ABSTRACT
The Tousled Like kinases (TLKs) are involved in numerous cellular functions, including the DNA Damage Response (DDR), but only a handful of substrates have been identified thus far. Through a novel proteomic screen, we have now identified 165 human proteins interacting with TLK1, and we have focused this work on NEK1 because of its known role in the DDR, upstream of ATR and Chk1. TLK1 and NEK1 were found to interact by coIP, and their binding is strengthened following exposure of cells to H2O2. Following incubation with doxorubicin, TLK1 and NEK1 relocalize with nuclear repair foci along with γH2AX. TLK1 phosphorylated NEK1 at T141, which lies in the kinase domain, and caused an increase in its activity. Following DNA damage, addition of the TLK1 inhibitor, THD, or overexpression of NEK1-T141A mutant impaired ATR and Chk1 activation, indicating the existence of a TLK1>NEK1>ATR>Chk1 pathway. Indeed, overexpression of the NEK1-T141A mutant resulted in an altered cell cycle response after exposure of cells to oxidative stress, including bypass of G1 arrest and implementation of an intra S-phase checkpoint.
Introduction
The human Tousled kinases (TLKs) are involved in DNA repair and are frequently overexpressed in several cancers (rev inCitation1). From a proteomic screen to identify the targets of TLK1B, we have identified NEK1, an important kinase that mediates the DNA damage response (DDR), thereby opening up a new avenue for a possible role of TLKs in dealing with the damage.
The ability of cells to sense and signal DNA damage is critical for genomic stability, and defects in this process are intimately linked to genomic instability and cancer etiology. In human cells, 2 checkpoint kinases, ATM and ATR are central effectors of the DNA damage relay.Citation2 Whereas ATM primarily responds to double-stranded breaks (DSB), ATR activity is elicited by a variety of DNA damaging agents and replication stress,Citation3 typically resulting in regions of ssDNA. ATR functions in a complex with its activating partner ATRIP.Citation4 RPA-coated ssDNA, a common intermediate of DNA replication and repair, plays a key role in recruiting and activating ATR-ATRIP, as well as recruiting the Rad9-Rad1-Hus1 (9–1–1) complex,Citation5,Citation6 another effector of the DDR that we identified as being regulated by TLK1.Citation7,Citation8 Once activated ATR phosphorylates its downstream effector kinase, Chk1, and together orchestrate the repair pathway and the cell cycle checkpoint. While the ATR-Chk1 kinases constitute a backbone of the DDR, other protein kinases modulate the strength of the response, including ATM, CDKs, PLK1, AKT, and important to this proposal, NEK1 (Never in Mitosis A-related kinase 1)Citation9-11 and TLK1.Citation7,Citation12,Citation13
Tousled Like kinases (TLK1 and TLK2) are serine/threonine kinases, which localize to the cytoplasm and nucleus and are known to autophosphorylate and dimerize.Citation14 Our laboratory identified a splice variant of TLK1 that encodes a 65 kDa protein (named TLK1B) that becomes translationally upregulated in response to genotoxic stress, including IR.Citation13 TLK1B is similar to TLK1 but lacks the first 169 aa. TLK1 and TLK1B share the same catalytic domain and we often refer them to as TLK1/1B. TLK1B overexpression protects cells from the genotoxic effects of IR.Citation12 Until now only a few direct interacting substrates of TLK1/1B have been identified, primarily histone H3, the histone chaperone Asf1, and Rad9.Citation7,Citation15,Citation16 As expected from the interacting substrates various studies have shown that TLKs play an important role in chromatin assembly, DNA repair, transcription, and chromosome segregation.Citation17-19 Knockout of the single TLK in Drosophila and C. elegans leads to an arrest in embryonic development,Citation17,Citation18 and expression of a dominant negative mutant in mouse cells causes missegregation of chromosomes resulting in aneuploidy.Citation20 TLK1/1B overexpression is observed in breast cancer, prostate cancer and cholangiocarcinoma, which often corresponds to reduced sensitivity toward chemotherapy due to efficient repair in those tumors (rev inCitation1). TLKs are regulated in a cell-cycle dependent manner and are maximally active during the S phase Citation21 and M.Citation22 Recently, we and others have found that TLK1 and TLK2 play critical roles in DDR recovery,Citation8,Citation23 and as guardians of the genome.Citation1 Hence, it was critical to identify additional substrates/interacting partners of TLK1/1B to elucidate the function of these proteins in the DDR.
The founding member of the NIMA family of protein kinases was originally identified in Aspergillus nidulans as a protein kinase essential for mitosis.Citation24 NIMA related kinases have adapted to a variety of cellular functions in addition to mitosis.Citation11 In human cells, 11 NEKs were identified that are involved in several functions. For example NEK2 is critical for centrosome duplication,Citation11 whereas NEK6, 7, 9 are regulators of the mitotic spindle and cytokinesis.Citation25 NEK1,Citation9,Citation10,Citation26 and NEK4Citation27 have been linked to the DDR and DNA repair pathways. NEK1 mediates Chk1 activation via an unclear mechanism since 2 papers reported opposite results using siRNA depletion experiments. One paper reported that NEK1 functions independently of ATR and does not affect ATR activity.Citation10 The other reported that NEK1 associates with ATR-ATRIP and primes ATR for the DDR.Citation28 NEK1 activity and relocalization to nuclei was reported to increase upon a variety of genotoxic stresses.Citation9,Citation10 A defect in DNA repair in NEK1-deficient cells is suggested by persistence of DSBs after low dose IR. NEK1-deficient cells fail to activate the checkpoint kinases Chk1 and Chk2, and fail to arrest properly at G1/S or G2/M-phase checkpoints after DNA damage.Citation26 NEK1-deficient cells suffer major errors in mitotic chromosome segregation and cytokinesis, and become aneuploid.Citation29 Genomic instability is also manifested in NEK1+/− mice, which late in life develop lymphomas with a higher incidence than wild type littermates.Citation29 However, it is not known what regulates NEK1 activity and its nuclear and cytoplasmic functions. Recently NEK1 was found to phosphorylate Rad54 at S572 regulating its activity in HR that in turn promotes the dissociation of Rad51 from DNA filaments during HR in G2 or during S at stalled forks.Citation30 We propose that TLK1, which we herein identify as interacting strongly with NEK1, is a key regulator of its activity, particularly in the DDR.
Results
Identification of TLK1B interacting proteome
To identify additional TLK1B interacting proteins, we obtained from Life Technologies a proteomic interaction study using biotinylated TLK1B to interrogate their ProtoArray system (9000 full length and native human proteins arrayed in duplicates on glass slides). The result included 165 proteins identified with high confidence. In Table S1 the hits are shown with signal intensity, negative signal (streptavidin only), concentration of TLK1B used in the assay, Z-score, variance between the 2 spots, and CI P-value (Chebyshev's Inequality P-value). The proteins were grouped by function with Reactome pathway database (http://www.reactome.org/) (), and the majority of hits were proteins involved in the cell cycle or DNA repair, although clearly TLK1B interacts with proteins involved in a variety of functions.
Table 1. Functional pathway interactions hierarchal order (Reactome Pathway Analysis)
For DNA repair, the proteins are in order of signal intensity: NEK1; APEX2; UBR2; RAD54; Deoxynucleotidyltransferase; LATS1; AlkB; FANCM; UVSSA. From these, we decided to focus our immediate attention on NEK1. NEK1 was reported to be a key modulator and interacting partner of ATR/ATRIP 28, and we had already identified a pathway by which TLK1 could regulate ATR via modulation of Rad9 activity.Citation8
NEK1 interacts with TLK1B
We have confirmed the interaction of TLK1B with NEK1, as GST-TLK1B pull-down () and by reciprocal immunoprecipitation of TLK1 and NEK1 (). In addition, we found that the association of TLK1 with NEK1 is strengthened by DNA damage (H2O2 which induces SSBs and DSBs). The function of the interaction of TLK1/1B with NEK1 remains to be established. With 2 previously known substrates of TLK1/1B (Asf1 and Rad9) the TLK1 protein functioned both as a chaperone and as a kinase, with distinct activities depending on the reactions under study.Citation7,Citation8,Citation31 The NEK1 protein typically migrated as 2 well-separated bands, and the slower migrating form can be converted to the faster form by treatment with phosphatase CIP (). It remains to be established if the phosphorylated form of NEK1 is the catalytically more active and if its phosphorylation is somehow regulated by TLK1/1B.
Figure 1 . TLK1B binds NEK1. (A) NEK1 was pulled-out with GST-TLK1B, but not GST, from 0.3 mg of Hek293 cell extract. (B) Immunoprecipitated NEK1 was treated with CIP to establish the mobility of the phosphorylated form. (C) The interaction between TLK1 and NEK1 is strengthened upon DNA damage. Hek293 cells were exposed to 0.2 mM H2O2 for the indicated time. Extracts were prepared and immunoprecipitated with TLK1 antiserum and probed for coIP with NEK1 serum, or for TLK1 (bottom). EXT (cell extract).
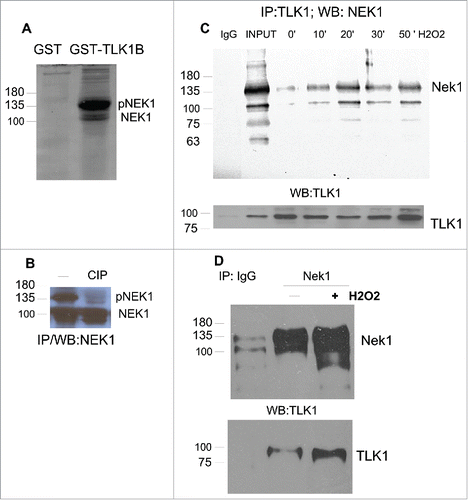
TLK1 and NEK1 relocalize with γH2AX nuclear foci after induction of DSBs
We determined by IF the localization of TLK1, NEK1, and pH2AX (γH2AX) in untreated cells or cells treated for 4h with doxorubicin, which results in production of DSBs that rapidly become decorated with pH2AX. After DNA damage, NEK1, which is mostly cytoplasmic in untreated cells (), relocalizes to the nuclei at sites of damageCitation10 and colocalizes with pH2AX staining. The NEK1 signal also increases as it was reported that its expression rapidly increases in presence of DSBs.Citation32 In undamaged cells, the distribution of TLK1 is equally localized in both cytoplasm and nuclei () as previously reported.Citation16 Upon induction of DSBs with doxorubicin, the expression of the TLK1B splice variants is rapidly induced,Citation13 resulting in the increased IF signal in these cells. We previously reported that, in contrast to the TLK1 isoform, TLK1B preferentially localizes to nuclei.Citation16 Therefore, treatment with doxorubicin results in both overall IF signal increase and in particular nuclear, although simple relocalization of TLK1/1B may also occur and explain the observation. Using a different NEK1 antibody (a mouse monoclonal) we were also able to show that a large fraction of TLK1 colocalizes in the nuclei with NEK1 after treatment with doxorubicin (), further demonstrating that the 2 proteins interact more avidly in presence of DSBs. In conclusion, both NEK1 and TLK1 colocalize preferentially to nuclei and with pH2AX upon induction of DSBs. Since we have shown that the TLK1-NEK1 interaction is strengthened upon DNA damage, it is likely that they both form a complex at sites of damage, decorated with pH2AX.
Figure 2. Colocalization of TLK1, NEK1, and γH2AX at sites of DNA damage following incubation with doxorubicin. A. Cells treated with or without doxorubicin (3uM) and then processed for IF as described in.Citation8 Cells were imaged on the Perkin elmer Mantra scope (20x). Raw files were obtained using the InForm. Colocalization layover was applied using InForm software which generates a pseudo pixel (yellow) where the 2 fluors colocalize. Antibodies used were: Rabbit anti-NEK1, Rabbit anti-TLK1, mouse anit γH2AX. B. Microscopy was performed following treatment with doxorubicin. Imaged were obtained at 60X and analyzed with Nikon software. Colocalization of NEK1 and TLK1 is shown in pink.
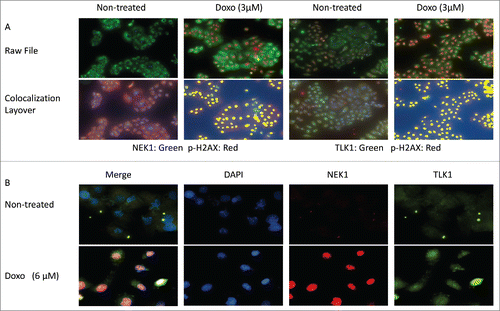
TLK1B phosphorylates NEK1 in the NT-kinase domain
NEK1 was identified as a dual specificity kinase during a screen to identify proteins phosphorylated on Tyr.Citation33 NEK1 contains an N-terminal kinase domain of approximately 260 aa, while a prominent portion of the NEK1 middle and C-tail is composed of several predicted coiled coil domains that were reported to promote dimerization and autophosphorylationCitation33 as well as interaction with other proteins.Citation34
To determine if TLK1B can phosphorylate NEK1 directly, we produced all recombinant proteins: GST-TLK1B; His-NEK1-FL (full length); His-NT-NEK1 amino-terminus kinase domain (first 480 aa); and His-CT-NEK1 (carboxy terminus 777 aa containing the coiled-coil repeats). NEK1 isolated from bacteria was already autophosphorylated (see below) and had very weak additional autophosphorylation with γ-ATPCitation32 (see ). Thus, we were able to carry out phosphorylation studies with TLK1B. In , we show that TLK1B phosphorylated very efficiently the NEK1-NT but weakly the NEK1-CT. A parallel western blot shows the NEK1-CT and NEK1-NT purified proteins.
Figure 3. TLK1 phosphorylates in vitro preferentially NEK1N-terminal fragment (NT). 3 µg of Ni-Sepharose purified recombinant NEK1-CT and NEK1-NT proteins expressed in bacteria following induction with IPTG were phosphorylated in a kinase reaction using 50 ng recombinant TLK1B and γP32-ATP. The samples were separated by SDS/PAGE and either exposed to film (autoradiography) or immunoblotted with anti-His antibody.
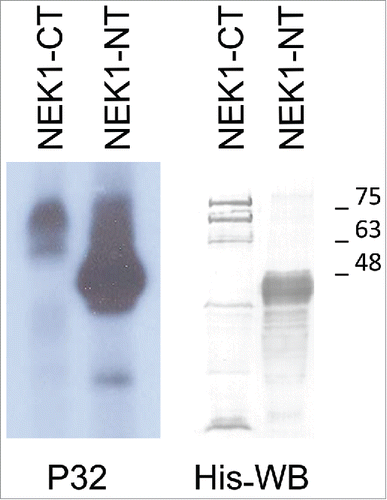
TLK1B phosphorylates NEK1-NT at T141
We performed phosphorylation reactions with FL-NEK1 or NT-NEK1 and GST-TLK1B, to determine the phosphorylation sites by LC-MS of tryptic digests (see Methods). This analysis revealed that the purified NEK1 protein in itself was autophosphorylated at several previously identified sites (PhosphoSitePlus), namely, T156, S417; and was additionally phosphorylated at S14 and S299, that are sites not reported previously (see Sup. Table 2 + Mass Spec Sup data). In the phosphorylation reaction with TLK1B, 2 additional phosphorylation sites were identified in both FL-NEK1 and NT-NEK1: T141 and Y315. Y315 was previously held as a putative autophosphorylation site of NEK1, whereas T141 is a novel site, which we attribute directly to the activity of TLK1B. In addition, since Y315 was not detected in the reaction with NEK1 alone, the simplest explanation is that the phosphorylation of T141 by TLK1B resulted in activation of the NEK1 kinase and autophosphorylation at Y315. Indeed it is noteworthy that TLK1B, which we previously showed acted primarily on the NT-NEK1, phosphorylated specifically T141 of NEK1 that lies within the kinase domain and may activate it.
We should stress that our analysis was somewhat limited since the peptide coverage was only 26% for the NT-kinase domain fragment (and 11% for the full length protein) and it is therefore possible that we have not identified all the sites phosphorylated by TLK1. However, additional site-directed mutagenesis work indicated that T141 is the main phosphorylation target of TLK1B (see below).
Kinase activity with recombinant NEK1 protein and its modulation by TLK1
We first asked with recombinant proteins if the activity of NEK1 can be regulated by TLK1B. The activity of NEK1 is typically determined using β-casein as the substrate, whereas TLK1 does not phosphorylate casein efficiently.Citation21 In fact, recombinant TLK1 even at high concentration (100 ng/µl) phosphorylated very weakly casein . NEK1 at that concentration phosphorylated casein efficiently, maximally between 10 and 15 minutes, but its activity was greatly stimulated when co-incubated with a small amount of TLK1B (5 ng/µl). Even after only 5 minutes of incubation, the signal was stronger than 15 minutes w/o TLK1B. This also suggested that TLK1B rapidly activates NEK1.
Figure 4. TLK1B addition stimulates the NEK1 kinase activity in vitro. (A) Kinase reactions (see methods) contained 100 ng/µl of the indicated recombinant proteins and 250 ng/µl β-casein as the substrate. The supplemented reaction with NEK1 contained 5 ng/µl TLK1B. After separation on a 10% PAGE, the gel was stained with coomassie blue (CB), dried and exposed to XR film for 4 h. (B) Phosphorylation of NEK1 by TLK1B occurs on T141. In kinase reactions, 1 µg of recombinant NEK1 or the NEK1-T141A mutant were incubated in absence or presence of 50 ng TLK1B. After separation on an 8% PAGE, the gel was stained with coomassie blue (CB), dried and exposed to XR film for 4 h. Note that the autophosphorylation of NEK1 was similar and weak for both wt and T141A NEK1 proteins. (C) The NEK1-T141A mutant kinase is not activated by incubation with TLK1B. Reactions were like in (A) but in addition we tested the activity of NEK1-T141A kinase.
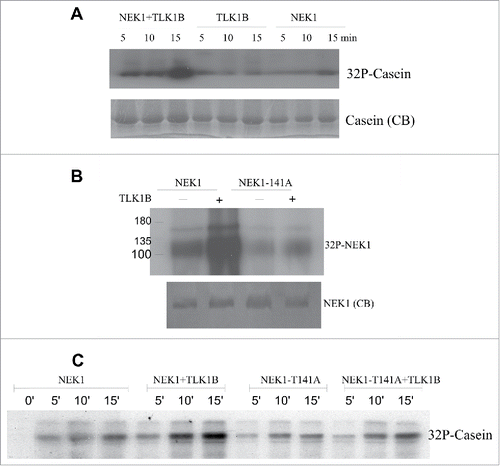
To confirm that TLK1B phosphorylates NEK1primarily at T141, we prepared recombinant NEK1-T141A to compare the extent of phosphorylation (radioactivity incorporation) by recombinant TLK1B respect to wt NEK1. Addition of a small amount of TLK1B (5 ng/µl) resulted in a strong increase in labeling in wt NEK1, suggesting either that NEK1 is a direct substrate of TLK1B eliciting P32 incorporation (at T141) or that TLK1B promotes the autophosphorylation of NEK1. However, the phosphorylation of NEK1-T141A was not increased following incubation with TLK1B (), therefore indicating that T141 is the primary phosphorylation target of TLK1B.
The increased activity of NEK1 in presence of TLK1B could be due to either an activating phosphorylation event (pT141), or simply via an interaction of the 2 proteins leading to a conformational change in NEK1 and increased activity. To establish which mechanism is more likely, we repeated the experiment in panel A, but using both wt NEK1 or NEK1-T141A recombinant proteins. Phosphorylation of casein was again used as readout for NEK1 activity. In , we show that the addition of a small amount of TLK1B resulted in a strong stimulation of wt NEK1 activity (just as in ). However, the addition of TLK1B to NEK1-T141A had a minimal effect on the activity of NEK1, indicating that the stimulation of NEK1 by TLK1B is most likely due its phosphorylation at T141.
The activity of NEK1 is regulated by the Cell Cycle and DNA damage (doxorubicin)
Although there is no direct evidence that human NEKs are essential for mitotic entry, unlike NIMA in the fungus Aspergillus nidulans, it is clear that several NEK family members have important roles in cell cycle control. To our knowledge, it has not been reported what is the pattern of activity of NEK1 during the cell cycle. In contrast, maximal activity of TLK1 was reported to occur during S-phase and at G2/M,Citation21,Citation22 and we wondered if a similar pattern of activity could be found for NEK1. To test this, cells were arrested at the G1/S boundary with hydroxyurea (HU), and then released into S-phase after washing with fresh medium. Finally, the cells were arrested in M-phase with nocodazole (see details in Materials and methods). Cell extracts from the different cell cycle phases () were prepared and equal amounts of protein were used to immunoprecipitate (IP) NEK1. Equal aliquots of the IP were then used to carry out kinase reactions, with 10 µCi γ-ATP32 and β-casein as the substrate, during a 15 minutes time course removing equal amounts at 5 minute intervals. shows that the kinase activity of IP-NEK1 was weak in G1-arrested cells, and increased in cells isolated from S and M phases, similar to what has been reported for TLK1 (the expression of NEK1 did not change during the cell cycle). It thus seems possible that both TLK1 and NEK1 may be required for specific functions during DNA replication and then mitosis, although it does not prove that the activity of TLK1 and NEK1 are linked. Unfortunately, it is difficult to test directly if TLK1 modulates the activity of NEK1 during the cell cycle since depletion of TLK1 with siRNA results in a S-phase arrest.Citation20
Figure 5. (A and B). The activity of NEK1 is cell cycle regulated. DU145 cells were arrested enriched in G1/S with HU, synchronously released in S after washing with fresh medium, and then arrested in M with nocodazole (see panel B). Cell extracts from equal cell numbers were prepared and NEK1 was isolated by IP (see methods) and tested for activity using β-casein as the substrate (panel A). C) The activity of NEK1 increases following addition of doxorubicin, but reduced by concomitant addition of THD. Cells were treated as described in Methods, and NEK1 was IP'd and tested for activity in vitro using β-casein.
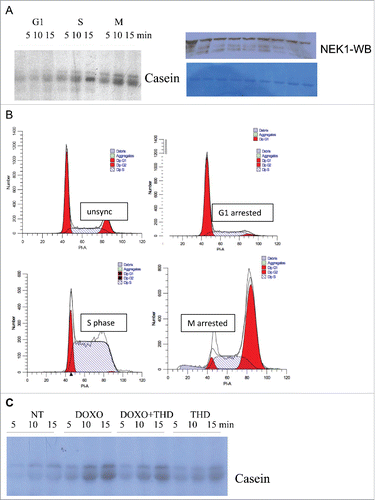
The activity of NEK1 was reported to increase in response to various types of DNA damage.Citation10 This is confirmed in , where NEK1 was IP'd from cells treated with doxorubicin (3 µM) and then tested in vitro for kinase activity. DNA damage with doxorubicin found NEK1 to be significantly more active than when isolated from untreated cells (NT). Whereas treatment of cells with THD, a specific inhibitor of TLK1 ,Citation35 had little effect on the activity of IP-NEK1, the addition of THD to cells treated with doxorubicin significantly reduced the activation of NEK1.
Overexpression of inactive TLK1B mutants found in cancers results in loss of phosphorylated NEK1
We wondered if dominant expression of kinase inactive mutants of TLK1B would have an effect on the pattern of phosphorylation of NEK1, as determined by mobility shift. We thus decided to focus on spontaneous TLK1 mutations found in cancers. There are, so far, 225 heterozygous mutations of TLK1 reported in COSMIC and we focused on 6 that were expected to affect the kinase activity (see ). We generated HEK293 stable cell lines overexpressing mutants and wild-type (WT) TLK1B in the pIRES2 vector, after selection for high GFP positive cells. Hek293 cells express TLK1 and not TLK1B, but we are aware that this experiment can work only if the TLK1B mutants have a dominant negative effect over the endogenous TLK1. Two frame-shift truncations (G622* and A583*) that encompass the kinase domain, and the mutant G417C in the consensus ATP binding pocket, resulted in loss of the phosphorylated NEK1 band (). We do not know if this phosphorylated form is the result of autokinase activity that is altered or if it is directly due to TLK1B acting on NEK1 as a substrate.
Phosphorylation of NEK1 by TLK1 is important for the DDR and cell cycle checkpoint
It was reported that NEK1 associates with ATR/ATRIP and primes it for activation in response to a variety of genotoxic agents, and that siRNA knockdown of NEK1 leads to a reduction in phospho-Chk1.Citation28 To determine the potential contribution of TLK1 to this pathway that converges on NEK1>ATR>Chk1, as a first experiment, we compared the activation of ATR following addition of hydroxyurea (HU) with that in cells simultaneously tread with thioridazine (THD, inhibitor of TLK1). We followed ATR autophosphorylation of T1989, which is a very early event in the activation of ATR. It was reported that cells lacking NEK1 fail to support ATR autophosphorylation at T1989.Citation28 In , we show that THD strongly inhibited the phosphorylation of ATR after treatment of cells with HU. We knew from a previous kinome studyCitation35 that THD does not affect ATR activity directly, and we suggest that the mechanism is indirect and via reduced activation of NEK1 owing to inhibition of TLK1 by THD.
Figure 7. (A) The activation of ATR (autophosphorylation at T1989) after 6h incubation with hydroxyurea (HU) is strongly reduced by concomitant addition of the TLK1 inhibitor, thioridazine (THD, 10 µM). (B) Activation of ATR (pATR-T1989) is impaired following 1h incubation with 0.2 mM H2O2 in cells expressing NEK1-T141A in contrast to wt-NEK1 expressing cells. Where indicated, siRNA to nek1 (si1) was added 24h before H2O2. (C) Activation of Chk1 (pChk1-S345) is reduced in cells overexpressing NEK1-T141A.
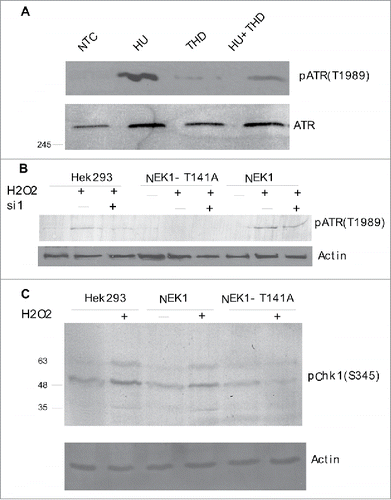
To establish more directly if the phosphorylation of NEK1-T141 has an effect on the DDR, we compared the activation of ATR and Chk1 in Hek293 cells overexpressing wt NEK1 or the T141A mutant. To activate the DDR, the cells were treated for 30 min with H2O2 (0.2mM) to induce genomic damage, and where indicated cells were pretreated for 24h with siRNA to NEK1 (si1, see also ). shows that H2O2 in Hek293 cells activated ATR (pATR-T1989) but the induction was reduced after treatment with si1. Cells expressing NEK1-T141A treated with H2O2 were unable to induce pATR. In contrast, cells expressing siRNA-resistant NEK1 induced pATR with H2O2. shows that cells overexpressing the T141A mutant are unable to activate Chk1, consistent with our proposed role for TLK1-mediated modulation of NEK1 activity via phosphorylation of T141.
Figure 8. (A) Expression of endogenous NEK1 in control H3k293 (293) cells and in and stably transfected NEK1 overexpressing constructs. The control and NEK1 overexpressing cells (siRNA resistant constructs) were treated for 18h with 2 different siRNAs, and cell extracts were prepared and immunoblotted for NEK1 and actin. (B) Hek293 controls and cells overexpressing wt-NEK1 or the NEK1-T141A mutants were treated (or not) with si1 to knockdown endogenous NEK1. Cells were then treated with H2O2 for 4h, as described in text, and then processed for cell cycle analysis with PtdIns staining.
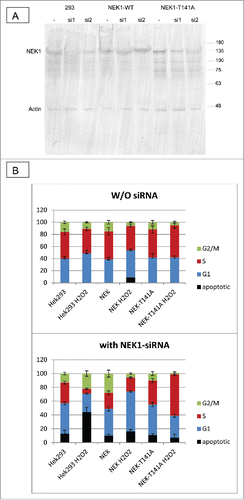
To establish the consequences of the NEK1-T141A mutation on the cell cycle after genotoxic stress, we repeated the treatment with H2O2 in the absence of the endogenous NEK1 after depletion with siRNA. Two different siRNAs to the 3’UTR of NEK1 were used to knockdown its expression), and si1, but not si2, was effective at reducing the expression of NEK1 by >80% ( top. As expected, the overexpressed wt-NEK1 and NEK1-T141A were resistant to the si1. Note also that the T141A mutant migrated slightly faster than the overexpressed wt-NEK1, although the difference is not as dramatic as in for the dominant KD-TLK1B mutants. It is possible that the overexpressed NEK1-T141A is phosphorylated at different compensatory sites in the absence of the important T141 site.
We thus treated the 3 cell lines (control, and overexpressing wt-NEK1 or T141A) with H2O2 for 4h, with and without pre-treatment of one day with si1, and determined the cell cycle distribution with PI and FACS analysis ( bottom). Without knockdown of the endogenous NEK1, the control Hek293 cells treated with H2O2 displayed a modest increase in G1 cells and corresponding reduction in S-phase cells. In cells overexpressing wt-NEK1, the same effect was observed. In contrast, cells overexpressing NEK1-T141A displayed an increase in S-phase cells with a matching decrease of the G2/M population, suggesting that the G1 checkpoint is bypassed. Much more pronounced effects were seen in cells pre-treated with si1. Firstly, the parental Hek293 cells treated with H2O2 became prominently apoptotic and with a nearly complete loss of the S-phase population. In contrast, cells rescued with wt-NEK1 and treated with H2O2 displayed an increase in the G1 population, a reduction of cells in G2/M, and little change in the S fraction. A very different result was seen in cells expressing NEK1-T141A, which showed that most cells accumulated in S, and not in G1. The simplest explanation is that the reduced Chk1 activation in response to H2O2 in these cells, which we attribute to impaired NEK1 function, would lead to a bypass of the G1 checkpoint and rather activation of an intra-S phase checkpoint with the accumulation of DNA damage.
Discussion
The TLKs have been implicated in many diverse functions like DNA repair and the DDR (rev inCitation1), but also aspects of transcription,Citation36,Citation37 possibly mRNA splicing,Citation38 chromatin assembly by Asf1 interaction,Citation15,Citation31 cell motility, and segregation of chromosomes.Citation20,Citation39 However, identification of TLKs direct substrates has been frustratingly slow, and yielded just a few examples (rev inCitation1). With even fewer targets identified using unbiased large screening methodsCitation7,Citation15,Citation40 that were subsequently confirmed by direct interaction. Therefore, we have engineered a new screen to identify the proteomic targets of TLK1 by interrogating the Life Technologies Protoarray composed of 9000 full length human proteins, with purified native TLK1B. This resulted in identification of 165 targets, of which only 3 proteins (out of 3 tested) have been so far independently confirmed with coimmunoprecipitation assays. Notably, Asf1A or Asf1B, which were identified in a previous interaction (yeast 2-hybrid) screen,Citation15 were not obtained in our screen that is based on full length, native proteins. In contrast, Aurora Kinase, which was previously identified in a yeast 2 hybrid screen from a C. elegans cDNA library,Citation40 was further supported as a strong interactor of TLK1 in our screen. Finally, Rad9, a protein which we identified as interacting with TLK1B in a phage display screen, was not present on the ProtoArray.
Because of our long-standing interest in the functions of TLKs in DNA repair and the DDR, we have chosen as our first candidate to investigate more thoroughly, NEK1. This kinase was implicated in the DDR, for interactions with ATR/ATRIP and checkpoint activation,Citation26,Citation28 and more recently found to participate in HR-mediated repair through phosphorylation of Rad54.Citation30 Notably, we previously implicated TLK1 activity in regulation of ATR-mediated DDR Citation8 and in HR-mediated repair.Citation41 This sheds light on the plausible role of TLK1 in regulation of NEK1 activity and seemed a most logical avenue to pursue. Indeed, we found in this work that TLK1/1B interacts with and colocalizes with NEK1 in the nuclei at sites of DNA damage along with γH2AX in cells treated with doxorubicin. Furthermore, the interaction of TLK1 and NEK1 appears to be strengthened with DNA damage, and the kinase activity of NEK1 increases with doxorubicin but can also be suppressed with the TLK inhibitor, THD. We subsequently found that TLK1 phosphorylates NEK1 at T141, which stimulates its kinase activity. The phosphorylation of NEK1 by TLK1 may be cell cycle regulated, although we presently do not have available a phospho-specific antiserum to determine this directly. However, the NEK1 kinase activity is regulated during the cell cycle, and matches the reported cell cycle dependent activity of TLK1Citation21,Citation22 suggesting a possible coordination of these 2 kinases. Notably, expression of the NEK1-T141A that cannot be phosphorylated by TLK1 resulted in a defect in the cell cycle checkpoint in response to treatment with H2O2. In these cells, the G1 checkpoint was largely abrogated, and cells accumulated in S-phase concomitant with the impairment of Chk1 activation. Since the activity of NEK1 has already been implicated in Chk1 activation, as shown by its loss in cells deficient in NEK1,Citation26,Citation28 the simplest explanation for our results is that the TLK1-mediated phosphorylation of NEK1-T141 is a key determinant of NEK1 activation upstream of ATR and Chk1. Recently NEK1 was found to phosphorylate Rad54 at S572, and this activity was shown to promote the release of Rad51 during the completion of HR before entry into mitosis. While the authors demonstrate that Rad54 phosphorylation occurs in G2 and not during S phase arrest, it was not reported how that correlates with the activity of NEK1 under different damage conditions (either presence of DSBs or stalled replication forks, for instance). We have now shown that the activity of NEK1 is cell cycle-regulated and greatly increased in S and G2, which correspond well to the pattern of activity of TLKs.Citation21 We also noticed that another protein identified as interacting strongly with TLK1B is Rad54B, a paralog of Rad54 that performs very similar functions and also binds to Rad51,Citation42 presenting the possibility that TLK1, NEK1, Rad54, Rad54B, and Rad51 may all be present in a complex particularly at sites of DNA damage.
Materials and methods
Hydroxyurea (Catalog No. H8627), Doxorubicin hydrochloride (Catalog No. D1515), Thioridazine hydrochloride (Catalog No. T9025), KU-55933 (Catalog No SML1109) and G418 disulfate salt (Catalog No. A1720) were purchased from Sigma. Dulbecco's modified Eagle's medium (DMEM) was obtained from Life Technologies (Catalog No. 12100–046), Fetal bovine serum (FBS) was obtained from Atlanta Biologicals (Catalog No. 900108).
Antibodies used in this study were: rabbit α-Actin (Ab1801, Abcam), rabbit α-Chk1 phospho-317 (AP3070a, Abgent), rabbit α-Chk1 phospho-345 (sc-17922, Santa Cruz Biotechnology), rabbit α-TLK1 (GTX102891, GeneTex), rabbit αNEK1 Antibody, A304–570A (Bethyl Lab), mouse monoclonal α Nek1 (E-10) (sc-398813 Santa Cruz Biotechnology), mouse monoclonal Anti-polyHistidine antibody (H1029, SIGMA), rabbit αATR antibody (A300–138A, Bethyl Lab), rabbit αpATR (phospho Thr1989) antibody (GTX128145, GeneTex), donkey α-goat IgG-HRP (sc-2020, Santa Cruz Biotechnology), α-rabbit IgG-HRP (7074S, Cell Signaling), α-mouse IgG-HRP (7076, Cell Signaling), mouse α- H2A.X phospho-139 (05–636, Millipore).
Protein-Protein interaction assays (ProtoArray)
This was a service provided by Life Technologies. Briefly, all arrays were blocked with blocking buffer (50 mM HEPES, 200 mM NaCl, 0.08% Triton X-100, 25% glycerol, 20 mM reduced glutathione, 1.0 mM DTT, 1X Synthetic Block) at 4°C for 1 hour in 4-well quadriPERM trays (Greiner) with gentle orbital shaking. Arrays were rinsed once in probe buffer (1X PBS, 0.1% Tween-20, 1X Synthetic Block) before incubation with probe solution. The probe, biotinylated TLK1 protein, was provided by LSUHSC and diluted to concentrations of 5 ng/μl and 50 ng/μl in probe buffer. The negative control array was incubated with probe buffer only. The positive control array was incubated with 50 ng/ml biotinylated V5-epitope tagged yeast calmodulin kinase diluted in probe buffer. Approximately 120 ml of each probe solution was pipetted onto the arrays and incubated under a Lifterslip™ (Thermo Scientific). All arrays were incubated at 4°C for 90 minutes and then washed in probe buffer (5 × 5 ml washes, 5 minutes each). All arrays were incubated with 5 ml of a solution of AlexaFluor®647-conjugated streptavidin (Life Technologies, 1 mg/ml) with gentle shaking in 4-well quadriPERM trays at 4°C for 90 minutes. After incubation, the arrays were washed as described above and quickly rinsed in water to remove residual salts from the surface of the array. Arrays were then immediately placed in a slide holder and spun in a table top centrifuge equipped with a microplate rotor at 1000 RPM for 2 minutes to dry. Arrays were then scanned, as described below.
Data acquisition and analysis
Arrays were scanned using an Axon 4000B fluorescent microarray scanner (Molecular Devices). GenePix 6.0 software was used to overlay the mapping of human proteins in the array list file to each array image using the irregular feature option. After aligning each of the 48 subarrays using spots from the AlexaFluor®-conjugated and murine antibodies printed in each subarray, pixel intensities for each spot on the array were determined from the software and saved to a text file formatted for use in GenePix, the GenePix Result file (.gpr filename extension). These files can be opened in text editing or spreadsheet programs.
Data was processed using Life Technologies’ proprietary ProtoArray® Prospector software. ProtoArray® Prospector includes a series of algorithms specifically designed to analyze data resulting from protein-protein interaction profiling studies, with the goal of identifying specific protein-protein interactors. Significance call queries were performed by analyzing the Prospector output in Microsoft Excel to identify hits on each protein array.
Cell culture
HEK293 cells, obtained from ATCC repository, were maintained in DMEM supplemented with 10% FBS and 1% penicillin-streptomycin at 37°C in a humidified incubator with 5% CO2.
siRNA, plasmids, transfections
Transfections of siRNA were performed using Lipofectamine 3000 (InVitrogen). The 2 siRNAs were made by Dharmaocon: NEK1 siRNA1: gcaaatgtctttaggttga; NEK1 siRNA2: acataagcttca aggacaa. The Nek1 mammalian expression vector was purchased from Origene (MR216282). The entire ORF or N-terminus (aa 1–480) and C-terminus (aa 482–1259) were subcloned in the pET30 bacterial expression vector (Novagen). The NEK1-T141A mutated plasmids were obtained by site-directed mutagenesis using QuickChange (Agilent).
Cell cycle analysis
HEK293 cells were seeded in 6-well plates at a density of 2 × 105 cells/well and treated or not overnight with NEK1 siRNA. The next day cells were treated for 4 h with 0.2 mM H2O2. DU145 cells were seeded in 6 well plates and treated with 2mM Hydroxyurea (Sigma Aldrich H8627) for 8 hours, washed and released in fresh culture media for 6 hours, and then treated with 0.1 µg/ml Nocodazole (Sigma Aldrich M1404). Cells were washed with 1X PBS and trypsinized, washed twice in ice-cold 1X-PBS and fixed in 70% ethanol overnight. Samples were then stained with Propidium Iodine 50 µg/ml (Sigma Aldrich P4170, 3.8mM Sodium Citrate and 0.5 µg/ml RNase A. Samples were then imperiled to FACS Calibur flow cytometer (Becton–Dickinson). Quantitative amounts of cell cycle phase (G1, S, G2, M) were determined by FACSDiva and Modfit modeling software.
Western blot analysis
Cells were lysed in 1X SDS sample buffer. Lysates were sonicated for 15 s and heated at 100ºC for 5 min. Proteins were separated on 6–12% SDS-PAGE gels and transferred to PVDF membranes (Millipore). Membranes were incubated with PBS containing 0.05% Tween 20 and 5% non-fat dry milk to block non-specific binding and were incubated with primary antibodies; membranes were then incubated with appropriate secondary antibodies conjugated to horseradish peroxidase. Immunoreactive bands were visualized using chemiluminescence reagent.
Immunoprecipitation
For co-immunoprecipitation (CoIP) experiment, cells were lysed in the lysis buffer (1% Triton X-100, 0.5% Na-Deoxycholate, 150 mM NaCl, 2.5 mM MgCl2, 1 mM EGTA, 1 mM EDTA, 20 mM Tris/HCl pH 8.0) supplemented with halt EDTA free protease and phosphatase inhibitors. Cell lysates were sonicated for 20 cycles using Diagenode Bioruptor. In total 0.3 mg of cell lysate was precleared with protein A/G Sepharose beads and then incubated overnight at 4°C with the indicated Ab's and then and tumbled with 30 μl of protein A/G Sepharose beads for 4 h at 4°C. After extensive washing in CoIP buffer, proteins were eluted by boiling treatment in 2X electrophoresis sample buffer before Western blotting analysis.
Mass spectrometric analysis of phosphopeptides
The sample preparation for mass spectrometry was described previously (43). Briefly, the peptide samples will be obtained after digestion by Promega trypsin, and then analyzed using LC-MS analysis (nanoAcquity UPLC-Synapt HDMS, waters corporation, MA). Mass spectra will be acquired in positive ion time-of-flight (TOF) V-mode. Data were acquired using MassLynxTM 4.1 software (waters corporation, MA) in an automatic data dependent acquisition mode. MS-TOF scans were acquired from m/z 300 to m/z 1500, and up to 3 precursor ions were selected for subsequent MS/MS scans from m/z 100 to 1500 using charge state recognition promote fragmentation. Mass spectrometry data was processed using PEAKs Studio 7.5 software (Bioinformatics Solutions Inc., Canada).
Kinase assay
For IP'd NEK1, these were as described in.Citation9 For assays with recombinant proteins, typical reactions contained 1 µg of kinases in 20 µl, unless otherwise specified in the figure legend.
Disclosure of potential conflicts of interest
The authors declare that no conflict of interest exists.
KCCY_A_1314421_Supplemental.zip
Download Zip (183 KB)Acknowledgments
We want to thank Dr. Paul Weinberger for helping us with the use of the Mantra Quantitative Pathology workstation and analysis. We thank the Mass Spectrometry Core at LSU Health-Shreveport supported by LSU Health Shreveport Foundation, Center for Cardiovascular Diseases and Sciences, and Feist-Weiller Cancer Center.
Funding
This work was supported by a grant from the Feist Weiller Cancer Center from LSUHSC, Shreveport, and DoD grant PC160398.
References
- De Benedetti A. The Tousled-Like-Kinases as guardians of genome integrity. ISRN Mol Biol 2012:2012:627596; PMID:23869254
- Ciccia A, Elledge SJ. The DNA damage response: making it safe to play with knives. Mol Cell 2010; 40:179–204; PMID:20965415; http://dx.doi.org/10.1016/j.molcel.2010.09.019
- Flynn RL, Zou L. ATR: a master conductor of cellular responses to DNA replication stress. Trends Biochem Sci 2011; 36:133–40; PMID:20947357; http://dx.doi.org/10.1016/j.tibs.2010.09.005
- Cortez D, Guntuku S, Qin J, Elledge SJ. ATR and ATRIP: partners in checkpoint signaling. Science 2001; 294:1713–6; PMID:11721054; http://dx.doi.org/10.1126/science.1065521
- Delacroix S, Wagner JM, Kobayashi M, Yamamoto K, Karnitz LM. The Rad9-Hus1-Rad1 (9-1-1) clamp activates checkpoint signaling via TopBP1. Genes Dev 2007; 21:1472–7; PMID:17575048; http://dx.doi.org/10.1101/gad.1547007
- Zou L, Elledge SJ. Sensing DNA damage through ATRIP recognition of RPA-ssDNA complexes. Science 2003; 300:1542–8; PMID:12791985; http://dx.doi.org/10.1126/science.1083430
- Sunavala-Dossabhoy G, De Benedetti A. Tousled homolog, TLK1, binds and phosphorylates Rad9; tlk1 acts as a molecular chaperone in DNA repair. DNA Repair 2009; 8:87–102; PMID:18940270; http://dx.doi.org/10.1016/j.dnarep.2008.09.005
- Awate S, De Benedetti A. TLK1B mediated phosphorylation of Rad9 regulates its nuclear/cytoplasmic localization and cell cycle checkpoint. BMC Mol Biol 2016; 17:3; PMID:26860083; http://dx.doi.org/10.1186/s12867-016-0056-x
- Chen Y, Chen PL, Chen CF, Jiang X, Riley DJ. Never-in-mitosis related kinase 1 functions in DNA damage response and checkpoint control. Cell Cycle 2008; 7:3194–201; PMID:18843199; http://dx.doi.org/10.4161/cc.7.20.6815
- Chen Y, Chen CF, Riley DJ, Chen PL. Nek1 kinase functions in DNA damage response and checkpoint control through a pathway independent of ATM and ATR. Cell Cycle 2011; 10:655–63; PMID:21301226; http://dx.doi.org/10.4161/cc.10.4.14814
- Meirelles GV, Perez AM, de Souza EE, Basei FL, Papa PF, Melo Hanchuk TD, Cardoso VB, Kobarg J. "Stop Ne(c)king around:" How interactomics contributes to functionally characterize Nek family kinases. World J Biol Chem 2014; 5:141–60; PMID:24921005
- Sunavala-Dossabhoy G, Balakrishnan S, Sen S, Nuthalapaty S, De Benedetti A. The radioresistance kinase TLK1B protects the cells by promoting repair of double strand breaks. BMC Mol Biol 2005; 6:19; PMID:16156902; http://dx.doi.org/10.1186/1471-2199-6-19
- Sunavala-Dossabhoy G, Fowler M, De Benedetti A. Translation of the radioresistance kinase TLK1B is induced by gamma-irradiation through activation of mTOR and phosphorylation of 4E-BP1. BMC Mol Biol 2004; 5:1; PMID:15070431; http://dx.doi.org/10.1186/1471-2199-5-1
- Shalom S, Don J. Tlk, a novel evolutionarily conserved murine serine threonine kinase, encodes multiple testis transcripts. Mol Reprod Dev 1999; 52:392–405; PMID:10092119; http://dx.doi.org/10.1002/(SICI)1098-2795(199904)52:4%3c392::AID-MRD8%3e3.0.CO;2-Y
- Sillje H, Nigg E. Identification of human Asf1 chromatin assembly factors as substrates of Tousled-like kinases. Curr Biol 2001; 11:1068–73; PMID:11470414; http://dx.doi.org/10.1016/S0960-9822(01)00298-6
- Li Y, DeFatta R, Anthony C, Sunavala G, De Benedetti A. A translationally regulated Tousled kinase phosphorylates histone H3 and confers radioresistance when overexpressed. Oncogene 2001; 20:726–38; PMID:11314006; http://dx.doi.org/10.1038/sj.onc.1204147
- Carrera P, Moshkin YM, Gronke S, Sillje HH, Nigg EA, Jackle H, Karch F. Tousled-like kinase functions with the chromatin assembly pathway regulating nuclear divisions. Genes Dev 2003; 17:2578–90; PMID:14561777; http://dx.doi.org/10.1101/gad.276703
- Han Z, Saam JR, Adams HP, Mango SE, Schumacher JM. The C. elegans Tousled-like kinase (TLK-1) has an essential role in transcription. Curr Biol 2003; 13:1921–9; PMID:14614817; http://dx.doi.org/10.1016/j.cub.2003.10.035
- Sunavala-Dossabhoy G, Li Y, Williams B, De Benedetti A. A dominant negative mutant of TLK1 causes chromosome missegregation and aneuploidy in normal breast epithelial cells. BMC Cell Biol 2003; 4:16; PMID:14583098; http://dx.doi.org/10.1186/1471-2121-4-16
- Sunavala-Dossabhoy G, Li Y, Williams B, De Benedetti A. A dominant negative mutant of TLK1 causes chromosome missegregation and aneuploidy in normal breast epithelial cells. BMC Cell Biol 2003; 4:16; PMID:14583098; http://dx.doi.org/10.1186/1471-2121-4-16
- Sillje HH, Takahashi K, Tanaka K, Van Houwe G, Nigg EA. Mammalian homologues of the plant Tousled gene code for cell-cycle-regulated kinases with maximal activities linked to ongoing DNA replication. Embo J 1999; 18:5691–702; PMID:10523312; http://dx.doi.org/10.1093/emboj/18.20.5691
- Ehsan H, Reichheld JP, Durfee T, Roe J. TOUSLED kinase activity oscillates during the cell cycle and interacts with chromatin regulators. Plant Physiol 2004; 134:1488–99; PMID:15047893; http://dx.doi.org/10.1104/pp.103.038117
- Bruinsma W, van den Berg J, Aprelia M, Medema RH. Tousled‐like kinase 2 regulates recovery from a DNA damage‐induced G2 arrest. EMBO Reports 2016; 17:659–70; PMID:26931568; http://dx.doi.org/10.15252/embr.201540767
- Osmani SA, Pu RT, Morris NR. Mitotic induction and maintenance by overexpression of a G2-specific gene that encodes a potential protein kinase. Cell 1988; 53:237–44; PMID:3359487; http://dx.doi.org/10.1016/0092-8674(88)90385-6
- Moniz L, Dutt P, Haider N, Stambolic V. Nek family of kinases in cell cycle, checkpoint control and cancer. Cell Div 2011; 6:18; PMID:22040655; http://dx.doi.org/10.1186/1747-1028-6-18
- Pelegrini AL, Moura DJ, Brenner BL, Ledur PF, Maques GP, Henriques JA, Saffi J, Lenz G. Nek1 silencing slows down DNA repair and blocks DNA damage-induced cell cycle arrest. Mutagenesis 2010; 25:447–54; PMID:20501547; http://dx.doi.org/10.1093/mutage/geq026
- Basei FL, Meirelles GV, Righetto GL, Dos Santos Migueleti DL, Smetana JH, Kobarg J. New interaction partners for Nek4.1 and Nek4.2 isoforms: from the DNA damage response to RNA splicing. Proteome Sci 2015; 13:11; PMID:25798074; http://dx.doi.org/10.1186/s12953-015-0065-6
- Liu S, Ho CK, Ouyang J, Zou L. Nek1 kinase associates with ATR-ATRIP and primes ATR for efficient DNA damage signaling. Proc Natl Acad Sci U S A 2013; 110:2175–80; PMID:23345434; http://dx.doi.org/10.1073/pnas.1217781110
- Chen Y, Chen CF, Chiang HC, Pena M, Polci R, Wei RL, Edwards RA, Hansel DE, Chen PL, Riley DJ. Mutation of NIMA-related kinase 1 (NEK1) leads to chromosome instability. Mol Cancer 2011; 10:5; PMID:21214959; http://dx.doi.org/10.1186/1476-4598-10-5
- Spies J, Waizenegger A, Barton O, Sürder M, Wright WD, Heyer WD, Löbrich M. Nek1 Regulates Rad54 to orchestrate homologous recombination and replication fork stability. Mol Cell 2016; 62:903–17; PMID:27264870; http://dx.doi.org/10.1016/j.molcel.2016.04.032
- De Benedetti A. Tousled kinase TLK1B counteracts the effect of Asf1 in inhibition of histone H3-H4 tetramer formation. BMC Res Notes 2009; 2:128; PMID:19586531; http://dx.doi.org/10.1186/1756-0500-2-128
- Polci R, Peng A, Chen PL, Riley DJ, Chen Y. NIMA-related protein kinase 1 is involved early in the ionizing radiation-induced DNA damage response. Cancer Res 2004; 64:8800–3; PMID:15604234; http://dx.doi.org/10.1158/0008-5472.CAN-04-2243
- Letwin K, Mizzen L, Motro B, Ben-David Y, Bernstein A, Pawson T. A mammalian dual specificity protein kinase, Nek1, is related to the NIMA cell cycle regulator and highly expressed in meiotic germ cells. Embo J 1992; 11:3521–31; PMID:1382974
- Surpili MJ, Delben TM, Kobarg J. Identification of proteins that interact with the central coiled-coil region of the human protein kinase NEK1. Biochemistry 2003; 42:15369–76; PMID:14690447; http://dx.doi.org/10.1021/bi034575v
- Ronald S, Awate S, Rath A, Carroll J, Galiano F, Dwyer D, Kleiner-Hancock H, Mathis JM, Vigod S, De Benedetti A. Phenothiazine inhibitors of TLKs affect double-strand break repair and DNA Damage Response Recovery and potentiate tumor killing with radiomimetic therapy. Genes Cancer 2013; 4:39–53; PMID:23946870; http://dx.doi.org/10.1177/1947601913479020
- Han Z, Saam J, Adams H, Mango S, Schumacher J. The C. elegans Tousled-like kinase (TLK-1) has an essential role in transcription. Curr Biol 2003; 13:1921–9; PMID:14614817; http://dx.doi.org/10.1016/j.cub.2003.10.035
- Wang Y, Liu J, Xia R, Wang J, Shen J, Cao R, Hong X, Zhu JK, Gong Z. The protein kinase TOUSLED is required for maintenance of transcriptional gene silencing in Arabidopsis. EMBO Rep 2007; 8:77–83; PMID:17110953; http://dx.doi.org/10.1038/sj.embor.7400852
- Kodym R, Henockl C, Furweger C. Identification of the human DEAD-box protein p68 as a substrate of Tlk1. Biochem Biophys Res Commun 2005; 333:411–7; PMID:15950181; http://dx.doi.org/10.1016/j.bbrc.2005.05.136
- Hashimoto M, Matsui T, Iwabuchi K, Date T. PKU-beta/TLK1 regulates myosin II activities, and is required for accurate equaled chromosome segregation. Mutat Res 2008; 657:63–7; PMID:18838128; http://dx.doi.org/10.1016/j.mrgentox.2008.09.001
- Han Z, Riefler GM, Saam JR, Mango SE, Schumacher JM. The C. elegans Tousled-like kinase contributes to chromosome segregation as a substrate and regulator of the Aurora B kinase. Curr Biol 2005; 15:894–904; PMID:15916946; http://dx.doi.org/10.1016/j.cub.2005.04.019
- Timiri Shanmugam PS, Nair RP, DeBenedetti A, Caldito G, Abreo F, Sunavala-Dossabhoy G. DNA damage response and repair data with pharmacological modulators of Tousled. Data Brief 2016; 7:1073–7; PMID:27408917; http://dx.doi.org/10.1016/j.dib.2016.03.075
- Tanaka K, Hiramoto T, Fukuda T, Miyagawa K. A novel human rad54 homologue, Rad54B, associates with Rad51. J Biol Chem 2000; 275:26316–21; PMID:10851248; http://dx.doi.org/10.1074/jbc.M910306199
- Jensen ON, Wilm M, Shevchenko A, Mann M. Sample preparation methods for mass spectrometric peptide mapping directly from 2-DE gels. Methods Mol Biol 1999; 112:513–30; PMID:10027274