ABSTRACT
An Aberration in megakaryopoiesis and thrombopoiesis, 2 important processes that maintain hemostasis, leads to thrombocytopenia. Though platelet transfusions are used to treat this condition, blood banks frequently face a shortage of platelets. Therefore, methods to generate platelets on a large scale are strongly desirable. However, to generate megakaryocytes (MKs) and platelets (PLTs) in numbers sufficient for clinical application, it is essential to understand the mechanism of platelet production and explore efficient strategies accordingly. We have earlier reported that the N-6 and N-3 poly-unsaturated fatty acids (PUFAs), Arachidonic acid (AA)/Docosahexanoic acid (DHA) have beneficial effect on the generation of MKs and PLTs from umbilical cord blood derived CD34+ cells. Here we tested if a similar effect is observed with peripheral blood derived CD34+ cells, which are more commonly used in transplantation settings. We found a significant enhancement in cell numbers, surface marker expression, cellular ploidy and expression of cytoskeletal components during PLT biogenesis in cultures exposed to media containing AA/DHA than control cultures that were not exposed to these PUFAs. The test cells engrafted more efficiently in NOD/SCID mice than control cells. AA/DHA appears to have enhanced MK/PLT generation through upregulation of the NOTCH and AKT pathways. Our data show that PUFAs could be valuable additives in the culture system for large scale production of platelets for clinical applications.
Introduction
Platelets have important and well-characterized roles in haemostasis and thrombosis,Citation1-3 maintenance of vascular integrity,Citation4 development of the lymphatic system,Citation5 and in innate immune response.Citation6 Any perturbations resulting in platelet number reduction leads to thrombocytopenia. This condition can arise as a consequence of chemotherapy, HIV infection, myelodysplastic syndrome, Immune thrombocytopenic purpura and bone marrow transplantation (BMT). Former and current treatment regimes for this disorder include platelet transfusion and administration of cytokines or thrombopoietin (TPO) mimetics. TPO mimetics were later found to generate neutralizing auto antibodies against endogenous TPO after haematopoietic stem cell transplant (HSCT), and their use was discontinued.Citation7 Platelet transfusion suffers from several limitations such as variability in quality and quantity of platelets, inability to freeze and store these platelets and short shelf life of platelets. Transfusion sometimes leads to bacterial infections and immunological reactions, and there is also frequently an acute shortage of platelets in blood banks.Citation8 This stresses the need for alternative approaches such as in vitro generation of MKs and platelets from HSCs. MK and platelets have been derived from CD34+ cells obtained from various sources like bone marrow (BM),Citation9-11 Umbilical cord blood(UCB)Citation12 and mobilized peripheral blood (MPB).Citation13-15 MK differentiation is controlled by various growth factors, transcription factors and cytokines. TPO is the primary regulator of megakaryopoiesisCitation16-17 whereas SCF, FLT-3, IL-3, IL-6 and IL-11 act as secondary regulators.Citation18 In attempts to further increase the efficiency of in vitro production of MKs and platelets various investigators have used additives like liquid crystal related compounds,Citation19 nicotinamide (vitamin B3),Citation20 heparin,Citation21 proteoglycans,Citation22 prostaglandins like 15-deoxy-delta-PGJ2.Citation23 Some investigators have used extracellular adhesive proteins like fibrinogen, fibronectin and VWFCitation24 to enhance platelet production.
Poly unsaturated fatty acids are important signaling mediators in many physiological and pathological conditions.Citation25 The n-6 linoleic acid (LA; 18:2n-6) and n-3 α-linolenic acid (ALA; 18:3n-3) are essential fatty acids which cannot be synthesized in the body. Arachidonic acid (20:4n-6) and Docosahexanoic acid (22:6n-3) are derivatives of LA and ALA respectively. They are further metabolized by different enzymes like cyclo-oxygenases (COX), lipoxygenase, and cytochrome P450 to give rise to different lipid mediators like prostaglandins (PG), leukotrienes (LT), thromboxanes (TX). All these compounds get incorporated into cell membrane and modulate the membrane functions.Citation26-27 PUFAs maintain platelet membrane structure and integrity and along with their metabolites they are known to regulate stem cell proliferation and regulation, cell cycle, survival, and induction of apoptosis.Citation28-33 Earlier we have reported that AA/DHA enhance in vitro generation of MK and PLT from CD34+ cells isolated from UCB. This beneficial effect was attributed to their antioxidant and anti-apoptotic properties. Citation34-35 In the present study, we determined if a similar effect would be observed with CD34+ cells from apheresis samples from healthy donors, since this source is more commonly used in BMT transplantations as compared with UCB or bone marrow. Apheresis sample collection is less invasive and less traumatic than bone marrow collection. Therefore peripheral blood HSCs differentiated to MKs and PLTs would be preferred for clinical applications.
Based on morphology, phenotype, ultrastructural and functional studies, we show here that AA/DHA addition to the culture medium results in enhancement of megakaryocytes and platelets. The mechanism of this beneficial effect could be attributed to the activation and upregulation of the Notch/AKT signaling pathways during the process of megakaryothrombopoiesis.
Results
AA/DHA promote Megakaryocyte differentiation
To evaluate Megakaryocyte differentiation,CD34+ cells were isolated from apheresis samples of healthy donors and were cultured in the presence of SCF and TPO,supplemented with or without 2 nutraceuticals i.e. arachidonic acid(AA) or docosahexanoic acid (DHA). The concentrations 200μM for AA and 1μM for DHA were selected from earlier work published from our group.Citation34 After 14 d of differentiation the total cells were harvested and viability was counted by trypan blue dye exclusion method, total cell yield was found to be 3-fold and 4-fold higher in AA and DHA respectively as compared with control () (*p ≤ 0.05, n = 3). Among the 2 test sets, DHA showed more cell yield than AA; however this difference was not statistically significant. The data suggests that AA and DHA also promote proliferation of cells. Change in morphology was also observed under the phase contrast microscope. Large granulated cells representing typical megakaryocyte morphology could be seen in all the 3 sets but their number/field was higher in AA/DHA as compared with control (). Details of morphology were further examined by scanning electron microscopy. shows extensions arising from mature megakaryocytes. These filopodia like structures were more pronounced in AA /DHA treated cells as compared with untreated control indicating that more pro-platelet like structures are formed in the presence of PUFAs. Megakaryocytes start expressing integrin CD41, CD61 and maturation marker CD42b on their surface upon differentiation from haematopoietic stem cells (HSCs). This specific marker expression was analyzed by flow cytometry. The expression of these markers 79.27%, 64.36% for CD41 and 80.53, 74.05% for CD61 in AA, DHA respectively was higher as compared with 39.87% for CD41 and 58.5% for CD61 for control as shown in representative FACS overlay (). The percentage of these markers was higher for AA as compared with DHA. The absolute number of cells expressing MK specific marker were significantly higher in test sets than control set () (n = 3) and percentage of MKs is shown in the Figure S1 (a). The percentage was higher for AA but the absolute number of cells expressing CD41 and CD42b markers was 1.42 and 1.84 fold higher for DHA as compared with AA whereas CD61 showed no difference. This shows that both AA/DHA favored MK differentiation but DHA had an upper edge over proliferation than AA. We also observed higher MFI with AA/DHA as additives as compared with control for CD41, CD61 and CD42b (Fig. S1b). This data reveals that AA and DHA favor megakaryocyte formation in comparison to control.
Figure 1. AA/DHA promotes Megakaryocyte differentiation – CD34+cells when grown in presence of AA/DHA favor megakaryocyte differentiation. (A) significantly higher TNC count in AA/DHA set (B). Morphology of cells in the 3 sets as seen under the Phase contrast microscope (40X) and (C) Scanning Electron microscope. (D) Representative overlay of one sample showing phenotypic marker expression for CD41,CD61 and CD42b of MKs on day 14 as labeled on X-axis respectively,the colors of overlay represents each set, blue- control, green- AA and orange-DHA. (E) Cumulative data from 3 samples.
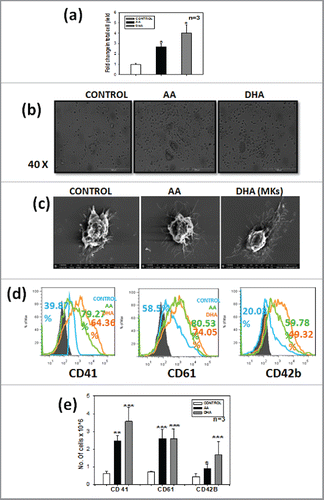
Presence of AA/DHA result in increased ploidy levels as evaluated by cell cycle analysis
Haematopoietic stem cells differentiate to megakaryocytes by undergoing a complex series of events. Endomitosis is one such key step of maturation in which the cell nuclei number increases from 2N upto 164N. In this study cellular ploidy was evaluated by propidium iodide staining. Data shows that AA/DHA promote higher ploidy i.e., cells having ploidy more than 4N as shown in representative flow profile ().The ploidy levels reached upto 16N in all the 3 sets but the percentage of cells in 4N, 8N and 16N were significantly higher in AA/DHA sets as compared with control (). This data was further confirmed by Wright's and Giemsa staining (). A Total of 500 cells were counted under the microscope. The number of large cells with polylobulated nuclei in AA (120 ± 3.1) and DHA (170 ± 3.4) were significantly higher than in control (40 ± 4.3) (). This suggests that AA /DHA promote megakaryocyte maturation. CFU-MK assay was performed further to evaluate the effect of AA/DHA on functionality of in vitro generated megakaryocytes. They were cultured in methylcellulose medium supplemented with a combination of growth factors as mentioned in methods. After 14 d, typical CFU-MK colonies with large size and spherical shape as shown in the inset image in were counted under the inverted microscope. AA (90 ± 12.4) and DHA (80 ± 9.0) show higher number of colonies than control (70 ± 14.1) and the difference was statistically significant (*p <0.05, n = 3).
Figure 2. Presence of AA/DHA result in increased ploidy levels as evaluated by cell cycle analysis – Increased ploidy was detected in AA /DHA set as compared with control on 14th day. (A) The histogram profile for one representative sample -upper panel and cumulative data for 3 different samples - lower panel. (B) Wright's and Giemsa stain show mature MKs, larger cells with polylobulated nuclei as shown by arrows (60X)-upper panel. Polyploid cells were found to be significantly higher in test set than control set-lower panel. (C) Significantly higher CFU-MK colonies were found in AA/DHA sets. Inset shows phase contrast image of a colony.
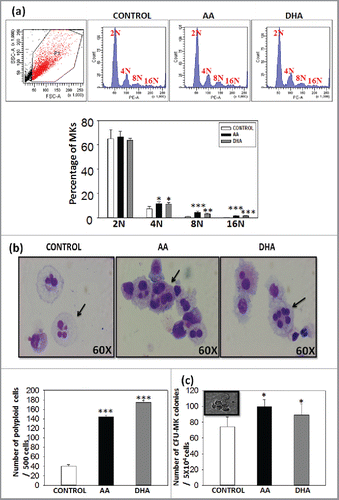
Cytoskeletal remodelling is induced in the presence of AA/DHA
After the process of endomitosis megakaryocytes extend pseudopodia like extensions. This process of proplatelet formation is dependent on microtubule and actin for increase in their length and branching respectively. They undergo polymerisation and rearrangement during MK maturation and platelet release. These cytoskeletal changes were also detected on the 14th day harvested cells by confocal microscopy. Phalloidin FITC staining shows F-actin organization which has higher intensity in test sets as compared with control . β-1 tubulin is a MK specific isoform which was also enhanced in the presence of AA and DHA along with α-tubulin .The mean fluorescence intensity was calculated by measuring intensity of cells in 10 random fields by Image J software and was found to be significantly higher in test sets than control set for actin, α-tubulin and for β-1 tubulin. Only for actin in DHA set the value did not reach significance. This data suggests that presence of AA and DHA induce active cytoskeletal remodelling during differentiation.
Figure 3. Cytoskeletal remodelling is induced in the presence of AA/DHA –Representative Immunofluoroscence images for (A) Actin (B) β-1 tubulin (C) α-tubulin. Cyan: nuclei stained with DAPI. Green: F-actin/ β-1 tubulin/α-tubulin. The last panel on the right is merged image of the 2 fluorescence images. Scale bar, 10µm. MFI for 10 random fields was calculated by using Image J software .AA/DHA show 1.5 to 3-fold higher MFI values than control.
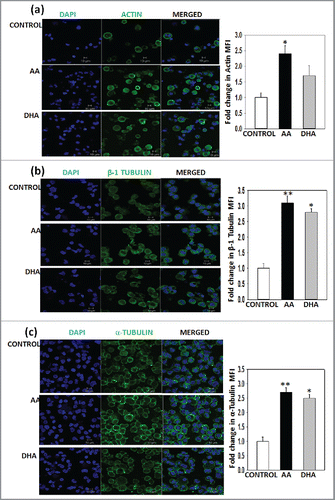
Presence of AA or DHA in the culture medium enhances platelet formation and function
Platelets are released from mature megakaryocytes during terminal differentiation. Culture derived platelets were harvested by centrifugation on day 14 and counted by using neubauer chamber. The data shows 2.8-fold and 4.4-fold increase in platelet number in AA and DHA set than control set respectively . Ultrastructure of culture derived platelets was studied by scanning electron microscopy as described in methods and acquired on FESEM instrument. The images show platelets with typical platelet morphology and their numbers were higher in test set as compared with control . Platelets were stained for CD41, CD61 and CD42b marker and analyzed by flow cytometry. Culture derived platelets were gated on peripheral blood platelet as a positive control shown in . shows representative histogram profile in which test set show higher percentage as compared with control set. The absolute number of platelets in test sets i.e., AA and DHA expressing CD41 2 and 2.5-fold, CD61 2.17 and 2.6-fold, CD42b 2.3 and 2.5-fold respectively higher than control and percentage of marker expression in platelets for 3 samples is mentioned in Figure S1(c). We also observed higher MFI for PLTs on day 14 with AA and DHA as additives on comparison to control MFI for CD41, CD61 and CD42b (Fig. S1d).Platelets are activated upon blood vessel injury due to release of extracellular matrix molecules. Due to their activation platelets migrate to the injured area and form a clot. Therefore we also checked the activation profile of culture derived platelets with 2 different agonist TRAP-6 or Type-1 collagen. Upon activation CD62p marker is expressed on the surface of platelets as detected by flow cytometry, it is representated by dot plot (Fig. S2).CD62p expression was 9.9 ± 1.7% and 7.8 ± 0.9% for AA and DHA set as compared with 2.5 ± 0.4% for control set for TRAP-6 and 9.2 ± 1.2% and 9.1 ± 1.6% for AA and DHA set as compared with 3.1 ± 1.2% for control set for Type I collagen . This data reveals that AA/DHA show better platelet functionality than control.
Figure 4. Presence of AA or DHA in the culture medium enhances platelet formation and function – Culture derived platelets were found to be significantly higher in test set than control set. (A) Total platelet yield (B) Scanning Electron microscopy (C) Cultured platelets were gated on peripheral blood platelet gate. Representative overlay showing percentage of CD41, CD61 and CD42b. (D) Cumulative data for 3 different samples. (E) and (F) Peripheral blood PLT and cultured PLTs were activated in the presence of 2 agonist TRAP-6 and Type-I collagen. The graph represents resting and activated platelets for 3 samples. The percentages of activated platelets are higher for test sets as compared with control set. (***p<0.001).
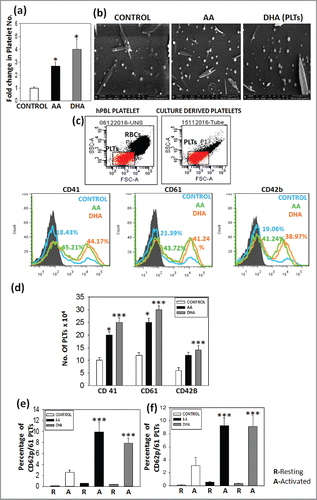
MKs generated in presence of AA/DHA show higher engraftment potential in NOD/SCID mice
In vitro generated MKs were infused in 4–6 week old NOD/SCID mice. The in vitro generated MKs and expanded CD34+ cells were coinfused in the ratio 4:1 in irradiated NOD-SCID mice. After 8 weeks, mice were killed and percentage of human cells engrafted in mouse bone marrow was analyzed by staining with human specific total leukocyte marker CD45 and mice CD45.1. We have first gated the cells positive for hCD45 against a background of mCD45.1 as shown in the . Then the hCD45 gated population was further observed for CD61 expression as shown by dot plot. Mice showing CD45/CD61 percentage ≥ 0.1% were considered positive for engraftment. The mean fluorescence Intensity of CD61 positive cells was calculated and was observed to be higher for AA/DHA as compared with control as seen in representative dot plot for each set . Cumulative data shows significant increase in CD61 MFI for AA/DHA than control in . The percent engraftment of hCD61 cells in gated CD45+ cells were significantly higher in test set than control set . The engraftment of human CD61 positive cells was 18.5 ± 0.9% for AA and 7.7 ± 0.36% for DHA and was significantly higher than control 3.6 ± 0.19%.The percent engraftment of hCD45 cells were significantly higher in test set than control set as shown in Fig. S3. The mice infused with only expanded CD34+ cells i.e., group I did not show any engraftment as compared with other 3 groups (II, III and IV) due to relatively lower number of cells infused during engraftment,(data not shown).
Figure 5. MKs generated in presence of AA/DHA show higher engraftment potential in NOD/SCID mice – (A) Engraftment of human cells in Bone marrow (BM) of NOD/SCID mice. The dot plot on lower panel shows higher expression of hCD61 cells in hCD45 gated cells of AA and DHA sets infused in NOD/SCID mice than control cells. (B) AA and DHA treated hCD61 cells also showed significantly higher MFI values (Graphical data). (C) The percent engraftment of hCD61 cells in gated CD45+ cells were significantly higher in test set than control set. (D) CFU-MK assay of BM cells of transplanted mice shows higher human CFU-MK colonies for mice receiving test cells than control cells. (***p<0.001, n = 4).
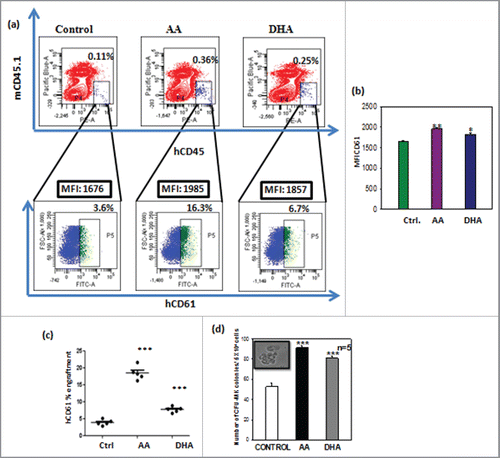
To further evaluate the human MKs engrafted in NOD-SCID mice, mouse BM cells were subjected to CFU-MK assay in vitro with human specific growth factors. The mice infused with cells expanded with AA and DHA show 91 ± 2, 81 ± 2.3 CFU-MK colonies respectively which were significantly higher than 52 ± 3 in control as shown in . The group I mice did not show any colonies as compared with other 3 groups. (Data not shown).
Presence of AA/DHA in cultures enhances MK specific transcription factors and modulates Notch signaling
Megakaryopoiesis and thrombopoiesis is regulated by various transcription factors either positively or negatively. GATA 1, GATA 2 and FOG-1 are required for early differentiation whereas NFE2 plays an important role during terminal differentiation. We also studied the same transcription factors at mRNA level by real time PCR. They were found to be upregulated in test set as compared with control, supporting our observation of the enhancement of platelet formation due to additives . Apart from transcription factors many other molecular mechanisms are involved in this process. Notch signaling is one of the important mechanisms that maintain equilibrium between progeny and differentiated population. Upon addition of AA and DHA, notch receptor Notch 1, ligand jagged 1, 2,Dll1 and their downstream target Hes 1 were upregulated as compared with control as shown by real time data in . Jagged 2 and Dll1 were also upregulated in test set than control set but it was not significant. This data confirms the role of Notch1 as a positive regulator of megakaryopoiesis. We performed immunofluoroscence studies to confirm these observations at protein level. Additional culture sets containing Notch inhibitor Gamma Secretase IX (GSIIX) 2 μM and AKT inhibitor (LY294002) 20μM were kept in these experiments. The expression of activated Notch1 (NICD) and Hes 1, AKT- the downstream target of notch and its activated form p-AKT along with MK marker CD41 was enhanced in AA/ DHA set as compared with control and was reduced in the presence of notch inhibitor GSIIX and AKT inhibitor LY294002. The mean fluorescence intensity was calculated for 10 random fields by image J software and was found to be significantly higher in AA and DHA set in comparison to control and reduced after inhibitor treatment as observed in graphs and immunofluoroscence images in Figs. S4-S8(a-b). These data suggests that AA / DHA enhance the production of MKs and platelet from HSCs by enhancing Notch1 and its downstream AKT pathway which is further confirmed by down regulation of these molecules upon inhibitor treatment.
Figure 6. Presence of AA/DHA in cultures enhances MK specific transcription factors and modulates Notch signaling - Upregulation of MK specific transcription factor and notch signaling in AA/ DHA cultured cells. Gene expression profile shows higher expression of (A) GATA 1, GATA2, NFE-2 and FOG-1 and (B) Notch1, Jagged 1, 2, DLL1 and Hes 1 for cells cultured in presence of AA/DHA as compared with control. Immunofluoroscence study was performed for (C) NICD (D) Hes1, (E) AKT and (F) P-AKT with CD41 in the absence and presence of inhibitors GSIIX and LY294002. Images were analyzed and MFI was calculated for 10 random fields by Image J software.
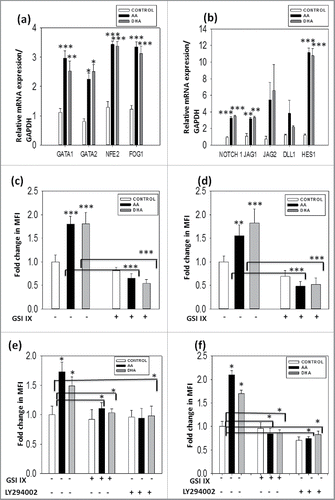
Taken together AA/DHA are useful nutraceuticals that promote megakaryopoiesis and thrombopoiesis.
Discussion
Thrombocytopenia is a major problem occurring during stem cell transplantation, chemotherapy and other hematological conditions. Platelet transfusion is widely used for treatment of such patients. However, due to the frequent shortage of platelets in blood banks, in vitro generation of platelets for clinical use becomes necessary. Many investigators worldwide are trying to achieve this goal but with limited success.Citation36-40 This is because the process of megakaryocyte and platelet formation is very complex and the mechanism is not yet completely understood. Two main approaches for in vitro MK production are genetic manipulations or altering media components. The main concern with the first approach is the safety issue regarding clinical use of this transdifferentiated MKs into patients.Citation41-42 Therefore many investigators opt for modifying the culture media components.Citation43-44 In a similar attempt, we have earlier shown that additives like AA/DHA in culture media enhance MK and platelet generation. We had attributed this beneficial effect to prevention of ROS and apoptosis.Citation34-35 The source used was CD34+ cells derived from UCB. However in a clinical scenario, autologous/ allogeneic apheresis samples are preferred for transplantation. Therefore in the present study we used PBL derived CD34+ cells as the starting source. These cells were obtained by GCSF induced mobilization in healthy patients. Isolation of CD34+ cells through administration of G-CSF is a less invasive procedure than the usual bone marrow harvest; rather it is the most preferable one.Citation45 According to the recent finding by Bernitz et al. (Accepted February 6, 2017, Blood 2017: blood-2016–11–752923;doi:https://doi.org/10.1182/blood-2016-11-752923) mobilized PB has surpassed harvested BM as a source of HSCs for clinical transplantation.Citation46 G-CSF mobilizes dormant haematopoietic stem cells from bone marrow to peripheral blood without proliferation. G-CSF treatment does not induce detectable engraftment of CD41high cells from bone marrow compartment limiting their regenerative properties. These cells mature to megakaryocyte only when cultured invitro. Thus, this data indicates there is no modulation of megakaryocyte potential of cells isolated after GCSF treatment.
Further, Lemoli et al. in early 1997 published a report comparing the colony forming unit cells (CFU-C) and LTC-IC assay for CD34+ cells isolated from Bone marrow and mobilized peripheral blood after GCSF treatment from the same donor.Citation47 The results obtained show no difference in their functional and kinetic potential even after GCSF mobilization and behave similar to CD34+ cells present in the bone marrow. Circulating and BM megakaryocyte precursors also show the same clonogeneic efficiency (CFU-MK) when cultured in the presence of other growth factors in vitro. This data clearly indicates that GCSF treatment does not induce any changes in megakaryocyte potential of mobilized CD34+ cells from bone marrow and also holds true in our system.
Peripheral blood HSCs upon differentiation with PUFAs as additives express higher MK and PLT specific surface markers as compared with control. Rasolva et.alCitation48 and Mattia et.alCitation49 have given a correlation between ploidy levels and the degree of MK maturation for releasing platelets. We also report that PUFAs enhance the ploidy level of MKs. Scanning electron microscope images show augmented proplatelet like protrusions in the presence of PUFAs as compared with control. Arachidonic acid stimulates cell adhesion by p38 MAP kinase pathway and it is also involved in direct remodelling of actin by differentially regulating RhoA and CDC42 GTPase.Citation50-51 These reports suggest some role of arachidonic acid in cytoskeletal reorganization. In agreement with these findings, we observed cytoskeletal remodelling upon addition of PUFAs to the culture medium which favors terminal differentiation. Apart from invitro study, in vivo assays are also required to study the transplantation potential of in vitro generated megakaryocyte progenitors toward platelet formation. Lia E. Perez et al. has reported NOD/SCID mice as the in vivo model to study human megakaryocytopoiesis and platelet production by xenogeneic transplantation of human PBSCs.Citation52 MR Tijssen et al. observed increased platelet formation due to combined transplantation of MKs derived from mobilized peripheral blood and unmanipulated CD34+ cells into NOD/SCID mice.Citation53 We show that engraftment of combined infusion of AA/DHA expanded MKs and expanded CD34+ cells further increase platelet formation as well as number of CFU-MK colonies as compared with only growth factor expanded CD34+ cells in vivo in NOD/SCID mice. This kind of study will be helpful in overcoming the problem of delayed platelet recovery after autologous and allogeneic transplantation.
We found that PUFAs not only enhance platelet number and surface marker expression, their functionality was also improved as confirmed by platelet activation in the presence of TRAP-6 and TYPE-I collagen. PUFAs and their metabolites like eicosanoids, prostaglandins are known to increase proliferation and modulate stem cell fate to different lineages. They activate PI3K/AKT, MAPK pathways during stem cell proliferation or differentiation. Notch signaling is one of the imperative pathways activated during development to maintain a balance of progenitor and differentiated population. Notch signaling regulates and influences the cell fate decisions by keeping the HSCs in an undifferentiated state and enhancing their self-renewal, regulating cellular differentiation. However, its role in haematopoietic stem cell lineage specification is not clear. It has been shown that Notch/delta1 positively regulates megakaryocyte formation from HSCsCitation54 as opposed to Notch/delta4 which negatively regulates its terminal differentiation to platelet.Citation55 Notch signaling affects the development of erythroid/megakaryocytic cells. They favor megakaryocytopoiesis over erythropoiesis.Citation56-58 Recently Mercher et al showed that Notch signaling plays an important role during megakaryopoiesis.Citation59 Earlier work from our lab where cord blood CD34+ cells were differentiated to megakaryocyte and platelets there also the role of Notch signaling was investigated, by checking for Notch-1, Notch-2, Notch-3 and Notch-4 transcript levels. We found an upregulation of transcript level of Notch −1 and its ligand Jagged-1, in the sets cultured with DHA/AA as compared with control. However there was no difference in gene expression of Notch 2 between control/DHA/AA sets, Whereas, Notch-3 and Notch-4 mRNA levels were undetectable (Unpublished Data).The preliminary data thus indicated that probably DHA/ AA modulates Notch-1 signaling. Delineation of the exact signaling mechanism that is involved was not studied at that time.
Therefore, we tried to delineate mechanism of beneficial effect of PUFAs by dissecting Notch signaling pathway. As per our expectation we indeed found an upregulation in the expression of Notch1, its ligand Jagged 1and downstream target gene Hes1 at mRNA level. There are reports on involvement of AKT signaling in megakaryopoiesis.Citation60 Signorello et.al have reported platelet activation by 2-AG (2 arachidonyl glycerol) stimulating arachidonic acid release through activation of PI3K/AKT pathway.Citation61 AKT lies downstream to Notch and it elevates AKT phosphorylation during murine megakaryopoiesis.Citation62,63 Since we found a positive role for Notch we were encouraged to investigate the involvement of its downstream AKT and Phospho–AKT in our culture system. They were also found to be upregulated at protein level as seen by immunofluoroscence studies. Our observation was further confirmed by use of Notch and AKT inhibitors. Thus PUFAs enhance megakaryopoiesis and thrombopoiesis through upregulation of NOTCH-AKT pathway. Further studies are required to evaluate the effect of specific metabolite products of these 2 PUFAs on specific subpopulations of HSCs that undergo MK differentiation.
Taken together, AA/DHA show a promising effect toward regulating megakaryocyte and platelet generation from CD34+ cells of apheresis sample. They can be used as culture media additives to scale up platelet production for clinical application.
Material and methods
Reagents
Serum-free medium Stem-pro (10639–011) and Dynal CD34+ Isolation kit (11301D) was purchased from Invitrogen. All human recombinant growth factors, such as TPO (300–18), SCF (300–07), IL-3(200–03), IL-6(200–06), and Flt-3 (300–19) were purchased from Peprotech Inc. The 2 poly-unsaturated fatty acids arachidonic acid (AA (A3555)) and Docosahexanoic acid (DHA (D2534).) were purchased from Sigma-Aldrich. Optimal concentrations of the nutraceuticals (AA 200 μM, DHA 1 μM) were selected from the earlier work published from our laboratory.Citation44-45 Methylcellulose was purchased from Sigma. γ-Secretase inhibitor GSI IX (565770, Calbiochem) and AKT inhibitor LY294002 (9901,Cell signaling technology) were used at a concentration of 2 μM and 20 μM respectively.
Isolation of CD34+ cells from apheresis samples
Apheresis samples from healthy donors were obtained from local hospitals after informed consent with the compliance of the institutional review board (IEC-Institutional ethical committee –NCCS and IC-SCR –Institutional Committee for Stem Cell Research, NCCS) according to Declaration of Helsinki. Consenting procedures were also approved by the NCCS - IC-SCR- Institutional Committee for Stem Cell Research, NCCS. The apheresis sample was collected in sterile heparinised bottles and processed within 24 h of collection. Mono-nuclear cells were frozen in medium (70% Iscove' s modified Dulbecco ' s medium (IMDM)+ 20% Fetal bovine serum (FBS) + 10% Dimethyl sulphoxide (DMSO)) by Mr. Frosty and stored in liquid Nitrogen containers (−196°C).Cells were revived and CD34+ cells were isolated from them through positive selection using Dynal magnetic beads according to the manufacturer's instructions.
Ex vivo expansion of MK progenitors
CD34+ cells were seeded in 24-well plates at a seeding density of 5 × 104 cells/well/ml in Stem-pro serum free medium. Growth factors SCF and TPO were used at a final concentration of 50 ng/ml each. Cells were cultured with (test cells) and without (control cells) the addition of either DHA (1 μM) or AA (200 μM) for 14 d and feeding with growth factors and additives was done once a week without any media change. Viable cell count of harvested cells in each set was estimated by trypan blue dye exclusion method.
Morphologic and phenotypic analysis
Phase contrast images of cells were taken on day 14 under inverted microscope. The harvested cells on day 14 were subjected to cytospin and stained with Wright's and Giemsa stain. Cells having typical features of megakaryocytic lineage, i.e., very large cells with poly-lobulated nuclei containing characteristic cytoplasmic granulation were counted as MKs. A total of 500 cells were counted under oil immersion microscope (Olympus) and the number of MKs scored was normalized to the total cell yield.
Cells were analyzed for the expression of surface markers such as CD41a, CD61 and CD42b by flow cytometry. Cells were washed with 1X phosphate-buffered saline (PBS) containing 0.1% bovine serum albumin (BSA) and 0.1% azide. They were then incubated with the specific antibody or an appropriate isotype control for 45 min at 4°C. The antibodies used for flow cytometry were anti-human CD61-PE (555754), CD42b-APC (551061), CD41a-APC (559777), CD62p-APC (550888) were purchased from BD PharMingen (Becton Dickinson). The cells were washed to remove excess antibody, then fixed using 1% paraformaldehyde solution and kept at 4°C till analyzed. A minimum of 10,000 events was acquired for each sample on FACS Canto II, (BD) flow cytometer.
Electron microscopy
MKs as well as PLTs were harvested on day 14 as described earlier. Smears were prepared on silicon wafers and air-dried. The smears were washed 3 times with 1X PBS. They were fixed with 2.5% glutarladehyde prepared in Na-phosphate buffer, pH = 7.2. Smears were incubated at room temperature for 30 minutes. Smears were washed 3 times with Na-phosphate buffer. Samples were dehydrated by different ethanol volumes starting from 10%, 30%, 50%, 70%, 80%, 90% and 100% for 10 minutes at room temperature. Then the samples were air-dried and added on SEM stub after gold sputtering. The samples were acquired on FESEM instrument.
Functional analysis of cultured MK
Colony-forming unit-MK assay
The culture media contained 30% human platelet-poor AB plasma plus growth factors in Iscove's modified Dulbecco's medium (IMDM; Gibco BRL Grand Island); 5 × 104 cells were seeded in methyl cellulose with growth factors (IL-3 and IL-6 at 10 ng/mL each, and TPO and SCF at 50 ng/mL each) in low adherence 35-mm plastic petriplates (Stem cell tech.).The plates were incubated at 37 °C in a humidified atmosphere containing 5% CO2 for 14 d. After 14 d of culture, typical MK colonies, with large cell size, spherical shape and refractile nature were counted under an inverted microscope.
Cellular ploidy
Cultured cells were harvested on day 14 and washed with 1X PBS. The cells were fixed with 70% ethanol. Thereafter the cells were incubated for 1 h at 4°C with Propidium iodide (50μg/ml) in a medium containing RNAase (100 ug/ml) and were analyzed by flow cytometry.
Platelet characterization
PLTs generated in culture were isolated by centrifugation at 120 g for 10 min; supernatant was again pelleted at 1000 g for 10 min. Platelets were characterized phenotypically by flow cytometry for the presence of surface markers CD41, CD61 and maturation marker CD42b.
Platelet activation
To study their functionality, PLTs were stimulated at 37°C with or without 5μM TRAP-6 (3497,Tocris Bioscience) or 10μM Type I collagen (C3867,Sigma) for 15 min in calcium containing HBSS, then fixed in an equal volume of 0.1% paraformaldehyde for 1 h and incubated with both anti-human CD62P-APC and anti-human CD61-PE antibodies. After incubation, cells were washed with PBS, and were analyzed on a flow cytometer.
Reverse-transcription – polymerase chain reaction (RT-PCR)
The mRNA was isolated by TRIzol™ method and reverse transcribed (RT) to cDNA strands, which were then used as templates for polymerase chain reactions (PCR). The primer sequences (IDT) used is listed in Fig. S9.
Immunofluoroscence study
Antibodies used for Immunofluorescence study Hes-1(11988), AKT (92725) were purchased from Cell Signalling Technology (CST) and NICD (ab8925), P-AKT (ab66138), CD41 (ab11024) from abcam. Ph-FITC (F-432), α-tubulin (138000) from Invitrogen and β-1 tubulin (ab11312) was purchased from Abcam. Harvested cells were stained with CD41 as MK marker and then with other protein markers like activated notch (NICD), Hes-1 and AKT and P-AKT. Actin, α-tubulin, β-1 tubulin staining was done for cytoskeletal changes. The cells were subjected to cytospin on glass slides, washed with 1X PBS and were fixed in 4% paraformaldehyde and blocked with1% PBS-BSA-azide for 1 h at RT. After rinsing 3 times with PBS, the cells were then permeabilized with 0.2% Triton X-100 in PBS for 20 min. Then cells were stained at 4°C overnight with monoclonal antibodies mentioned above. After washing, the appropriate secondary antibody Goat anti mouse Alexa 488 and donkey anti rabbit Alexa 594 was applied at RT for 1 h. After washing step, nuclei were counter- stained with DAPI and after final washing; the cells were mounted in mounting medium. Immunofluorescence images were obtained using Zeiss LSM 510 Meta laser scanning microscope.
Engraftment in NOD/SCID mice
Protocols for animal experiments were approved and in accordance with the IAEC-NCCS Institutional Animal Ethical Committee-NCCS/CPCSEA- Committee for the Purpose of Control and Super vision of Experiments on Animals. (Approval number: IAC UC-Institutional Animal Care and Use Committee, EAF-Experimental Animal Facility/2015/B-252). Throughout the experiments animals were under the super vision of trained personnel. NOD/SCID mice were obtained from Jack-son Laboratories and bred in the NCCS animal facility. For engraftment studies, mice at 4 – 6 weeks of age were irradiated with 350 rads of sub-lethal dose from a CoCitation60 source (Gamma chamber 5000, BRIT, Mumbai). The mice were divided into 4 groups:-
Group I-2 × 105 expanded CD34 +cells only
Group II-2 × 105 expanded CD34 +cells + 8 × 105 control MK progenitors (1:4 ratio).
Group III- 2 × 105 expanded CD34 +cells + 8 × 105 AA MK progenitors (1:4 ratio).
Group IV- 2 × 105 expanded CD34 +cells + 8 × 105 DHA MK progenitors (1:4 ratio).
CD34+ were expanded for 10 d with SCF, TPO, Flt3 and IL-6, each added at a final concentration of 50 ng/ml. Five mice were kept in each group. All sets of mice were killed after 8 w and their bone marrow cells were harvested. For phenotypic analysis, cells were stained with antihuman CD61-PE antibody and antihuman CD45-FITC antibody (P7687Sigma). Cells were then analyzed by flow cytometry.
CFU-MK assay was performed on mouse bone marrow cells using human-specific growth factors and methyl cellulose as described earlier. For this the cells were seeded at a density of 5 × 104 cells/plate. The experimental design for in vivo engraftment assay is shown in Fig. S10.
Statistical analysis
All values are expressed as means ± SEM for at least 3 independent experiments. The statistical differences between groups were analyzed using a one-way repeated measure analysis of variance (one-way RM ANOVA) using the software SIGMA STAT and Probability values of p ≤ 0.05 (∗), p ≤ 0.01 (∗∗) and p ≤ 0.001 (∗∗∗) were considered statistically significant.
Abbreviations
AA | = | arachidonic acid |
APC | = | allophycocyanin |
DHA | = | Docosahexanoic acid |
MKs | = | Megakaryocytes |
PE | = | phycoerythrin |
PLTs | = | platelets |
PUFAs | = | poly-unsaturated fatty acids |
TPO | = | Thrombopoietin |
TRAP-6 | = | thrombin receptor activating peptide- 6 |
Disclosure of potential conflicts of interest
The authors declare no competing financial interests.
Supplemental_materials.zip
Download Zip (11.8 MB)Acknowledgments
The authors would like to thank Dr. S.C.Mande, Director, NCCS, for support, Mrs. Nikhat Khan for technical help, and NCCS core facilities- Flow cytometry, Immunofluorescence Microscopy Facility and experimental animal facility, Central Instrumentation Facility at Savitribai Phule pune university for electron microscopy and Dr Jyoti Rao for proofreading the manuscript.
Funding
The University Grants Commission (UGC), Government of India, New Delhi, is thanked for doctoral fellowship of AD.
References
- Chang Y, Bluteau D, Debili N, Vainchenker W. From hematopoietic stem cells to platelets. J Thromb Haemost 2007; 5 Suppl 1:318-27. Review; PMID:17635743; http://dx.doi.org/10.1111/j.1538-7836.2007.02472.x
- Italiano JE Jr. Unraveling mechanisms that control platelet production. Semin Thromb Hemost 2013; 39(1):15-24; PMID:23266965
- Thon JN, Italiano JE. Platelet formation. Semin Hematol 2010; 47(3):220-6; PMID:20620432; http://dx.doi.org/10.1053/j.seminhematol.2010.03.005
- Leslie M. Cell biology. Beyond clotting: the powers of platelets. Science 2010; 328(5978):562-4; PMID:20430990; http://dx.doi.org/10.1126/science.328.5978.562
- Verheul HM, Hoekman K, Luykx-de Bakker S, Eekman CA, Folman CC, Broxterman HJ, Pinedo HM. Platelet: transporter of vascular endothelial growth factor. Clin Cancer Res 1997; 3(12 Pt 1):2187-90; PMID:9815613
- Semple JW, Italiano JE Jr, Freedman J. Platelets and the immune continuum. NatRev Immunol 2011; 11(4):264-74; http://dx.doi.org/10.1038/nri2956
- Peeters K, Stassen JM, Collen D, Van Geet C, Freson K. Emerging treatments for thrombocytopenia: increasing platelet production. Drug Discov Today 2008; 13(17–18):798-806; PMID:18602017; http://dx.doi.org/10.1016/j.drudis.2008.06.002
- Lambert MP, Sullivan SK, Fuentes R, French DL, Poncz M. Challenges and promises for the development of donor-independent platelet transfusions. Blood 2013; 121(17):3319-24; PMID:23321255; http://dx.doi.org/10.1182/blood-2012-09-455428
- Falcieri E, Bassini A, Pierpaoli S, Luchetti F, Zamai L, Vitale M, Guidotti L, Zauli G. Ultrastructural characterization of maturation, platelet release, and senescence of human cultured megakaryocytes. Anat Rec 2000; 258(1):90-9; PMID:10603452; http://dx.doi.org/10.1002/(SICI)1097-0185(20000101)258:1%3c90::AID-AR10%3e3.0.CO;2-G
- Nagler A, Eldor A, Naparstek E, Mumcuoglu M, Slavin S, Deutsch VR. Ex vivo expansion of megakaryocyte precursors by preincubation of marrow allografts with interleukin-3 and granulocyte-macrophage colony-stimulating factor in vitro. Exp Hematol 1995; 23(12):1268-74; PMID:7589281
- Schattner M, Lefebvre P, Mingolelli SS, Goolsby CL, Rademaker A, White JG, Foster D, Green D, Cohen I. Thrombopoietin-stimulated ex vivo expansion of human bone marrow megakaryocytes. Stem Cells 1996; 14(2):207-14; PMID:8991540; http://dx.doi.org/10.1002/stem.140207
- Li X, Chen FP, Liu J, Wu XH, Jiang TB, Tang XY. In vitro differentiation into megakaryocytes and generation of platelets from CD34+ cells of umbilical cord blood. Zhong Nan Da Xue Bao Yi Xue Ban 2006; 31(5):776-81. Chinese
- Bertolini F, Battaglia M, Pedrazzoli P, Da Prada GA, Lanza A, Soligo D, Caneva L, Sarina B, Murphy S, Thomas T, et al. Megakaryocytic progenitors can be generated ex vivo and safely administered to autologous peripheral blood progenitor cell transplant recipients. Blood 1997; 89(8):2679-88; PMID:9108385
- Lefebvre P, Winter JN, Meng Y, Cohen I. Ex vivo expansion of early and late megakaryocyte progenitors. J Hematother Stem Cell Res 2000; 9(6):913-21; PMID:11177605; http://dx.doi.org/10.1089/152581600750062363
- Maurer AM, Liu Y, Caen JP, Han ZC. Ex vivo expansion of megakaryocytic cells. Int J Hematol 2000; 71(3):203-10. Review; PMID:10846824
- Wendling F. Thrombopoietin: its role from early hematopoiesis to platelet production. Haematologica 1999; 84(2):158-66. Review; PMID:10091415
- Cramer EM, Norol F, Guichard J, Breton-Gorius J, Vainchenker W, Massé JM, Debili N. Ultrastructure of platelet formation by human megakaryocytes cultured with the Mpl ligand. Blood 1997; 89(7):2336-46; PMID:9116277
- Lazzari L, Henschler R, Lecchi L, Rebulla P, Mertelsmann R, Sirchia G. Interleukin-6 and interleukin-11 act synergistically with thrombopoietin and stem cell factor to modulate ex vivo expansion of human CD41+ and CD61+ megakaryocytic cells. Haematologica 2000; 85(1):25-30; PMID:10629587
- Terasawa R, Fukushi Y, Monzen S, Miura T, Takahashi K, Yoshizawa A, Kashiwakura I. The promoting activity on human megakaryocytopoiesis and thrombopoiesis by liquid crystal-related compounds. Biol Pharm Bull 2009; 32(6):976-81; PMID:19483301; http://dx.doi.org/10.1248/bpb.32.976
- Giammona LM, Fuhrken PG, Papoutsakis ET, Miller WM. Nicotinamide (vitamin B3) increases the polyploidisation and proplatelet formation of cultured primary human megakaryocytes. Br J Haematol 2006; 135(4):554-66; PMID:17054670; http://dx.doi.org/10.1111/j.1365-2141.2006.06341.x
- Feng Y, Zhang L, Xiao ZJ, Li B, Liu B, Fan CG, Yuan XF, Han ZC. An effective and simple expansion system for megakaryocyte progenitor cells using a combination of heparin with thrombopoietin and interleukin-11. Exp Hematol 2005; 33(12):1537-43; PMID:16338497; http://dx.doi.org/10.1016/j.exphem.2005.08.010
- Kashiwakura I, Takahashi K, Monzen S, Nakamura T, Takagaki K. Ex vivo expansions of megakaryocytopoiesis from placental and umbilical cord blood CD34(+) cells in serum-free culture supplemented with proteoglycans extracted from the nasal cartilage of salmon heads and the nasal septum cartilage of whale. Life Sci 2008; 82(19–20):1023-31; PMID:18407295; http://dx.doi.org/10.1016/j.lfs.2008.03.001
- O'Brien JJ, Baglole CJ, Garcia-Bates TM, Blumberg N, Francis CW, Phipps RP. 15-deoxy-Delta12, 14 prostaglandin J2-induced heme oxygenase-1 in megakaryocytes regulates thrombopoiesis. J Thromb Haemost 2009; 7(1):182-9; PMID:18983509; http://dx.doi.org/10.1111/j.1538-7836.2008.03191.x
- Balduini A, Pallotta I, Malara A, Lova P, Pecci A, Viarengo G, Balduini CL, Torti M. Adhesive receptors, extracellular proteins and myosin IIA orchestrate proplatelet formation by human megakaryocytes. J Thromb Haemost 2008; 6(11):1900-7; PMID:18752571; http://dx.doi.org/10.1111/j.1538-7836.2008.03132.x
- Harris W. Omega-6 and omega-3 fatty acids: partners in prevention. Curr OpinClin Nutr Metab Care 2010; 13(2):125-9; http://dx.doi.org/10.1097/MCO.0b013e3283357242
- Langelier B, Linard A, Bordat C Lavialle M, Heberden C. Long chain-polyunsaturated fatty acids modu-late membrane phospholipid composition and protein localization in lipid rafts of neu-ral stem cell cultures. J Cell Biochem 2010; 110:1356-64; PMID:20564231; http://dx.doi.org/10.1002/jcb.22652
- Lefils J, Géloën A, Vidal H, Lagarde M, Bernoud-Hubac N. Dietary DHA: time course of tissue uptake and effects on cytokine secretion in mice. Br J Nutr 2010; 104(9):1304-12; PMID:20487585; http://dx.doi.org/10.1017/S0007114510002102
- Krishnamurti C, Stewart MW, Cutting MA, Rothwell SW. Assessment of omega-fatty-acid-supplemented human platelets for potential improvement in long-term storage. Thromb Res 2002; 105(2):139-45; PMID:11958804; http://dx.doi.org/10.1016/S0049-3848(02)00009-9
- Mutanen M, Freese R. Polyunsaturated fatty acids and platelet aggregation. Curr Opin Lipidol 1996; 7(1):14-9. Review; PMID:8925182; http://dx.doi.org/10.1097/00041433-199602000-00004
- Kang JX, Wan JB, He C. Concise review: Regulation of stem cell proliferation and differentiation by essential fatty acids and their metabolites. Stem Cells 2014; 32(5):1092-8. Review; PMID:24356924; http://dx.doi.org/10.1002/stem.1620
- Lee MY, Ryu JM, Lee SH, Park JH, Han HJ. Lipid rafts play important role for maintenance of embryonic stem cell self-renewal. J Lipid Res 2010; 51(8):2082-9; PMID:20110442; http://dx.doi.org/10.1194/jlr.M001545
- Yamazaki S, Iwama A, Takayanagi S, Morita Y, Eto K, Ema H, Nakauchi H. Cytokine signals modulated via lipid rafts mimic niche signals and induce hibernation in hematopoietic stem cells. EMBO J 2006; 25(15):3515-23; PMID:16858398; http://dx.doi.org/10.1038/sj.emboj.7601236
- Lands B. Consequences of essential fatty acids. Nutrients 2012; 4(9):1338-57. Review; PMID:23112921; http://dx.doi.org/10.3390/nu4091338
- Siddiqui NF, Shabrani NC, Kale VP, Limaye LS. Enhanced generation of megakaryocytes from umbilical cord blood-derived CD34(+) cells expanded in the presence of two nutraceuticals, docosahexanoic acid and arachidonic acid, as supplements to the cytokine-containing medium. Cytotherapy 2011; 13(1):114-28; PMID:20230224; http://dx.doi.org/10.3109/14653241003588858
- Shabrani NC, Khan NF, Kale VP, Limaye LS. Polyunsaturated fatty acids confer cryoresistance on megakaryocytes generated from cord blood and also enhance megakaryocyte production from cryopreserved cord blood cells. Cytotherapy 2012; 14(3):366-80; PMID:22250991; http://dx.doi.org/10.3109/14653249.2011.649186
- De Bruyn C, Delforge A, Martiat P, Bron D. Ex vivo expansion of megakaryocyte progenitor cells: cord blood versus mobilized peripheral blood. Stem Cells Dev 2005; 14(4):415-24; PMID:16137231; http://dx.doi.org/10.1089/scd.2005.14.415
- Matsunaga T, Tanaka I, Kobune M, Kawano Y, Tanaka M, Kuribayashi K, Iyama S, Sato T, Sato Y, Takimoto R, et al. Ex vivo large-scale generation of human platelets from cord blood CD34+ cells. Stem Cells 2006; 24(12):2877-87; PMID:16960134; http://dx.doi.org/10.1634/stemcells.2006-0309
- Luo Q, Song G, Zou C. A two-phase culture system for megakaryocyte differentiation of human mobilized peripheral blood CD34+ cells. Sheng Wu Yi XueGong Cheng Xue Za Zhi 2010; 27(2):373-8. Chinese
- Simmons PJ, Robinson SN, Munsell MF, Thomas MW, Javni JA, Brouard N, Zweidler-McKay PA, Shpall EJ. Expression of a surface antigen (MA6) by peripheral blood CD34+ cells is correlated with improved platelet engraftment and May explain delayed platelet engraftment following cord blood transplantation. Stem Cells Dev 2015; 24:1066-72
- Ivetic N, Nazi I, Karim N, Clare R, Smith JW, Moore JC, Hope KJ, Kelton JG, Arnold DM. Producing megakaryocytes from a human peripheral blood source. Transfusion 2016; 56(5):1066-74; PMID:26756864; http://dx.doi.org/10.1111/trf.13461
- Ono Y, Wang Y, Suzuki H, Okamoto S, Ikeda Y, Murata M, Poncz M, Matsubara Y. Induction of functional platelets from mouse and human fibroblasts byp45NF-E2/Maf. Blood 2012; 120(18):3812-21; PMID:22855609; http://dx.doi.org/10.1182/blood-2012-02-413617
- Pulecio J, Alejo-Valle O, Capellera-Garcia S, Vitaloni M, Rio P, Mejía-Ramírez E, Caserta I, Bueren JA, Flygare J, Raya A. Direct Conversion of Fibroblasts to Megakaryocyte Progenitors. Cell Rep 2016; 17(3):671-683; PMID:27732845; http://dx.doi.org/10.1016/j.celrep.2016.09.036
- Van den Oudenrijn S, von dem Borne AE, de Haas M. Influence of medium components on ex vivo megakaryocyte expansion. J Hematother Stem Cell Res 2001; 10(1):193-200; PMID:11276373; http://dx.doi.org/10.1089/152581601750098516
- Norol F, Vitrat N, Cramer E, Guichard J, Burstein SA, Vainchenker W, Debili N. Effects of cytokines on platelet production from blood and marrow CD34+ cells. Blood 1998; 91(3):830-43; PMID:9446643
- Hölig K. G-CSF in Healthy Allogeneic Stem Cell Donors. Transfus Med Hemother 2013; 129(14):1901-1912; PMID:24179471; http://dx.doi.org/10.1159/000354196
- Bernitz JM, Daniel M, Fstkchyan YS, Moore K. Granulocyte-colony stimulating factor mobilizes dormant hematopoietic stem cells without proliferation in mice. Blood 2017
- Lemoli RM, Tafuri A, Fortuna A, Petrucci MT, Ricciardi MR, Catani L, Rondelli D, Fogli M, Leopardi G, Ariola C, et al. Cycling status of CD34+ cells mobilized into peripheral blood of healthy donors by recombinant human granulocyte colony-stimulating factor. Blood 1997; 89 No 4:1189-96; PMID:9028941
- Raslova H, Kauffmann A, Sekkaï D, Ripoche H, Larbret F, Robert T, Tronik LeRoux D, Kroemer G, Debili N, Dessen P, et al. Interrelation between polyploidization and megakaryocyte differentiation: a gene profiling approach. Blood 2007; 109(8):3225-34; PMID:17170127; http://dx.doi.org/10.1182/blood-2006-07-037838
- Mattia G, Vulcano F, Milazzo L, Barca A, Macioce G, Giampaolo A, Hassan HJ. Different ploidy levels of megakaryocytes generated from peripheral or cord bloodCD34+ cells are correlated with different levels of platelet release. Blood 2002; 99(3):888-97; PMID:11806991; http://dx.doi.org/10.1182/blood.V99.3.888
- Roberts LA, Glenn H, Hahn CS, Jacobson BS. Cdc42 and RhoA are differentially regulated during arachidonate-mediated HeLa cell adhesion. J Cell Physiol 2003; 196(1):196-205; PMID:12767056; http://dx.doi.org/10.1002/jcp.10303
- Garcia MC, Williams J, Johnson K, Olden K, Roberts JD. Arachidonic acid stimulates formation of a novel complex containing nucleolin and RhoA. FEBS Lett 2011; 585(4):618-22; PMID:21281639; http://dx.doi.org/10.1016/j.febslet.2011.01.035
- Perez LE, Rinder HM, Wang C, Tracey JB, Maun N, Krause DS. Xenotransplantationof immunodeficient mice with mobilized human blood CD34+ cells provides an in vivo model for human megakaryocytopoiesis and platelet production. Blood 2001; 97(6):1635-43; PMID:11238102; http://dx.doi.org/10.1182/blood.V97.6.1635
- Tijssen MR, van Hennik PB, di Summa F, Zwaginga JJ, van der Schoot CE, Voermans C. Transplantation of human peripheral blood CD34-positive cells in combination with ex vivo generated megakaryocytes results in fast platelet formation in NOD/SCID mice. Leukemia 2008; 22(1):203-8; PMID:17943170; http://dx.doi.org/10.1038/sj.leu.2404979
- Lam LT, Ronchini C, Norton J, Capobianco AJ, Bresnick EH. Suppression of erythroid but not megakaryocytic differentiation of human K562 erythro leukemic cells by notch-1. J Biol Chem 2000; 275(26):19676-84; PMID:10783395; http://dx.doi.org/10.1074/jbc.M002866200
- Poirault-Chassac S, Six E, Catelain C, Lavergne M, Villeval JL, Vainchenker W, Lauret E. Notch/Delta4 signaling inhibits human megakaryocytic terminal differentiation. Blood 2010; 116(25):5670-8; PMID:20829371; http://dx.doi.org/10.1182/blood-2010-05-285957
- Zheng C, Yang R, Han Z, Zhou B, Liang L, Lu M. TPO-independent megakaryocytopoiesis. Crit Rev Oncol Hematol 2008; 65:212-22; PMID:18093840; http://dx.doi.org/10.1016/j.critrevonc.2007.11.003
- Cortin V, Pineault N, Garnier A. Ex vivo megakaryocyte expansion and platelet production from human cord blood stem cells. Methods Mol Biol 2009; 482:109-26; PMID:19089352
- Sun L, Hwang WY, Aw SE. Biological characteristics of megakaryocytes: specific lineage commitment and associated disorders. Int J Biochem Cell Biol 2006; 38:1821-6; PMID:16730215; http://dx.doi.org/10.1016/j.biocel.2006.03.011
- Mercher T, Cornejo MG, Sears C, Kindler T, Moore SA, Maillard I, Pear WS, Aster JC, Gilliland DG. Notch signaling specifies megakaryocyte development from hematopoietic stem cells. Cell Stem Cell 2008; 3:314-26; PMID:18786418; http://dx.doi.org/10.1016/j.stem.2008.07.010
- Majka M, Ratajczak J, Villaire G, Kubiczek K, Marquez LA, Janowska-Wieczorek A, Ratajczak MZ. Thrombopoietin, but not cytokines binding to gp130 protein-coupled receptors, activates MAPKp42/44, AKT, and STAT proteins in normal human CD34+ cells, megakaryocytes, and platelets. Exp Hematol 2002; 30:751-60
- Signorello MG, Leoncini G. Effect of 2-arachidonoylglycerol on myosin light chain phosphorylation and platelet activation: The role of phosphatidylinositol 3kinase/AKT pathway. Biochimie 2014; 105:182-91; PMID:25068972; http://dx.doi.org/10.1016/j.biochi.2014.07.014
- Roy A, Basak NP, Banerjee S. Notch1 intracellular domain increases cytoplasmicEZH2 levels during early megakaryopoiesis. Cell Death Dis 2012; 3:e380; PMID:22914328; http://dx.doi.org/10.1038/cddis.2012.119
- Cornejo MG, Mabialah V, Sykes SM, Khandan T, Lo Celso C, Lopez CK, Rivera-Muñoz P, Rameau P, Tothova Z, Aster JC, et al. Crosstalk between NOTCH and AKT signaling during murine megakaryocyte lineage specification. Blood 2011; 118(5):1264-73; PMID:21653327; http://dx.doi.org/10.1182/blood-2011-01-328567