ABSTRACT
Previously, we have identified the Grainyhead transcription factor 2 gene (GRHL2) as notably hypomethylated in high-grade (HG) serous epithelial ovarian tumors, compared with normal ovarian tissues. GRHL2 is known for its functions in normal tissue development and wound healing. In the context of cancer, the role of GRHL2 is still ambiguous as both tumorigenic and tumor suppressive functions have been reported for this gene, although a role of GRHL2 in maintaining the epithelial status of cancer cells has been suggested. In this study, we report that GRHL2 is strongly overexpressed in both low malignant potential (LMP) and HG serous epithelial ovarian tumors, which probably correlates with its hypomethylated status. Suppression of the GRHL2 expression led to a sharp decrease in cell proliferation, migration and invasion and induced G1 cell cycle arrest in epithelial ovarian cancer (EOC) cells displaying either epithelial (A2780s) or mesenchymal (SKOV3) phenotypes. However, no phenotypic alterations were observed in these EOC cell lines following GRHL2 silencing. Gene expression profiling and consecutive canonical pathway and network analyses confirmed these data, as in both these EOC cell lines, GRHL2 ablation was associated with the downregulation of various genes and pathways implicated in cell growth and proliferation, cell cycle control and cellular metabolism. Taken together, our data are indicative for a strong oncogenic potential of the GRHL2 gene in EOC progression and support recent findings on the role of GRHL2 as one of the major phenotypic stability factors (PSFs) that stabilize the highly aggressive/metastatic hybrid epithelial/mesenchymal (E/M) phenotype of cancer cells.
Introduction
Epithelial ovarian cancer (EOC) accounts for 5% of all cancers in women and is the leading cause of death from gynecologic malignancies.Citation1 Despite treatment improvements, long-term survival rates for patients with advanced disease remain disappointing.Citation2 The molecular basis of EOC initiation and progression is still poorly comprehended. To establish novel therapeutic and diagnostic strategies against this deadly disease, it is essential to understand its molecular pathology. Recently, the importance of epigenetic perturbation of gene regulation in cancer,Citation3 including EOC,Citation4 has begun to be more fully appreciated. The most studied epigenetic alteration is DNA methylation, the addition of a methyl moiety to the cytosine-5 position within the context of a CpG dinucleotide, mediated by DNA methyltransferases.Citation3 Similar to other malignancies, aberrant DNA methylation occurs in EOC and contributes to ovarian tumorigenesis and mechanisms of chemoresistance.Citation4 Applying a more global array-based technology, several studies have demonstrated that DNA methylation changes in ovarian cancer are cumulative with disease progression and chemotherapy (CT) resistance.Citation5-7 Using a similar approach (methylated DNA immunoprecipitation coupled to CpG island tiling arrays) we have recently shown that DNA hypermethylation occurs in all (including less invasive/early) stages of ovarian tumorigenesis. In contrast, advanced EOC was exclusively associated with DNA hypomethylation of several oncogenes, implicated in EOC tumor invasion/metastasis and probably chemoresistance.Citation8 The GRHL2 gene was among the genes identified to be notably hypomethylated in high grade (HG) serous EOC tumors.Citation8 GRHL2 belongs to the Grainyhead transcription factor family (GRHL), which also includes GRHL1 and GRHL3. Grainyhead family factors have been shown to play important roles in neural tube closure,Citation9 wound healingCitation10 and epidermal formation.Citation11 In the context of cancer, the roles of GRHL proteins seem rather complex and even contradictory in some cases: depending on the cancer type, they may act as tumor suppressors or oncogenes.Citation12 Thus, accumulating data are indicative for an oncogenic role of GRHL2 in lung, oral, colorectal, liver and renal cancers,Citation13-17 while a loss or strong reduction in GRHL2 expression was shown to be associated with cervical carcinogenesis.Citation18 Interestingly, GRHL2 has shown both tumor-promoting and tumor suppressing action in breastCitation19 and gastricCitation20,21 cancers, as the reasons for these discrepancies in results from different studies are currently unclear.Citation19 Moreover, studies have shown an implication of GRHL2 in the maintenance of the epithelial phenotype of cancer cells by regulating the expression of the cell–cell adhesion receptor E-cadherin,Citation9,22,23 and by targeting ZEB1, a transcription factor known to modulate epithelial-to-mesenchymal transition (EMT).Citation15,19,24,25
The implication of GRHL2 in EOC etiology has not been profoundly studied. Only one report suggests for a role of GRHL2 in modulating EOC phenotype, as a gatekeeper function of GRHL2 has been postulated in maintaining the EOC epithelial status via transcriptional regulation of epithelial-specific miRNAs (miR200a/200b) and histone modifications.Citation26 This prompted us to further investigate if GRHL2 displays elevated expression levels in serous EOC tumors with different malignant potential, and whether this gene is functionally implicated in EOC tumorigenesis. In this study, we present experimental data indicative of GRHL2 overexpression in HG serous EOC tumors and LMP tumors when compared with normal tissues, which probably correlates with its hypomethylated status. We confirmed the strong GRHL2 expression in EOC cells with epithelial phenotype (A2780s); however GRHL2 expression was also detected in EOC cells bearing a mesenchymal phenotype (SKOV3). We also show that GRHL2 silencing resulted in inhibiting proliferation, migration and invasion and mediating cell cycle arrest in both these EOC cell lines, suggestive for a putative oncogenic role of the GRHL2 gene in EOC.
Results
Analysis of GRHL2 protein expression and DNA methylation status in serous EOC tumors
We initially evaluated GRHL2 protein expression by immunohistochemistry (IHC) in numerous serous EOC tumors and normal ovarian tissue samples, using tissue microarrays (TMAs). Our TMAs included triplicate cores of 117 serous EOC tumors, including 13 LMP and 104 HG tumors. Thirteen normal ovarian tissues samples were also included as controls. Table S1 shows the major clinical characteristics of these patients for whom extensive follow-up clinical data (up to 5-years) were available. The age ranged from 41 to 83 years (median: 66 years). High-grade tumors were all grade 3 (100%) including stage III (69%) and stage IV (31%) tumors. The majority of patients (93%) received a combination of platinum and paclitaxel. The median baseline CA125 was around 800. Forty percent of the patients had a progression or a recurrence within the first 6 months of follow-up; for 39 % of the patients the progression-free survival (PFS) interval was in the range of 7 to 24 months, and 21% of the patients displayed PFS values higher than 25 months. As seen in and , GRHL2 displayed significantly higher expression in LMP and HG serous EOC tumors compared with normal tissues (p = 0.0185 and p < 0.0001 respectively), which possibly correlates with the GRHL2 hypomethylation status in advanced disease. Indeed, alternative methylation analysis (using the bisulfite sequencing PCR, or BSP approach) in an independent set of serous HG EOC tumors, confirmed the hypomethylation status of the putative GRHL2 promoter region in the tumor samples compared with normal ovarian tissues (Figure S1), which is indicative for possible implications of epigenetic mechanisms in the control of GRHL2 expression in EOC. However, we did not observe any significant differences between the levels of GRHL2 expression and patients' PFS values (p = 0.739; see Figure S2A), which suggests that staining intensity for GRHL2 in pre-treatment surgical EOC specimens is not predictive of PFS. Similarly, GRHL2 expression displayed no correlation with PFS and overall survival (OS) upon analyzing the TCGA and the KM plotter human ovarian cancer data sets,Citation27,28 (accessible via http://www.cbioportal.org/ and www.kmplot.com/ovar Web portals; see Figures S2B-S2E).
Figure 1. Analysis of GRHL2 expression in serous EOC tumors by IHC. A. Representative IHC images of GRHL2 protein expression in normal ovarian tissues, low-malignant potential (LMP) tumors and high-grade (HG) tumors. B. Box-plot presentation of GRHL2 protein expression levels in normal ovarian tissues, LMP tumors and HG tumors. C. Western-blot analysis of endogenous GRHL2 protein expression in different EOC cell lines.
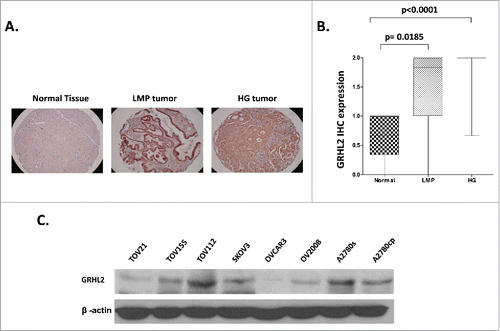
Modulation of GRHL2 expression in EOC cells: possible implications in EOC cell proliferation, cell cycle control, migration and invasion
Due to a previously described role of GRHL2 in suppressing the oncogenic EMT,Citation24 we decided to verify if GRHL2 gene depletion could produce any cancer-related phenotypic changes in the A2780s EOC cell line bearing an epithelial phenotype, as this cell line was among the EOC cell lines displaying strong endogenous GRHL2 expression (). The CRISPR/Cas9 system was used for the generation of stable A2780s GRHL2 knockout (KO) clones, as clone selection was based on Western blot validation of the GRHL2 protein expression levels in the selected clones, compared with control CRISPR/Cas9 clones. Two GRHL2 KO A2780s clones (A1 and A2) were selected for further analyses (Figure S3A), as the impact of the GRHL2 depletion was investigated on A2780s cell proliferation, cell cycle control, migration and invasion.
The GRHL2 gene ablation led to a sharp decrease in the number of viable adherent A2780s cells (represented by cell index), compared with control cells (). This observation was further supported by the reduced number of colony formation upon GRHL2 suppression ( and ). The GRHL2 depletion also induced G1 cell cycle arrest () which could explain the drastic reduction in the proliferation rates of these EOC cells observed earlier. Moreover, GRHL2 suppression significantly inhibited both migration and invasion of A2780s cells. Indeed and as shown in (migration) and (invasion), the numbers of A2780s cells that passed through the filter using the A1 and A2 A2780s KO clones were remarkably less than that in the negative control (Ctrl) clone.
Figure 2. Analyses of alterations in functional phenotypes upon CRISPR/Cas9-mediated GRHL2 knockout (KO) in A2780s cells. (A) effect of GRHL2 KO on cell proliferation. (B, C) Representative images from one of the 3 independent experiments showing migration (B, left) and invasion (C, left) in the control clone and GRHL2-KO clones A1 and A2 (at magnification ×400). The bar graphs in panels B (right) and C (right) are quantitative determinations of data obtained by selecting 10 random fields per filter (at magnification× 40) under phase contrast microscopy. Differences between control cells and KO cells were determined by a Student's t-test. Error bars denote ± SEM and *indicates statistical significance (p ≤ 0.05).
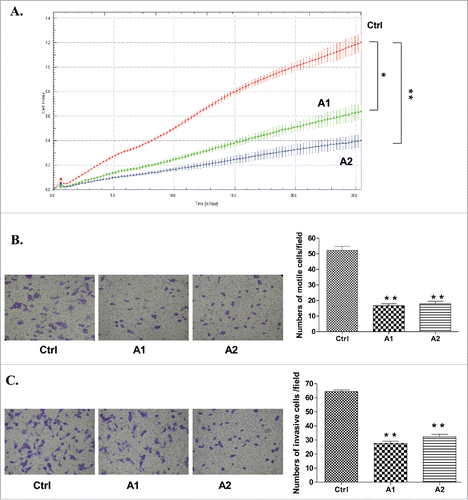
Figure 3. Effect of GRHL2 suppression on cell cycle control in EOC cells. (A) Effect of GRHL2 suppression on cell cycle control in A2780s cells. Cell-cycle profile was examined by flow cytometry and percentages of cells in G0/G1, S, and G2/M phase in the GRHL2 KO clone A1 were compared with the mock-transfected control (Ctrl) clone. Propidium iodide staining shows an increased fraction of cells in the G1-phase and a decrease of cells in the S-phase at 0 h, and especially at 6 and 12 h post hydroxyurea removal in the A1 clone, when compared with the control clone (Ctrl). (B) Effect of GRHL2 suppression on cell cycle control in SKOV3 cells. Cell-cycle profiles were examined by flow cytometry and percentages of cells in G0/G1, S, and G2/M phase in the shRNA-GRHL2 clone 2 (sh-S2) were compared with the mock-transfected control (Ctrl) clone. Propidium iodide staining shows a significantly increased fraction of cells in the G1-phase and a strong decrease of cells in the S-phase at 0, 6 and 12 h post hydroxyurea removal in the sh-S2 clone, when compared with the control clone (Ctrl).
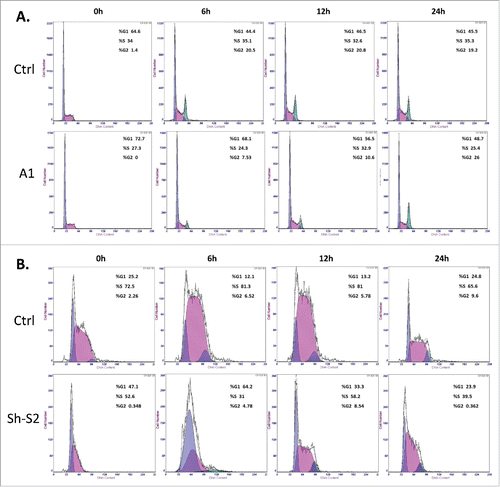
The mesenchymal-type SKOV3 EOC cell line was previously characterized as a cell line displaying no endogenous GRHL2 expression;Citation29 however, in our hands SKOV3 cells displayed intermediate GRHL2 expression levels (), which prompted us to modulate the GRHL2 expression in this cell line (both suppression and overexpression). As we were not successful in generating GRHL2 KO clones in SKOV3 cells using the CRISPR/Cas9 methodology, we consecutively used the short hairpin RNA (shRNA) approach for stable GRHL2 knockdown (KD) in SKOV3 cells. Clone selection for further analyses was based on qPCR and Western blot validation of the GRHL2 mRNA/protein expression levels in selected clones, compared with empty vector-transfected clones. Among the clones analyzed, the shRNA-GRHL2 KD clones 2 (sh-S2) and 5 (sh-S5) displayed a significant decrease in GRHL2 mRNA and protein expression levels compared with the mock-transfected control (see Figures S3B and S3C), and were selected for further analyses. In concordance with our results obtained in GRHL2 KO A2780s cells, SKOV3 GRHL2 KD clones S2 and S5 exhibited decreased proliferation (), which was consecutively confirmed by a similar, and even much stronger effect on the regulation of the cell cycle (arrest in G1; see ). Similarly, SKOV3 GRHL2 KD clones also displayed lower migration and invasion capacities, when compared with control cells (see and ).
Figure 4. Analyses of alterations in functional phenotypes upon shRNA-mediated GRHL2 knockdown (KD) in SKOV3 cells. (A) Effect of GRHL2 KD clones on cell proliferation compared with the control clone (B, C) Representative images from one of the 3 independent experiments showing migration (B, left) and invasion (C, left) in the control clone and GRHL2-knockdown clones sh-S2 and sh-S5 (at magnification ×400). The bar graphs in panels B (right) and C (right) are quantitative determinations of data obtained by selecting 10 random fields per filter (at magnification× 40) under phase contrast microscopy. Differences between control cells and KD cells were determined by a Student's t-test. Error bars denote ± SEM and *indicates statistical significance (p ≤ 0.05).
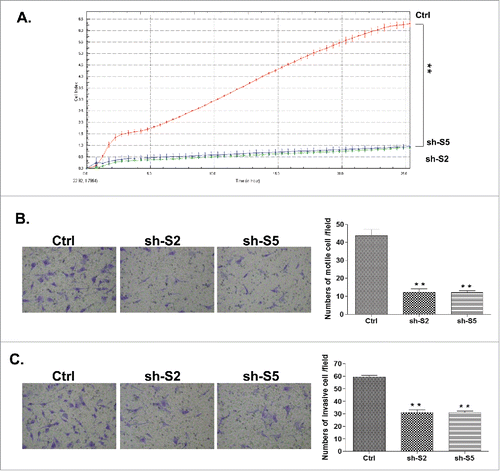
In parallel, GRHL2 was ectopically expressed in SKOV3 cells, and the selection of one GRHL2 stably overexpressing clone (clone pCMV-GRHL2) was confirmed by Western blot analysis (Figure S3C). Analogous experiments performed with clone pCMV-GRHL2 displayed no significant effects of GRHL2 overexpression on SKOV3 cell proliferation, cell cycle control, migration and invasion rates (see Figures S4A-F), suggesting that the endogenous GRHL2 expression in this cell line is sufficient for modulating these cellular functions.
We also found that the suppression of GRHL2 expression was not associated with any changes in E-cadherin expression in A2780s cells and ZEB1 expression in SKOV3 cells (see Figures S3A and S3C), as parental and GRHL2 KO A2780s cells displayed no ZEB1 expression and accordingly, E-cadherin was not expressed in parental and GRHL2 KD SKOV3 cells. Additionally, no EMT-related phenotypic alterations were observed in both cell lines following GRHL2 KO/KD (data not shown). However, GRHL2 silencing in both A2780s and SKOV3 cell lines directed the downregulation of the EOC oncogene ErbB3,Citation30 as previously shown in EOC and breast cancer.Citation26,29 (see Figures S3A and S3C).
Finally, modulations of GRHL2 expression had no significant impact on cisplatin and paclitaxel sensitivity in A2780s and SKOV3 cells (see Figure S5).
Molecular mechanisms of GRHL2 action in EOC cells
To better understand the molecular mechanisms of GRHL2 action in EOC cells, we used the Agilent Whole Human Genome 4×44K microarrays (containing 44,000 genes) to identify global gene expression changes upon GRHL2 KO in A2780s cells and GRHL2 expression modulation (suppression or overexpression) in SKOV3 cells. The gene expression patterns of the previously selected clones were compared against the corresponding control clone. All microarray experiments were performed in duplicates, as two hybridizations were performed for each GRHL2 suppressing/overexpressing cell clone against the corresponding control, using a fluorescent dye reversal (dye-swap) technique. For all comparisons, a subset of common differentially expressed genes was selected by initial filtering on confidence at p-value ≤ 0.05, followed by filtering on expression level (≥ 1.5-fold). Using these selection criteria, we found 478 genes to be upregulated and 701 genes to be downregulated in A2780s cells following GRHL2 KO (Table S3), while 544 genes were found to be upregulated and 542 genes to be downregulated in SKOV3 cells following GRHL2 KD, (Table S4), and 1051 genes were upregulated and 1081 were downregulated in SKOV3 upon GRHL2 overexpression (Table S5).
Consecutive canonical pathway and network analyses generated through the use of the Ingenuity Pathway Analysis (IPA) software sustained the cancer-related phenotypic changes in A2780s and SKOV3 cells following GRHL2 suppression. Thus, the top downregulated canonical pathways in A2780s cells were mostly related to cell metabolism and energy production (oxidative phosphorylation, mitochondrial dysfunction), cellular growth and proliferation (TGF-β signaling, human ES cell pluripotency) and cell death and survival (induction of apoptosis by HIV1) (see ), while upregulated canonical pathways were predominantly associated with cellular function and maintenance (aldosterone signaling in epithelial cells, ILK signaling, ephrin B signaling), cell death and survival (sphingosine-1-phosphate signaling) and cell cycle regulation (glioblastoma multiforme signaling) (see ). GRHL2 knockdown displayed a much more profound effect in SKOV3 cells, as numerous oncogenic canonical pathways, implicated in control cellular growth and proliferation, were significantly downregulated (including ERK/MAPK, HGF, IGF-1, phospholipase C, B cell receptor, CRH, Tec kinase, IL-6, IL-8, PI3K/AKT, IL1, Integrin, oncostatin M, EGF and TGF-β signaling pathways; see ). GRHL2 ablation in SKOV3 cells also correlated with predominant upregulation and/or modulation of cell cycle control canonical pathways (aryl hydrocarbon receptor signaling, G2/M DNA damage checkpoint regulation, mitotic roles of Polo-like kinase, estrogen-mediated cell cycle regulation, G1/S checkpoint regulation), as well as some metabolism-related pathways (endocytosis signaling and phagosome maturation) (see ). Interestingly, GRHL2 overexpression in SKOV3 cells was exclusively associated with prevalent downregulation of cell cycle control-related canonical pathways (), as identical pathways (including mitotic roles of Polo-like kinase, G2/M DNA damage checkpoint regulation, aryl hydrocarbon receptor signaling, estrogen-mediated cell cycle regulation) were reversibly regulated upon GRHL2 KD, compared with GRHL2 overexpression. GRHL2 overexpression in SKOV3 cells also resulted in the modulation of pathways implicated in cholesterol and amino-acid metabolism and cellular immune/inflammatory response (interferon signaling) (). Importantly, the above data confirmed our cell cycle functional analyses and are strongly suggestive for a role of GRHL2 in cell cycle regulation of EOC cells, where knocking down GRHL2 inhibited G1/S cell cycle progression which was more prominently evident in the cells displaying a mesenchymal phenotype.
Figure 5. Top canonical pathways that were significantly dysregulated upon GRHL2 knockout (KO) in A2780s cells. A. Canonical pathways associated with upregulated genes upon GRHL2 KO; B. Canonical pathways associated with downregulated genes upon GRHL2 KO. Top functions are displayed that meet the Benjamini-Hochberg (B-H) multiple testing correction p-value of 0.05.
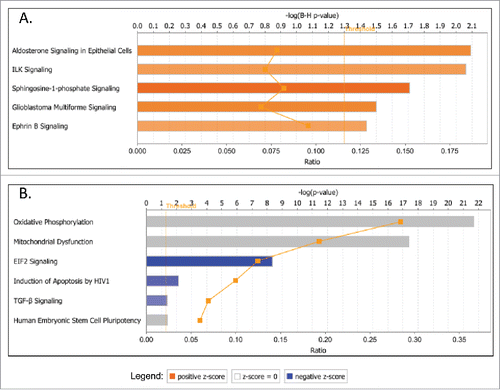
Figure 6. Top canonical pathways that were significantly dysregulated upon GRHL2 knockdown (KD) in SKOV3 cells. A. Canonical pathways associated with upregulated genes upon GRHL2 KD; B. Canonical pathways associated with downregulated genes upon GRHL2 KD. Top functions are displayed that meet the B-H multiple testing correction p-value of 0.05.
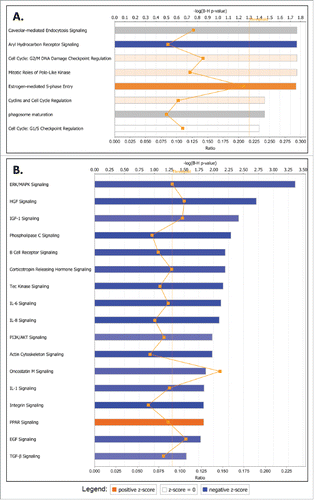
Figure 7. Top canonical pathways that were significantly dysregulated upon GRHL2 overexpression in SKOV3 cells. A. Canonical pathways associated with upregulated genes upon GRHL2 overexpression; B. Canonical pathways associated with downregulated genes upon GRHL2 overexpression. Top functions are displayed that meet the B-H multiple testing correction p-value of 0.05.
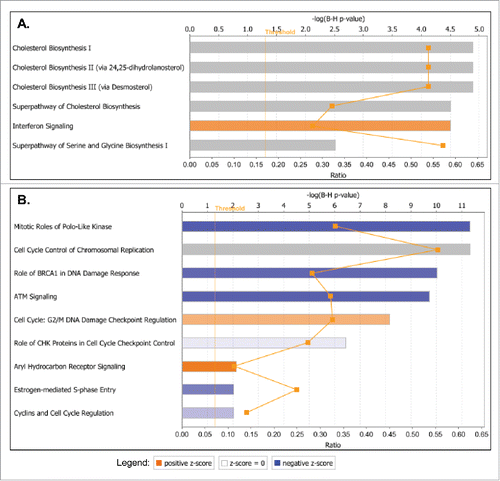
Common IPA networks, obtained upon merging the 4 top-scoring networks following GRHL2 suppression were indicative for some important gene nodes linked to GRHL2 suppression in EOC cells, as most of these substantiate and/or complement the functional data obtained. As shown in Figure S6, GRHL2 KO in A2780s cells led to a strong downregulation of several gene nodes known to be implicated in EOC tumorigenesis including RAN, RPA3, RPLP1, PAPP-A, RCE1, and SNRPE (Figure S6). Likewise, several gene nodes with proven EOC oncogenic function (including SMAD3, RUNX2, CTGF, INHBA, NAMPT, VEGF, ATF4, ETS1 and ITGA4) were downregulated upon GRHL2 suppression in SKOV3 cells (Figure S7). A number of other oncogene nodes were also found to be downregulated following GRHL2 suppression in A2780s cells (including CUL5, LSM5, RPA4, OGT, SNRNP35, ICT1 and MRPL39) and SKOV3 cells (including HMOX1, APP, TRIB3, JUNB, PLOD2 and PTX3) (see Figures S6 and S7).
Validation of microarray findings with quantitative PCR (qPCR)
To validate microarray results, we arbitrarily selected 8 differentially expressed genes from each of the 3 experimental conditions (GRHL2 KO in A2780s cells, GRHL2 KD and GRHL2 overexpression in SKOV3 cells) and quantified their expression by qPCR upon comparison to the corresponding controls. Figure S8 summarizes the gene expression measurements of all validated genes. We found that both methods (microarray analysis and qPCR) detected similar patterns for the up- and downregulated genes selected for validation.
Discussion
The mechanisms for the tumorigenesis, progression and biologic aggressiveness of EOC have not been yet fully clarified. In the present study, we demonstrated that GRHL2 is overexpressed in LMP and HG serous ovarian tumors as compared with normal ovarian tissues. Similarly, a recent analysis was also indicative for the significant overexpression of GRHL2 in serous EOC compared with normal ovarian tissuesCitation29 In addition, GRHL2 gene is located in the chromosomal region 8q22.3, and this region is frequently amplified in many cancers, including ovarian cancer.Citation31 Applying an independent (bisulfite sequencing PCR, or BSP) approach, we also confirmed the hypomethylation status of the putative GRHL2 gene promoter region in HG EOC tumors, suggestive for epigenetic regulation of GRHL2 expression in late disease. Indeed, a role of DNA methylation in regulating GRHL2 expression during EMT has been previously demonstrated.Citation32 Moreover, our functional analyses are strongly indicative for evident oncogenic capacity of GRHL2 in EOC, including its potential implication in EOC cell proliferation, cell cycle control, migration and invasion in EOC cell lines displaying both epithelial (A2780s) and mesenchymal (SKOV3) phenotypes, in concordance with similar findings on the role of GRHL2 in other cancers of promoting tumor growth and metastasis.Citation15,16,19,20,23,31,33-35 Microarray experiments were highly supportive of these findings, as in both cell lines, GRHL2 suppression was mostly associated with the downregulation of cellular growth and proliferation canonical pathways, with a more profound effect observed in SKOV3 cells, as some metabolism and energy production pathways (including oxidative phosphorylation and mitochondrial dysfunction) were also downregulated in A2780s cells ( and ). Likewise, GRHL2 overexpression in SKOV3 cells correlated with the overexpression of metabolism-related pathways that were mostly coupled with cholesterol biosynthesis (). The later results were not unexpected due to a previously demonstrated role of GRHL2 in modulating intracellular metabolism, including mitochondrial oxidative function and glutaminolysis.Citation36 Moreover, alterations in GRHL2 expression were strongly related to modulations in cell cycle control-related canonical pathways, which were especially evident in SKOV3 cells, as several cell cycle control pathways were reversibly regulated following GRHL2 suppression or overexpression in this cell line. Similar implications of GRHL2 in controlling cell cycle were shown when characterizing its oncogenic function in colorectal cancer.Citation15,37 Additionally, network analysis was indicative for several gene nodes with oncogenic (including EOC oncogenic) function that were downregulated in both cell lines upon GRHL2 suppression (Figures S6 and S7).
Literature data concerning the role of GRHL2 in EOC etiology are rather scarce; only one report by Chung et al. was indicative for an implication of GRHL2 in maintaining the epithelial phenotype of EOC cells.Citation26 Similar to their findings, we found that mesenchymal EOC cells (SKOV3) express lower GRHL2 levels compared with EOC cell bearing the epithelial phenotype (A2780s; see ), and that GRHL2 expression in EOC cells positively correlated with the expression of the EOC oncogene ErbB3, while GRHL2 suppression was not associated with any changes in ZEB1 and E-cadherin expression (Figures S3A and S3C). However, by applying gap closure migration assays, Chung et al. showed that GRHL2 suppression in EOC cells with an epithelial/intermediate epithelial (E/IE) phenotype (PEO1, OVCA420, OVCA429) resulted in increased speed of individual cell locomotion, although the observed changes in cell migration/invasion were not due to increased cell proliferation.Citation26 Moreover, based on the transcriptomic data deposited in the Cancer Cell Line Encyclopedia (CCLE),Citation38 and using their previously developed EMT scoring scheme from cancer-specific transcriptomic EMT signatures,Citation39 Chung et al. found that in EOC patients, GRHL2-high expression tumors showed better OS as compared with GRHL2-low expression tumors,Citation26 suggestive for a potential tumor suppressor role of this gene in EOC etiology. Again, and similar to the GRHL2 data accumulated in other cancer types, their results are rather contradictory to our data, since our analyses, as well as consecutive in silico analyses based both on the TCGA and the KM plotter human ovarian cancer data sets.Citation27,28 displayed no association of GRHL2 expression with PFS and/or OS of EOC patients.
However, these discrepancies found in GRHL2 function in different cancers, and even in the same cancer type, could be explained by some recent findings on the role of GRHL2 as one of the major phenotypic stability factors (PSFs) that stabilize the hybrid epithelial/mesenchymal (E/M) phenotype of cancer cells.Citation13 Numerous studies have previously suggested that EMT and its reverse process mesenchymal to epithelial transition (MET) play central roles in tumor invasion and metastasis (reviewed in refs. Citation40-45). This gene program permits epithelial cells to acquire mesenchymal properties with augmented migratory ability. The migrating cancer cells then undergo a reverse MET, which allows the mesenchymal cells to re-differentiate into epithelial structures and re-acquire cell–cell contacts and polarity, needed for enhanced colonization of secondary sites.Citation46-50 However, this EMT/MET model is now strongly debated, since it has been lately shown that circulating tumor cells can form clusters containing cells with both epithelial/mesenchymal or hybrid E/M phenotype that display higher metastatic potential and increased drug resistance compared with either epithelial or mesenchymal cells alone.Citation51-53 Moreover, recent experiments demonstrated that a hybrid E/M phenotype can be stable both in vitro and in vivo,Citation54-56 and that breast and ovarian cancer metastases are mainly driven by hybrid E/M cells.Citation57,58 Thus in cancer, GRHL2 might not simply represent an EMT repressor as previously suggested,Citation24,26,59 but could rather function as a PSF for the hybrid E/M (or partial EMT) cellular phenotype, together with some other recently identified PSFs, including OVOL2 and miR-145.Citation13,60 Interestingly, OVOL2 was previously characterized as a GRHL2 target gene during epithelial morphogenesis;Citation61-63 however, it was later demonstrated that OVOL2 and GRHL2 act independently as PSFs for hybrid E/M cells,Citation13 and we found no changes in OVOL2 expression upon GRHL2 suppression in EOC cells (data not shown).
GRHL2 KD in lung cancer cells bearing a hybrid E/M phenotype led to the suppression of cell proliferation and collective cell migration (the hallmark of partial EMT),Citation13 quite similar to our data obtained upon GRHL2 suppression in EOC cells. This is not surprising since both our EOC cells used (SKOV3 and A2780s) bear some features of the hybrid E/M phenotype. Indeed, the SKOV3 cell line has been morphologically categorized as either mesenchymal or epithelial cell lineCitation64 and the A2780s cell line has been characterized with intermediate (round) cellular phenotype.Citation64 In our hands, the SKOV3 line displayed a typical spindle-like morphology and predominant expression of mesenchymal markers, although a strong expression of the epithelial marker EPCAM was also observed in this cell line.Citation65 Similarly, the A2780s cell line displayed an E/IE (round) phenotype accompanied with robust expression of epithelial markers;Citation65 however, a significant expression of the mesenchymal marker vimentin was also found in this cell line (data not shown). In contrast, the EOC cell lines used by Chung et al. were bearing mostly the epithelial phenotype, which could explain the discrepancies between their and our data when analyzing the GRHL2 function in EOC cells.
In conclusion, we have shown that the GRHL2 transcription factor is significantly overexpressed in LMP and especially in HG serous EOC tumors, as compared with normal ovarian tissue. Further validation of the GRHL2 methylation status in EOC tumors was indicative for a possible implication of epigenetic mechanisms in GRHL2 overexpression in metastatic tissues. Consecutive functional analyses of GRHL2 in EOC cells pointed toward its association with EOC cell proliferation, cell cycle control, migration and invasion. Our data support recent findings for the role of GRHL2 as a putative PSF of the more aggressive and highly metastatic hybrid E/M cancer cell phenotype. Hence, GRHL2 is possibly required for EOC tumor and cancer cell growth and invasion, and could represent a potential EOC therapeutic target. Further in vitro and in vivo studies are warranted to more completely elucidate the functional implications of the GRHL2 gene in ovarian tumorigenesis.
Patients and methods
Patients and tissue specimens
Snap frozen and formalin-fixed paraffin-embedded (FFPE) tissues of 117 serous EOC tumors were provided by the Banque de tissus et de données of the Réseau de recherche sur le cancer of the Fonds de recherche du Québec - Santé at the Hotel-Dieu de Quebec Hospital, Quebec, Canada, which is affiliated with the Canadian Tumor Repository Network. These clinical specimens included 13 borderline, or LMP tumors, 52 HG adenocarcinomas and 52 omental metastases. None of the patients received chemotherapy before surgery (see Table S1 for detailed clinicopathological characteristics). All tumors were histologically classified according to the criteria defined by the World Health Organization.Citation66 The CT treatment was completed for all patients and the response to treatment was known. Disease progression was evaluated following the guidelines of the Gynecology Cancer Intergroup.Citation66 Progression free survival (PFS) was defined as the time from surgery to the first observation of disease progression, recurrence or death. Thirteen normal ovarian samples were derived from women subjected to hysterectomy with oophorectomy due to non-ovarian pathologies. This study was approved by the Clinical Research Ethics Committee of the Hotel-Dieu de Quebec Hospital and patients gave written consent for tissue collection and analyses.
Cell cultures
The EOC cell lines OVCAR3 and SKOV3 were purchased from the American Tissue Type Collection (Manassas, VA); OV2008, TOV-112 and TOV-21 cell lines were a kind gift from Dr. Anne-Marie Mes-Masson (Université de Montréal), while A2780s and A2780cp cell lines were a kind gift from Dr. Benjamin Tsang (University of Ottawa). The TOV-155 cell line was recently established in our laboratory as spontaneously immortalized EOC cell line from poorly differentiated serous EOC tumor and the detailed characterization of this cell line will be reported elsewhere. The cell lines were passed in different culture media supplemented with 10% fetal bovine serum, as described previously.Citation65,67
Bisulfite sequencing PCR (BSP) analysis
BSP analysis was performed, as described previously.Citation65,67 Briefly, genomic DNA extracted from EOC tumors and control ovarian tissues was isolated using the Qiagen DNeasy Blood and Tissue Kit. Bisulfite modification of genomic DNAs was done using the Methyl Detector kit (Active Motif, Carlsbad, CA). For BSP, a 327, 281 and 280 bp fragments were amplified using primer pairs specific for bisulfite modified sequences but not harboring CpGs (Table S2). Two fragments are located at nt −734 to nt −407, and nt −306 to nt −25 downstream of the GRHL2 transcription start codon and the third at nt +437 to nt +717 upstream of ATG codon (see Figure S1). BSP primer selection was performed using the Methyl Primer Express Software v1.0 (Applied Biosystems). PCR was done for 30 cycles (94°C, 45 s; 60°C, 45 s; 72°C, 45 s). PCR products were sent for dideoxy-sequencing analysis at the Genomics Analysis Platform at Laval University (http://www.bioinfo.ulaval.ca/seq/en/).
Tissue microarrays (TMAs) construction and immunohistochemistry (IHC)
TMA construction and IHC were performed as described previously.Citation65,67 Sections were incubated with anti-GRHL2 antibody (1:100 dilution) (GenTex) at 4°C overnight and GRHL2 protein expression was assessed by semi-quantitative scoring of the intensity of staining and recorded as absent (0), weak (1+), moderate (2+) or strong (3+). The relationship between GRHL2 expression in serous ovarian carcinomas and normal ovarian tissues was evaluated by the Mann-Whitney test. A significant association was considered when p-value was below 0.05. A Kaplan Meier curve and the log-rank test were performed based on PFS values to test the effect of the intensity of GRHL2 (3, 2 versus 0, 1) on disease progression.
CRISPR/Cas9 mediated GRHL2 knockout in A2780s cells
CRISPR/Cas9 mediated GRHL2 knockout in A2780s cells was done according to the manufacturer's guidelines (Santa Cruz Biotechnology, Inc.), as described previously.Citation65 Briefly, 1.5×105 cells were plated onto 6×30-mm well plates and allowed to grow to 70% confluence. Five microliters of UltraCruz Transfection Reagent (sc-395739) were added to 2 μg of GRHL2 CRISPR/Cas9 KO Plasmid (sc-408284) and 2 μg of GRHL2 HDR Plasmid (sc-408284-HDR). The complexes were incubated at room temperature for 20 min and then overlaid onto the cells. The plate was then incubated at 37°C, 5% CO2 for 48 h. Stably transfected clones were selected by adding puromycin (1 µg/ml). Selected clones were transfected with Cre vector (sc-418923) for the removal of genetic material flanked by LoxP sites. Control CRISPR/Cas9 Plasmid (sc-418922) containing a non-targeting 20 nt scramble guide RNA (gRNA) was used as a negative control.
Short hairpin RNA (shRNA) - mediated GRHL2 knockdown in SKOV3 cells
The shRNA-mediated GRHL2 knockdown in SKOV3 cells was performed, as described previously.Citation65 Briefly, GRHL2 shRNA cloned into the pLKO.1-puro vector (targeting the GRHL2 mRNA sequence 5′-GCCTTCAAAGCAGATGAAAGAA-3′) was retrieved from the Sigma Mission TRC human 1.5 shRNA library (clone number TRCN0000015809). Viral supernatants were generated by transfecting 293T cells with the shRNA constructs and the packaging vectors psPAX2 and pMD2.G (Addgene, Cambridge, MA). The high-titer lentiviral supernatants in the presence of 8 mg/ml polybrene were used to infect SKOV3 cells. Two days later, infected cells were treated with puromycin (0.5 μg/ml) for the selection of stably-transduced clones. The pLKO.1-puro vector encoding a scramble sequence not matching any mammalian sequence was used for the generation of mock-transduced (control) clones. Stable clones with inhibited GRHL2 expression were evaluated and validated by quantitative RT-PCR and Western blot.
Ectopic GRHL2 expression in SKOV3 cells
For GRHL2 ectopic expression, human GRHL2 cDNA cloned in the pCMV6 entry eukaryotic expression vector (pCMV-GRHL2) was purchased from OriGene Technologies, Inc. (Rockville, MD). Transfection with ExGen 500 (Fermentas Canada Inc., Burlington ON) was performed according to the manufacturer's guidelines. Briefly, 1×105 SKOV3 cells were plated onto 6×30-mm well plates and allowed to grow to 70% confluence. Ten microliters of ExGen 500 were added to 2 µg of plasmid DNA dissolved in 190 µl of 150 mM NaCl. The complexes were incubated at room temperature for 10 min and then overlaid onto the cells in 1.8 ml medium. The plates were then incubated at 37°C, 5% CO2 for 48 h. Stably transfected clones were selected by adding neomycin (500 µg/ml) and further cultivation for about 2 weeks. Cells were also mock-transfected with the empty pCMV6 vector, and stably transfected clones were isolated as controls. The GRHL2 expression level of selected GRHL2-overexpressing and control clones was confirmed by Western blot.
Western blot analysis
Western blot analysis was performed as described previously.Citation65,67 The following antibodies were used for monitoring protein expression: anti-GRHL2 (GenTex), anti-ErbB3, anti-ZEB1, anti-OVOL2, anti-vimentin and anti-β-actin (Santa Cruz Biotechnology), the latter used as internal standard.
Functional assays
Different functional assays, including real time cell proliferation monitoring (using the xCELLigence Real-Time Cell Analyzer), cell migration and invasion assays were performed as previously shown.Citation65,67
Flow cytometry
Flow cytometer analysis was performed, as previously shown.Citation67,68 Briefly, 7.5 × 104 SKOV3 Ctrl, SKOV3 GRHL2 KD, SKOV3 pCMV-GRHL2, A2780s ctrl, and A2780s GRHL2 KO, cells were treated with 20 mM hydroxyurea (Sigma) for synchronization at the G1/S boundary. After 16 h of incubation, cells were washed once with PBS and resuspended in 1 ml of complete media (time 0). Then, cells were harvested by trypsinization at 0, 6, 12 and 24 h, washed 3 times with ice-cold PBS and fixed with ice-cold 95% ethanol overnight. Cells were washed with PBS (3×) and incubated with propidium iodide (PI) solution, containing 50 μg/ml of PI (Sigma) dissolved in 0.1% sodium citrate (Sigma), 1mg/ml RNAse (Sigma) and 0.1% Nonidet P40 (Sigma), and samples were left in the dark at room temperature for 60 min. Flow cytometric analysis was performed on a Beckman Coulter EPICS XL-MCL analyzer. The cell cycle phase distribution was calculated from the resultant DNA using the cell QuesPro software.
MTS cell cytotoxicity assay
A CellTiter 96 One Solution cell proliferation assay (MTS assay) was used according to the manufacturer's instructions (Promega) to measure the cell growth inhibition effects of cisplatin and paclitaxel in A2780s GRHL2 KO clones, SKOV3 GRHL2 KD clones and SKOV3 pCMV-GRHL2 overexpression clones. The cells were seeded (at 2 × 104 cells/ml) on 96-well plates in triplicates and incubated for 72 hours with the different drugs used with concentrations ranging between 0.01 µM to 100 µM. After 72 hours, 20 µl of the CellTiter 96 One Solution from the kit was added to the culture medium containing the samples. The plates are then incubated for 1 to 4 hours at 37°C in a humidified, 5% CO2 incubator. The absorbance values (490 nm) were recorded with a plate reader and the relative cell numbers were then calculated.
Gene expression profiling and data analysis
Gene expression profiling and consecutive microarray data analyses were performed using the Agilent Whole Human Genome 4 × 44K microarrays (containing 44, 000 genes), as described before.Citation65,67 Pathway and network analysis of the microarray data was completed using the Ingenuity Pathway Analysis (IPA) software (see http://www.Ingenuity.com). The microarray data have been deposited to the GEO database (http://www.ncbi.nlm.nih.gov/geo/) with accession number GSE90132.
Quantitative PCR (qPCR)
Quantitative PCR was performed as described previously using the primers listed in Table S2.Citation65,67
Disclosure of potential conflicts of interest
No potential conflicts of interest were disclosed.
1295181_Supplemental_Material.zip
Download Zip (5.5 MB)Acknowledgements
This study was sustained by a grant to D.B. from the Cancer Research Society of Canada. Clinical specimens were provided by the Banque de tissus et de données of the Réseau de recherche sur le cancer of the Fonds de recherche du Québec - Santé (FRQ-S), associated with the Canadian Tumor Repository Network (CTRNet).
References
- Siegel RL, Miller KD, Jemal A. Cancer statistics, 2015. CA Cancer J Clin 2015; 65:5-29; PMID:25559415; http://dx.doi.org/10.3322/caac.21254
- Marchetti C, Pisano C, Facchini G, Bruni GS, Magazzino FP, Losito S, Pignata S. First-line treatment of advanced ovarian cancer: Current research and perspectives. Expert Rev Anticancer Ther 2010; 10:47-60; PMID:20014885; http://dx.doi.org/10.1586/era.09.167
- Jones PA, Baylin SB. The epigenomics of cancer. Cell 2007; 128:683-92; PMID:17320506; http://dx.doi.org/10.1016/j.cell.2007.01.029
- Balch C, Fang F, Matei DE, Huang TH, Nephew KP. Minireview: Epigenetic changes in ovarian cancer. Endocrinology 2009; 150:4003-11; PMID:19574400; http://dx.doi.org/10.1210/en.2009-0404
- Bauerschlag DO, Ammerpohl O, Brautigam K, Schem C, Lin Q, Weigel MT, Hilpert F, Arnold N, Maass N, Meinhold-Heerlein I, et al. Progression-free survival in ovarian cancer is reflected in epigenetic DNA methylation profiles. Oncology 2011; 80:12-20; PMID:21577013; http://dx.doi.org/10.1159/000327746
- Watts GS, Futscher BW, Holtan N, Degeest K, Domann FE, Rose SL. DNA methylation changes in ovarian cancer are cumulative with disease progression and identify tumor stage. BMC Med Genomics 2008; 1:47; PMID:18826610; http://dx.doi.org/10.1186/1755-8794-1-47
- Li M, Balch C, Montgomery JS, Jeong M, Chung JH, Yan P, Huang TH, Kim S, Nephew KP. Integrated analysis of DNA methylation and gene expression reveals specific signaling pathways associated with platinum resistance in ovarian cancer. BMC Med Genomics 2009; 2:34; PMID:19505326; http://dx.doi.org/10.1186/1755-8794-2-34
- Keita M, Wang ZQ, Pelletier JF, Bachvarova M, Plante M, Gregoire J, Renaud MC, Mes-Masson AM, Paquet ER, Bachvarov D. Global methylation profiling in serous ovarian cancer is indicative for distinct aberrant DNA methylation signatures associated with tumor aggressiveness and disease progression. Gynecol Oncol 2013; 128:356-63; PMID:23219462; http://dx.doi.org/10.1016/j.ygyno.2012.11.036
- Pyrgaki C, Liu A, Niswander L. Grainyhead-like 2 regulates neural tube closure and adhesion molecule expression during neural fold fusion. Dev Biol 2011; 353:38-49; PMID:21377456; http://dx.doi.org/10.1016/j.ydbio.2011.02.027
- Moussian B, Uv AE. An ancient control of epithelial barrier formation and wound healing. Bioessays 2005; 27:987-90; PMID:16163734; http://dx.doi.org/10.1002/bies.20308
- Senga K, Mostov KE, Mitaka T, Miyajima A, Tanimizu N. Grainyhead-like 2 regulates epithelial morphogenesis by establishing functional tight junctions through the organization of a molecular network among claudin3, claudin4, and Rab25. Mol Biol Cell 2012; 23:2845-55; PMID:22696678; http://dx.doi.org/10.1091/mbc.E12-02-0097
- Mlacki M, Kikulska A, Krzywinska E, Pawlak M, Wilanowski T. Recent discoveries concerning the involvement of transcription factors from the Grainyhead-like family in cancer. Exp Biol Med (Maywood) 2015; 240:1396-401; PMID:26069269; http://dx.doi.org/10.1177/1535370215588924
- Jolly MK, Tripathi SC, Jia D, Mooney SM, Celiktas M, Hanash SM, Mani SA, Pienta KJ, Ben-Jacob E, Levine H. Stability of the hybrid epithelial/mesenchymal phenotype. Oncotarget 2016; 7:27067-84. PMID:27008704; http://dx.doi.org/10.18632/oncotarget.8166
- Chen W, Yi JK, Shimane T, Mehrazarin S, Lin YL, Shin KH, Kim RH, Park NH, Kang MK. Grainyhead-like 2 regulates epithelial plasticity and stemness in oral cancer cells. Carcinogenesis 2016; 37:500-10; PMID:26933170; http://dx.doi.org/10.1093/carcin/bgw027
- Quan Y, Jin R, Huang A, Zhao H, Feng B, Zang L, Zheng M. Downregulation of GRHL2 inhibits the proliferation of colorectal cancer cells by targeting ZEB1. Cancer Biol Ther 2014; 15:878-87; PMID:24756066; http://dx.doi.org/10.4161/cbt.28877
- Tanaka Y, Kanai F, Tada M, Tateishi R, Sanada M, Nannya Y, Ohta M, Asaoka Y, Seto M, Shiina S, et al. Gain of GRHL2 is associated with early recurrence of hepatocellular carcinoma. J Hepatol 2008; 49:746-57; PMID:18752864; http://dx.doi.org/10.1016/j.jhep.2008.06.019
- Butz H, Szabo PM, Nofech-Mozes R, Rotondo F, Kovacs K, Mirham L, Girgis H, Boles D, Patocs A, Yousef GM. Integrative bioinformatics analysis reveals new prognostic biomarkers of clear cell renal cell carcinoma. Clin Chem 2014; 60:1314-26; PMID:25139457; http://dx.doi.org/10.1373/clinchem.2014.225854
- Torres-Reyes LA, Alvarado-Ruiz L, Pina-Sanchez P, Martinez-Silva MG, Ramos-Solano M, Olimon-Andalon V, Ortiz-Lazareno PC, Hernandez-Flores G, Bravo-Cuellar A, Aguilar-Lemarroy A, et al. Expression of transcription factor grainyhead-like 2 is diminished in cervical cancer. Int J Clin Exp Pathol 2014; 7:7409-18; PMID:25550776
- Werner S, Frey S, Riethdorf S, Schulze C, Alawi M, Kling L, Vafaizadeh V, Sauter G, Terracciano L, Schumacher U, et al. Dual roles of the transcription factor grainyhead-like 2 (GRHL2) in breast cancer. J Biol Chem 2013; 288:22993-3008; PMID:23814079; http://dx.doi.org/10.1074/jbc.M113.456293
- Xiang J, Fu X, Ran W, Chen X, Hang Z, Mao H, Wang Z. Expression and role of grainyhead-like 2 in gastric cancer. Med Oncol 2013; 30:714; PMID:24068586; http://dx.doi.org/10.1007/s12032-013-0714-5
- Cheng L, Wang P, Yang S, Yang Y, Zhang Q, Zhang W, Xiao H, Gao H. Identification of genes with a correlation between copy number and expression in gastric cancer. BMC Med Genomics 2012; 5:14; PMID:22559327; http://dx.doi.org/10.1186/1755-8794-5-14
- Werth M, Walentin K, Aue A, Schonheit J, Wuebken A, Pode-Shakked N, Vilianovitch L, Erdmann B, Dekel B, Bader M, et al. The transcription factor grainyhead-like 2 regulates the molecular composition of the epithelial apical junctional complex. Development 2010; 137:3835-45; PMID:20978075; http://dx.doi.org/10.1242/dev.055483
- Xiang X, Deng Z, Zhuang X, Ju S, Mu J, Jiang H, Zhang L, Yan J, Miller D, Zhang HG. Grhl2 determines the epithelial phenotype of breast cancers and promotes tumor progression. PloS One 2012; 7:e50781; PMID:23284647; http://dx.doi.org/10.1371/journal.pone.0050781
- Cieply B, Riley Pt, Pifer PM, Widmeyer J, Addison JB, Ivanov AV, Denvir J, Frisch SM. Suppression of the epithelial-mesenchymal transition by Grainyhead-like-2. Cancer Res 2012; 72:2440-53; PMID:22379025; http://dx.doi.org/10.1158/0008-5472.CAN-11-4038
- Cieply B, Farris J, Denvir J, Ford HL, Frisch SM. Epithelial-mesenchymal transition and tumor suppression are controlled by a reciprocal feedback loop between ZEB1 and Grainyhead-like-2. Cancer Res 2013; 73:6299-309; PMID:23943797; http://dx.doi.org/10.1158/0008-5472.CAN-12-4082
- Chung VY, Tan TZ, Tan M, Wong MK, Kuay KT, Yang Z, Ye J, Muller J, Koh CM, Guccione E, et al. GRHL2-miR-200-ZEB1 maintains the epithelial status of ovarian cancer through transcriptional regulation and histone modification. Sci Rep 2016; 6:19943; PMID:26887977; http://dx.doi.org/10.1038/srep19943
- Gao J, Aksoy BA, Dogrusoz U, Dresdner G, Gross B, Sumer SO, Sun Y, Jacobsen A, Sinha R, Larsson E, et al. Integrative analysis of complex cancer genomics and clinical profiles using the cBioPortal. Sci Signal 2013; 6:pl1. PMID:23550210; http://dx.doi.org/10.1126/scisignal.2004088
- Gyorffy B, Lanczky A, Szallasi Z. Implementing an online tool for genome-wide validation of survival-associated biomarkers in ovarian-cancer using microarray data from 1287 patients. Endocr Relat Cancer 2012; 19:197-208; PMID:22277193; http://dx.doi.org/10.1530/ERC-11-0329
- Riethdorf S, Frey S, Santjer S, Stoupiec M, Otto B, Riethdorf L, Koop C, Wilczak W, Simon R, Sauter G, et al. Diverse expression patterns of the EMT suppressor grainyhead-like 2 (GRHL2) in normal and tumour tissues. Int J Cancer 2016; 138:949-63; PMID:26355710; http://dx.doi.org/10.1002/ijc.29841
- Davies S, Holmes A, Lomo L, Steinkamp MP, Kang H, Muller CY, Wilson BS. High incidence of ErbB3, ErbB4, and MET expression in ovarian cancer. Int J Gynecol Pathol 2014; 33:402-10; PMID:24901400; http://dx.doi.org/10.1097/PGP.0000000000000081
- Dompe N, Rivers CS, Li L, Cordes S, Schwickart M, Punnoose EA, Amler L, Seshagiri S, Tang J, Modrusan Z, et al. A whole-genome RNAi screen identifies an 8q22 gene cluster that inhibits death receptor-mediated apoptosis. Proc Natl Acad Sci USA 2011; 108:E943-51; PMID:21949371; http://dx.doi.org/10.1073/pnas.1100132108
- Carmona FJ, Davalos V, Vidal E, Gomez A, Heyn H, Hashimoto Y, Vizoso M, Martinez-Cardus A, Sayols S, Ferreira HJ, et al. A comprehensive DNA methylation profile of epithelial-to-mesenchymal transition. Cancer Res 2014; 74:5608-19; PMID:25106427; http://dx.doi.org/10.1158/0008-5472.CAN-13-3659
- Yang X, Vasudevan P, Parekh V, Penev A, Cunningham JM. Bridging cancer biology with the clinic: Relative expression of a GRHL2-mediated gene-set pair predicts breast cancer metastasis. PloS One 2013; 8:e56195; PMID:23441166; http://dx.doi.org/10.1371/journal.pone.0056195
- Kang X, Chen W, Kim RH, Kang MK, Park NH. Regulation of the hTERT promoter activity by MSH2, the hnRNPs K and D, and GRHL2 in human oral squamous cell carcinoma cells. Oncogene 2009; 28:565-74; PMID:19015635; http://dx.doi.org/10.1038/onc.2008.404
- Leth-Larsen R, Terp MG, Christensen AG, Elias D, Kuhlwein T, Jensen ON, Petersen OW, Ditzel HJ. Functional heterogeneity within the CD44 high human breast cancer stem cell-like compartment reveals a gene signature predictive of distant metastasis. Mol Med 2012; 18:1109-21; PMID:22692575; http://dx.doi.org/10.2119/molmed.2012.00091
- Farris JC, Pifer PM, Zheng L, Gottlieb E, Denvir J, Frisch SM. Grainyhead-like 2 reverses the metabolic changes induced by the oncogenic epithelial-mesenchymal transition: Effects on anoikis. Mol Cancer Res 2016; 14:528-38; PMID:27084311; http://dx.doi.org/10.1158/1541-7786.MCR-16-0050
- Quan Y, Xu M, Cui P, Ye M, Zhuang B, Min Z. Grainyhead-like 2 Promotes Tumor Growth and is Associated with Poor Prognosis in Colorectal Cancer. J Cancer 2015; 6:342-50; PMID:25767604; http://dx.doi.org/10.7150/jca.10969
- Barretina J, Caponigro G, Stransky N, Venkatesan K, Margolin AA, Kim S, Wilson CJ, Lehar J, Kryukov GV, Sonkin D, et al. The Cancer Cell Line Encyclopedia enables predictive modelling of anticancer drug sensitivity. Nature 2012; 483:603-7; PMID:22460905; http://dx.doi.org/10.1038/nature11003
- Tan TZ, Miow QH, Miki Y, Noda T, Mori S, Huang RY, Thiery JP. Epithelial-mesenchymal transition spectrum quantification and its efficacy in deciphering survival and drug responses of cancer patients. EMBO Mol Med 2014; 6:1279-93; PMID:25214461; http://dx.doi.org/10.15252/emmm.201404208
- Tsai JH, Yang J. Epithelial-mesenchymal plasticity in carcinoma metastasis. Genes Dev 2013; 27:2192-206; PMID:24142872; http://dx.doi.org/10.1101/gad.225334.113
- Tania M, Khan MA, Fu J. Epithelial to mesenchymal transition inducing transcription factors and metastatic cancer. Tumour Biol 2014; 35:7335-42; PMID:24880591; http://dx.doi.org/10.1007/s13277-014-2163-y
- Findlay VJ, Wang C, Watson DK, Camp ER. Epithelial-to-mesenchymal transition and the cancer stem cell phenotype: Insights from cancer biology with therapeutic implications for colorectal cancer. Cancer Gene Ther 2014; 21:181-7; PMID:24787239; http://dx.doi.org/10.1038/cgt.2014.15
- Thiery JP. Epithelial-mesenchymal transitions in tumour progression. Nat Rev Cancer 2002; 2:442-54; PMID:12189386; http://dx.doi.org/10.1038/nrc822
- Nagafuchi A, Shirayoshi Y, Okazaki K, Yasuda K, Takeichi M. Transformation of cell adhesion properties by exogenously introduced E-cadherin cDNA. Nature 1987; 329:341-3; PMID:3498123; http://dx.doi.org/10.1038/329341a0
- Perl AK, Wilgenbus P, Dahl U, Semb H, Christofori G. A causal role for E-cadherin in the transition from adenoma to carcinoma. Nature 1998; 392:190-3; PMID:9515965; http://dx.doi.org/10.1038/32433
- Baum B, Settleman J, Quinlan MP. Transitions between epithelial and mesenchymal states in development and disease. Semin Cell Dev Biol 2008; 19:294-308; PMID:18343170; http://dx.doi.org/10.1016/j.semcdb.2008.02.001
- Celia-Terrassa T, Meca-Cortes O, Mateo F, Martinez de Paz A, Rubio N, Arnal-Estape A, Ell BJ, Bermudo R, Diaz A, Guerra-Rebollo M, et al. Epithelial-mesenchymal transition can suppress major attributes of human epithelial tumor-initiating cells. J Clin Invest 2012; 122:1849-68; PMID:22505459; http://dx.doi.org/10.1172/JCI59218
- Elloul S, Vaksman O, Stavnes HT, Trope CG, Davidson B, Reich R. Mesenchymal-to-epithelial transition determinants as characteristics of ovarian carcinoma effusions. Clin Exp Metastasis 2010; 27:161-72; PMID:20213325; http://dx.doi.org/10.1007/s10585-010-9315-2
- Wells A, Yates C, Shepard CR. E-cadherin as an indicator of mesenchymal to epithelial reverting transitions during the metastatic seeding of disseminated carcinomas. Clin Exp Metastasis 2008; 25:621-8; PMID:18600305; http://dx.doi.org/10.1007/s10585-008-9167-1
- Thiery JP, Acloque H, Huang RY, Nieto MA. Epithelial-mesenchymal transitions in development and disease. Cell 2009; 139:871-90; PMID:19945376; http://dx.doi.org/10.1016/j.cell.2009.11.007
- Jolly MK, Boareto M, Huang B, Jia D, Lu M, Ben-Jacob E, Onuchic JN, Levine H. Implications of the Hybrid Epithelial/Mesenchymal Phenotype in Metastasis. Front Oncol 2015; 5:155; PMID:26258068; http://dx.doi.org/10.3389/fonc.2015.00155
- Grosse-Wilde A, Fouquier d'Herouel A, McIntosh E, Ertaylan G, Skupin A, Kuestner RE, del Sol A, Walters KA, Huang S. Stemness of the hybrid Epithelial/Mesenchymal State in Breast Cancer and Its Association with Poor Survival. PloS One 2015; 10:e0126522; PMID:26020648; http://dx.doi.org/10.1371/journal.pone.0126522
- Andriani F, Bertolini G, Facchinetti F, Baldoli E, Moro M, Casalini P, Caserini R, Milione M, Leone G, Pelosi G, et al. Conversion to stem-cell state in response to microenvironmental cues is regulated by balance between epithelial and mesenchymal features in lung cancer cells. Mol Oncol 2016; 10:253-71; PMID:26514616; http://dx.doi.org/10.1016/j.molonc.2015.10.002
- Watanabe K, Villarreal-Ponce A, Sun P, Salmans ML, Fallahi M, Andersen B, Dai X. Mammary morphogenesis and regeneration require the inhibition of EMT at terminal end buds by Ovol2 transcriptional repressor. Dev Cell 2014; 29:59-74; PMID:24735879; http://dx.doi.org/10.1016/j.devcel.2014.03.006
- Abell AN, Jordan NV, Huang W, Prat A, Midland AA, Johnson NL, Granger DA, Mieczkowski PA, Perou CM, Gomez SM, et al. MAP3K4/CBP-regulated H2B acetylation controls epithelial-mesenchymal transition in trophoblast stem cells. Cell Stem Cell 2011; 8:525-37; PMID:21549327; http://dx.doi.org/10.1016/j.stem.2011.03.008
- Lecharpentier A, Vielh P, Perez-Moreno P, Planchard D, Soria JC, Farace F. Detection of circulating tumour cells with a hybrid (epithelial/mesenchymal) phenotype in patients with metastatic non-small cell lung cancer. Br J Cancer 2011; 105:1338-41; PMID:21970878; http://dx.doi.org/10.1038/bjc.2011.405
- Sarrio D, Rodriguez-Pinilla SM, Hardisson D, Cano A, Moreno-Bueno G, Palacios J. Epithelial-mesenchymal transition in breast cancer relates to the basal-like phenotype. Cancer Res 2008; 68:989-97; PMID:18281472; http://dx.doi.org/10.1158/0008-5472.CAN-07-2017
- Strauss R, Sova P, Liu Y, Li ZY, Tuve S, Pritchard D, Brinkkoetter P, Moller T, Wildner O, Pesonen S, et al. Epithelial phenotype confers resistance of ovarian cancer cells to oncolytic adenoviruses. Cancer Res 2009; 69:5115-25; PMID:19491256; http://dx.doi.org/10.1158/0008-5472.CAN-09-0645
- Pifer PM, Farris JC, Thomas AL, Stoilov P, Denvir J, Smith DM, Frisch SM. Grainyhead-like 2 inhibits the coactivator p300, suppressing tubulogenesis and the epithelial-mesenchymal transition. Mol Biol Cell 2016; 27:2479-92; PMID:27251061; http://dx.doi.org/10.1091/mbc.E16-04-0249
- Jia D, Jolly MK, Boareto M, Parsana P, Mooney SM, Pienta KJ, Levine H, Ben-Jacob E. OVOL guides the epithelial-hybrid-mesenchymal transition. Oncotarget 2015; 6:15436-48; PMID:25944618; http://dx.doi.org/10.18632/oncotarget.3623
- Gao X, Vockley CM, Pauli F, Newberry KM, Xue Y, Randell SH, Reddy TE, Hogan BL. Evidence for multiple roles for grainyhead-like 2 in the establishment and maintenance of human mucociliary airway epithelium. [corrected]. Proc Natl Acad Sci USA 2013; 110:9356-61; PMID:23690579; http://dx.doi.org/10.1073/pnas.1307589110
- Aue A, Hinze C, Walentin K, Ruffert J, Yurtdas Y, Werth M, Chen W, Rabien A, Kilic E, Schulzke JD, et al. A Grainyhead-Like 2/Ovo-Like 2 Pathway Regulates Renal Epithelial Barrier Function and Lumen Expansion. J Am Soc Nephrol 2015; 26:2704-15; PMID:25788534; http://dx.doi.org/10.1681/ASN.2014080759
- Walentin K, Hinze C, Werth M, Haase N, Varma S, Morell R, Aue A, Potschke E, Warburton D, Qiu A, et al. A Grhl2-dependent gene network controls trophoblast branching morphogenesis. Development 2015; 142:1125-36; PMID:25758223; http://dx.doi.org/10.1242/dev.113829
- Beaufort CM, Helmijr JC, Piskorz AM, Hoogstraat M, Ruigrok-Ritstier K, Besselink N, Murtaza M, van IWF, Heine AA, Smid M, et al. Ovarian cancer cell line panel (OCCP): Clinical importance of in vitro morphological subtypes. PloS One 2014; 9:e103988; PMID:25230021; http://dx.doi.org/10.1371/journal.pone.0103988
- Faddaoui A, Bachvarova M, Plante M, Gregoire J, Renaud MC, Sebastianelli A, Gobeil S, Morin C, Macdonald E, Vanderhyden B, et al. The mannose receptor LY75 (DEC205/CD205) modulates cellular phenotype and metastatic potential of ovarian cancer cells. Oncotarget 2016; 7:14125-42; PMID:26871602; http://dx.doi.org/10.18632/oncotarget.7288
- Taylor PT, Haverstick D. Re: New guidelines to evaluate the response to treatment in solid tumors (ovarian cancer). J Natl Cancer Inst 2005; 97:151; author reply 2; PMID:15657346; http://dx.doi.org/10.1093/jnci/dji027
- Wang ZQ, Faddaoui A, Bachvarova M, Plante M, Gregoire J, Renaud MC, Sebastianelli A, Guillemette C, Gobeil S, Macdonald E, et al. BCAT1 expression associates with ovarian cancer progression: Possible implications in altered disease metabolism. Oncotarget 2015; 6:31522-43; PMID:26372729; http://dx.doi.org/10.18632/oncotarget.5159
- Keita M, Bachvarova M, Morin C, Plante M, Gregoire J, Renaud MC, Sebastianelli A, Trinh XB, Bachvarov D. The RUNX1 transcription factor is expressed in serous epithelial ovarian carcinoma and contributes to cell proliferation, migration and invasion. Cell Cycle 2013; 12:972-86; PMID:23442798; http://dx.doi.org/10.4161/cc.23963