ABSTRACT
Cyclin proteins are the key regulatory and activity partner of cyclin-dependent kinases (CDKs), which play pivotal regulatory roles in cell cycle progression. In the present study, we identified a Cyclin L1 and 2 CDK11 2 CDK11 splice variants, CDK11A and CDK11B, from silkworm, Bombyx mori. We determined that both Cyclin L1 and CDK11A/B are nuclear proteins, and further investigations were conducted to elucidate their spatiofunctional features. Cyclin L1 forms a complex with CDK11A/B and were co-localized to the nucleus. Moreover, the dimerization of CDK11A and CDK11B and the effects of Cyclin L1 and CDK11A/B on cell cycle regulation were also investigated. Using overexpression or RNA interference experiments, we demonstrated that the abnormal expression of Cyclin L1 and CDK11A/B leads to cell cycle arrest and cell proliferation suppression. Together, these findings indicate that CDK11A/B interacts with Cyclin L1 to regulate the cell cycle.
KEYWORDS:
Introduction
Cyclin-dependent kinases (CDKs) are serine/threonine kinases whose activity requires binding of specific cyclin subunits to play critical roles in the control of the cell cycle or transcription.Citation1 CDKs generally contain a catalytic serine/threonine kinase subunit and a regulatory cyclin subunit, and their activity is dependent on their association with cyclins and endogenous inhibitors (Ink4, Cip/Kip inhibitors), as well as by positive and negative phosphorylation events.Citation2,3 Cyclins are periodically synthesized and destroyed during the cell cycle, thereby regulating CDK activity in a timely manner.Citation4 In mammals, a total of 11 classical CDKs (CDK1–11) and 2 newly proposed family members (CDK12 and CDK13), as well as other proteins with structure or function similar to that of CDKs have been identified to date.Citation1 Cyclin proteins are mainly classified into 2 major groups based on their function, namely, the cell cycle regulator cyclins (A, B, D, E, and F) and the transcription regulator cyclins (C, H, K, L1, T1, and T2). Some other cyclins have been identified; however, their functions remain unknown.Citation5
CDK11 (also known as PITSLRE) protein kinases are the products of 2 highly homologous p34cdc2-related genes (CDC2L 1 and CDC2L 2) that respectively express 3 major isoforms, CDK11p110, CDK11p58,and CDK11P46.Citation6 The large CDK11p110 isoform that is associated with cyclin L is involved in the regulation of transcription, pre-mRNA splicing, and autophagy.Citation7-9 The CDK11p58 isoforms are produced by the same mRNAs through the use of an internal ribosome entry site (IRES) during the G2/M phase of the cell cycle.Citation10 Although CDK11p110 and CDK11p58 share the same kinase domain at its C-terminus, the 2 isoforms exhibit different functions. CDK11p58 is located in the cytoplasm and nucleus, and specifically expressed at G2/M phase. It alsoapparently interacts with cyclin D3 and plays an important role in the duplication of the centrioles, spindle dynamics, and sister chromatid cohesion at the centromeres during mitosis.Citation11-16 CDK11p110/p58−/− mice embryonic lethality at the blastocyst stage is accompanied by mitotic arrest.Citation17 CDK11p46 primarily contains the catalytic kinase domain and is generated by caspase-dependent cleavage of CDK11p110 and CDK11p58 during apoptosis.Citation18 The ectopic expression of CDK11p46 induces apoptosis.Citation19 CDK11 is highly conserved in various species including human, mouse, Drosophila melanogaster, Xenopus, and Caenorhabditis elegans, thereby suggesting that CDK11 plays important functions.
Cyclin L1 (CCNL1) is a nuclear protein that belongs to the cyclin family. It contains a C-terminal arginine and serine-rich (RS) domain and the characteristic cyclin box.Citation20 The RS domain is similar to the serine/arginine-rich (SR) family of splicing factors that are required at various steps of spliceosome assembly and regulated splicing.Citation21 In human, various cyclin L1 and L2 isoforms have been reported to interact with CDK11 and splicing factors and are involved in the regulation of splicing.Citation22 It has also been reported that cyclin L1 plays a critical role in the progression of head and neck squamous cell carcinoma (HNSCCC) and breast cancer.Citation23,24
In the present study, we identified a cyclin L1 and 2 CDK11 splice variants in silkworm and have localized these to the nucleus using their nuclear localization signals (NLS). In addition, we also investigated the interactions between cyclin L1, CDK11A, and CDK11B. Furthermore, the abnormal expression of cyclin L1 and CDK11A/B resulted in cell cycle arrest and cell proliferation suppression. Taken together, the findings of the present study may contribute information that may be used in the elucidation of the mechanism underlying Cyclin L1 and CDK11 nuclear localization and cell cycle regulation in silkworm.
Results
Cloning and identification of BmCyclin L1, BmCDK11A,and BmCDK11B
The 3 genes, BmCyclin L1, BmCDK11A,and BmCDK11B, were cloned from larval cDNA of the Dazao strain. BmCyclin L1 has 1,254 bp of coding sequence (CDS) that encodes a 417-amino acid protein that consists of 2 conservative CYCLIN domains (). Multiple sequence analysis demonstrated that BmCyclin L1 is highly conserved (). Phylogenetic tree analysis showed that BmCyclin L1 and Danaus plexippus cyclin L1 could be clustered together as Lepidoptera cyclin L1 (). These results suggest that BmCyclin L1 is a member of the cyclin L1 subfamily.
Figure 1. Sequences analysis and phylogenetic trees of BmCyclin L1 and BmCDK11A/B. (A) Multiple sequence alignment of cyclin L1 from Bombyx mori, Homo sapiens, Mus musculus, Xenopus laevis, and Danio rerio, containing 2 conservative CYCLIN domains (red and orange lines). The protein domains were predicted using the online prediction SMART software (http://smart.embl-heidelberg.de/). (B) Gene structure of 2 CDK11 spliceosomes, CDK11A and CDK11B. (C) Multiple sequence alignment of CDK11 from B. mori, Papilio xuthus, H. sapiens, M. musculus, X. laevis, and D. rerio, containing the S-TKc domain (red line). (D) Phylogenetic tree (N-J) of cyclin L1 from vertebrates and invertebrates. (E) Phylogenetic tree (N-J) of CDK11.
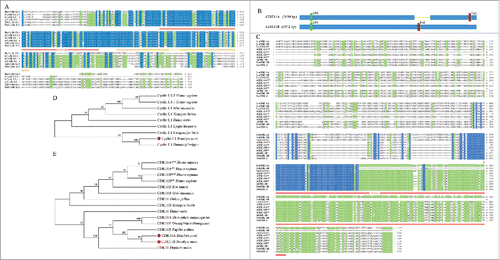
We obtained 2 splice variants for CDK11, BmCDK11A, and BmCDK11B, which might have possibly arisen from the same gene. The CDS of BmCDK11A is 2,694 bp in length and encodes an 897-amino acid protein, whereas BmCDK11B is 1,812 bp in length and encodes a 603-amino acid polypeptide. We also determined that BmCDK11A mRNA has 145-bp deletion that results in a change in the stop codon, thereby generating a longer protein (). Sequence analysis showed that BmCDK11A/Bcontains a non-conserved N-terminal and a highly conserved C-terminal, whereas BmCDK11Ahas a conserved S-TKc domain (), also known as the catalytic domain. BmCDK11B lacks most of the C-terminal region, and thus only mainly consists of the Pkinase domain. Phylogenetic tree analysis indicated that BmCDK11A/B, Papilio Xuthus CDK11B, and Drosophila melanogaster CDK11A/E form one group (). These results suggest that BmCDK11Aand BmCDK11B belong to the CDK11 family.
Identification of NLS in cyclin L1 and CDK11
Immunofluorescence analysis localized both cyclin L1 and CDK11A/B to the nucleus (). To further assess the position of cyclin L1 and CDK11A/B within this particular organelle, we initially used the online prediction software, cNLS Mapper (http://nls-mapper.iab.keio.ac.jp/cgi-bin/NLS_Mapper_form.cgi) and NucPred (http://www.sbc.su.se/∼maccallr/nucpred/cgi-bin/single.cgi) to identify their corresponding nuclear localization signals (NLS), which indicated that they may have their own NLS. Next, we constructed a series of recombinant vectors that express various cyclin L1 or CDK11deletion mutants, to which we fused the N-terminus of DsRed tag (). The vectors of various cyclin L1 and CDK11deletion mutants were then transfected into BmNS cells and the distribution of the fusion protein were determined at 48 h post transfection by confocal microscopy.
Figure 2. Identification of nuclear localization signal (NLS) in cyclin L1 and CDK11. (A) Schematic representation of the DsRed-tagged cyclin L1 deletion constructs. (B) Schematic representation of the DsRed-tagged CDK11 deletion constructs. (C) Amino acid residues 362–394 of cyclin L1 contribute to the nuclear localization. The full-length and various deletion fragments of cyclin L1 were transfected into BmNS cells and the distribution of fusion protein were determined at 48 h post transfection by immunostaining. (D) Amino acid residues 345–372 of CDK11 A/B contribute to their nuclear localization. The distribution of fusion protein was analyzed by using confocal microscopy.
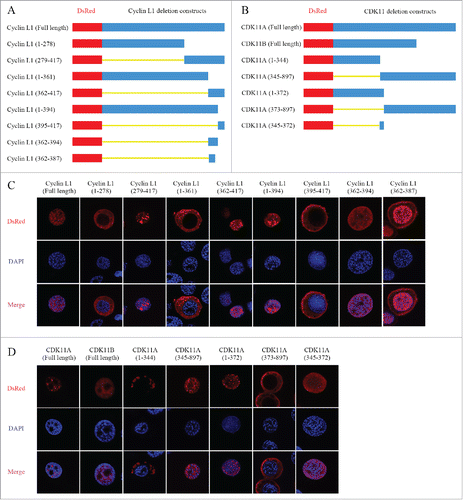
The fusion proteins produced by the amino acid resides 279–417, 362–417, and 1–394 of cyclin L1 were localized to the nucleus, whereas amino acids 1–278, 1–361, and 395–417 were detected in the cytoplasm (). These results indicated that amino acids 362–394 may represent the NLS domain of cyclin L1. To confirm our hypothesis, a deletion mutant vector consisting of amino acids 362–394 was constructed and was also localized to the nucleus (). To identify the core NLS domain, we further constructed a vector consisting of amino acids 362–387 of cyclin L1, and our results showed that most of the polypeptide was localized to the nucleus, whereas the rest were detected in the cytoplasm (). Together, these results suggest that the amino acid residues 362–394 contain the core NLS domain and contribute to the nuclear localization of cyclin L1.
The same approach was used to identify the NLS of CDK11. Confocal microscopy indicated that the amino acids 1–344 and 373–897 of CDK11 were distributed in the cytoplasm, whereas amino acids 345–897 and 1–372 were localized to the nucleus (). The overlapping amino acids 345–372 was then identified as the core NLS domain that is responsible for the nuclear localization of CDK11 (). These results showed that amino acids 345–372 of CDK11A and CDK11Bserve as the NLS.
Formation of the cyclin L1 and CDK11A/B complex
Cyclin L1 is the regulatory partner of CDK11 in other species; however, no report has established this relationship in silkworm. Flag-tagged Cyclin L1 and HA-tagged CDK11A/B proteins were prepared by lysing co-transfected BmNS cells. Co-IP experiments were then conducted to confirm the interaction between cyclin L1 and CDK11A or CDK11B. Western blot analysis confirmed that cyclin L1 protein was present in the CDK11A and CDK11B IPs and vice versa (), thereby suggesting that cyclin L1 and CDK11A/B could form the complex.
Figure 3. Cyclin L1 form a complex with CDK11A/B. (A) Co-immunoprecipitation (Co-IP) assays of the interaction of cyclin L1 with CDK11A and CDK11B, respectively. The red arrow indicates the IgG heavy chain. (B) Immunofluorescence analysis of the co-localization of cyclin L1 with CDK11A and CDK11B, respectively. Nuclei were stained with DAPI.
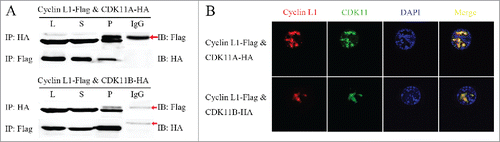
Fluorescence co-localization experiments involving cyclin L1 and CDK11A or CDK11B were used to further determine the above results. The expression vectors of cyclin L1 and CDK11A or CDK11B wereco-transfected into BmNS cells. Exogenous cyclin L1 protein exhibited a very similar nuclear staining and co-localized with exogenous CDK11A or CDK11B protein (). These findings indicated that again, cyclin L1 and CDK11A/B form the proteincomplex.
The dimerization between CDK11A, CDK11B, and themselves
-Co-immunoprecipitation confirmed the interactions involving CDK11A, CDK11B, and themselves. The Flag-tagged CDK11A and CDK11Bexpression vectors were constructed and co-transfected into BmNS cells using HA-tagged CDK11A and CDK11B expression vectors. Western blot analysis of the co-IPs detected the Flag-tagged CDK11Aprotein in the HA-tagged CDK11A and CDK11BIPs. Flag-tagged CDK11Bwas also identified in the HA-tagged CDK11B IPs (). Reverse co-IP experiments generated similar results (). The recombinant vectors were also used in fluorescence co-localization experiments to determine protein-protein interactions. Different tagged fusion proteins of CDK11A and CDK11B were co-localized to the nucleus (). These results indicated that CDK11A, CDK11B, and themselves formed dimers.
The cell cycle regulation by cyclin L1, CDK11A/B and their complex
To investigate whether the complex affects cell cycle progression, recombinant overexpression plasmids were transfected into BmNS cells (Figs S1A, B), and then the cell proliferation activity and cell cycle were detected. MTT was used to generate a growth curve assay, and pIZ/V5-His blank vectors were used as controls. Transfection of the single cyclin L1 overexpression (cyclin L1-OE) plasmid, CDK11A-OE plasmid, or CDK11B-OE plasmids resulted in an inhibition of proliferation activity of the BmNS cells. Stronger inhibitory effects were observed when the cyclin L1-OE and CDK11A/B- OE plasmids were co-transfected into the BmNS cells (). We further analyzed the effects of overexpression of cyclin L1, CDK11A, and CDK11B on the cell cycle by flow cytometry, and the cell rate at the G0/G1 phase increased to 34.78%, 35.83%, and 32.32%, in contrast to 26.73% in the control cells (). Meanwhile, the cells rates at the G0/G1 phase increased to 40.91% and 37.58% when cyclin L1- CDK11A, or Cyclin L1- CDK11Bwere co-transformed (). These results demonstrate that the overexpression of cyclin L1, CDK11A, and CDK11B leads to cell cycle arrest and cell growth inhibition, and cyclin L1 and CDK11A/Bform as complex that co-regulates the cell cycle.
Figure 5. Cyclin L1 and CDK11A/(B)complex suppress cell proliferation and induce cell cycle arrest. (A) MTT was used for the growth curve assay of cyclin L1 and CDK11A/B overexpression BmNS cells at 24 h, 48 h, and 72 h after transfection. (B) Flow cytometry was used for the cell cycle analysis of cyclinL1 and CDK11A/B overexpression cells at 48 h after transfection. Piz/V5-His plasmids were used as controls and nuclei were stained with PtdIns. (C) Statistical analysis of the cell percentage of each phase, including G0/G1, S, and G2/M (*P<0.05, **P<0.01). (D) The proliferation of cyclin L1 and CDK11 RNA interference cells through the MTT assay. The expression of CDK11A and CDK11B were knocked down by using a CDK11-RNAi plasmid. (E) The cell cycle distribution of cyclin L1-RNAi, CDK11-RNAi, and co-RNAi cells were determined by flow cytometry. (F) BmNS cell distribution at different cell cycle phases after cyclin L1 and CDK11 RNA interference, respectively, or both (*P<0.05, **P<0.01).
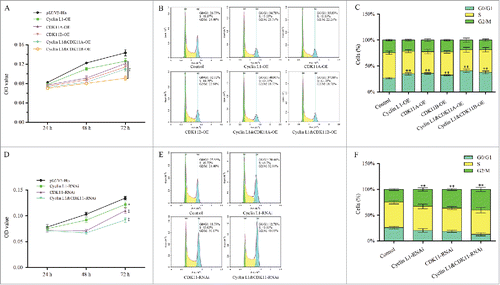
Next, microRNA-mediated RNA interference (RNAi) vectors were used to knock down the expression of cyclin L1 and CDK11. CDK11-RNAi vectors were designed according to the common area of CDK11A and CDK11B, and thus could knock down both CDK11A and CDK11Bexpression. The interference effects of cyclin L1-RNAi and CDK11-RNAi were analyzed by qRT-PCR at 48 h post-transfection, which showed an inhibition of the expression of the target genes (Figs S1A, B). Then, the proliferation of BmNS cells transiently transfected with cyclin L1-RNAi, CDK11-RNAi, or co-transfected were analyzed by using the MTT assay. The results showed that co-transfection enhance the inhibitory activity of cyclin L1 and CDK11 via inhibition of the proliferation activity of the BmNS cells (). To further confirm the RNAi effects of cyclin L1 and CDK11 on the cell cycle, flow cytometry was performed to analyze the BmNS cells at 48 h after introduction of the cyclin L1-RNAi plasmids and CDK11-RNAi plasmids transfection. The results showed that the cells were arrested at the G2/M phase compared with that in the control cells, and the number of G2/M cells was 24.40% of that of the control cells, 32.84% of the cyclin L1-RNAi cells, and 36.17% of the CDK11-RNAi cells (). Furthermore, the number of G2/M cells of the co-transfection group was higher than that of the other groups (). These results confirmed that cyclin L1 and CDK11 synergistically regulate the cell cycle of silkworm BmNS cells in vitro.
Discussion
In the present study, we identified a cyclin L1 homolog and 2 CDK11 transcripts, CDK11A and CDK11B, in Bombyx mori that might have arisen from the same gene through alternative splicing. Multiple sequence alignment showed that cyclin L1 possesses the typical CYCLIN domain and CDK11A/B has a highly conserved C-terminal catalytic domain. Phylogenetic tree analyses indicated that cyclin L1 and CDK11A/B belong to the same group as that of other insects. These findings indicated that cyclin L1 and CDK11 play important roles and may have relatively conserved functions over the course of evolution.
Cyclin proteins function as the key regulatory and activity factors that periodically regulate the activity of specific CDKs.Citation25 Co-IP experiments and double fluorescence co-localization experiments indicated that exogenous cyclin L1 and CDK11A/B form complexes and are co-located in the nucleus. Cyclin L1 and CDK11p110 were co-localize to the nucleus, which is in agreement with the findings of previous reports that cyclin L act as the regulatory partner and interaction factor of CDK11.Citation22 Moreover, the nuclear localization model of cyclin L1 and CDK11A/B were analyzed by using deletion mutant experiments. Both cyclin L1 and CDK11A/B only contained one NLS. The core NLS domain of cyclin L1 consisted of amino acid residues 362–394, whereas the core NLS domain of CDK11A and CDK11B involved amino acid residues 345–372. In addition, we characterized the homodimerization of CDK11A and CDK11B, respectively. A previous study reported that both CDK11p110 andCDK11p58 existed as homodimers and were involved in CHK2-dependent phosphorylation or autophosphorylation.Citation26 Another study suggested that the dimers and monomers are located in different sites and have varying biologic functions because studies of ERK2 have shown that only homodimers are transported to the nucleus.Citation27 Thus, the functions and the mechanism underlying dimerization remain unclear.
Our in vitro experiments also showed that the cyclin L1-CDK11A/B complex may play a special role in cell cycle regulation in silkworm. Overexpression and RNAi plasmids were transfected into the BmNS cells to generate cell models that abnormally expressed cyclin L1 and CDK11A/B. Our results showed that the overexpression of cyclin L1 and CDK11A/B inhibited cell growth and induced cell cycle arrest at the G0/G1 phase, whereas silencing these proteins caused an accumulation of cells at the G2/M phase, thereby inhibiting cell proliferation. These findings indicate that these collective treatment groups exhibited greater inhibition or more extensive arrest effects than individual treatment groups. These findings thus indicated that cyclin L1 and CDK11A/B cooperatively regulate the cell cycle by forming a complex in silkworm. Previous studies have reported that the cyclin L1-CDK11 complex is involved in pre-mRNA splicing, transcription, RNA processing, and the regulation of cell cycle. In fission yeast, the complex regulates the assembly of the RNA polymerase II mediator complex by phosphorylating Med4 and Med27.Citation28 CDK11 also interacts with general pre-mRNA splicing factors RNPS1 and 9G8.Citation22 In Xenopus laevis, CDK11 acts as a RanGTP-dependent microtubule stabilization factor that regulates the assembly rate of spindle.Citation29 CDK11p110/p58-null mutant mice display early embryonic lethality during the blastocyst stage of embryonic development due to the cells that exhibit proliferative defects, mitotic arrest, and apoptosis.Citation17 CDK11p58 is located in the cytoplasm and nucleus and is specifically expressed at the G2/M phase.Citation11 The kinase activity of CDK11p58 is mainly involved in mitosis, which include maintenance of sister chromatid cohesion, bipolar spindle formation, and centrosome duplication and maturation.Citation14-16 In addition, they also play important roles in tumor growth; for example, cyclin L1 is overexpressed in human head and neck squamous cell carcinoma and have a special role in the regulation of the breast cancer cell cycle.Citation23,24 CDK11p110 is crucial for the growth of human breast cancer cell proliferation and liposarcoma Cells.Citation30,31 We speculated that the abnormal expression of cyclin L1 and CDK11A/B may also influence the transcription or the mitotic processing. This may also explain why the abnormal expression of cyclin L1 and CDK11A/B causes cell cycle arrest and suppress cell proliferation.
In conclusion, we identified a B. mori gene cyclin L1 and 2 CDK11 transcripts, CDK11A and CDK11B, which form protein complexes in the nucleus. Cyclin L1 was localized to the nucleus based on the NLS, which was situated in amino acids 362–394. On the other hand, CDK11A and CDK11B share the common amino acids 345–372 in their NLSs. Our findings also suggest that the cyclin L1-CDK11A/B complex plays important relatively roles in the regulation the cell cycle in silkworm. However, the functional mechanism and the regulatory relationships of cyclin L1 and CDK11A/B require further research.
Methods and materials
Silkworm strain and cell cultures
The larval cDNA was extracted from third-day fifth-instar Dazao larvae and the silkworm strain Dazao was maintained at the Gene Resource Library of Domesticated Silkworm of Southwest University, Chongqing, China. B. mori-derived BmN-SWU1 (BmNS) cells were cultured in TC-100 insect medium at 27°C.Citation32
Phylogenetic reconstruction
The homologous protein sequences of cyclin L1 and CDK11 from different species were acquired from NCBI. Then, MUSCLE was used to align the sequences and the neighbor joining method was used to estimate the phylogenetic trees in MEGA5.0. The detailed protocol was performed according to a previous study.Citation33
Plasmid construction
Cyclin L1 and CDK11A/B were amplified from larval cDNA using gene specific primers. Cyclin L1: Forward 5′ ATGACCACGACGACCGTAC 3′ and reverse 5′ CTAATGTTTCCTTTTGTCATCCC 3′ and CDK11A/B: Forward 5′AATTGTTGCGTCTAATGCCAT 3′ and Reverse 5′ GACGCTTCGGTAATTAAAAATGTC 3′. Next, all the amplified products were cloned into the pMD19-T vector (Takara, Dalian, China) and sequenced, respectively. The correct CDS sequences of the genes were cloned into insect expression vector pIZ/V5-His (Invitrogen, US) to which Flag or HA tags were fused to obtain the overexpression plasmids of the target genes, Cyclin L1, CDK11A, and CDK11B. The deletion mutant fragments of cyclin L1 and CDK11 were amplified from the overexpression plasmids and cloned into pIZ/V5-His vector by fusing the N-terminus of DsRed tag.
MicroRNA-mediated RNA interference (RNAi) vectors of cyclin L1 and CDK11 containing a DsRed fluorescent protein gene were fused to a pIZ/V5-His vector and silkworm endogenous microRNA backbones (bom-mir-279) that harbored the constructed target sequences of Cyclin L1 and CDK11. The special artificial synthetic siRNA duplex sequences targeting the cyclin L1 or CDK11 genes were selected using Invitrogen BLOCK-iT™ RNAi Designer (http://rnaidesigner.thermofisher.com/rnaiexpress/setOption.do?designOption=mirnaandpid=7795643179598091088) and synthesized by GenScript Corporation (Nanjing, China) as described previously.Citation34
Cell transient transfection
BmNS cells at log phase were seeded onto coverslips (Fisher Scientific, Waltham, MA) in 24-well plates or culture flasks (corning) for approximately 24 h before transfection. The plasmids and X-treme GENE HP DNA Transfection Reagent (Roche) were mixed and transfected into each well, according to the manufacturer's instruction. The transfection medium was replaced with new medium containing antibiotic and serum at 6 h post transfection.
Co-immunoprecipitation (Co-IP) assay and Western blotting
BmNS was collected and washed twice with PBS at 72 h after transfection following lysed with the lysis buffer (Beyotime, China) for 30 min at 4°C. Then, the cells lysates (L) were centrifuged at 13,000×g for 30 min at 4°C to obtain the supernatant (S). The anti-Flag/HA-tags antibody (Ab, 3 µg) or mouse control IgG were diluted in 200 µL PBS and incubated with 50 µL Dynabeads® (Life Technologies, US) for 10 min at room temperature to obtain the Dynabeads-Ab complex. Next, the supernatant was incubated with Dynabeads®-Ab complex to allow formation of the new complex, Dynabeads®-Ab- antigen (Ag). The new complex was washed thrice using 200 µL washing buffer for each and denatured at 100°C with 5× SDS-PAGE loading buffer (Beyotime). Protein samples were separated via 12% SDS-PAGE and the bands were transferred onto PVDF membranes. The PVDF membranes were incubated with the primary antibody, anti-Flag/anti-HA (1/5000, Sigma, US), and secondary antibody, HRP-conjugated anti-mouse IgG/HRP conjugated anti-mouse IgG (1/10000, Beyotime). The protein bands were analyzed with s ECL Western Blotting Detection System (Bio-Rad, US).
Immunofluorescence
Cells were fixed with 4% paraformaldehyde for 15 min, permeated in 0.5% Triton X-100 for 10 min at room temperature, and then blocked with Immunol Staining Blocking Buffer for 1 h at 37°C (Beyotime). The cells were incubated with the primary antibody, anti-Flag/anti-HA (1/200, Sigma, US) and anti-RFP (1/200, Abcam, UK), and secondary antibody, Alexa 555 conjugated donkey anti-mouse (1:500; Life Technologies) or Alexa 488-conjugated donkey anti-mouse (1/500; Life Technologies). Nuclei were stained with DAPI (2, 4-diamidino-2-phenylindole, Beyotime). Finally, the distribution of proteins was observed under a confocal microscope (Olympus, Japan).
Quantitative real time-PCR (qRT-PCR) assay
Total RNA was purified from each sample using a Total RNA Kit II (OMEGA) and reverse transcribed into cDNA. The specific primers of Cyclin L1 and CDK11, Cyclin L1: Forward 5′ CCCACACTGGTTCCTTCTATT 3′ and Reverse 5′ GGTGATTCGGGTTTCTTTTC 3′ and CDK11A/B: Forward 5′ CGAAGTGAGTTAGAAGCAAGACG 3′ and Reverse 5′ CAGAATCTCCATACGAAGGTGAC 3′, were used for the analysis of transcription levels. The house-keeping gene, ribosomal protein gene (rpl3) and Action3 were used as internal gene, rpl3: forward 5′ CGGTGTTGTTGGATACATTGAG 3′ and reverse 5′ GCTCATCCTGCCATTTCTTACT 3′, Action3: forward 5′ AACACCCCGTCCTGCTCACTG 3′ and reverse 5′ GGGCGAGACGTGTGATTTCCT 3′. The qRT-PCR was performed in a 15-μL reaction mixture (1 μL of cDNA, 0.5 mM of each primer, and 2× iTaq™ Universal SYBR Green Supermix (Bio-Rad) in each well of 96-well plate and each test was repeated thrice.
MTT assay
The cell growth curve assay was analyzed by using an MTT cell proliferation and cytotoxicity assay kit (Beyotime). Cells were seeded into 96-well plates and 100 µL of the MTT solution (5 mg/mL) was added to each well at 24 h, 48 h, and 72 h after transfection. The plates were incubated at 37°C for 4 h, then 100 µL of the formazan dissolving reagent was added to each well, and then again incubated at 37°C for 4 h. Cellular viability was determined at a wavelength of 570 nm.
Flow cytometry analysis (FCM)
A cell cycle and apoptosis analysis kit (Beyotime) was used for the analysis of cell cycle. After 48 h of transfection with the overexpression and RNAi plasmids of cyclin L1 and CDK11A/B, the BmNS cells were collected and washed with PBS and fixed with 70% ethanol at 4°C overnight. Then, the fixed cells were washed twice with PBS and treated with RNaseA and propidium iodide (PI) at 37°C for 30 min. Finally, the cells were then analyzed using a flow cytometer (Becton Dickinson USA).
Statistical analysis
Data were expressed as the mean ± SEM of 3 independent experiments. All the statistically significant differences among various treatments were assessed by using the Student's t test. A P-value < 0.05 was considered significant, here indicated with “*” and P-value < 0.01 was considered very significant, here indicated with “**.”
Disclosure of potential conflicts of interest
The authors declare that they have no competing financial interests.
Author contributions statement
Conceived and designed the experiments: Tai-hang Liu, Yun-fei Wu, and Xiao-long Dong. Performed the experiments performance: Tai-hang Liu, Yun-fei Wu, Xiao-long Dong, Cai-xia Pan and Guo-yu Du. Data analysis: Tai-hang Liu, Ji-gui Yang, Wei Wang and Xi-yan Bao. Reagents/materials/analysis tools contribution: Peng Chen, Min-hui Pan and Cheng Lu. Paper written: Tai-hang Liu and Yun-fei Wu.
1304339_Supplemental_Material.doc
Download MS Word (379 KB)Acknowledgments
We thank Dr. Xiao-lin Zhou for the help in performing the experiments. The National Natural Science Foundation of China (Grant Nos. 31272505, 31472152, and 31572466) and China Agriculture Research System (CARS-22) supported this study.
References
- Malumbres M, Harlow E, Hunt T, Hunter T, Lahti JM, Manning G, Morgan DO, Tsai LH, Wolgemuth DJ. Cyclin-dependent kinases: a family portrait. Nat Cell Biol 2009; 11:1275-6; PMID:19884882; http://dx.doi.org/10.1038/ncb1109-1275
- Malumbres M, Barbacid M. Cell cycle, CDKs and cancer: a changing paradigm. Nat Rev Cancer 2009; 9:153-66; PMID:19238148; http://dx.doi.org/10.1038/nrc2602
- Malumbres M. Cyclin-dependent kinases. Genome Biol 2014; 15:122; PMID:25180339
- Malumbres M, Barbacid M. Mammalian cyclin-dependent kinases. Trends Biochem Sci 2005; 30:630-41; http://dx.doi.org/10.1016/j.tibs.2005.09.005
- Yang L, Li N, Wang C, Yu Y, Yuan L, Zhang M, Cao X. Cyclin L2, a novel RNA polymerase II-associated cyclin, is involved in pre-mRNA splicing and induces apoptosis of human hepatocellular carcinoma cells. J Biol Chem 2004; 279:11639-48; PMID:14684736; http://dx.doi.org/10.1074/jbc.M312895200
- Trembley JH, Loyer P, Hu DL, Li TY, Grenet J, Lahti JM, Kidd VJ. Cyclin dependent kinase 11 in RNA transcription and splicing. Prog Nucleic Acid Re 2004; 77:263-88; http://dx.doi.org/10.1016/S0079-6603(04)77007-5
- Loyer P, Busson A, Trembley JH, Hyle J, Grenet J, Zhao W, Ribault C, Montier T, Kidd VJ, Lahti JM. The RNA binding motif protein 15B (RBM15B/OTT3) is a functional competitor of serine-arginine (SR) proteins and antagonizes the positive effect of the CDK11p110-cyclin L2alpha complex on splicing. J Biol Chem 2011; 286:147-59; PMID:21044963; http://dx.doi.org/10.1074/jbc.M110.192518
- Hu D, Mayeda A, Trembley JH, Lahti JM, Kidd VJ. CDK11 complexes promote pre-mRNA splicing. J Biol Chem 2003; 278:8623-9; PMID:12501247; http://dx.doi.org/10.1074/jbc.M210057200
- Wilkinson S, Croft DR, O'Prey J, Meedendorp A, O'Prey M, Dufes C, Ryan KM. The cyclin-dependent kinase PITSLRE/CDK11 is required for successful autophagy. Autophagy 2011; 7:1295-301; PMID:21808150; http://dx.doi.org/10.4161/auto.7.11.16646
- Xiang JL, Lahti JM, Grenet J, Easton J, Kidd VJ. Molecular-Cloning And Expression Of Alternatively Spliced Pitslre Protein-Kinase Isoforms. J Biol Chem 1994; 269:15786-94; PMID:8195233
- Zhang S, Cai M, Zhang S, Xu S, Chen S, Chen X, Chen C, Gu J. Interaction of p58(PITSLRE), a G2/M-specific protein kinase, with cyclin D3. J Biol Chem 2002; 277:35314-22; PMID:12082095; http://dx.doi.org/10.1074/jbc.M202179200
- Duan Y, He X, Yang H, Ji Y, Tao T, Chen J, Hu L, Zhang F, Li X, Wang H, et al. Cyclin D3/CDK11(p58) complex involved in Schwann cells proliferation repression caused by lipopolysaccharide. Inflammation 2010; 33:189-99; PMID:20066559; http://dx.doi.org/10.1007/s10753-009-9173-8
- Li Z, Wang H, Zong H, Sun Q, Kong X, Jiang J, Gu J. Downregulation of beta1,4 galactosyltransferase 1 inhibits CDK11(p58)-mediated apoptosis induced by cycloheximide. Biochem Biophys Res Commun 2005; 327:628-36; PMID:15629159; http://dx.doi.org/10.1016/j.bbrc.2004.12.047
- Rakkaa T, Escude C, Giet R, Magnaghi-Jaulin L, Jaulin C. CDK11(p58) kinase activity is required to protect sister chromatid cohesion at centromeres in mitosis. Chromosome Res 2014; 22:267-76; PMID:24436071; http://dx.doi.org/10.1007/s10577-013-9400-x
- Petretti C, Savoian M, Montembault E, Glover DM, Prigent C, Giet R. The PITSLRE/CDK11(p58) protein kinase promotes centrosome maturation and bipolar spindle formation. EMBO Rep 2006; 7:418-24; PMID:16462731; http://dx.doi.org/10.1038/sj.embor.7400639
- Hu DL, Valentine M, Kidd VJ, Lahti JM. CDK11(p58) is required for the maintenance of sister chromatid cohesion. J Cell Sci 2007; 120:2424-34; http://dx.doi.org/10.1242/jcs.007963
- Li TY, Inoue A, Lahti JM, Kidd VJ. Failure to proliferate and mitotic arrest of CDK11(p110/p58)-null mutant mice at the blastocyst stage of embryonic cell development. Mol Cell Biol 2004; 24:3188-97; http://dx.doi.org/10.1128/Mcb.24.8.3188-3197.2004
- Beyaert R, Kidd VJ, Cornelis S, VandeCraen M, Denecker G, Lahti JM, Gururajan R, Vandenabeele P, Fiers W. Cleavage of PITSLRE kinases by ICE/CASP-1 and CPP32/CASP-3 during apoptosis induced by tumor necrosis factor. J Biol Chem 1997; 272:11694-7; PMID:9115219; http://dx.doi.org/10.1074/jbc.272.18.11694
- Shi JQ, Nelson MA. The cyclin-dependent kinase 11 interacts with NOT2. Biochem Bioph Res Co 2005; 334:1310-6; http://dx.doi.org/10.1016/j.brrc.2005.07.026
- Dickinson LA, Edgar AJ, Ehley J, Gottesfeld JM. Cyclin L is an RS domain protein involved in pre-mRNA splicing. J Biol Chem 2002; 277:25465-73; http://dx.doi.org/10.1074/jbc.M202266200
- Tannukit S, Wen X, Wang HJ, Paine ML. TFIP11, CCNL1 and EWSR1 protein-protein interactions, and their nuclear localization. Int J Mol Sci 2008; 9:1504-14; http://dx.doi.org/10.3390/ijms9081504
- Loyer P, Trembley JH, Grenet JA, Busson A, Corlu A, Zhao W, Kocak M, Kidd VJ, Lahti JM. Characterization of cyclin L1 and L2 interactions with CDK11 and splicing factors - Influence of cyclin L isoforms on splice site selection. J Biol Chem 2008; 283:7721-32; PMID:18216018; http://dx.doi.org/10.1074/jbc.M708188200
- Muller D, Millon R, Theobald S, Hussenet T, Wasylyk B, du Manoir S, Abecassis J. Cyclin LI (CCNLI) gene alterations in human head and neck squamous cell carcinoma. Br J Cancer 2006; 94:1041-4; PMID:16598186; http://dx.doi.org/10.1038/sj.bjc.6603036
- Li P, Ma YJ, Wang AG, Gong PT, Li JH, Yang J, Ouyang HS, Zhang XC. A fine balance between CCNL1 and TIMP1 contributes to the development of breast cancer cells. Biochem Bioph Res Co 2011; 409:344-9; PMID:21586274; http://dx.doi.org/10.1016/j.bbrc.2011.05.021
- Satyanarayana A, Kaldis P. Mammalian cell-cycle regulation: several Cdks, numerous cyclins and diverse compensatory mechanisms. Oncogene 2009; 28:2925-39; PMID:19561645; http://dx.doi.org/10.1038/onc.2009.170
- Choi HH, Choi HK, Jung SY, Hyle J, Kim BJ, Yoon K, Cho EJ, Youn HD, Lahti JM, Qin J, et al. CHK2 kinase promotes pre-mRNA splicing via phosphorylating CDK11(p110). Oncogene 2014; 33:108-15; PMID:23178491; http://dx.doi.org/10.1038/onc.2012.535
- Chi YY, Zhang CY, Zong HL, Hong Y, Kong XF, Liu HO, Zou WY, Wang YL, Yun XJ, Gu JX. Thr-370 is responsible for CDK11(p58) autophosphorylation, dimerization, and kinase activity. J Biol Chem 2011; 286:1748-57; PMID:21078675; http://dx.doi.org/10.1074/jbc.M110.107367
- Drogat J, Migeot V, Mommaerts E, Mullier C, Dieu M, van Bakel H, Hermand D. Cdk11-CyclinL controls the assembly of the RNA polymerase II mediator complex. Cell Rep 2012; 2:1068-76; http://dx.doi.org/10.1016/j.celrep.2012.09.027
- Yokoyama H, Gruss OJ, Rybina S, Caudron M, Schelder M, Wilm M, Mattaj IW, Karsenti E. Cdk11 is a RanGTP-dependent microtubule stabilization factor that regulates spindle assembly rate. J Cell Biol 2008; 180:867-75; PMID:18316407; http://dx.doi.org/DOI 10.1083/jcb.200706189
- Zhou Y, Han C, Li D, Yu Z, Li F, Li F, An Q, Bai H, Zhang X, Duan Z, et al. Cyclin-dependent kinase 11(p110) (CDK11(p110)) is crucial for human breast cancer cell proliferation and growth. Sci Rep 2015; 5:10433; PMID:25990212; http://dx.doi.org/10.1038/srep10433
- Jia B, Choy E, Cote G, Harmon D, Ye SN, Kan QC, Mankin H, Hornicek F, Duan ZF. Cyclin-dependent kinase 11 (CDK11) is crucial in the growth of liposarcoma cells. Cancer Lett 2014; 342:104-12; http://dx.doi.org/10.1016/j.canlet.2013.08.040
- Pan MH, Cai XJ, Liu M, Lv J, Tang H, Tan J, Lu C. Establishment and characterization of an ovarian cell line of the silkworm, Bombyx mori. Tissue Cell 2010; 42:42-6; http://dx.doi.org/10.1016/j.tice.2009.07.002
- Hall BG. Building Phylogenetic Trees from Molecular Data with MEGA. Mol Biol Evol 2013; 30:1229-35; PMID:23486614; http://dx.doi.org/10.1093/molbev/mst012
- Liu TH, Dong XL, Pan CX, Du GY, Wu YF, Yang JG, Chen P, Lu C, Pan MH. A newly discovered member of the Atlastin family, BmAtlastin-n, has an antiviral effect against BmNPV in Bombyx mori. Sci Rep 2016; 6:28946; PMID:27353084; http://dx.doi.org/10.1038/srep28946