes, meiotic spindles are organized by the assembly of numerous small asters that gather at the acentriolar spindle poles.Citation2 It is well known that centrosome components such as γ-tubulin, pericentrin, and the nuclear mitotic apparatus (NuMA) protein participate in meiotic spindle organization in oocytes.Citation2 Until now, we had only fragmented knowledge about centrosome functions in oocytes. In the present study, we showed that although centriolin was concentrated at the spindle poles at metaphase I and II, it was not involved in meiotic spindle organization or spindle pole formation, since normal spindles were organized after its depletion by siRNA or MO injection.
Centriolin is localized to the centrosomes in a wide variety of species. At the metaphase to anaphase transition, centriolin staining at the centrosomes diminished, but then appeared as 1 or 2 dots adjacent to the intercellular bridge during cytokinesis. Centriolin silencing induced cytokinesis failure, suggesting its important role in late cytokinesis in vertebrates.Citation9 Centriolin interacts with the proteins of vesicle-targeting exocyst and vesicle-fusion SNARE complexes to regulate the terminal step of cytokinesis, called abscission.Citation13 In our study, we found that centrosomal centriolin relocates from the spindle poles to the midbody during the metaphase/telophase transition in meiotic oocytes. Unexpectedly, knockdown of centriolin with either siRNA or MO did not inhibit cytokinesis, and polar bodies were extruded even after the protein was depleted. Thus, unlike in mitosis, centriolin is not a key player during cytokinesis in meiotic oocytes.
Two asymmetric divisions take place in female meiosis to generate a haploid gamete. Although knockdown of centriolin did not affect cytokinesis or polar body emission, we found that a large proportion of oocytes underwent symmetric divisions or extruded a large polar body. After mouse oocyte GVBD, microtubules assemble to form a centrally located meiotic spindle that then migrates to the oocyte cortex where it is anchored by an actin filament cap.Citation14 Oocyte asymmetric spindle migration and first polar body extrusion are driven by actin filaments.Citation15,16 Actin-binding proteins (ABPs), such as Arp2/3 complex or formin-2, initiate actin filament formation, which means they play a key role in oocyte maturation.Citation17 The roles of microfilaments and/or microtubules in spindle migration and anchorage to the oocyte cortex have been reported for various invertebrate and mammalian oocytes,Citation2 and several factors have recently been shown to regulate oocyte polarity and asymmetric division.Citation18-21 However, the role of centrosomes in this process is still not well understood. We showed that depletion of centriolin caused a failure of peripheral meiotic spindle migration and polarity establishment in a high proportion of oocytes, as well as elongated central spindles, leading to symmetric divisions, as indicated by the production of 2 same-sized cells or the formation of a large polar body. It was recently reported that centriolin depletion displaces the centrosomal componentsCitation22 and misoriented spindles are associated with loss of a unique set of centrosome proteins (ninein, Cep215, and centriolin) from the spindle poles.Citation10 However, how depletion of centriolin in meiotic oocytes leads to failure of asymmetric division requires further study.
In summary, unlike in mitotic cells, the centriole appendage protein, centriolin does not play a critical role in the meiotic cell cycle and the regulation of cytokinesis. However, it is a host regulatory component involved in the establishment of polarity and asymmetric division in meiotic oocytes.
Abbreviations
GV | = | germinal vesicle |
GVBD | = | germinal vesicle breakdown |
MI | = | first metaphase |
MII | = | second metaphase |
MTOCs | = | microtubule organizing centers |
Disclosure of potential conflicts of interest
No potential conflicts of interest were disclosed.
Acknowledgments
The authors thank all members in Dr. Kim NH's laboratory and Zhang T. and Qi ST from Institute of Zoology, Chinese Academy of Sciences for thier technical help and discussions.
Funding
This study was supported by the Next-Generation BioGreen21 Program (PJ011126), of the Rural Development Administration (RDA), Republic of Korea
References
- Qiao J, Wang ZB, Feng HL, Miao YL, Wang Q, Yu Y, Wei YC, Yan J, Wang WH, Shen W, et al. The root of reduced fertility in aged women and possible therapentic options: current status and future perspects. Mol Aspects Med 2014; 38:54-85; PMID:23796757; http://dx.doi.org/10.1016/j.mam.2013.06.001
- Schatten H, Sun QY. Centrosome dynamics during mammalian oocyte maturation with a focus on meiotic spindle formation. Mol Reprod Dev 2011; 78(10–11):757-68; PMID:21887720; http://dx.doi.org/10.1002/mrd.21380
- Maro B, Howlett SK, Webb M. Non-spindle microtubule organizing centers in metaphase II-arrested mouse oocytes. J Cell Biol 1985; 101(5 Pt 1):1665-72; PMID:2865266; http://dx.doi.org/10.1083/jcb.101.5.1665
- Schatten G, Simerly C, Schatten H. Maternal inheritance of centrosomes in mammals? Studies on parthenogenesis and polyspermy in mice. Proc Natl Acad Sci USA 1991; 88(15):6785-9; PMID:1862101; http://dx.doi.org/10.1073/pnas.88.15.6785
- Deng M, Suraneni P, Schultz RM, Li R. The Ran GTPase mediates chromatin signaling to control cortical polarity during polar body extrusion in mouse oocytes. Dev Cell 2007; 12(2):301-8; PMID:17276346; http://dx.doi.org/10.1016/j.devcel.2006.11.008
- Bobinnec Y, Khodjakov A, Mir LM, Rieder CL, Eddé B, Bornens M. Centriole disassembly in vivo and its effect on centrosome structure and function in vertebrate cells. J Cell Biol 1998; 143(6):1575-89; PMID:9852152; http://dx.doi.org/10.1083/jcb.143.6.1575
- Ou YY, Mack GJ, Zhang M, Rattner JB. CEP110 and ninein are located in a specific domain of the centrosome associated with centrosome maturation. J Cell Sci 2002; 115(Pt 9):1825-35 PMID: 11956314
- Szebenyi G, Hall B, Yu R, Hashim AI, Krämer H. Hook2 localizes to the centrosome, binds directly to centriolin/CEP110 and contributes to centrosomal function. Traffic 2007; 8(1):32-46; PMID:17140400; http://dx.doi.org/10.1111/j.1600-0854.2006.00511.x
- Gromley A, Jurczyk A, Sillibourne J, Halilovic E, Mogensen M, Groisman I, Blomberg M, Doxsey S. A novel human protein of the maternal centriole is required for the final stages of cytokinesis and entry into S phase. J Cell Biol 2003; 161(3):535-45; PMID:12732615; http://dx.doi.org/10.1083/jcb.200301105
- Chen CT, Hehnly H, Yu Q, Farkas D, Zheng G, Redick SD, Hung HF, Samtani R, Jurczyk A, Akbarian S, et al. A unique set of centrosome proteins requires pericentrin for spindle-pole localization and spindle orientation. Curr Biol 2014; 24(19):2327-34; PMID:25220058; http://dx.doi.org/10.1016/j.cub.2014.08.029
- Zhang T, Zhang GL, Ma JY, Qi ST, Wang ZB, Wang ZW, Luo YB, Jiang ZZ, Schatten H, Sun QY. Effects of DNA damage and short-term spindle disruption on oocyte meiotic maturation. Cell Biol 2014; 142(2):185-94.
- Luksza M, Queguigner I, Verlhac MH, Brunet S. Rebuilding MTOCs upon centriole loss during mouse oogenesis. Dev Biol 2013; 382(1):48-56; PMID:23954884; http://dx.doi.org/10.1016/j.ydbio.2013.07.029
- Gromley A, Yeaman C, Rosa J, Redick S, Chen CT, Mirabelle S, Guha M, Sillibourne J, Doxsey SJ. Centriolin anchoring of exocyst and SNARE complexes at the midbody is required for secretory-vesicle-mediated abscission. Cell 2005; 123(1):75-87; PMID:16213214; http://dx.doi.org/10.1016/j.cell.2005.07.027
- Ai JS, Wang Q, Yin S, Shi LH, Xiong B, OuYang YC, Hou Y, Chen DY, Schatten H, Sun QY. Regulation of peripheral spindle movement and spindle rotation during mouse oocyte meiosis: new perspectives. Microsc Microanal 2008; 14(4):349-56; PMID:18598570; http://dx.doi.org/10.1017/S1431927608080343
- Almonacid M, Terret MÉ, Verlhac MH. Actin-based spindle positioning: new insights from female gametes. J Cell Sci 2014; 127(Pt 3):477-83; PMID:24413163; http://dx.doi.org/10.1242/jcs.142711
- Yi K, Li R. Actin cytoskeleton in cell polarity and asymmetric division during mouse oocyte maturation. Cytoskeleton (Hoboken) 2012; 69(10):727-37; PMID:22753278; http://dx.doi.org/10.1002/cm.21048
- Jo YJ, Jang WI, Kim NH, Namgoong S. Tropomodulin-3 is essential in asymmetric division during mouse oocyte maturation. Sci Rep 2016; 6:29204; PMID:27374327; http://dx.doi.org/10.1038/srep29204
- Lee SE, Sun SC, Choi HY, Uhm SJ, Kim NH. mTOR is required for asymmetric division through small GTPases in mouse oocytes. Mol Reprod Dev 2012; 79(5):356-66; PMID:22407942; http://dx.doi.org/10.1002/mrd.22035
- Zhang Y, Duan X, Cao R, Liu HL, Cui XS, Kim NH, Rui R, Sun SC. Small GTPase RhoA regulates cytoskeleton dynamics during porcine oocyte maturation and early embryo development. Cell Cycle 2014; 13(21):3390-403; PMID:25485583; http://dx.doi.org/10.4161/15384101.2014.952967
- Guo L, Wang ZB, Wang HH, Zhang T, Qi ST, Ouyang YC, Hou Y, Sun QY. Nek11 regulates asymmetric cell division during mouse oocyte meiotic maturation. Biochem Biophys Res Commun 2016; 474(4):667-72; PMID:27150633; http://dx.doi.org/10.1016/j.bbrc.2016.05.002
- Namgoong S, Kim NH. Roles of actin binding proteins in mammalian oocyte maturation and beyond. Cell Cycle 2016; 15(14):1830-43; PMID:27152960; http://dx.doi.org/10.1080/15384101.2016.1181239
- Hehnly H, Chen CT, Powers CM, Liu HL, Doxsey S. The centrosome regulates the Rab11- dependent recycling endosome pathway at appendages of the mother centriole. Curr Biol 2012; 22(20):1944-50; PMID:22981775; http://dx.doi.org/10.1016/j.cub.2012.08.022