ABSTRACT
DNA replication is rigorously controlled in cells to ensure that the genome duplicates exactly once per cell cycle. Geminin is a small nucleoprotein, which prevents DNA rereplication by directly binding to and inhibiting the DNA replication licensing factor, Cdt1. In this study, we have identified 2 Geminin genes, BmGeminin1 and BmGeminn2, in silkworm, Bombyx mori. These genes contain the Geminin conserved coiled-coil domain and are periodically localized in the nucleus during the S-G2 phase but are degraded at anaphase in mitosis. Both BmGeminin1 and BmGeminin2 are able to homodimerize and interact with BmCdt1 in cells. In addition, BmGeminin1 and BmGeminin2 can interact with each other. Overexpression of BmGeminin1 affects cell cycle progression: cell cycle is arrested in S phase, and RNA interference of BmGeminin1 leads to rereplication. In contrast, overexpression or knockdown of BmGeminin2 with RNAi did not significantly affect cell cycle, while more rereplication occurred when BmGeminin1 and BmGeminin2 together were knocked down in cells than when only BmGeminin1 was knocked down. These data suggest that both BmGeminin1 and BmGeminin2 are involved in the regulation of DNA replication. These findings provide insight into the function of Geminin and contribute to our understanding of the regulation mechanism of cell cycle in silkworm.
Introduction
In metazoans, DNA replication is strictly regulated to ensure proper DNA duplication during cell cycle, as its aberration can lead to tumorigenesis or cell death.Citation1,2 Accordingly, initiation of DNA replication is limited to once per cell cycle, and is controlled by the stepwise assembly of a pre-replicative complex (pre-RC).Citation3-5 Cdt1 is an essential component for the assembly of pre-RC, which mediates the loading of mini-chromosome maintenance protein (MCM) onto chromatin that is the limiting step to prevent the reinititation of replication.Citation3,6-9 The formation of pre-RC is tightly regulated in all eukaryotes and its inappropriate assembly has been reported to lead to rereplication. An important regulatory step in preventing rereplication is that just after initiation of DNA replication Cdt1 should release from the DNA replication origins and be bound by Geminin.Citation3,10,11
Geminin is a small nucleoprotein, which regulates DNA replication by directly binding to and inhibiting the DNA replication licensing factor, Cdt1.Citation3,11,12 Geminin was initially identified in Xenopus laevis as a substrate of the anaphase-promoting-complex (APC) at the end of mitosisCitation10 and later was found to inhibit DNA replication by preventing the MCM complex from binding the chromatin.Citation11 Subsequently, Geminin has been found in various multicellular eukaryotes including Homo sapiens, Mus musculus, Drosophila melanogaster and Caenorhabditis elegans.Citation9,10,13-15 In X. laevis, 2 Geminin proteins, Geminin H and Geminin L, with high amino acid identities (89%) have been identified.Citation10 However, only one Geminin protein has been identified in other organisms. Geminin has a relatively complex structure, and contains a conserved coiled-coil central region at the carboxyl terminus. The coiled-coil domain mediates Geminin self-association and interactions with Cdt1. The active form of Geminin is a homodimer to which Cdt1 associates.Citation16,17 Mutations that disrupt the dimerization of Geminin or the interaction of Geminin with Cdt1 abolish the ability of Geminin to inhibit replication in Xenopus egg extracts and in mammalian cell lines.Citation16 Another important structure of Geminin is the destruction box (D-box) at its N-terminus, which is recognized by APC during mitosis for ubiquitin-mediated degradation during cell cycle.Citation10,18 Geminin has profound effects on cell proliferation. It binds and inhibits Cdt1 and eventually disrupts the reassembly of pre-RC in replication origins before DNA synthesis begins, thus preventing a second round of DNA replication during the S-M phase.Citation17 Depletion of Geminin causes rereplication, DNA damage, G2/M arrest,Citation19-21 and centrosome over-duplicationCitation22 in a cell type-specific manner.Citation12,23 Overexpression of Geminin is also cell type-specific, leading to arrest at the G1/S phase transition or a defective S-phase.Citation12,24 Geminin is also reported to be a regulator of development and differentiation because overexpression of the protein induced uncommitted ectodermal cells to differentiate into neurons in Xenopus embryos.Citation25
In this study, we discovered 2 Geminin homologs in silkworm, which is one of the most economically important lepidopterans.Citation26,27 Unlike most species that contain only one Geminin protein, we found 2 Geminin proteins in silkworm.Citation9,13-15 We found that BmGeminin1 and BmGeminin2 are nucleoproteins and are periodically distributed during the cell cycle. BmGeminin1 and BmGeminin2 can homodimerize and can interact with BmCdt1. Our results also showed that BmGeminin1 and BmGeminin2 are involved in DNA replication. Identification of Geminin homologs in silkworm and understanding their function in this study lays the foundation for further research on the regulation mechanism of cell cycle in silkworm.
Results
Identification of 2 Geminin homologs
We cloned and identified 2 genes containing the coiled-coil domain of Geminin. One of them was located on nscaf2828 in chromosome 8; we named this gene BmGeminin1, which encoded a protein with 170 amino acids (GenBank: AGB51149). The second homolog was located on nscaf1690 in chromosome 1; we named this as BmGeminin2, which encoded a protein with 161 amino acids (GenBank: AGB51150). Multiple sequence alignment of Geminin homologs from H. sapiens, M. musculus, X. laevis, B. mori, and D. melanogaster is shown in . Both BmGeminin1 and BmGeminin2 exhibited high amino acid identity with other homologs in their central coiled-coil region. Another region of high conservation between BmGeminin1 and other Geminin homologs was detected at the N-terminal region encompassing the D-box. However, BmGeminin2 lacked a D-box. Analysis of the phylogenetic tree showed that BmGeminin1, BmGeminin2 and Geminin from other species clustered into a separate group. Geminin-related proteins, Idas and GEMC1, formed separate groups (). These results suggested that BmGeminin1 and BmGeminin2 are homologs of Geminin.
Figure 1. Bioinformatics analysis of BmGeminin. (A) Multiple sequence alignment of Geminin from H. sapiens, M. musculus, X. laevis, B. mori, and D. melanogaster. Identical and similar residues are indicated in dark and light gray, respectively. Amaranthine column, conserved coiled-coil region; DNA replication domain are underlined in blue; Red box, D-box. (B) The phylogenetic tree of Geminin.
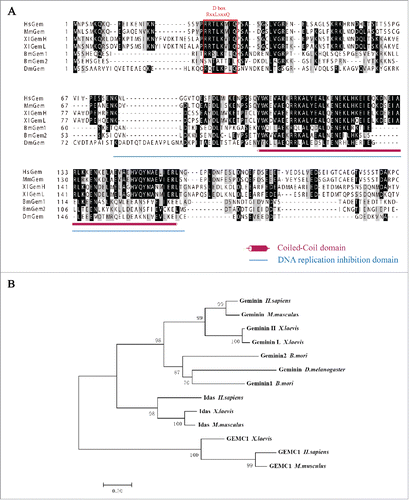
BmGeminin1 and BmGeminin2 are nuclear proteins periodically expressed during the cell cycle
The endogenous expression of BmGeminin1 and BmGeminin2 in BmN-SWU1 cells, an ovarian cell line from B. mori, was tested by Western blot analysis. Geminin antibodies recognized both BmGeminin1 and BmGeminin2. The results showed that both BmGeminin1 and BmGeminin2 were expressed in BmN-SWU1 cells (Figure S1). Moreover, the subcellular localization of BmGeminin1 and BmGeminin2 detected by immunofluorescence indicated the presence of endogenous BmGeminin1 and BmGeminin2 localized in the nuclei ().
Figure 2. The expression pattern of BmGeminin in BmN-SWU1 cells. (A) Immunofluorescence analysis of the location of endogenous BmGeminin in BmN-SWU1 cells by using anti-BmGeminin monoclonal antibodies. DNA was stained with DAPI. (B) Dual labeling of BmN-SWU1 cells with anti-BrdU and anti-BmGeminin antibodies. Red arrow indicates BmGeminin positive cells, green arrow indicates BrdU positive cells, and yellow arrow indicates cells positive for both BmGeminin and BrdU; Double-labeling of BmN-SWU1 cells with anti-tubulin and anti-BmGeminin antibodies. Cells are arranged by mitotic stage based on DAPI and tubulin staining. Metaphase cells are indicated with red arrows and labeled “m,” anaphase cells are indicated with green arrows and labeled “a,” and telophase cells are indicated with yellow arrows and labeled “t.” (C) Ratio of cells positive for anti-BrdU and anti-BmGeminin antibodies. Gem−/BrdU+ represents the proportion of BmGeminin negative cells in BrdU-positive cells; Gem+/BrdU+ represents the proportion of BmGeminin positive cells in BrdU-positive cells (D) Ratio of Geminin positive cells in metaphase, anaphase and telophase, respectively. (E) Western blot analysis if the expression of BmGeminin in BmN-SWU1 cells, which were synchronized in G1 phase with Aphidicolin, S phase with HU, and G2/M phase with Nocodazole. Cells in the control group were treated with DMSO. Tubulin protein was used as the loading control. (F) The periodical expression of BmGeminin in BmN-SWU1 cells.
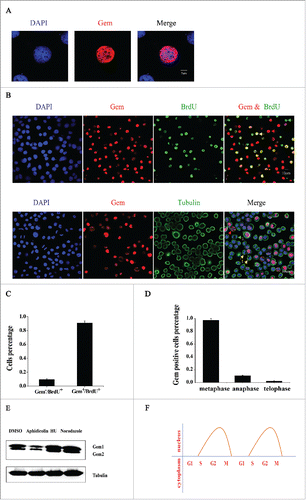
To explore the expression of BmGeminin (BmGeminin1 and BmGeminin2) throughout the cell cycle, BmN-SWU1 cells were first immunostained with anti-BmGeminin and anti-BrdU antibodies (). BrdU-labeling identifies cells in the S phase of the cell cycle. Counting BmGeminin or BrdU positive cells indicated that 9.3% of the BrdU-positive cells were BmGeminin negative (Gem−/BrdU+) and that 90.7% of the BrdU-positive cells were BmGeminin positive (Gem+/BrdU+) (). These results suggested that most cells in the S phase were BmGeminin positive and only a small number of these cells were BmGeminin negative. We also double-labeled the cells with tubulin and Geminin. shows arrangement of cells by mitotic stage based on DAPI and tubulin staining. The results showed that BmGeminin was present in metaphase but was mostly degraded in anaphase. Counting BmGeminin positive cells indicated that BmGeminin accumulated during prophase and metaphase and was degraded at anaphase (). Moreover, we also determined the endogenous expression of BmGeminin in G1, S, and G2/M cells synchronized using Aphidicolin, Hydroxyurea (HU) and Nocodazole, respectively (Figure S2). The results showed that the expression of BmGeminin was significantly reduced in G1 cells but significantly increased in S and G2/M cells compared with the control (). Therefore, the periodical expression of BmGeminin1 and BmGeminin2 in BmN-SWU1 cells were as expected (). As shown in this model, BmGeminin1 and BmGeminin2 were virtually absent in the G1 phase. BmGeminin1 and BmGeminin2 accumulated during S-G2 phase and were degraded at anaphase. In conclusion, the expression of BmGeminin1 and BmGeminin2 were periodic during the cell cycle.
The interactions between BmGeminin1, BmGeminin2 and BmCdt1
Immunofluorescence analysis of the localization of BmGeminin1, BmGeminin2 and BmCdt1 in cells showed co-localization of BmGeminin1 with BmCdt1, BmGeminin2 with BmCdt1, BmGeminin1 with self, BmGeminin2 with self, and BmGeminin1 with BmGeminin2 suggesting that interactions between the proteins may exist ( and ). The BiFC system analysis also indicated a likely interaction between BmGeminin1 and BmGeminin2 with BmCdt1, BmGeminin1 with self, BmGeminin2 with self, and BmGeminin1 with BmGeminin2 ( and ).
Figure 3. The interactions between BmGeminin1, BmGeminin2 and BmCdt1. (A) Immunofluorescence analysis of the co-localization of BmGeminin1 and BmGeminin2 with BmCdt1, respectively. DNA was stained with DAPI. (B) Immunofluorescence analysis of the co-localization of BmGeminin1 with self, BmGeminin1 with BmGeminin2, and BmGeminin2 with self. DNA was stained with DAPI. (C) BiFC system analysis of the interaction of BmGeminin1, BmGeminin2 and BmCdt1. BmGeminin1 or BmGeminin2 and BmCdt1 were co-transfected into BmN-SWU1 cells. Cellular nuclei were stained with DAPI. Auto-fluorescence signals were visualized using confocal microscopy. (D) BiFC system analysis of the interaction of BmGeminin1 with self, BmGeminin1 with BmGeminin2, BmGeminin2 with self. BmGeminin1 or BmGeminin2 were co-transfected into BmN-SWU1 cells. Cellular nuclei were stained with DAPI. Auto-fluorescence signals were visualized using confocal microscopy. (E) Co-immunoprecipitation (Co-IP) assays of the interaction of BmGeminin1 and BmGeminin2 with BmCdt1. Red arrow indicates the IgG heavy chain, blue arrow indicates the BmGeminin1 protein band, and purple arrow indicates the BmGeminin2 protein band. Input: cell lysates. IgG: IP with control mouse IgG. (F) Co-IP assays of BmGeminin1 and BmGeminin2 self-association or interaction with each other in cells. Red arrow indicates IgG heavy chain, blue arrow indicates the BmGeminin1 protein band, and purple arrow indicates the BmGeminin2 protein band. Input: cell lysates. IgG: IP with control mouse IgG.
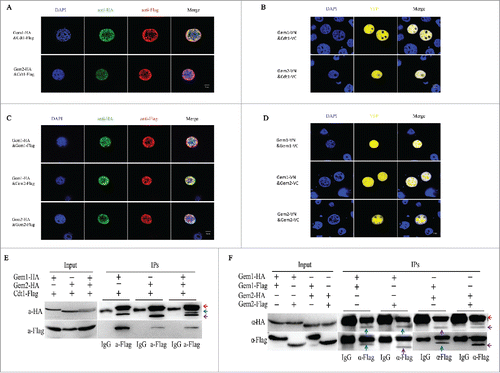
To further verify the ability of BmGeminin1 and BmGeminin2 to interact with BmCdt1, co-immunoprecipitation experiments were conducted. BmN-SWU1 cells were co-transfected with Gem1-HA or Gem2-HA and Cdt1-Flag recombinant plasmid followed by immunoprecipitation with anti-FLAG-tag monoclonal antibody. As shown in , both Gem1-HA and Gem2-HA were detected in the Cdt1-Flag immunoprecipitates. The results suggested that both BmGeminin1 and BmGeminin2 can interact with BmCdt1 in cells. In addition, when BmN-SWU1 cells were co-transfected with Gem1-HA, Gem2-HA and Cdt1-Flag, Gem1-HA and Gem2-HA were able to interact with Cdt1-Flag at the same time (). To further explore whether BmGeminin1 and BmGeminin2 are able to self-associate or interact with each other in cells, BmGeminin1 and BmGeminin2 fused with different tags were co-transfected into BmN-SWU1 cells. As shown, Gem1-HA co-precipitated with Gem1-Flag and Gem2-HA co-precipitated with Gem2-Flag, suggesting that Gem1-Gem1 and Gem2-Gem2 complexes can form in cells (). Moreover, Gem1-HA co-precipitated with Gem2-Flag and Gem2-HA co-precipitated with Gem1-Flag, suggesting that BmGeminin1 and BmGeminin2 can interact with each other.
BmGeminin1 regulates DNA replication and cell cycle progression
To investigate whether overexpression of BmGeminin1 affects cell cycle progression, BmGeminin1 recombinant plasmid was transfected into BmN-SWU1 cells, and the relative expression level of BmGeminin1 was assessed by quantitative real-time reverse transcription PCR (qRT-PCR) 72 h post-transfection. qRT-PCR data showed that the expression level of BmGeminin1 was significantly higher in transfected cells (Figure S4A). Then, we collected the cells 72 h post-transfection and performed flow cytometry analysis. Compared with the control, the number of G2/M cells overexpressing BmGeminin1 was approximately 30% lower, the number of cells in S phase was approximately 28% higher and there was no significant change in the number of cells at G1 phase. This indicated that overexpression of BmGeminin1 led to cell cycle arrest in S phase and prevented the progression to G2/M phase (). To further determine the reason behind the increase in S phase cell numbers after BmGeminin1 overexpression, the BmGeminin1 transfected BmN-SWU1 cells were incubated with BrdU (). BrdU-labeling drastically decreased (15.4 ± 0.6%) in cells overexpressing BmGeminin1 compared with the control (51.2 ± 3.2%) (). These data suggested that the overexpression of BmGeminin1 could result in the inhibition of DNA replication so that cells accumulating BmGeminin1 in the S-phase are unable to efficiently progress to G2 and mitosis.
Figure 4. Overexpression of BmGeminin1 and BmGeminin2 in BmN-SWU1 cells. (A) Cell cycle analysis of BmN-SWU1 cells transfected with BmGeminin1 and BmGeminin2 recombinant plasmids (BmGem1-GFP, BmGem2-GFP) by flow cytometry. GFP was used as the control. (B) Anti-BrdU antibody labeled BmN-SWU1 cells transfected with BmGeminin1 and BmGeminin2. Green fluorescence represents positive cells. (C) Ratio of positive cells labeled with BrdU (**P < 0.01).
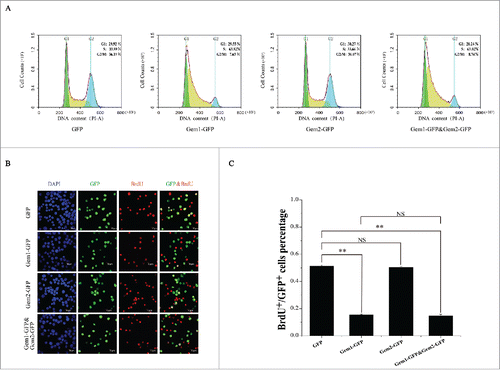
Figure 5. Knockdown of BmGeminin1 or BmGeminin2 in BmN-SWU1 cells with RNA interference. (A) Morphological changes in BmN-SWU1 cells after transfection with BmGeminin1 or BmGeminin2 miRNA. Green fluorescence represents the transfection positive cells. (B) Statistical analysis of the percentage of enlarged cells among the transfection positive cells. (C) DNA content and cell cycle analysis of BmN-SWU1 cells transfected with BmGeminin1 or BmGeminin2 by flow cytometry. 2C and 4C correspond to the DNA content of a normal diploid genome. M1 represents aneuploidy and C represents chromatin-value.
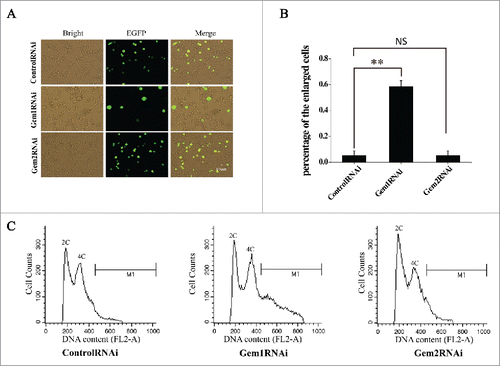
To further verify if BmGeminin1 is implicated in the regulation of DNA replication, microRNA-mediated RNA interference was performed to silence the expression of BmGeminin1 in BmN-SWU1 cells and the relative expression level of BmGeminin1 was assessed by qRT-PCR 7 d post-transfection. qRT-PCR data showed that the transcription of BmGeminin1 was inhibited significantly (Figure S4C). The size of BmN-SWU1 cells increased markedly after treatment with BmGeminin1 RNAi (). The diameter of the normal cells was 8–13 μm and the diameter of the enlarged cells was 14–25 μm. Counting the enlarged cells in the transfected positive cells indicated that knockdown of BmGeminin1 increased the percentage of enlarged cells by 6-fold compared with the control (). To determine if the enlargement of cells occurred after BmGeminin1 knockdown by RNAi due to changes in DNA replication, the microRNA transfected cells were collected for flow cytometry analysis. The results revealed that nearly 30% of BmGeminin1 RNAi treated cells contained more than 4C (C, chromatin-value) DNA suggesting that rereplication occurs in cells with BmGeminin1 knockdown (). These results suggested that BmGeminin1 implicated in the inhibition of rereplication.
BmGeminin2 strengthen the function of BmGeminin1 in regulating DNA replication
Overexpression or RNAi of BmGeminin2 had no significant effects on the cell cycle progression and DNA replication ( and ). Given the ability of BmGeminin2 to form complexes with BmGeminin1 (), we investigated whether BmGeminin2 can affect BmGeminin1 function of regulating DNA replication by co-overexpression and co-interference. BmGeminin1 and BmGeminin2 recombinant plasmid were transfected into BmN-SWU1 cells simultaneously. qRT-PCR data suggested that the expression level of BmGeminin1 and BmGeminin2 were significantly higher in transfected cells (Figure S4B). Flow cytometry analysis showed that compared with the control, the number of G2/M cells overexpressing both BmGeminin1 and BmGeminin2 was approximately 30% lower, the number of cells in S phase was approximately 27% higher and there was no significant change in the number of cells at G1 phase, that result similar to solely overexpression of BmGeminin1. BrdU-labeling analysis also showed that drastically decreased (14.6 ± 1.2%) in cells overexpressing both BmGeminin1 and BmGeminin2 compared with the control (51.2 ± 3.2%), that result similar to solely overexpression of BmGeminin1 (). Meanwhile, BmN-SWU1 cells were transfected with BmGeminin1 and BmGeminin2 microRNA to simultaneously knockdown BmGeminin1 and BmGeminin2. The relative expression levels of BmGeminin1 and BmGeminin2 were assessed by qRT-PCR 7 d post-transfection. The data showed that the transcription of BmGeminin1 and BmGeminin2 were inhibited significantly (Figure S4D). As shown in and , cells treated with BmGeminin1 and BmGeminin2 microRNA had 9-fold higher numbers of enlarged cells compared with the control group (). Flow cytometry analysis also showed that nearly 80% of cells with BmGeminin1 and BmGeminin2 knocked down contained more than 4N DNA content with a continuous peak extending from 4N to 8N (), suggesting that more rereplication occurred in BmGeminin1 and BmGeminin2 knockdown cells than in cells with only BmGeminin1 knockdown. These results indicated that BmGeminin2 also implicated in the inhibition of rereplication, likely strengthening the function of BmGeminin1 in regulating DNA replication.
Figure 6. Simultaneous RNA interference of BmGeminin1 and BmGeminin2 in BmN-SWU1 cells. (A) Cell morphological changes were observed by fluorescence microscopy after BmN-SWU1 cells were co-transfected with BmGeminin1 and BmGeminin2 miRNA (BmGeminin1 and BmGeminin2 RNAi); green fluorescence represents transfection positive cells. (B) Statistical analysis of the percentage of enlarged cells among the transfection positive cells. (C) DNA content and cell cycle analysis of BmN-SWU1 cells after BmGeminin1 and BmGeminin2 RNAi by flow cytometry.
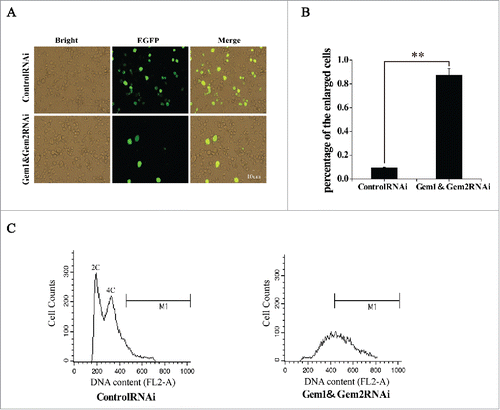
Discussion
In this study, we identified 2 silkworm proteins, containing the conserved coiled-coil domain from Geminin. We named them as BmGeminin1 and BmGeminin2. The central coiled-coil domain of Geminin is conserved from C. elegans to H. sapiens,Citation9,10,13-15 suggesting that it is important for the biologic function of Geminin. Formation of Geminin homodimers is dependent on the coiled-coil domain and the Geminin homodimer binds Cdt1 with high affinity.Citation16 Both Geminin dimerization and Geminin-Cdt1 interaction are essential for the inhibition of DNA replication by Geminin.Citation28 Consistent with other reports, in silkworm also both BmGeminin1 and BmGeminin2 can bind to self and BmCdt1 in cells. Therefore, we can conclude that BmGeminin1 and BmGeminin2 are indeed Geminin orthologs. Interestingly, BmGeminin1 and BmGeminin2 can interact with each other indicating a mutual regulation may exist between BmGeminin1 and BmGeminin2. Previous studies have reported only one Geminin ortholog in various species including H. sapiens, M. musculus, D. melanogaster and C. elegans,Citation9,13-15 except X. laevis,Citation10 which has 2 Geminin proteins, Geminin H and Geminin L. Geminin H and Geminin L share 89% amino acid identity and more importantly they were found to have identical characteristics and functions.Citation10,25 However, BmGeminin1 and BmGeminin2 exhibit overall low amino acid identity (42%) and thereby differ significantly from X. laevis. Because of this low similarity, we propose that BmGeminin1 and BmGeminin2 may have different properties or functions.
Overexpression of BmGeminin1 led to cell cycle arrest in the S phase thus blocking progression into the G2/M phase. In human cell lines, overexpression of Geminin is cell type-specific, leading to arrest at the G1/S phase transition or a defective S-phase followed by apoptosis.Citation24 Similarly, overexpression of Geminin in Drosophila embryos also inhibited DNA replication and resulted in apoptosis.Citation9 DNA replication in eukaryotic cells initiates from thousands of origins of replication to ensure the timely completion of replication.Citation29 It is inferred that when overexpressed, BmGeminin1 may inhibit DNA replication from most origins likely by binding to and inhibiting BmCdt1. This may activate DNA replication checkpoints, a classical mechanism by which cells with damaged or unreplicated DNA are prevented from proceeding to mitosis, so that cell cycle arrest in S phase prevents progression into G2/M phase. In conclusion, BmGeminin1 likely inhibits DNA replication and is implicated in cell cycle regulation. Knockdown of BmGeminin1 by RNAi led to rereplication in BmN-SWU1 cells thus demonstrating that BmGeminin1 is essential to prevent rereplication. It is reported that inactivation of Geminin may also lead to rereplication in normal and tumor cells from humans and activate the Chk1-dependent checkpoint.Citation19 However, depletion of Geminin in Xenopus was not sufficient to induce rereplication. In Xenopus, Geminin mRNA elimination arrested embryonic development in the G2 phase caused by the activation of Chk121. Silencing of Geminin by RNAi in Drosophila Schneider D2 cells lead to the cessation of mitosis and asynchronous rereplication of the genome, indicating that Geminin is also required for the suppression of rereplication and for the stability of genome in Drosophila cells.Citation30 When BmGeminin1 was knocked down in silkworm, it is presumable that BmCdt1 was active as no enough Geminin to bind so that replication origin was repeatedly firing the S phase. Overexpression and RNAi of BmGeminin2 showed no apparent effects of BmGeminin2 on DNA replication and cell cycle regulation. However, rereplication occurred in cells with simultaneous knockdown of BmGeminin1 and BmGeminin2 than in cells where only BmGeminin1 was knocked down. It indicated that BmGeminin2 are also implicated in DNA rereplication inhibition. In X. laevis, Geminin H and Geminin L were equally potent in inhibiting DNA replication.Citation10 While BmGeminin1 and BmGeminin2 could inhibit DNA replication with BmGeminin1 playing a major role and BmGeminin2 playing an auxiliary role. We can presume that in the absence of BmGeminin1 under some circumstances, BmGeminin2 may step in to inhibit DNA replication.
Geminin preferentially forms stable coiled-coil heterodimers with Idas, a protein that shares a homologous coiled-coil domain with Geminin.Citation31 This Idas-Geminin heterodimer binds Cdt1 less strongly than Geminin-Geminin and still has high affinity with Cdt1.Citation31 The Idas homodimer has no effects on DNA replication, while the Idas-Geminin complex is still able to inhibit DNA replication but with about 15 times less effective than the Geminin homodimer.Citation31 In this study, we have shown that BmGeminin1 and BmGeminin2 could form homodimers and interact with BmCdt1, respectively (). In addition, BmGeminin1 and BmGeminin2 could form a heterodimer (). Moreover, when BmGeminin1 and BmGeminin2 were co-expressed in cells, BmCdt1 could interact with both proteins (). These results indicate that the heterodimer formed by BmGeminin1 and BmGeminin2 may also interact with BmCdt1. As for BmGeminin1 homodimers, BmGeminin2 homodimers and BmGeminin1-BmGeminin2 heterodimers, we presume that the preference in the formation of these complexes, and their stability and affinity to bind BmCdt1 may also differ similar to Geminin homologs in vertebrates. The dimerization of Geminin and its interaction with Cdt1 through the coiled-coil domain is required for inhibiting DNA replication.Citation28 While the integrity of the coiled-coil motif is necessary, the adjacent N- and C-terminal short sequences are also required for DNA replication inhibition activity of Geminin.Citation32 The predicted DNA replication inhibition region of BmGeminin1 and BmGeminin2 are shown in . As shown, the overall homology of this region in BmGeminin1 and BmGeminin2 is not high to suggest a difference in inhibiting DNA replication and cell cycle regulation. We presume that the homodimer and heterodimer forms of BmGeminin1 and BmGeminin2 could interact with BmCdt1, although the properties of these complexes may differ. Our data suggest that BmGeminin1 homodimer-BmCdt1 may inhibit DNA replication more strongly, followed by BmGeminin1-BmGeminin2 heterodimer-BmCdt1, and BmGeminin2 homodimer-BmCdt1. In conclusion, both BmGeminin1 and BmGeminin2 are likely to play a role in inhibiting DNA replication. However, BmGeminin1 may play a major role while BmGeminin2 may play an auxiliary role. Additional functions of BmGeminin2 in cell cycle regulation should be determined in further studies.
Materials and methods
Bioinformatics analysis
Using previously published Geminin protein sequences from vertebrates and invertebrates as query in Blast, we searched for Geminin homologs in the silkworm genome database (http://silkworm.genomics.org.cn/). We obtained 2 groups of Expressed Sequence Tags (EST) (Group1: CK492416, AV406281, CK517603, BY938432, and Group2: CK517819, BP181490, CK562097, CK543067) with both encoding a protein containing a conserved Geminin coiled-coil domain after splicing. The protein from group1 was named BmGeminin1 (BmGem1 or Gem1) and that from group2 was named BmGeminin2 (BmGem2 or Gem2). Using the same method, we searched for Cdt1 homologs in B. mori and obtained one group of ESTs. We named the protein encoded by these ESTs as BmCdt1 or Cdt1. Then, known sequences of Geminin homologs from different species were downloaded from NCBI and aligned using Clustal X.Citation33 The phylogenetic tree was reconstructed using the neighbor-joining method in MEGA7.0.Citation34 The tree was drawn to scale with branch lengths reflecting the number of substitutions per site. Numbers on the branches indicate the hits supporting the branching pattern from 10,000 bootstraps.
Plasmid construction
BmGeminin1, BmGeminin2 and BmCdt1 were amplified from silkworm cDNA using gene specific primers. BmGeminin1: Forward 5′ TATTTGTGTATCGGGATTGT 3′ and Reverse 5′ TTAGTGGTATCCTCTTCTGT 3′, BmGeminin2: Forward 5′ TTTGACCGTTGGCATTTTT 3′ and Reverse 5′ TTTCACAAACCACAGCATAG 3′, and BmCdt1: Forward 5′ GGAGGGAAAACATTGAGGCA 3′, and Reverse 5′ CATGTTTTGGCAATTACAGTCA 3′. Then, the amplified products were cloned into the pMD19-T vector (Takara, Dalian, China).
The CDS sequences of BmGeminin1 and BmGeminin2 were amplified from the corresponding pMD19-T plasmids by PCR using the following primers: BmGeminin1: Forward 5′ CGGCGGggtaccATGAGAGCTCGCATGAACAAC 3′ and Reverse 5′ TCCTCCccgcggATCATTTTTAGTGGTATCCTC 3′, and BmGeminin2: Forward 5′ CGGCGGggtaccATGATGAGTGAGCATTCTGGCG 3′ and Reverse 5′ TCCTCCccgcggTTCTATTGGTTCAATTCCATT 3′. The eGFP gene from the peGFP-N1 plasmid (BD Biosciences Clontech) was also cloned into the insect expression vector, pIZ/V5-His (Invitrogen) to construct the pIZ-eGFP vector. The PCR products were cloned into pIZ-eGFP at the Kpn I and Sac II restriction sites. Similarly, BmGeminin1 and BmGeminin2 with different tags (HA 5′ TACCCATACGATGTTCCAGATTACGCT 3′ Flag 5′ GATTACAAGGATGACGACGATAAG 3′) were also cloned into pIZ/V5-His.
Cell culture and transient transfections
The cell line, BmN-SWU1Citation35 derived from silkworm ovary was cultured at 27°C in TC-100 insect medium (United States Biological) supplemented with 10% fetal bovine serum (FBS, Gibco), penicillin (200 U/mL), and streptomycin (200 U/mL). For transient transfection, cells were transfected using FuGENE HD Transfection Reagent, following the instructions of the manufacturer (Roche Applied Science, Mannheim, Germany) and incubated for the times indicated.
Prokaryotic expression, antibody production, affinity purification and antibody characterization
BmGeminin1 and BmGeminin2 were sub-cloned into the prokaryotic expression vector, pET28a. The recombinant plasmids were then transformed into BL21 cells (TransGen Biotech) and were induced with IPTG (0.5 mM) for 5 h at 37°C when the OD of cells was between 0.5–0.6. Cell pellets were sonicated in sonication buffer (300 mM NaCl, 50 mM Na2HPO4/NaH2PO4, 5 mM imidazole, 5 mM β-mercaptoethanol), centrifuged and the supernatants were loaded on Ni-NTA (Qiagen) columns. BmGeminin1 and BmGeminin2 proteins were eluted from the columns with 250 mM imidazole followed by dialysis with 25 mM Tris, 50 mM NaCl buffer to remove imidazole. Then, the recombinant BmGeminin1 and BmGeminin2 proteins were used to immunize rabbits to generate anti-BmGeminin1 and anti-BmGeminin2 antibodies, respectively. Crude sera were affinity purified against the respective proteins used for immunization. Specificity of the purified antibodies was determined by western blot analysis. The results showed that both antibodies could recognize BmGeminin1 and BmGeminin2. Therefore, we used BmGeminin1 antibody to detect both BmGeminin1 and BmGeminin2 in the subsequent experiments.
Immunofluorescence
Cells were fixed in 4% paraformaldehyde for 15 min and permeated with 0.5% Triton X-100 for 15 min. Then, the cells were washed 3 times with phosphate-buffered saline (PBS, Beyotime Institute of Biotechnology, China) containing 5% Tween (PBST) and blocked with 3% BSA and 10% sheep serum in PBS (blocking solution) at 37°C for 1 h. The cells were further incubated with primary antibodies (1:200) in blocking solution for 1.5 h, washed 6 times with PBST and incubated for 1 h with the following secondary antibodies in blocking solution: Alexa 555 conjugated donkey anti-rabbit (1:500; Life Technologies) or Alexa 488-conjugated donkey anti- mouse (1:500; Life Technologies). After washing 6 times in PBST, cellular DNA was stained with DAPI (2, 4-diamidino-2-phenylindole). Finally, the cells were observed under a confocal microscope (Olympus, Tokyo, Japan).
For double immunostaining with BrdU and BmGeminin, cells were incubated with 5 mg/mL BrdU (Roche) for 2 h before fixing. Cells were then fixed in 4% paraformaldehyde, and washed twice with PBST as above, followed by 20 min incubation with 2 M HCl, wash with PBST, and then incubating in the blocking solution for 1 h. Cells were incubated with anti-BrdU antibody (1:50; Roche) or anti-BmGeminin 1 antibody (1:200) in blocking solution for 1.5 h at 37 °C, washed 6 times with PBST, and incubated for 1 h with Alexa 555 conjugated donkey anti-rabbit antibody (1:500; Life Technologies) or Alexa 488-conjugated donkey anti-mouse antibody (1:500; Life Technologies) in blocking solution. DNA was stained with DAPI.
Co-immunoprecipitation (Co-IP)
BmN-SWU1 cells were transfected with 2.5 μg of each of the plasmids and 10 μl of X-treme GENEHP DNA Transfection Reagent (Roche). The cells were collected 72 h post-transfection and washed twice with PBS. Resuspended cells in 1 mL immunoprecipitation (IP) buffer (25 mM Tris, 150 mM NaCl, [pH 7.2]) containing 10 μl protease inhibitors (PMSF). The cells were lysed at 4°C for 30 min and the protein suspension was centrifuged at 13,000 × g for 30 min at 4°C, and 500 μl of the supernatant was added to the anti-FLAG-tag antibody and incubated at 4°C overnight. Then, the protein mixture was added to the Protein A+G Agarose (Beyotime Institute of Biotechnology, China) and incubated at 4°C for 6–9 h. The protein suspension was centrifuged at 3,000 × g for 5 min at 4°C and captured proteins were eluted in IP buffer. Then, 10 μl of 5 × SDS sample buffer was added to the purified protein, boiled in water for 10 min, and centrifuged at 13,000 × g at room temperature for 5 min. Proteins in the supernatant were separated on 12% SDS-PAGE and detected by western blot analyses. The antibodies used in western blot analyses were: mouse anti-FLAG-tag monoclonal antibody (1:5,000) and mouse anti-HA-tag polyclonal antibody (1:5,000).
Bimolecular fluorescence complementation analysis (BiFC)
Bimolecular fluorescence complementation (BiFC) analysis enables direct visualization of protein interactions in living cells. The BiFC assay is based on the discoveries that 2 non-fluorescent fragments of a fluorescent protein can associate to form a fluorescent complex. Association of the fragments can be facilitated by fusing them to 2 proteins that interact with each other.Citation36,37 In this study, one of 2 components of a yellow fluorescent protein termed C-terminus-Venus (VC, amino acid residues 155–238) and N-terminus-Venus (VN, amino acid residues 1–173), was used to investigate protein-protein interactions.Citation38 VC and VN fragments of fluorescent proteins were fused to BmGeminin1, BmGeminin2 or BmCdt1, respectively. If 2 fused proteins associate in close proximity, the complementary VN and VC fragments fold into an active structure, which can be detected by fluorescence microscopy.Citation39 A series of BiFC plasmids were constructed with BmGeminin1, BmGeminin2 and BmCdt1 fused at the N-terminus with VN or VC, respectively. The cells were seeded on coverslips in 24-well plates (Corning) and transfected with recombinant BiFC plasmids. Nuclei of the cells were stained with DAPI. All cells were visualized with the FV1200 laser scanning confocal microscope (Olympus, Tokyo, Japan).
Quantitative real time-PCR (qRT-PCR)
Total RNA was purified from each sample using the Total RNA Kit II (OMEGA) and reverse transcribed into cDNA. Primers used for qRT-PCR were: BmGeminin1: Forward 5′ AGACCAACGAAGGGACTGAAA 3′ and Reverse 5′ GATGCTCCTTTAGGATTTGTTAGA 3′, BmGeminin2: Forward 5′ GAGTGAGCATTCTGGCGAAGA 3′ and Reverse 5′ TCAAGGCACAAGTCGTTCTCTG3′. The house-keeping gene, ribosomal protein gene (rpl3) (forward 5′ CGGTGTTGTTGGATACATTGAG 3′ and reverse 5′ GCTCATCCTGCCATTTCTTACT 3′) was used as the reference gene. qRT-PCR was performed in 15 μl reaction volumes containing 1 μl of cDNA, 0.5 mM of each primer and 2 × iTaqTM Universal SYBR Green Supermix (Bio-Rad) in 96-well plates. The reaction conditions were 94ºC for 30 s, followed by 40 cycles at 95ºC for 5 s and 60ºC for 15 s. Then, the melt curve analysis was performed from 65ºC to 95ºC with 0.5ºC increment for 5 s in each step. Each test was repeated 3 times and the results were analyzed by using student's t-test to determine the statistical significance between the differences. P-value < 0.05 indicated significant difference and P-value < 0.01 indicated highly significant difference.
RNA interference (RNAi) of BmGeminin1 and BmGeminin2 in Bombyx mori cell lines
The siRNA sequences of BmGeminin1 and BmGeminin2 were predicted based on an online software (http://rnaidesigner.invitrogen.com/rnaiexpress/sort.do). Two siRNA sequences per gene were selected and inserted into silkworm endogenous microRNA backbones: bom-mir-279, replacing the endogenous microRNA.Citation40 Then, the whole sequence was synthesized by GenScript (Nanjing, China) and sub-cloned into pIZ-EGFP. These recombinant plasmids were used to transfect BmN-SWU1 cells, which were collected 7 d post-transfection for further testing. We also selected one BmGeminin1 siRNA and one BmGeminin2 siRNA to simultaneously knockdown both BmGeminin1 and BmGeminin2. In addition, mutated siRNA were used as controls.
Flow cytometry
The Cells were washed with PBS and fixed with 70% ethanol at 4°C over-night. After washing with PBS, the cells were treated with RNaseA and propidium iodide (PI) at 37°C for 30 min. Cells were then analyzed immediately by the flow cytometer (Becton Dickinson USA).
Disclosure of potential conflicts of interest
No potential conflicts of interest were disclosed.
1282582_Supplemental_Material.docx
Download MS Word (1.5 MB)Funding
This research was supported by grants of National Natural Science Foundation of China (Nos.31272505, 31472152 and 31572466), China Agriculture Research System (CARS-22).
References
- Kelly TJ, Brown GW. Regulation of chromosome replication. Annual review of biochemistry 2000; 69:829-80; PMID:10966477; http://dx.doi.org/10.1146/annurev.biochem.69.1.829
- Pines J, Hunter T. The differential localization of human cyclins A and B is due to a cytoplasmic retention signal in cyclin B. EMBO J 1994; 13:3772-81; PMID:8070405
- Tada S, Li A, Maiorano D, Mechali M, Blow JJ. Repression of origin assembly in metaphase depends on inhibition of RLF-B/Cdt1 by geminin. Nat Cell Biol 2001; 3:107-13; PMID:11175741; http://dx.doi.org/10.1038/35055000
- Mahbubani HM, Chong JP, Chevalier S, Thommes P, Blow JJ. Cell cycle regulation of the replication licensing system: involvement of a Cdk-dependent inhibitor. J Cell Biol 1997; 136:125-35; PMID:9008708
- Blow JJ, Laskey RA. A role for the nuclear envelope in controlling DNA replication within the cell cycle. Nature 1988; 332:546-8; PMID:3357511; http://dx.doi.org/10.1038/332546a0
- Maiorano D, Moreau J, Mechali M. XCDT1 is required for the assembly of pre-replicative complexes in Xenopus laevis. Nature 2000; 404:622-5; PMID:10766247; http://dx.doi.org/10.1038/35007104
- Nishitani H, Lygerou Z, Nishimoto T, Nurse P. The Cdt1 protein is required to license DNA for replication in fission yeast. Nature 2000; 404:625-8; PMID:10766248; http://dx.doi.org/10.1038/35007110
- Whittaker AJ, Royzman I, Orr-Weaver TL. Drosophila double parked: a conserved, essential replication protein that colocalizes with the origin recognition complex and links DNA replication with mitosis and the down-regulation of S phase transcripts. Genes Dev 2000; 14:1765-76; PMID:10898791
- Quinn LM, Herr A, McGarry TJ, Richardson H. The Drosophila Geminin homolog: roles for Geminin in limiting DNA replication, in anaphase and in neurogenesis. Genes Dev 2001; 15:2741-54; PMID:11641279; http://dx.doi.org/10.1101/gad.916201
- McGarry TJ, Kirschner MW. Geminin, an inhibitor of DNA replication, is degraded during mitosis. Cell 1998; 93:1043-53; PMID:9635433
- Wohlschlegel JA, Dwyer BT, Dhar SK, Cvetic C, Walter JC, Dutta A. Inhibition of eukaryotic DNA replication by geminin binding to Cdt1. Science 2000; 290:2309-12; PMID:11125146; http://dx.doi.org/10.1126/science.290.5500.2309
- Pefani DE, Dimaki M, Spella M, Karantzelis N, Mitsiki E, Kyrousi C, Symeonidou IE, Perrakis A, Taraviras S, Lygerou Z. Idas, a novel phylogenetically conserved geminin-related protein, binds to geminin and is required for cell cycle progression. J Biol Chem 2011; 286:23234-46; PMID:21543332; http://dx.doi.org/10.1074/jbc.M110.207688
- Yanagi K, Mizuno T, Tsuyama T, Tada S, Iida Y, Sugimoto A, Eki T, Enomoto T, Hanaoka F. Caenorhabditis elegans geminin homologue participates in cell cycle regulation and germ line development. J Biol Chem 2005; 280:19689-94; PMID:15811859; http://dx.doi.org/10.1074/jbc.C500070200
- Dhar SK, Yoshida K, Machida Y, Khaira P, Chaudhuri B, Wohlschlegel JA, Leffak M, Yates J, Dutta A. Replication from oriP of Epstein-Barr virus requires human ORC and is inhibited by geminin. Cell 2001; 106:287-96; PMID:11509178
- Yanagi K, Mizuno T, You Z, Hanaoka F. Mouse geminin inhibits not only Cdt1-MCM6 interactions but also a novel intrinsic Cdt1 DNA binding activity. J Biol Chem 2002; 277:40871-80; PMID:12192004; http://dx.doi.org/10.1074/jbc.M206202200
- Saxena S, Yuan P, Dhar SK, Senga T, Takeda D, Robinson H, Kornbluth S, Swaminathan K, Dutta A. A dimerized coiled-coil domain and an adjoining part of geminin interact with two sites on Cdt1 for replication inhibition. Mol Cell 2004; 15:245-58; PMID:15260975; http://dx.doi.org/10.1016/j.molcel.2004.06.045
- Thepaut M, Maiorano D, Guichou JF, Auge MT, Dumas C, Mechali M, Padilla A. Crystal structure of the coiled-coil dimerization motif of geminin: structural and functional insights on DNA replication regulation. J Mol Biol 2004; 342:275-87; PMID:15313623; http://dx.doi.org/10.1016/j.jmb.2004.06.065
- Yoshida K, Oyaizu N, Dutta A, Inoue I. The destruction box of human Geminin is critical for proliferation and tumor growth in human colon cancer cells. Oncogene 2004; 23:58-70; PMID:14712211; http://dx.doi.org/10.1038/sj.onc.1206987
- Zhu W, Chen Y, Dutta A. Rereplication by depletion of geminin is seen regardless of p53 status and activates a G2/M checkpoint. Mol Cell Biol 2004; 24:7140-50; PMID:15282313; http://dx.doi.org/10.1128/MCB.24.16.7140-7150.2004
- Melixetian M, Ballabeni A, Masiero L, Gasparini P, Zamponi R, Bartek J, Lukas J, Helin K. Loss of Geminin induces rereplication in the presence of functional p53. J Cell Biol 2004; 165:473-82; PMID:15159417; http://dx.doi.org/10.1083/jcb.200403106
- McGarry TJ. Geminin deficiency causes a Chk1-dependent G2 arrest in Xenopus. Mol Biol Cell 2002; 13:3662-71; PMID:12388764; http://dx.doi.org/10.1091/mbc.E02-04-0199
- Tachibana KE, Gonzalez MA, Guarguaglini G, Nigg EA, Laskey RA. Depletion of licensing inhibitor geminin causes centrosome overduplication and mitotic defects. EMBO Rep 2005; 6:1052-7; PMID:16179947; http://dx.doi.org/10.1038/sj.embor.7400527
- Zhu W, Depamphilis ML. Selective killing of cancer cells by suppression of geminin activity. Cancer Res 2009; 69:4870-7; PMID:19487297; http://dx.doi.org/10.1158/0008-5472.CAN-08-4559
- Shreeram S, Sparks A, Lane DP, Blow JJ. Cell type-specific responses of human cells to inhibition of replication licensing. Oncogene 2002; 21:6624-32; PMID:12242660; http://dx.doi.org/10.1038/sj.onc.1205910
- Kroll KL, Salic AN, Evans LM, Kirschner MW. Geminin, a neuralizing molecule that demarcates the future neural plate at the onset of gastrulation. Development 1998; 125:3247-58; PMID:9671596
- Altman GH, Diaz F, Jakuba C, Calabro T, Horan RL, Chen J, Lu H, Richmond J, Kaplan DL. Silk-based biomaterials. Biomaterials 2003; 24:401-16; PMID:12423595
- MacIntosh AC, Kearns VR, Crawford A, Hatton PV. Skeletal tissue engineering using silk biomaterials. J Tissue Eng Regen Med 2008; 2:71-80; PMID:18383453; http://dx.doi.org/10.1002/term.68
- Benjamin JM, Torke SJ, Demeler B, McGarry TJ. Geminin has dimerization, Cdt1-binding, and destruction domains that are required for biological activity. J Biol Chem 2004; 279:45957-68; PMID:15308655; http://dx.doi.org/10.1074/jbc.M407726200
- De Marco V, Gillespie PJ, Li A, Karantzelis N, Christodoulou E, Klompmaker R, van Gerwen S, Fish A, Petoukhov MV, Iliou MS, et al. Quaternary structure of the human Cdt1-Geminin complex regulates DNA replication licensing. Proc Nat Acad Sci U S A 2009; 106:19807-12; PMID:19906994; http://dx.doi.org/10.1073/pnas.0905281106
- Mihaylov IS, Kondo T, Jones L, Ryzhikov S, Tanaka J, Zheng J, Higa LA, Minamino N, Cooley L, Zhang H. Control of DNA replication and chromosome ploidy by geminin and cyclin A. Mol Cell Biol 2002; 22:1868-80; PMID:11865064
- Caillat C, Fish A, Pefani DE, Taraviras S, Lygerou Z, Perrakis A. The structure of the GemC1 coiled coil and its interaction with the Geminin family of coiled-coil proteins. Acta Crystallogr D Biol Crystallogr 2015; 71:2278-86; PMID:26527144; http://dx.doi.org/10.1107/S1399004715016892
- Luo L, Yang X, Takihara Y, Knoetgen H, Kessel M. The cell-cycle regulator geminin inhibits Hox function through direct and polycomb-mediated interactions. Nature 2004; 427:749-53; PMID:14973489; http://dx.doi.org/10.1038/nature02305
- Thompson JD, Gibson TJ, Plewniak F, Jeanmougin F, Higgins DG. The CLUSTAL_X windows interface: flexible strategies for multiple sequence alignment aided by quality analysis tools. Nucleic Acids Res 1997; 25:4876-82; PMID:9396791; http://dx.doi.org/gka797 [pii]
- Kumar S, Stecher G, Tamura K. MEGA7: molecular evolutionary genetics analysis version 7.0 for bigger datasets. Mol Biol Evol 2016; 33:1870-4; PMID:27004904; http://dx.doi.org/10.1093/molbev/msw054
- Pan MH, Cai XJ, Liu M, Lv J, Tang H, Tan J, Lu C. Establishment and characterization of an ovarian cell line of the silkworm, Bombyx mori. Tissue Cell 2010; 42:42-6; PMID:19665160; http://dx.doi.org/10.1016/j.tice.2009.07.002
- Kerppola TK. Design and implementation of bimolecular fluorescence complementation (BiFC) assays for the visualization of protein interactions in living cells. Nat Protoc 2006; 1:1278-86; PMID:17406412; http://dx.doi.org/10.1038/nprot.2006.201
- Kerppola TK. Bimolecular fluorescence complementation (BiFC) analysis as a probe of protein interactions in living cells. Annu Rev Biophys 2008; 37:465-87; PMID:18573091; http://dx.doi.org/10.1146/annurev.biophys.37.032807.125842
- Nyfeler B, Michnick SW, Hauri HP. Capturing protein interactions in the secretory pathway of living cells. Proc Nati Acad Sci U S A 2005; 102:6350-5; PMID:15849265; http://dx.doi.org/10.1073/pnas.0501976102
- Remy I, Michnick SW. Application of protein-fragment complementation assays in cell biology. Biotechniques 2007; 42:137, 9, 41 passim; PMID:17373475; http://dx.doi.org/000112396 [pii]
- Zhang J, He Q, Zhang CD, Chen XY, Chen XM, Dong ZQ, Li N, Kuang XX, Cao MY, Lu C, et al. Inhibition of BmNPV replication in silkworm cells using inducible and regulated artificial microRNA precursors targeting the essential viral gene lef-11. Antiviral Res 2014; 104:143-52; PMID:24486953; http://dx.doi.org/10.1016/j.antiviral.2014.01.017