ABSTRACT
Smc1β is a meiosis-specific cohesin subunit that is essential for sister chromatid cohesion and DNA recombination. Previous studies have shown that Smc1β-deficient mice in both sexes are sterile. Ablation of Smc1β during male meiosis leads to the blockage of spermatogenesis in pachytene stage, and ablation of Smc1β during female meiosis generates a highly error-prone oocyte although it could develop to metaphase II stage. However, the underlying mechanisms regarding how Smc1β maintains the correct meiotic progression in mouse oocytes have not been clearly defined. Here, we find that GFP-fused Smc1β is expressed and localized to the chromosomes from GV to MII stages during mouse oocyte meiotic maturation. Knockdown of Smc1β by microinjection of gene-specific morpholino causes the impaired spindle apparatus and chromosome alignment which are highly correlated with the defective kinetochore-microtubule attachments, consequently resulting in a prominently higher incidence of aneuploid eggs. In addition, the premature extrusion of polar bodies and escape of metaphase I arrest induced by low dose of nocodazole treatment in Smc1β-depleted oocytes indicates that Smc1β is essential for activation of spindle assembly checkpoint (SAC) activity. Collectively, we identify a novel function of Smc1β as a SAC participant beyond its role in chromosome cohesion during mouse oocyte meiosis.
Introduction
Cohesin complex is well known as its roles in mediating chromosome cohesion and segregation in both mitosis and meiosis.Citation1 To ensure correct meiotic chromosome segregation, sister chromatid cohesion (SCC) needs to be maintained from its establishment in prophase I oocytes before birth until continuation of meiosis into metaphase II upon oocyte maturation in the adult.Citation2 At anaphase II cohesin is cleaved by separase between sister chromatids, allowing them segregated to generate haploid gametes.Citation1-6 Aging human oocytes suffer a steep increase in chromosome missegregation and aneuploidy, which may be caused by loss of SCC through slow deterioration of cohesin.Citation7-9 This hypothesis assumes that cohesin expression in embryonic oocytes is sufficient to provide adequate long-term SCC. With increasing age, mouse oocytes deficient in the meiosis-specific cohesin Smc1β massively lose SCC and chiasmata.Citation9,10 Specific inactivation of mouse Smc1β gene at the primordial follicle stage shortly after birth when oocytes had just entered meiosis I dictyate arrest has normal chiasma positions and SCC in the adult, suggesting that Smc1β cohesin needs only be expressed during prophase I before the primordial follicle stage to ensure SCC up to advanced age of mice.Citation28
Beyond its well-known role in SCC, Smc1β also exerts other functions in various biologic events. Smc1β determines meiotic chromatin loop organization and proper axes/loop structure of axial elements (AEs) and synaptonemal complexes (SCs). In the absence of Smc1β, AEs and SCs are greatly shortened to about half length of those in wild-type mice.Citation11,12 In addition, Smc1β serves a specific role at telomeres independent of its role in determining AE/SC length and loop extension. In Smc1β−/− meiocytes, one fifth of their telomeres fail to attach to the nuclear envelope.Citation13 Telomeres in Smc1β−/− spermatocytes and oocytes lose their structural integrity and suffer a range of abnormalities, suggesting that a telomere structure protected from DNA rearrangements depends on Smc1β. Smc1β-deficient mice in both sexes are sterile. Ablation of Smc1β during male meiosis leads to the blockage of spermatogenesis in pachytene stage, and ablation of Smc1β during female meiosis generates a highly error-prone oocyte although it could develop to metaphase II stage.Citation11 Loss of Smc1β affects spermatocytes more dramatically than oocytes, for spermatocytes arrest in mid-pachytene with a failure to complete synapsis, Smc1β-deficient spermatocytes die in midpachytene.Citation11 Despite accumulating previous research, the functional roles of Smc1β during oocyte meiotic maturation remain elusive.
Here, we report that Smc1β localizes at chromosomes during mouse oocyte meiosis and is required for normal spindle assembly, proper chromosome alignment and correct kinetochore-microtubule attachments. Notably, Smc1β is involved in the activation of SAC function to maintain the euploidy during mouse oocyte maturation.
Results
Smc1β localizes to the chromosomes during mouse oocyte meiosis
To examine the localization of Smc1β during meiotic maturation in mouse oocytes, a construct fused with a fluorescent tag GFP to the C-terminus of Smc1β was made and in vitro transcribed into cRNA. Following microinjection with cRNA, oocytes were arrested at GV stage for another 6 h to allow cRNA to be fully translated to protein, and then cultured to specific time points to observe Smc1β-GFP under the confocal microscope. The result showed that Smc1β exhibited a co-localization with chromosome staining by Hoechst from GV to MII stages (), indicating that Smc1β is localized to chromosomes during oocyte meiosis.
Figure 1. Subcellular localization of Smc1β during mouse oocyte meiotic maturation. Mouse oocytes were microinjected with Smc1β-GFP cRNA at GV stage, and then cultured to GVBD, Pro-MI, MI, ATI and MII stages, respectively, followed by nuclear staining with Hoechst (blue). GV, oocytes at germinal vesicle stage; GVBD, oocytes at germinal vesicle breakdown stage; Pro-MI, oocytes at first prometaphase stage; MI, oocytes at first metaphase stage; ATI, oocytes at first anaphase/telophase stage; MII, oocytes at second metaphase stage. Scale bar, 20 μm.
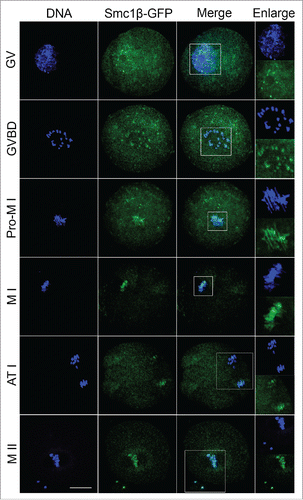
Depletion of Smc1β impairs spindle assembly and chromosome alignment during mouse oocyte meiosis
To clearly define the role of Smc1β during meiotic maturation, a morpholino-based gene-silencing approach was applied to deplete Smc1β. Fully-grown GV oocytes were microinjected with non-targeting or Smc1β-targeting morpholinos and arrested in medium supplemented with milrinone for 20 h, allowing sufficient time to inhibit mRNA translation. As shown in , the protein level of Smc1β in Smc1β-KD oocytes was pronouncedly decreased compared with control oocytes, confirming the knockdown effect through morpholino injection. Then oocytes were immunostained with anti-α-tubulin-FITC antibody and counterstained with Hoechst to observe the spindle morphologies as well as the chromosome alignment. The staining result showed that a great mass of control oocytes displayed a typical barrel-shape spindle apparatus with a well-aligned chromosome on the equatorial plate (). However, a diversity of disorganized spindle apparatuses with scattered chromosomes was present in Smc1β-KD oocytes (). Quantitatively, almost 80% of oocytes displayed the aberrant spindle morphologies (78.3% ± 2.1%, n = 142) and more than 80% of oocytes exhibited misaligned chromosomes (81% ± 1.0%, n = 140) compared with approximately 20% of defects in controls (20% ± 1.0%, n = 121; 17.5% ± 0.5%, n = 127; p < 0.05; ). Thus, our data reveal that Smc1β participates in the regulation of spindle assembly and chromosome alignment during mouse oocyte meiotic maturation.
Figure 2. Knockdown of Smc1β causes spindle/chromosome abnormalities in mouse oocytes. (A) Protein levels of Smc1β in control and Smc1β-MO (morpholino injected) oocytes. The blots were probed with anti-Smc1β antibody and anti-GAPDH antibody, respectively. (B) Representative images of spindle morphologies and chromosome alignment in control and Smc1β-MO oocytes. Oocytes were immunostained with anti-α-tubulin-FITC antibody to visualize spindles and counterstained with Hoechst to visualize chromosomes. Scale bar, 20μm. (C) The proportion of abnormal spindles was recorded in control and Smc1β-MO oocytes. Data were presented as mean percentage (mean ± SEM) of at least 3 independent experiments. Asterisk denotes statistical difference at a p < 0.05 level of significance. (D) The proportion of misaligned chromosomes was recorded in control and Smc1β-MO oocytes. Data were presented as mean percentage (mean ± SEM) of at least 3 independent experiments. Asterisk denotes statistical difference at a p < 0.05 level of significance.
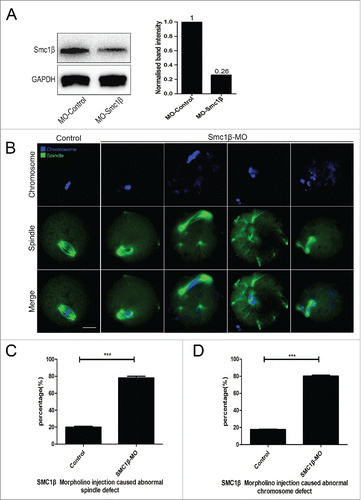
Depletion of Smc1β compromises kinetochore-microtubule attachments during mouse oocyte meiosis
Impaired spindle formation and incorrect chromosome alignment predicts that the interaction between kinetochores and microtubules might be defective. To test this possibility, we assessed the stability of kinetochore-microtubule attachments by using cold treatment to depolymerize unstable microtubules upon depletion of Smc1β. To this end, MI oocytes were briefly chilled to induce depolymerization of microtubules that are not attached to kinetochores, and then immunostained with CREST to detect kinetochores, with anti-α-tubulin-FITC antibody to visualize the microtubules and counterstained with Hoechst to observe chromosomes. The staining result showed that kinetochores were fully attached by microtubules and chromosomes were well-aligned after cold treatment in most of control oocytes (13.2% ± 1.4%, n = 98; ). By contrast, a prominently increased rate of kinetochores with very few cold-stable microtubules was observed in Smc1β-KD oocytes (74.7% ± 2.3%, n = 101, p < 0.05; ). Collectively, this observation suggests that kinetochore-microtubule attachments are less stable after depletion of Smc1β, which might lead to the failure of spindle assembly and chromosome alignment.
Figure 3. Knockdown of Smc1β leads to the disrupted kinetochore-microtubule attachment in mouse oocytes. (A) Representative images of kinetochore-microtubule attachments in control and Smc1β-MO oocytes. Oocytes were immunostained with anti-α-tubulin-FITC antibody to visualize spindles, with CREST to visualize kinetochores, and counterstained with Hoechst to visualize chromosomes. Scale bar, 5 μm. (B) The proportion of defective kinetochore-microtubule attachments was recorded in control and Smc1β-MO oocytes. Data were presented as mean percentage (mean ± SEM) of at least 3 independent experiments. Asterisk denotes statistical difference at a p < 0.05 level of significance.
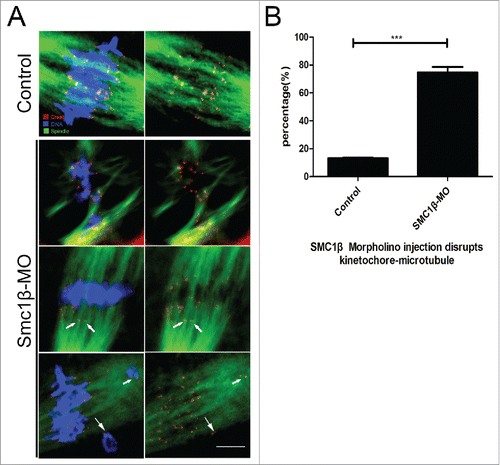
Smc1β is required for prevention of aneuploidy in mouse oocytes
Since chromosome misalignment is highly correlated with the generation of aneuploidy, an abnormal number of chromosomes which might lead to miscarriage, embryonic lethality or genetic disorders in mouse eggs, we then performed the karyotyping analysis of MII eggs by chromosome spreading. As shown in , the number of single chromosomes (univalents) in the normal eggs was 20, which is the prerequisite for genomic integrity in the mouse. However, a remarkably higher incidence of aneuploid eggs that had more or less 20 univalents was found in Smc1β-KD oocytes compared with controls (13.9% ± 1.4%, n = 59 VS 83.8% ± 0.8%, n = 61, p < 0.05; ), indicating that oocytes are unable to correctly assemble the spindles and properly align the chromosomes to generate euploid eggs in the absence of Smc1β.
Figure 4. Knockdown of Smc1β results in the generation of aneuploidy in mouse eggs. (A) Representative images of euploid and aneuploid MII eggs. Chromosome spread was performed to calculate the number of chromosomes. Chromosomes were counterstained with PtdIns. Scale bar, 5 μm. (B) The proportion of aneuploid eggs was recorded in control and Smc1β-MO oocytes. Data were presented as mean percentage (mean ± SEM) of at least 3 independent experiments. Asterisk denotes statistical difference at a p < 0.05 level of significance.
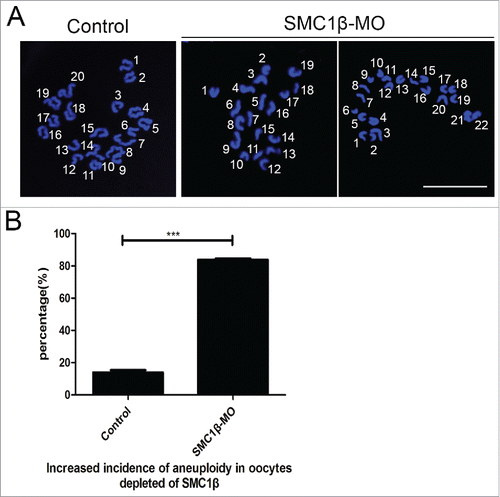
Smc1β is necessary for activation of SAC during oocyte meiosis
We next examined the effect of Smc1β knockdown on the meiotic progression. After culture of GV oocytes to the specific time points, the rates of GVBD and polar body extrusion were counted in the control and Smc1β-KD oocytes, respectively. The result showed that loss of Smc1β did not affect either germinal vesicle breakdown or extrusion of first polar body (72.2% ± 3.4%, n = 143 VS 75.6% ± 1.5%, n = 178; 69.8% ± 1.7%, n = 130 VS 67.2% ± 2.2%, n = 166; ), 2 critical developmental events during oocyte maturation. However, at the time point of 7 h post-GVBD, a higher percentage of PBE was observed in Smc1β-KD oocytes than that in controls (13.6% ± 1.1%, n = 137 VS 32.5% ± 0.9%, n = 170, p < 0.05; ), suggesting that polar bodies were precociously extruded in the absence of Smc1β.
Figure 5. Effects of Smc1β Knockdown on the meiotic progression and SAC activity in mouse oocytes. (A) The proportion of germinal vesicle breakdown was recorded in control and Smc1β-MO oocytes. (B) The proportion of polar body extrusion was recorded in control and Smc1β-MO oocytes at the time point of 12 h post-GVBD. (C) The proportion of precocious polar body extrusion was recorded in control and Smc1β-MO oocytes at the time point of 7 h post-GVBD. (D) The proportion of overriding MI arrest was recorded in control and Smc1β-MO oocytes by low dose of nocodazole treatment. Data were presented as mean percentage (mean ± SEM) of at least 3 independent experiments. Asterisk denotes statistical difference at a p < 0.05 level of significance.
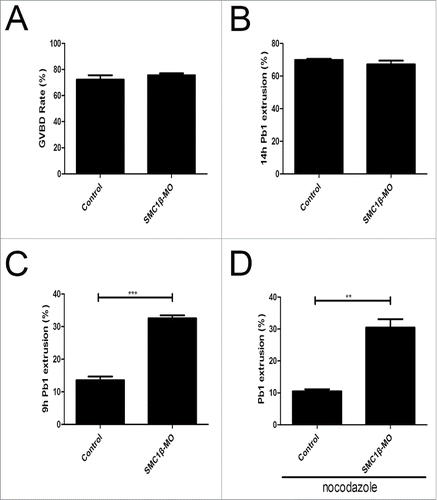
The premature PBE indicates that SAC activity is compromised in Smc1β-KD oocytes. To further verify this possibility, we tested whether oocytes depleted of Smc1β would override the MI arrest induced by low dose of nocodazole treatment, indicative of SAC inactivation. For this purpose, GV oocytes were cultured to 4 h post-GVBD and then transferred to the medium supplemented with 0.04 µg/ml of nocodazole for 6 h to observe the polar body extrusion. The result showed that only about 8% of control oocytes could abrogate MI arrest and extrude the first polar body in the presence of low dose of nocodazole. Whereas Smc1β-KD oocytes showed a prominent increase in the overriding rate and around 30% of oocytes finally reached the MII stage (10.5% ± 0.6%, n = 161 VS 30.5% ± 2.6%, n = 187, p < 0.05; ). Therefore, the above observations reveal that Smc1β is required for SAC activation and might regulate SAC proteins during meiosis.
Discussion
The cohesin complex and its accessory factors contribute in many ways to genomic organization and stability. Proteins in this network have been shown to execute functions in chromosome segregation, gene regulation, DSB repair and chromosome morphology.Citation1 In meiosis, the generation of mammalian gametes comprises 2 subsequent cell divisions (meiosis I and II) during their development to reduce the ploidy.Citation9,10 Any errors in these processes generating aneuploidy would lead to the miscarriage, age-related infertility, and high incidence of genetic disorders such as Down syndrome in humans.Citation14-17
Meiotic cohesin complexes are composed of 4 essential subunits, including a meiosis-specific Smc1 subunit (Smc1β), 2 additional α-kleisins (Rad21L and Rec8) and another stromal antigen protein (Stag3).Citation18,19 Previous studies have investigated the functions of Smc1β in determining the axis-loop structure of synaptonemal complexes, in providing sister chromatid cohesion in metaphase I and thereafter, in protecting telomere structure, and in synapsis.Citation13 However, litter is known about the roles of Smc1β during the development window of oocyte meiotic maturation.
Prevention of generating aneuploid cells is very important for the health of offspring during meiotic divisions and aneuploid embryos usually perish in utero, and even when they survive to term, they suffer from severe congenital birth defects.Citation20 Loss of sister chromatid cohesion during meiosis has been postulated as a key factor in age-related non-disjunction in human oocytes.Citation21-25 Notably, a recent research has shown that the integrity of the spindle checkpoint response depends on the centromeric cohesin in mammalian oocytes.Citation26
In this study, we found that Smc1β accumulated on the chromosomes during mouse oocyte meiotic maturation. This localization pattern is consistent with the typical chromatin distribution of cohesin subunits in mitosis. Also, it is in line with Smc1β localization on chromosome axes in early meiosis.Citation11,27,28 To clearly define its role during meiotic maturation, loss-of-function experiments were performed by gene-targeting morpholino microinjection in GV oocytes. In mammalian females, cohesin complex has been loaded and locked on chromosomes in fetal oocytes, and would not undergo turnover after birth during arrest at the prolonged dictyate stage.Citation29,30 Thus, during the window of oocyte meiotic maturation, cohesin complex no longer needs Smc1β, suggesting that another pool of Smc1β might play a particular role in this process. As expected, our data showed that spindle assembly was disrupted in Smc1β-KD oocytes. Accompanied by this defect, scattered and lagging chromosomes were also observed in the absence of Smc1β.
The observation of spindle/chromosome abnormalities further prompted us to examine the kinetochore-microtubule attachment following knockdown of Smc1β. The kinetochore contains approximately 100 different proteins that clustered in several different complexes on centromeric DNA, including inner kinetochore proteins, outer kinetochore proteins, as well as regulatory proteins.Citation31-33 Through these multiprotein structures, kinetochore attaches chromosomes to spindle microtubules and maintain the attachment to growing or disassembling microtubule to drive chromosomes segregation.Citation34-36 Therefore, if errors of kinetochore-microtubule attachment could not be corrected until anaphase, they would cause chromosome misalignment and missegregation.Citation37,38 We thus propose that Smc1β is involved in the maintenance of the attachment between kinetochores and microtubules during mouse oocyte meiosis. Consistent with our expectation, the quantitative data showed that an apparently increased rate of kinetochores was unattached by microtubules upon cool treatment which is able to depolymerize unattached microtubules. As a result, loss of Smc1β generated an elevated incidence of aneuploid eggs which are highly correlated with miscarriage, birth defects and genetic disorders.
Finally, we tested the effect of Smc1β on the meiotic progression. Knockdown of Smc1β did not affect GVBD and PBE, 2 key events occurring during meiotic maturation. However, it did cause the precocious PBE at anaphase phase, indicating that SAC activity is compromised in depletion of Smc1β. Another line of evidence to prove that SAC function is impaired in Smc1β-depleted oocytes is that oocytes were able to override the MI arrest induced by nocodazole in the absence of Smc1β. These observations are coincident with the recent proposed concept that the spindle checkpoint response depends on centromeric cohesin in mammalian oocytes.Citation26
In summary, our study presents several lines of evidence revealing that Smc1β is a SAC regulator that maintains normal spindle assembly, proper chromosome alignment and correct kinetochore-microtubule attachment to prevent generation of aneuploid eggs, discovering a new molecular determinant controlling oocyte development.
Materials and methods
Antibodies
Rabbit polyclonal anti-Smc1β antibody was purchased from Abcam (Cambridge, MA, USA; Cat#: EP2879Y); mouse monoclonal anti-α-tubulin-fluorescein isothiocyanate (FITC) antibody was purchased from Sigma (St. Louis, MO, USA; Cat#: F2168); human anti-centromere antibody was purchased from Antibodies Inc. (Davis, CA, USA; Cat#: 15–234); Alexa Fluor 555 conjugated goat anti-Human IgG (H+L) was purchased from ThermoFisher (Waltham, MA, USA; Cat#: A-21433).
Oocyte collection and culture
All experiments were approved by the Animal Care and Use Committee of Nanjing Agricultural University, China and were performed in accordance with institutional guidelines. Female ICR mice (4–6 weeks) were killed by cervical dislocation after intraperitoneal injections of 5 IU pregnant mare serum gonadotropin (PMSG) for 46 h. Fully-grown oocytes arrested at prophase of meiosis I were collected from ovaries in M2 medium (Sigma, St. Louis, MO, USA). Only those immature oocytes displaying a germinal vesicle (GV) were cultured further in M16 medium (Sigma, St. Louis, MO, USA) under liquid paraffin oil at 37°C in an atmosphere of 5% CO2 incubator for in vitro maturation. At different time points after culture, oocytes were collected for subsequent analysis.
cRNA construct and in vitro transcription
For in vitro transcription, full-length Smc1β cDNA was sub-cloned into pcDNA3-EGFP vector. Capped cRNA was synthesized from linearized plasmid using T7 mMessage mMachine kit (ThermoFisher, Waltham, MA, USA), and purified with MEGAclear kit (ThermoFisher, Waltham, MA, USA). Typically, 10–12 pl (4% of the oocyte volume) of 0.5–1.0 ug/ul cRNA was injected into oocytes. The oocytes were then arrested at the GV stage in M16 medium containing 2.5 µM milrinone for 6 h, allowing enough time for translation, and then released into milrinone-free M16 medium for further study.
Morpholino knockdown
Smc1β-targeting morpholino (5′-GAGCAGCAGCTCAAGGTGCCCCAT-3′) was obtained from Gene Tools LLC (Philomath, OR, USA), and then diluted to 1uM as working concentration. For knockdown experiment, about 5–10 pl of morpholino was microinjected into the cytoplasm of fully grown GV oocytes using a Narishige microinjector (Tokyo, Japan). A non-targeting morpholino was injected as a control. To facilitate the morpholino-mediated inhibition of mRNA translation, oocytes were arrested at GV stage in M16 medium containing 2.5 µM milrinone for 20 h, and then cultured in milrinone-free M16 medium for subsequent experiments.
Immunofluorescence and confocal microscopy
Oocytes were fixed in 4% paraformaldehyde in PBS (pH 7.4) for 30 minutes and permeabilized in 0.5% Triton-X-100 for 20 min at room temperature. Then, oocytes were blocked with 1% BSA-supplemented PBS for 1 h and incubated with anti-α-tubulin-FITC (1:200) or anti-centromere (1:200) antibodies at 4°C overnight. After washing 4 times (5 min each) in PBS containing 1% Tween 20 and 0.01% Triton-X 100, oocytes were incubated with an appropriate secondary antibody for 1 h at room temperature. After washing 3 times, oocytes were counterstained with PI (Propidium Iodide) or Hoechst 33342 (10 µg/ml) for 10 min. Finally, oocytes were mounted on glass slides and observed under a confocal laser scanning microscope (Carl Zeiss 700).
Western blotting
A pool of 300 oocytes was lysed in 4 × LDS sample buffer (ThermoFisher, Waltham, MA, USA) containing protease inhibitor, and then separated on 10% Bis-Tris precast gels and transferred onto PVDF membranes. The blots were blocked in TBST (Tris-buffred saline containing 0.1% Tween 20) containing 5% low fat dry milk for 1 h at room temperature and then incubated with anti-Smc1β antibody (1:1000) overnight at 4°C. After 3 times of washes in TBST, the blots were incubated with 1:10,000 dilution of HRP (Horse Radish Peroxidase) conjugated secondary antibodies for 1 h at room temperature. Chemiluminescence was detected with ECL Plus Western Blotting Detection System (GE, Piscataway, NJ, USA) and protein bands were visualized by Tanon-3900. The blots were then stripped and reblotted with anti-β-actin antibody (1:5000) for loading control.
Chromosome spread
Oocytes were exposed to Tyrode's buffer (pH 2.5) for about 30 s at 37°C to remove zona pellucidae. After recovery in M2 medium for 10 min, oocytes were fixed in a drop of 1% paraformaldehyde with 0.15% Triton X-100 on a glass slide. After air drying, chromosomes were counterstained with PtdIns and examined under a laser scanning confocal microscope.
Statistical analysis
The data were expressed as mean ± SEM and analyzed by one-way ANOVA, followed by LSD's post hoc test, which was provided by SPSS16.0 statistical software. The level of significance was accepted as p < 0.05.
Author contributions
Y.M. and B.X. designed the research; Y.M., C.Z., Z.C., X.D., M.Z. and Y.L. performed the experiments; Y.M. and B.X. analyzed the data; Y.M. and B.X. wrote the manuscript.
Disclosure of potential conflicts of interest
No potential conflicts of interest were disclosed.
Funding
This work was supported by the National Natural Science Foundation (31571545) and Natural Science Foundation of Jiangsu Province (BK20150677).
References
- Xiong B, Gerton JL. Regulators of the cohesin network. Annu Rev Biochem 2010; 79(1):131-53; PMID:20331362; http://dx.doi.org/10.1146/annurev-biochem-061708-092640
- Michaelis C, Ciosk R, Nasmyth K. Cohesins: chromosomal proteins that prevent premature separation of sister chromatids. Cell 1997; 91:35-6; PMID:9335333; http://dx.doi.org/10.1016/S0092-8674(01)80007-6
- Nasmyth K. Disseminating the genome: joining, resolving, and separating sister chromatids during mitosis and meiosis. Annu Rev Genet 2001; 35:673-45; PMID:11700297; http://dx.doi.org/10.1146/annurev.genet.35.102401.091334
- Guacci V, Koshland D, Strunnikov A. A direct link between sister chromatid cohesion and chromosome condensation revealed through the analysis of MCD1 in S. cerevisiae. Cell 1997; 91:47-8; PMID:9335334; http://dx.doi.org/10.1016/S0092-8674(01)80008-8
- Losada A, Hirano M, Hirano T. Identification of Xenopus SMC protein complexes required for sister chromatid cohesion. Gene Dev 1998; 12:1986-97; PMID:9649503; http://dx.doi.org/10.1101/gad.12.13.1986
- Buonomo SBC, Clyne RK, Fuchs J, Loidl J, Uhlmann F, Nasmyth K. Disjunction of homologous chromosomes in meiosis I depends on proteolytic cleavage of the meiotic cohesin REC8 by separin. Cell 2000; 103:387-98; PMID:11081626; http://dx.doi.org/10.1016/S0092-8674(00)00131-8
- Herbert M, Kalleas D, Cooney D, Lamb M, Lister L. Meiosis and maternal aging: insights from aneuploid oocytes and trisomy births. CSH Perspect Biol 2015; 7:a017970
- Jones KT, Lane SI. Molecular causes of aneuploidy in mammalian eggs. Development 2013; 140:3719-3730; PMID:23981655; http://dx.doi.org/10.1242/dev.090589
- Eppig JJ. Coordination of nuclear and cytoplasmic oocyte maturation in eutherian mammals. Reprod Fert Develop 1996; 8:485-89; http://dx.doi.org/10.1071/RD9960485
- Uchiyama S, Fukui K. Condensin in Chromatid Cohesion and Segregation. Cytogenet Genome Res 2015; 147:212-16; PMID:26998746; http://dx.doi.org/10.1159/000444868
- Revenkova E, Eijpe M, Heyting C. Cohesin SMC1 beta is required for meiotic chromosome dynamics, sister chromatid cohesion and DNA recombination. Nat Cell Biol 2004; 6(6):555-62; PMID:15146193; http://dx.doi.org/10.1038/ncb1135
- Biswas U, Wetzker C, Lange J. Meiotic cohesin SMC1β provides prophase I centromeric cohesion and is required for multiple synapsis-associated functions. Plos Genet 2013; 9(12):e1003985; PMID:24385917; http://dx.doi.org/10.1371/journal.pgen.1003985
- Adelfalk C, Janschek J, Revenkova E. Cohesin Smc1βprotects telomeres in meiocytes. J Cell Biol 2009; 187(2):185-99; PMID:19841137; http://dx.doi.org/10.1083/jcb.200808016
- MacLennan M, Crichton JH, Playfoot CJ, Adams IR. Oocyte development, meiosis and aneuploidy. Semin Cell Dev Biol 2015; 45:68-6; PMID:26454098; http://dx.doi.org/10.1016/j.semcdb.2015.10.005
- Baudot A, de la Torre V, Valencia A. Mutated genes, pathways and processes in tumours. EMBO Rep 2010; 11:805-810; PMID:20847737; http://dx.doi.org/10.1038/embor.2010.133
- Yin S, Sun XF, Schatten H, Sun QY. Molecular insights into mechanisms regulating faithful chromosome separation in female meiosis. Cell Cycle 2008; 7:2997-05; PMID:18802407; http://dx.doi.org/10.4161/cc.7.19.6809
- Jordan P. Initiation of homologous chromosome pairing during meiosis. Biochem Soc T 2006; 34:545-49; http://dx.doi.org/10.1042/BST0340545
- Gruber S. Evidence that loading of cohesin onto chromosomes involves opening of its SMC hinge. Cell 2006; 127:523-37; PMID:17081975; http://dx.doi.org/10.1016/j.cell.2006.08.048
- Gutiérrez-Caballero C, Herrán Y, Sánchez-Martín M. Identification and molecular characterization of the mammalian α-kleisin RAD21L. Cell Cycle 2011; 10(9):1477-87; PMID:21527826; http://dx.doi.org/10.4161/cc.10.9.15515
- Hassold T, Hunt P. To err (meiotically) is human: the genesis of human aneuploidy. Nat Rev Genet 2001; 2:280-91; PMID:11283700; http://dx.doi.org/10.1038/35066065
- Wolstenholme J, Angell RR. Maternal age and trisomy–a unifying mechanism of formation. Chromosoma 2000; 109:435-38; PMID:11151672; http://dx.doi.org/10.1007/s004120000088
- Eichenlaub-Ritter U, Vogt E, Yin H, Gosden R. Spindles, mitochondria and redox potential in ageing oocytes. Reprod Biomed Online 2004; 8:45-8; PMID:14759287; http://dx.doi.org/10.1016/S1472-6483(10)60497-X
- Hodges CA, Revenkova E, Jessberger R, Hassold TJ, Hunt PA. Smc1βeta-deficient female mice provide evidence that cohesins are a missing link in age-related non-disjunction. Nat Genet 2005; 37:1351-55; PMID:16258540; http://dx.doi.org/10.1038/ng1672
- Subramanian VV, Bickel SE. Aging predisposes oocytes to meiotic non-disjunction when the cohesin subunit SMC1 is reduced. Plos Genet 2008; 4:e1000263; PMID:19008956; http://dx.doi.org/10.1371/journal.pgen.1000263
- Vogt E, Kirsch-Volders M, Parry J. Spindle formation, chromosome segregation and the spindle checkpoint in mammalian oocytes and susceptibility to meiotic error. Mutat Res-Gen Tox En 2008; 651(1):14-9; http://dx.doi.org/10.1016/j.mrgentox.2007.10.015
- Tachibana-Konwalski K, Godwin J, Borsos M. Spindle assembly checkpoint of oocytes depends on a Kinetochore structure determined by Cohesin in Meiosis I. Curr Biol 2013; 23(24):2534-9; PMID:24291092; http://dx.doi.org/10.1016/j.cub.2013.10.052
- Eijpe M, Offenberg H, Jessberger R, Revenkova E, Heyting C. Meiotic cohesin REC8 marks the axial elements of rat synaptonemal complexes before cohesins SMC1beta and SMC3. J Cell Biol 2003; 160:657-670; PMID:12615909; http://dx.doi.org/10.1083/jcb.200212080
- Revenkova E, Herrmann K, Adelfalk C, Jessberger R. Oocyte cohesin expression restricted to predictyate stages provides full fertility and prevents aneuploidy. Curr Biol 2010; 20:1529-33; PMID:20817531; http://dx.doi.org/10.1016/j.cub.2010.08.024
- Hirano T. Chromosome cohesion, condensation and separation. Annu Rev Biochem 2000; 69:115-44; PMID:10966455; http://dx.doi.org/10.1146/annurev.biochem.69.1.115
- Tachibana K, Godwin J, van der Weyden L, Champion L, Kudo NR, Adams DJ, Nasmyth K. Rec8-containing cohesin maintains bivalents without turnover during the growing phase of mouse oocytes. Gene Dev 2010 24; 2505-16; PMID:20971813; http://dx.doi.org/10.1101/gad.605910
- Miell MD, Straight AF. In vitro kinetochore assembly. Methods Mol Biol. 2016; 1413:111-33; PMID:27193846.
- Wynne DJ, Funabiki H. Kinetochore function is controlled by a phospho-dependent coexpansion of inner and outer components. J Cell Biol 2015; 210:899-16; PMID:26347137; http://dx.doi.org/10.1083/jcb.201506020
- Nijenhuis W, Vallardi G, Teixeira A, Kops G J, Sauri A T. Negative feedback at kinetochores underlies a responsive spindle checkpoint signal. Nat Cell Biol 2014; 16:1257-64; PMID:25402682; http://dx.doi.org/10.1038/ncb3065
- Moon HM. LIS1 controls mitosis and mitotic spindle organization via the LIS1-NDEL1-dynein complex. Hum Mol Genet 2014; 23:449-66; PMID:24030547; http://dx.doi.org/10.1093/hmg/ddt436
- Walczak CE, Heald R. Mechanisms of mitotic spindle assembly and function. Int Rev Cytol 2008; 265:111-158; PMID:18275887
- Rieder CL, Salmon ED. The vertebrate cell kinetochore and its roles during mitosis. Trends Cell Biol 1998; 8:310-18; PMID:9704407; http://dx.doi.org/10.1016/S0962-8924(98)01299-9
- Kleyman M, Kabeche L, Compton DA. STAG2 promotes error correction in mitosis by regulating kinetochore-microtubule attachments. J Cell Sci 2014; 127:4225-33; PMID:25074805; http://dx.doi.org/10.1242/jcs.151613
- Shomper M, Lappa C, FitzHarris G. Kinetochore microtubule establishment is defective in oocytes from aged mice. Cell Cycle 2014; 13:1171-79; PMID:24553117; http://dx.doi.org/10.4161/cc.28046