ABSTRACT
The release of inflammatory cytokines has been implicated in the toxicity of conventional radiotherapy (CRT). Transforming growth factor β (TGF-β) has been suggested to be a risk marker for pulmonary toxicity following radiotherapy. Pulsed low-dose rate radiotherapy (PLDR) is a technique that involves spreading out a conventional radiotherapy dose into short pulses of dose with breaks in between to reduce toxicities. We hypothesized that the more tolerable toxicity profile of PLDR compared with CRT may be related to differential expression of inflammatory cytokines such as TGF-β in normal tissues. To address this, we analyzed tissues from mice that had been subjected to lethal doses of CRT and PLDR by histology and immunohistochemistry (IHC). Equivalent physical doses of CRT triggered more cellular atrophy in the bone marrow, intestine, and pancreas when compared with PLDR as indicated by hematoxylin and eosin staining. IHC data indicates that TGF-β expression is increased in the bone marrow, intestine, and lungs of mice subjected to CRT as compared with tissues from mice subjected to PLDR. Our in vivo data suggest that differential expression of inflammatory cytokines such as TGF-β may play a role in the more favorable normal tissue late response following treatment with PLDR.
Introduction
Pulsed low-dose-rate (PLDR) radiotherapy is a novel technique that has been used clinically for re-irradiation in settings where recurrent radiation is expected to be unacceptably toxic.Citation1-Citation4 This involves spreading out a conventional radiation therapy dose into short pulses of dose with breaks in between. By example, a PLDR regimen may involve delivering a dose of 2 Gy as 10 pulses of 0.2 Gy, each 3 minutes apart. The technique is based on 3 observations: low dose hyper-radiosensitivity, evidence of a transition dose beyond which DNA damage repair is triggered, and the observation of improved DNA damage repair for normal tissue at low dose rates.Citation5-Citation10 The theory is that tumor cells have a higher dose threshold to initiate DNA repair than normal tissues.Citation11,Citation12 PLDR theoretically delivers short pulses of low dose that lies between the repair thresholds of tumor cells and normal tissues, to trigger repair in normal tissues only. Therefore, PLDR takes advantage of both tumor hyper-radiosensitivity at low doses and improved normal tissue repair at low-dose rates.
PLDR has been performed successfully in multiple patient populations, including women with chest wall recurrences of breast cancer and patients with recurrent gliomas in the brain,Citation1,Citation2 and with advanced treatment techniques such as volumetric modulated arc therapy (VMAT) and intensity modulated radiation therapy (IMRT).Citation3,Citation4 While cell survival data from cell lines and in vivo animal experiments have been published, no mechanistic data are available to explain the phenomenon of improved clinical toxicity with this treatment technique. Therefore, we performed in vivo experiments in mice to investigate the effect of PLDR and conventional radiotherapy (CRT) on normal tissues. In particular, we were interested in the expression of TGF-β in irradiated normal tissues of mice.
Results and discussion
In accordance with what has been previously published we observed histopathological signs of reduced toxicity in mice following PLDR when compared with equivalent doses of CRTCitation5 (). One striking difference observed was the reduction in atrophy in the bone marrow ( and ) and cecum ( and ), 2 organs known to be highly sensitive to the acute toxic effects of radiotherapy both in experimental animal models and in patient subjected to radiotherapy.Citation13-Citation15 One mouse also developed pancreatitis. However, it is unclear to what extent this is genuine radiation toxicity since radiation-induced damage to the pancreas has rarely been documented.
Figure 1. Pulsed Low Dose Rate Radiotherapy (PLDR) is associated with reduced levels of bone marrow and intestinal atrophy at equivalent doses of Conventional Radiotherapy (CRT). H/E staining shows cellular atrophy in the bone marrow (BM) (A) and the cecum (B) following treatment with CRT and PLDR. Morphometric quantitation using the NIH Image J software of atrophic areas in the BM, pancreas and intestine is shown (C). The arithmetic mean of a minimum of 5 analyzed tissue areas from 1–2 mice is shown. Error bars represent the standard error. BM, bone marrow.
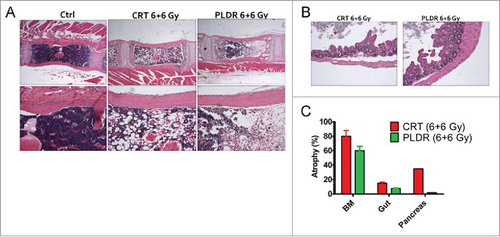
To address the molecular changes that potentially could drive the differential toxic response, we subjected the isolated tissues to immunohistochemical (IHC) staining using the well-known radiotherapy marker TGF-β16 (). The IHC data indicated that the expression of TGF-β is significantly higher in tissues following CRT than in those following PLDR (bone marrow in , small intestine in , and lung in ), although some tissues showed differential acute tissue responses to the different radiotherapy protocols. Of note, the dose of thoracic radiotherapy is often limited by the risk of pneumonitis and pulmonary fibrosis that can develop several months post radiation.Citation17-Citation19 Although it is not clear how much the limitations of lung dose affect efficacy, particularly in the TBI/SCT population from which this data are largely derived, use of PLDR may impact on therapeutic index in the clinic.
Figure 2. TGF-β is expressed at higher levels in tissues in vivo following CRT treatment. Tissues were subjected to immunohistochemical analysis for TGF-β and increased expression of the cytokine was observed in the bone marrow (A) at doses of 18 Gy, the intestine at doses of 8 Gy (B) and in lung at doses of 12 Gy (C). The areas in the tissues showing increased expression of TGF-β were quantitated using the NIH Image J software (E). The arithmetic mean of a minimum of 5 analyzed tissue areas from 1–2 mice is shown. Error bars represent the standard error. BM, bone marrow; SI, small intestine.
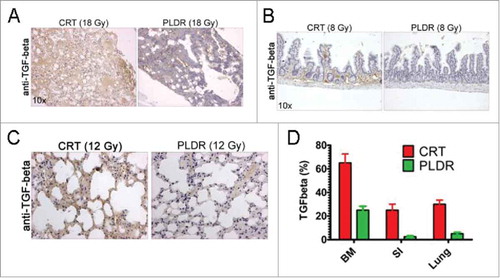
The striking decreases in atrophy in murine bone marrow, small intestine and pancreas after PLDR are consistent, possibly, with the result of the decreased TGF-β staining in bone marrow, small intestine and lung when compared with conventional radiation. TGF-β is activated after radiotherapy and has been implicated in the conversion of fibroblasts into myofibroblasts responsible for extracellular matrix deposition, playing a critical role in radiation-induced lung fibrosis. In preclinical experiments, TGF-β inhibitors have been shown to protect mice from pulmonary fibrosis following 20 Gy of ionizing radiation delivered to the thorax.Citation20 Consequently, our findings may suggest how mechanistically PLDR is associated with a reduced incidence of pulmonary side-effects following chest radiation.
These in vivo data reinforce the clinical point that PLDR radiation is qualitatively different from conventional radiotherapy in terms of normal tissue injury. These data suggest various possible applications of PLDR, from expanded use in the setting of prior radiation to higher tolerable doses in patients with newly diagnosed cancer. We are currently opening a phase I study of chemoradiation using PLDR for patients with newly diagnosed locally advanced non-small cell lung cancer and esophageal cancer, to assess whether PLDR will decrease the risk of acute esophagitis, commonly seen in this setting.
Material and methods
Mice and treatment
Male balb/c mice were subjected to the same total physical doses of whole-body radiation using 2 different radiation delivery protocolsCitation5 according to an approved Fox Chase Cancer Center and Temple Health Institutional Animal Care and Use Committee protocol. Conventional radiotherapy (CRT) was delivered as a single dose of 8 Gy whereas PLDR involved delivery in 40 fractions of 0.2 Gy separated by 3 minutes. The mice were irradiated using a Siemens Artiste linear accelerator with 6 MV photon beams (Radiation Oncology Department, Fox Chase Cancer Center). The mice were killed by CO2 inhalation at 14-days following radiation treatment and their brain, heart, lungs, liver, spleen, gastrointestinal, urinary and reproductive organs, and sternal bone marrow were removed, fixed in formalin, processed and embedded in paraffin for histological and immunohistochemical evaluation. Tissue sections were analyzed for histopathological changes using hematoxylin and eosin (H/E). H/E stained tissues were subjected to morphometric evaluation using the NIH Image J software when histopathological abnormalities were detected in the tissues.
Immunohistochemistry
Slide sections were rehydrated and subjected to antigen retrieval in citric acid buffer (1.0 mM, pH 6.0). Endogenous peroxidases were blocked with 10% hydrogen peroxide. The slides were blocked for 30 minutes in 5% goat serum and subsequently incubated with the primary rabbit antibodies toward TGF-β1 (C-16, 1:200, Santa Cruz) at room temperature overnight. Slides were then washed and incubated with secondary peroxidase conjugated antibodies using the ImmPRESS™ HRP Anti-Rabbit IgG (Peroxidase) Polymer Detection Kit (Vector Laboratories). Following washing in PBS the slides were stained using the 3,3′-diaminobenzidine tetrahydrochloride (DAB) Peroxidase (HRP) Substrate Kit, 3,3′-diaminobenzidine (Vector Laboratories). The slides were counterstained using hematoxylin. TGFβ stained slides were evaluated blindly and the area % positive for TGF-β stained determined using the NIH Image software.
Abbreviations
BM | = | bone marrow |
CRT | = | conventional radiotherapy |
DAB | = | 3,3′-diaminobenzidine tetrahydrochloride |
H/E | = | hematoxylin and eosin |
HRP | = | horse-radish peroxidase |
IHC | = | immunohistochemistry |
PLDR | = | pulsed low-dose rate radiation |
SI | = | small intestine |
TGF-β | = | transforming growth factor β. |
Disclosure of potential conflicts of interest
No potential conflicts of interest were disclosed.
Funding
This work was supported by a grant from the American Cancer Society to W.S.E-D. W.S.E-D. is an American Cancer Society Research Professor.
References
- Richards GM, Tome WA, Robins HI, Stewart JA, Welsh JS, Mahler PA, Howard SP. Pulsed reduced dose-rate radiotherapy: a novel locoregional retreatment strategy for breast cancer recurrence in the previously irradiated chest wall, axilla, or supraclavicular region. Breast Cancer Res Treat 2009; 114:307-13; PMID:18389365; https://doi.org/10.1007/s10549-008-9995-3
- Adkison JB, Tome W, Seo S, Richards GM, Robins HI, Rassmussen K, Welsh JS, Mahler PA, Howard SP. Reirradiation of large-volume recurrent glioma with pulsed reduced-dose-rate radiotherapy. Int J Radiat Oncol Biol Phys 2011; 79:835-41; PMID:20472350; https://doi.org/10.1016/j.ijrobp.2009.11.058
- Ma CM, Lin MH, Dai XF, Koren S, Klayton T, Wang L, Li JS, Chen L, Price RA. Investigation of pulsed low dose rate radiotherapy using dynamic arc delivery techniques. Phys Med Biol 2012; 57:4613-26; PMID:22750648; https://doi.org/10.1088/0031-9155/57/14/4613
- Lin MH, Price RA, Jr, Li J, Kang S, Li J, Ma CM. Investigation of pulsed IMRT and VMAT for re-irradiation treatments: dosimetric and delivery feasibilities. Phys Med Biol 2013; 58:8179-96; PMID:24200917; https://doi.org/10.1088/0031-9155/58/22/8179
- Zhang P, Wang B, Chen X, Cvetkovic D, Chen L, Lang J, Ma CM. Local tumor control and normal tissue toxicity of pulsed low-dose rate radiotherapy for recurrent lung cancer: an in vivo animal study. Dose Response 2015; 13:1559325815588507; PMID:26675811
- Joiner MC, Marples B, Lambin P, Short SC, Turesson I. Low-dose hypersensitivity: current status and possible mechanisms. Int J Radiat Oncol Biol Phys 2001; 49:379-89; PMID:11173131; https://doi.org/10.1016/S0360-3016(00)01471-1
- Marples B, Collis SJ. Low-dose hyper-radiosensitivity: past, present, and future. Int J Radiat Oncol Biol Phys 2008; 70:1310-8; PMID:18374221; https://doi.org/10.1016/j.ijrobp.2007.11.071
- Bedford JS, Mitchell JB. Dose-rate effects in synchronous mammalian cells in culture. Radiat Res 1973; 54:316-27; PMID:4735769; https://doi.org/10.2307/3573709
- Yeoh EK, Krol R, Dhillon VS, Botten R, Di Matteo A, Butters J, Brock AR, Esterman A, Salisbury C, Fenech M. Predictors of radiation-induced gastrointestinal morbidity: A prospective, longitudinal study following radiotherapy for carcinoma of the prostate. Acta Oncologica 2016; 55:604-10; PMID:27046049; https://doi.org/10.3109/0284186X.2015.1118658
- Lin PS, Wu A. Not all 2 Gray radiation prescriptions are equivalent: Cytotoxic effect depends on delivery sequences of partial fractionated doses. Int J Radiat Oncol Biol Phys 2005; 63:536-44; PMID:16168846; https://doi.org/10.1016/j.ijrobp.2005.06.010
- Tome WA, Howard SP. On the possible increase in local tumour control probability for gliomas exhibiting low dose hyper-radiosensitivity using a pulsed schedule. Br J Radiol 2007; 80:32-7; PMID:16945935; https://doi.org/10.1259/bjr/15764945
- Steel GG. The ESTRO Breur lecture. Cellular sensitivity to low dose-rate irradiation focuses the problem of tumour radioresistance. Radiother Oncol 1991; 20:71-83
- Finnberg N, Gruber JJ, Fei P, Rudolph D, Bric A, Kim SH, Burns TF, Ajuha H, Page R, Wu GS, et al. DR5 knockout mice are compromised in radiation-induced apoptosis. Mol Cell Biol 2005; 25:2000-13; PMID:15713653; https://doi.org/10.1128/MCB.25.5.2000-2013.2005
- Fleenor CJ, Higa K, Weil MM, DeGregori J. Evolved cellular mechanisms to respond to genotoxic insults: implications for radiation-induced hematologic malignancies. Radiat Res 2015; 184:341-51; PMID:26414506; https://doi.org/10.1667/RR14147.1
- Fei P, Bernhard EJ, El-Deiry WS. Tissue-specific induction of p53 targets in vivo. Cancer Res 2002; 62:7316-27; PMID:12499275
- Okunieff P, Chen Y, Maguire DJ, Huser AK. Molecular markers of radiation-related normal tissue toxicity. Cancer Metastasis Rev 2008; 27:363-74; PMID:18506399; https://doi.org/10.1007/s10555-008-9138-7
- Kong FM, Zhao L, Hayman JA. The role of radiation therapy in thoracic tumors. Hematol Oncol Clin North Am 2006; 20:363-400; PMID:16730299; https://doi.org/10.1016/j.hoc.2006.01.021
- Roeder F, Friedrich J, Timke C, Kappes J, Huber P, Krempien R, Debus J, Bischof M. Correlation of patient-related factors and dose-volume histogram parameters with the onset of radiation pneumonitis in patients with small cell lung cancer. Strahlenther Onkol 2010; 186:149-56; PMID:20165822; https://doi.org/10.1007/s00066-010-2018-4
- Finnberg N, Klein-Szanto AJ, El-Deiry WS. TRAIL-R deficiency in mice promotes susceptibility to chronic inflammation and tumorigenesis. J Clin Invest 2008; 118:111-23; PMID:18079962; https://doi.org/10.1172/JCI29900
- Dadrich M, Nicolay NH, Flechsig P, Bickelhaupt S, Hoeltgen L, Roeder F, Hauser K, Tietz A, Jenne J, Lopez R, et al. Combined inhibition of TGFbeta and PDGF signaling attenuates radiation-induced pulmonary fibrosis. Oncoimmunology 2016; 5:e1123366; PMID:27467922; https://doi.org/10.1080/2162402X.2015.1123366