ABSTRACT
Histone deacetylases (HDACs) have been implicated in diverse biologic processes including transcriptional regulation, signal transduction, and developmental control. However, the role of HDAC6 in mammalian oocytes remains unknown. In the present study, by using Tubastatin A (TubA), a selective HDAC6 inhibitor, we examined the effects of HDAC6 on maturational progression and meiotic apparatus in mouse oocytes. We found that HDAC6 inhibition results in maturation arrest and disruption of spindle morphology and chromosome alignment. In line with this observation, confocal microscopy revealed that kinetochore-microtubule attachment, a critical mechanism controlling chromosome movement, is compromised in TubA-treated oocytes markedly. Moreover, we noted that HDAC6 inhibition significantly increases the acetylation levels of α-tubulin in mouse oocytes, which may be associated with the defective phenotypes of TubA-treated oocytes by altering microtubule stability and dynamics. In sum, we discover a novel function of HDAC6 during oocyte maturation and suggest a potential pathway modulating meiotic apparatus assembly.
Introduction
During meiotic maturation, spindle assembly and chromosome alignment need to be accurately controlled to produce a healthy oocyte. Any errors in this process can lead to a high aneuploidy incidence,Citation1 which is a main cause of spontaneous abortion or birth defects in humans.Citation2 Although many molecules and pathways have been found to lead to defects in oocyte meiosis, the mechanisms for regulating meiotic apparatus remain to be discovered.
Epigenetic regulation of gene expression is a dynamic and reversible process that does not involve DNA sequence changes but can be inherited, closely related to cell proliferation, differentiation and apoptosis.Citation3 The covalent modification of histones modulates the state of chromatin, which in turn affects the expression of genes and thus plays an important role in epigenetic regulation. Histone acetylation is catalyzed by histone acetyltransferases (HATs), whereas the reverse reaction is performed by histone deacetylases (HDACs).Citation4,5 To date, there are 18 mammalian HDAC enzymes divided into 4 classes: the class I yeast gene Rpd3-like proteins (HDAC1, 2, 3, and 8); the class II is subdivided into classes IIa (HDAC4, 5, 7, and 9) and IIb (HDAC6 and 10); the class III includes Sirtuin family of enzymes (SIRT1∼7); and the class IV protein (HDAC11).Citation6,7 HDACs have been widely reported to function in gene transcription, signal transduction, and other physiologic and pathological processes.Citation3-6,8 For example, HDAC1 is involved in histone acetylation and gene expression during mouse preimplantation embryo development. HDAC2 regulates chromosome separation and kinetochore function by deacetylation of H4K16 during oocyte maturation.Citation9 HDAC4 is essential for stabilizing the genomes of fully grown oocytes.Citation10 Nonetheless, to date, not much is known about the function of other HDACs in mammalian oocytes.
HDAC6 is a unique cytoplasmic deacetylase controlling various biologic processes by deacetylation of vast non-histone substrates.Citation11 Recently, HDAC6 was found to be present in the cytoplasm of non-muscle cells, which have been implicated in regulating cell migration and microtubule dynamics.Citation7 André Verdel et al. found that overexpression of HDAC6 in germinal vesicles and pronuclei would alter the nuclear structure and cause premature compaction of the chromatin.Citation12 Inhibition of HDAC6 leads to morphological abnormalities in rat brain oligodendrocytes potentially by changing tubulin acetylation.Citation13 Moreover, tubulin acetylation has been shown to modulate the ability of microtubules to bind to microtubule-associated proteins (MAPs) and motor proteins, and thus may regulate MT stability and function.Citation14,15 Recent studies also showed that tubulin acetylation restricts the number of protofilaments in nematode touch receptor neurons, suggesting that tubulin acetylation is involved in the organization of microtubules.Citation16 In this study, by using Tubastatin A (TubA, a widely used specific inhibitor for HDAC6), we found that HDAC6 inhibition adversely affects the maturational progression, spindle organization, and chromosome alignment during mouse oocyte meiosis.
Results
HDAC6 localization in oocyte meiosis
We examined the subcellular localization of HDAC6 from GV (Germinal Vesicle) to MII (Metaphase II) stage using immunofluorescence microscopy. As shown in , HDAC6 predominantly accumulated in the nucleus at GV stage. Shortly after GVBD (Germinal Vesicle Breakdown), HDAC6 resided in the entire oocytes, and many of them appeared to concentrate around the chromosomes (arrowheads). Strikingly, HDAC6 showed a spindle-like localization pattern in metaphase oocytes. These results indicate that HDAC6 may be a micortubule-associated protein in mouse oocytes. This distribution pattern prompted us to explore the potential roles of HDAC6 during oocyte maturation.
Figure 1. Cellular localization of HDAC6 in mouse oocytes. Oocytes at GV, Pre-Metaphase I, and Metaphase II stages were immunolabeled with HDAC6 antibody (green) and counterstained with propidium iodide for DNA (red), respectively. Representative images were acquired under the confocal microscope. HDAC6 signals are indicated by arrowheads. Scale bar, 25 μm.
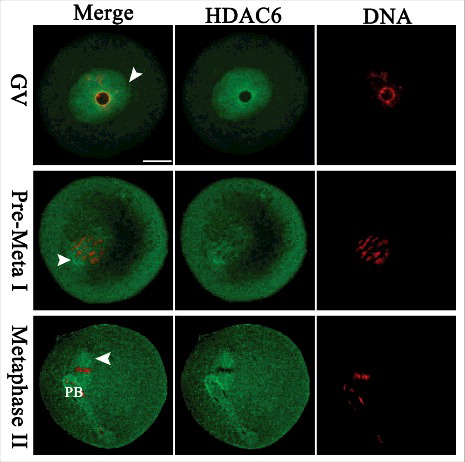
HDAC6 inhibition affects maturational progression in mouse oocytes
To investigate the function of HDAC6 in oocyte meiosis, GV oocytes were cultured in maturation medium containing different doses of TubA. The rates of GVBD rate and first polar body (pb1) extrusion were analyzed as illustrated in . At 3 hours, the GVBD rate was significantly decreased in 1.0 μg/ml and 10.0 μg/ml TubA group compared with control group (), indicating the block of meiotic resumption. Similarly, after 14 hours culture, the pb1 extrusion rates in 1.0 μg/ml and 10 μg/ml TubA group were much lower than control group (), indicating that TubA-treated oocytes failed to complete meiosis I and form the first polar body (, blue arrows). Besides, the symmetric division in some oocytes was frequently observed (, red arrowheads). 1.0 μg/ml of TubA was therefore selected as the final working concentration to suppress HDAC6 in the following experiments. Together, these results suggest that HDAC6 inhibition adversely affects oocyte maturation and meiotic divisions.
Figure 2. Effects of HDAC6 inhibition on oocyte maturation. Different concentrations of TubA were used to examine the effects of HDAC6 inhibition on mouse oocyte maturation. Fully grown oocytes treated with TubA were cultured for 14 hours to evaluate the rate of GVBD and Pb1 extrusion. (A) Phase-contrast images of control and TubA-treated (1.0 μg/ml) oocytes. Blue arrows indicate the oocytes that fail to extrude polar bodies; Red arrowheads indicate the oocytes with symmetric division; Asterisks indicate the oocytes with normal division. Scale bar, 100 μm. (B, C) Quantitative analysis of GVBD rate and Pb1 extrusion rate in control (n = 118) and TubA-treated (n = 120) oocytes. The graph shows the mean ± SD of the results obtained in 3 independent experiments. *P<0.05; **P < 0.01; ***P < 0.001 vs. control.
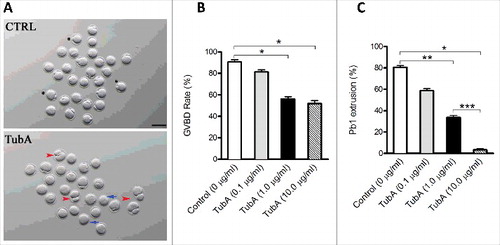
HDAC6 inhibition disrupts spindle organization and chromosome alignment in meiotic oocytes
HDAC6 distribution and its effects on maturational progression prompted us to ask whether HDAC6 inhibition affects the meiotic apparatus in oocytes. To test this, oocytes were immunostained with anti-α-tubulin antibody to show spindle morphology and counterstained with propidium iodide (PI) to observe chromosomes. Confocal microscopy revealed that control metaphase oocytes usually present a typical barrel-shape spindle and well-aligned chromosomes at the equator (). In sharp contrast, we found a variety of disorganized spindle (, arrow) and misaligned chromosomes (, arrowheads) in TubA-treated oocytes. Quantification data further confirmed that the percentage of TubA-treated oocytes with defective spindle/chromosomes was significantly higher than control cells (). Taken together, these results suggest that HDAC6 plays a considerable role in chromosome movement and spindle assembly in meiosis.
Figure 3. Effects of HDAC6 inhibition on spindle assembly and chromosome organization in oocytes. (A) Control and TubA-treated oocytes were immunostained with anti-α-tubulin antibody to visualize the spindle (green) and counterstained with PtdIns to visualize chromosomes (red). Representative confocal sections are shown. Control metaphase oocytes (a) present a typical barrel-shape spindle and well-organized chromosomes on the metaphase plate; (b, c) TubA-treated oocytes frequently show abnormal spindles (arrow) and chromosome misalignment (arrowheads). Scale bar, 25 μm. (B) Quantification of control (n = 120) and TubA-treated (n = 126) oocytes with spindle/chromosome defects. Data are expressed as mean percentage ± SD from 3 independent experiments. *p< 0.05 vs. controls.
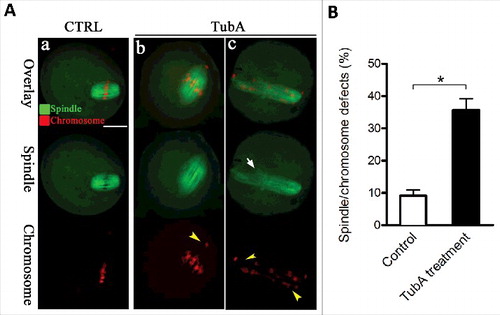
HDAC6 inhibition induces erroneous kinetochore-microtubule attachments in oocytes
The attachment between spindle microtubules and kinetochores provides the major force that drives chromosome segregation and, therefore, must be established correctly.Citation17 Next, we asked whether kinetochore-microtubule (K-MT) attachments were impaired after HDAC6 inhibition. To examine the interaction between kinetochores and microtubules, metaphase I oocytes were immunolabeled with CREST to track kinetochores (purple), anti-tubulin antibody to detect microtubules (green), and Hoechst 33342 for chromosomes (blue) (). Remarkably, quantitative analysis revealed that the frequency of K-MT misattachments was significantly increased in TubA-treated oocytes when compared with control oocytes (). By confocal scanning, we found that most normal oocytes presented the classic amphitelic attachments, in which a pair of kinetochores are connected to the opposite spindle poles, respectively, (, white arrows). Whereas attachment loss (kinetochores unattached to either pole) and merotelic attachment (one kinetochore attached to both poles) were readily observed in TubA-treated oocytes (, yellow arrowheads). Collectively, these data imply that inhibition of HDAC6 weaken the pulling forces across K-MT attachments, which could result in the spindle defects and chromosome misalignment.
Figure 4. Effects of HDAC6 inhibition on kinetochore-microtubule attachments. (A) Representative images of K-MT attachments in control and TubA-treated oocytes. Oocytes at MI stage were immunolabeled with anti-tubulin antibody to visualize spindles (green), with CREST to detect kinetochores (purple), and counterstained with Hoechst 33342 to show chromosomes (blue). Scale bar, 10 μm. Representative confocal sections showed the amphitelic attachment in control oocytes (white arrows), and lost/merotelic attachments (yellow arrowheads) in TubA-treated oocytes. (B) Quantitative analysis of the proportion of defective K-MT attachments in control (n = 15) and TubA-treated (n = 16) oocytes. Data were presented as mean percentage ± SD from 3 independent experiments. *p< 0.05 vs. controls.
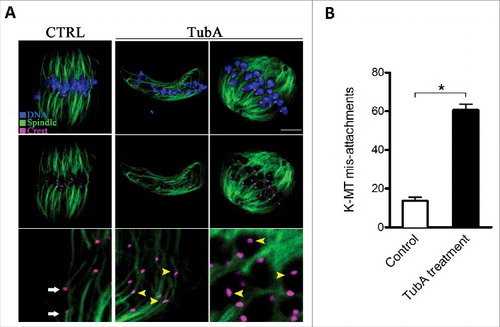
HDAC6 inhibition increases the acetylation levels of α-tubulin in mouse oocytes
In addition to histones, HDAC6 interacts with a variety of non-histones such as tubulin, dynein, Bax, and GRK2 in both nucleus and cytoplasm.Citation4 Tubulin was shown to be subjected to acetylation, which occurs on lysine 40 of the α-tubulin subunit.Citation18,19 HDAC6 containing tandem catalytic domains and an ubiquitin-binding zinc finger displays deacetylase activity toward acetylated microtubules.Citation20 Considering the role of HDAC6 in meiotic apparatus assembly of mouse oocytes, we decided to explore whether it affects the acetylation status of tubulin. As shown in , we found that the acetylation levels of α-tubulin were dramatically increased in TubA-treated oocytes in comparison with controls (). Notably, α-tubulin acetylation serves as a marker for the presence of stable microtubules, may influence the activity of microtubule-associated proteins and microtubule-based motors. Collectively, HDAC6 inhibition results in α-tubulin hyperacetylation in mouse oocytes, which may consequently lead to spindle disorganization and chromosome misalignment through impacting microtubule stabilization.
Figure 5. Effects of HDAC6 inhibition on the acetylation state of tubulin in mouse oocytes. (A) Representative confocal sections showing the acetylated tubulin (green) and chromosomes (red) in control and TubA-treated oocytes. Scale bar, 30 μm. (B) Quantitative analysis of the fluorescence intensity of acetylated α-tubulin in control (n = 36) and TubA-treated (n = 38) oocytes. Data were presented as mean percentage ± SD from 3 independent experiments. *p < 0.05 vs. controls.
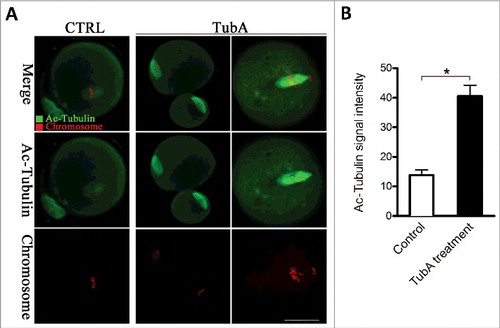
Discussion
HDAC6 gene is localized to the X chromosome p11.22 ∼23 region and encoded by 28 exons.Citation21 HDAC6, a class IIb member, with 1215 amino acid residues, largest in the HDAC family, contains tandem catalytic domains and an ubiquitin-binding zinc finger.Citation20 However, to date, little was known about the localization and function of HDAC6 in mammalian oocytes. In this study, we showed that HDAC6 was predominantly distributed in the nucleus at GV stage, accumulated around chromosomes after meiotic resumption, and then showed a spindle-like pattern in metaphase oocytes (). These findings indicate that HDAC6 may be involved in the microtubule-related cellular events. Accurate alignment and segregation of chromosomes is a crucial event in meiosis.Citation22 In support of this, HDAC6 inhibition in oocyte disrupted maturational progression, spindle formation, and chromosome movement as well (). These remarkable phenotypes prompted us to search for the underlying pathways. Chromosome movement depends on the bioriented interaction of chromosome to spindle through the end-on attachment of microtubules to kinetochores. Kinetochore is a protein complex of nearly 100 proteins that connects centromere DNA with spindle microtubules and thereby couples with the forces generated by microtubule dynamics to provide power for chromosomal motions.Citation23,24 Defects in chromosome alignment may therefore arise from the microtubule misattachment to kinetochores. In line with this notion, we found that HDAC6 inhibition apparently impaired the K-MT interactions in meiotic oocytes (). Taking together, these data suggest that HDAC6 inhibition leads to reduction in the K-MT stability and the pulling forces across kinetochores, which may contribute to the spindle disorganization and chromosome alignment failure we observed here.
One of the important substrates of HDAC6 is α-tubulin, which forms dimers with β-tubulin in microtubules. HDAC6 has been shown to be able to deacetylate lysine 40 of α-tubulin and regulate microtubule-dependent cell motility.Citation25 Zilberman et al. reported that inhibition of HDAC6 catalytic activity might affect microtubule dynamics by promoting the interaction of HDAC6 with tubulin and/or with other microtubule regulatory proteins.Citation26 In the present study, we observed a drastic increase in the acetylation levels of α-tubulin in TubA-treated oocytes (), suggesting that HDAC6 in mouse oocytes indeed controls the tubulin acetylation state. Tubulin subunits are subjected to various posttranslational modifications, including tyrosination, phosphorylation, polyglycylation and acetylation.Citation27 Notably, tubulin acetylation was demonstrated to influence the ability of microtubules to bind microtubule-associated proteins and motor proteins and, consequently, may regulate microtubule stability and function.Citation16,28,29 For example, recent studies showed that tubulin acetylation restricts the number of protofilaments in nematode touch receptor neurons, indicating that tubulin acetylation participates in microtubule organization.Citation15 Thus, hyperacetylation of microtubule may compromise kinetochore function, generating the defective meiotic apparatus. It is worth noting that the stability of kinetochore-microtubule is reduced, and pulling forces across kinetochores are apparently defective in oocytes when HDAC6 activity was inhibited (). Cumulatively, a plausible hypothesis is that hyperacetylation of α-tubulin induced by HDAC6 inhibition impairs microtubule stability and K-MT attachment, consequently resulting in oocyte meiotic spindle defects and chromosome misalignment. Acetylated microtubules have been detected in mouse oocytes;Citation30 however, their roles in meiosis are not clear. Our ongoing research is trying to clarify this issue.
In summary, proper assembly of meiotic apparatus is vital for producing a good quality oocyte. Our data indicate HDAC6 as a cytoskeletal modulator functions during this process, providing a novel mechanism controlling oocyte maturation. Any errors during meiotic division could result in pregnancy loss and infertility, our findings are therefore essential for preventing birth defects and reproductive disease.
Materials and methods
All chemicals and reagents were purchased from Sigma (St. Louis, MO, USA) unless stated otherwise. ICR mice were used in this study. All experiments were approved by the Animal Care and Use Committee of Nanjing Medical University and were performed in accordance with institutional guidelines.
Antibodies
Rabbit polyclonal anti-HDAC6 antibody (Cat#: BS1652) was purchased from Bioworld. Mouse monoclonal FITC-conjugated α-tubulin antibody (Cat#: T6074) was purchased from Sigma. Mouse monoclonal anti-acetylated tubulin antibody (Cat#: T7451) was obtained from Sigma. Human anti-centromere CREST antibody (Cat#: 15-234) was purchased from Antibodies Inc. (Davis, CA, USA). Cy5-conjugated donkey anti-human IgG (Cat#: 709–605–149) was purchased from Jackson ImmunoResearch Laboratory (West Grove, PA, USA). FITC-conjugated goat anti-rabbit IgG was purchased from Termo Fisher Scientifc (Rockford, IL, USA).
Oocyte collection and culture
Three-week old female ICR mice were killed by cervical dislocation for oocyte collection. Cumulus-enclosed oocytes were retrieved by manual rupturing of antral ovarian follicles. Fully-grown denuded oocytes were collected by removing cumulus cells with repeated mouth-pipetting. GV oocytes were in vitro matured in M16 medium under mineral oil at 37oC in a 5% CO2 incubator.
Tubastatin A treatment
TubA (Cat#: S8049, Selleckchem) solution prepared in dimethyl sulfoxide (DMSO) was diluted to yield a final concentration of 0.1 μg/ml, 1 μg/ml and 10 μg/ml in culture medium, respectively. To explore the role of HDAC6 in meiotic maturation, fully-grown oocytes were cultured in M16 medium containing different doses of TubA for 14 hours. Correspondingly, 0.1% DMSO was included as a control.
Immunofluorescence and confocal microscopy
Oocytes were fixed in freshly prepared 4% paraformaldehyde in PBS (PH 7.4) for 30 minutes, and then treated with 0.5% Triton X-100 for 20 minutes. Following blocking in 1% BSA in PBS for 1 h at room temperature, the processed samples were incubated overnight at 4°C with primary antibodies: anti-HDAC6 antibody (1:100), FITC-conjugated α-tubulin antibody (1:200) or anti-acetyl-tubulin antibody (1:200). After 3 washes for 5 minutes each, the oocytes were labeled with secondary FITC-conjugated antibody for 1 hour at room temperature. Chromosomes were evaluated by staining with propidium iodide (red) for 10 min. After washes in PBS, oocytes were mounted on anti-fade medium (Vectashield, Burlingame, CA, USA). All samples were examined under a laser scanning confocal microscope (LSM 700; Zeiss, Oberkochen, Germany).
To detect kinetochores, oocytes were immunolabeled with CREST (1:500) as described previously.Citation31 After 3 washes for 5 minutes each, the oocytes were labeled with secondary FITC-or Cy5-conjugated antibody for 1 hour at room temperature. Nuclear status was stained with Hoechst 33342 (blue) for 10 min. After multiple washes in PBS, oocytes were mounted on glass slides in a drop of antifade medium (Vectashield, Burlingame, CA, USA). To assess the kinetochore-microtubule (K-MT) attachments, oocytes were briefly chilled at 4°C to induce depolymerization of non-kinetochore microtubules just before fixation. To quantify the intensity of fluorescence, Image J software (NIH) was used as described previously.Citation32
Statistical analysis
Data are presented as means ± SD, unless otherwise stated. Statistical comparisons were made with Student's t test and ANOVA when appropriate. Values of P < 0.05 were considered to be significant.
Disclosure of potential conflicts of interest
No potential conflicts of interest were disclosed.
Competing financial interests
The authors declare no competing financial interests.
Additional information
Funding
References
- Hassold T, Hunt P. To err(meiotically) is human: The genesis of human aneuploidy. Nat Rev Genet 2001; 2:280–91; PMID:11283700; https://doi.org/10.1038/35066065
- Dumont J, Desai A. Acentrosomal spindle assembly and chromosome segregation during oocyte meiosis. Trends Cell Biol 2012; 22:241–9; PMID:22480579; https://doi.org/10.1016/j.tcb.2012.02.007
- Arrowsmith CH, Bountra C, Fish PV, Lee K, Schapira M. Epigenetic protein families: A new frontier for drug discovery. Nat Rev Drug Discov 2012; 11:384–400; PMID:22498752; https://doi.org/10.1038/nrd3674
- Haakenson J, Zhang X. HDAC6 and ovarian cancer. Int J Mol Sci 2013; 14:9514–35; PMID:23644884; https://doi.org/10.3390/ijms14059514
- Bassett SA, Barnett MP. The role of dietary histone deacetylases (HDACs) inhibitors in health and disease. Nutrients 2014; 6:4273–301; PMID:25322459; https://doi.org/10.3390/nu6104273
- Simões-Pires C, Zwick V, Nurisso A, Schenker E, Carrupt PA, Cuendet M. HDAC6 as a target for neurodegenerative diseases: What makes it different from the other HDAC? Mol Neurodegener 2013; 8:7; PMID:23356410; https://doi.org/10.1186/1750-1326-8-7
- Seto E, Yoshida M. Erasers of histone acetylation: The histone deacetylase enzymes. Cold Spring Harb Perspect Biol 2014; 6:a018713; PMID:24691964; https://doi.org/10.1101/cshperspect.a018713
- Shein NA, Shohami E. Histone deacetylase inhibitors as therapeutic agents for acute central nervous system injuries. Mol Med 2011; 17:448–56; PMID:21274503; https://doi.org/10.2119/molmed.2011.00038
- Ma P, Schultz RM. HDAC1 and HDAC2 in mouse oocytes and preimplantation embryos: Specificity versus compensation. Cell Death Differ 2016; 23:1119–27; PMID:27082454; https://doi.org/10.1038/cdd.2016.31
- Kageyama S, Liu H, Nagata M, Aoki F. Stage specific expression of histone deacetylase 4 (HDAC4) during oogenesis and early preimplantation development in mice. J Reprod Dev 2006; 52:99–106; PMID:16293940; https://doi.org/10.1262/jrd.17044
- Kai Z, Yingchun J, Zhendan He, Kitazato K, Wang Y. Cellular defense or viral assist: The dilemma of HDAC6. J Gen Virol 2017; 98:322–337; DOI:10.1099/jgv.0.000679. Epub 2017 Apr 1. PMID: 27959772
- Verdel A, Seigneurin-Berny D, Faure AK, Eddahbi M, Khochbin S, Nonchev S. HDAC6-induced premature chromatin compaction in mouse oocytes and fertilised eggs. Zygote 2004; 11:323–8; https://doi.org/10.1017/S0967199403002387
- Noack M, Leyk J, Richter-Landsberg C. HDAC6 inhibition results in tau acetylation and modulates tau phosphorylation and degradation in oligodendrocytes. Glia 2014; 62:535–47; PMID:24464872; https://doi.org/10.1002/glia.22624
- Topalidou I, Keller C, Kalebic N, Nguyen KC, Somhegyi H, Politi KA, Heppenstall P, Hall DH, Chalfie M. Genetically separable functions of the MEC-17 tubulin acetyltransferase affect microtubule organization. Curr Biol 2012; 22:1057–65; PMID:22658602; https://doi.org/10.1016/j.cub.2012.03.066
- Cueva JG, Hsin J, Huang KC, Goodman MB. Posttranslational acetylation of alpha-tubulin constrains protofilament number in native microtubules. Curr Biol 2012; 22:1066–74; PMID:22658592; https://doi.org/10.1016/j.cub.2012.05.012
- Sudo H, Baas PW. Acetylation of microtubules influences their sensitivity to severing by katanin in neurons and fibroblasts. J Neurosci 2010; 30:7215–26; PMID:20505088; https://doi.org/10.1523/JNEUROSCI.0048-10.2010
- Tanaka TU, Clayton L, Natsume T. Three wise centromere functions: See no error, hear no break, speak no delay. EMBO Rep 2013; 14:1073–83; PMID:24232185; https://doi.org/10.1038/embor.2013.181
- Nogales E. High-resolution model of the microtubule. Cell 1999; 96:79–88; PMID:9989499; https://doi.org/10.1016/S0092-8674(00)80961-7
- Piperno G, LeDizet M, Chang XJ. Microtubules containing acetylated α-tubulin in mammalian cells in culture. J Cell Biol 1987; 104:289–302; PMID:2879846; https://doi.org/10.1083/jcb.104.2.289
- Bertos NR, Gilquin B, Chan GK, Yen TJ, Khochbin S, Yang XJ. Role of the tetradecapeptide repeat domain of human histone deacetylase 6 in cytoplasmic retention. J Biol Chem 2004; 279:48246–54; PMID:15347674; https://doi.org/10.1074/jbc.M408583200
- Mahlknecht U, Schnittger S, Landgraf F, Schoch C, Ottmann OG, Hiddemann W, Hoelzer D. Assignment of the human histone deacetylase 6 gene (HDAC6) to X chromosome p11.23 by in situ hybridization. Cytogenet Cell Genet 2001; 93:135–6; PMID:11474198; https://doi.org/10.1159/00005696710.1159/000056968
- Nakagawa S, FitzHarris G. Intrinsically defective microtubule dynamics contribute to age-related chromosome segregation errors in mouse oocyte meiosis-I. Curr Biol 2017; 27:1040–7; PMID:28376326; https://doi.org/10.1016/j.cub.2017.02.025
- Tanaka K. Regulatory mechanisms of kinetochore-microtubule interaction in mitosis. Cell Mol Life Sci 2013; 70:559–79; PMID:22752158; https://doi.org/10.1007/s00018-012-1057-7
- Foley EA, Kapoor TM. Microtubule attachment and spindle assembly checkpoint signalling at the kinetochore. Nat Rev Mol Cell Biol 2013; 14:25–37; PMID:23258294; https://doi.org/10.1038/nrm3494
- Wang Z, Leng Y, Wang J, Liao HM, Bergman J, Leeds P, Kozikowski A, Chuang DM. Tubastatin A, an HDAC6 inhibitor, alleviates stroke-induced brain infarction and functional deficits: Potential roles of alpha-tubulin acetylation and FGF-21 up-regulation. Sci Rep 2016; 6:19626; PMID:26790818; https://doi.org/10.1038/srep19626
- Zilberman Y, Ballestrem C, Carramusa L, Mazitschek R, Khochbin S, Bershadsky A. Regulation of microtubule dynamics by inhibition of the tubulin deacetylase HDAC6. J Cell Sci 2009; 122:3531–41; PMID:19737819; https://doi.org/10.1242/jcs.046813
- MacRAE TH. Tubulin post-translational modifications-enzymes and their mechanisms of action. Eur J Biochem 1997; 244:265–78; PMID:9118990; https://doi.org/10.1111/j.1432-1033.1997.00265.x
- Dompierre JP, Godin JD, Charrin BC, Cordelieres FP, King SJ, Humbert S, Saudou F. Histone deacetylase 6 inhibition compensates for the transport deficit in Huntington's disease by increasing tubulin acetylation. J Neurosci 2007; 27:3571–83; PMID:17392473; https://doi.org/10.1523/JNEUROSCI.0037-07.2007
- Reed NA, Cai D, Blasius TL, Jih GT, Meyhofer E, Gaertig J, Verhey KJ. Microtubule acetylation promotes kinesin-1 binding and transport. Curr Biol 2006; 16:2166–72; PMID:17084703; https://doi.org/10.1016/j.cub.2006.09.014
- de Pennart H, Houliston E, Maro B. Post-translational modifications of tubulin and the dynamics of microtubules in mouse oocytes and zygotes. Bio Cell 1988; 64:375–8; PMID:2906552
- Ma R, Hou X, Zhang L, Sun SC, Schedl T, Moley K, Wang Q. Rab5a is required for spindle length control and kinetochore-microtubule attachment during meiosis in oocytes. FASEB J 2014; 28:4026–35; PMID:24876181; https://doi.org/10.1096/fj.14-250886
- Wang Q, Chi MM, Moley KH. Live imaging reveals the link between decreased glucose uptake in ovarian cumulus cells and impaired oocyte quality in female diabetic mice. Endocrinology 2012; 153:1984–9; PMID:22294751; https://doi.org/10.1210/en.2011-1815