ABSTRACT
Blood flow provides endothelial cells (ECs) lining the inside of blood vessels with mechanical stimuli as well as humoral stimuli. Fluid shear stress, the frictional force between flowing blood and ECs, is recognized as an essential mechanical cue for vascular growth, remodeling, and homeostasis. ECs differentially respond to distinct flow patterns. High laminar shear flow leads to inhibition of cell cycle progression and stabilizes vessels, whereas low shear flow or disturbed flow leads to increased turnover of ECs and inflammatory responses of ECs prone to atherogenic. These differences of EC responses dependent on flow pattern are mainly ascribed to distinct patterns of gene expression. In this review, we highlight flow pattern-dependent transcriptional regulation in ECs by focusing on KLF2 and NFκB, major transcription factors responding to laminar flow and disturbed flow, respectively. Moreover, we introduce roles of a new flow-responsive transcriptional co-regulator, YAP, in blood vessel maintenance and discuss how these transcriptional regulators are spatiotemporally regulated by flow and then regulate EC functions in normal and pathological conditions.
Overview of endothelial cell (EC) responses to shear stress
Endothelial cells (ECs) lining blood vessels are continuously exposed to blood flow. While blood flow affects ECs by conveying molecules including growth factors, cytokines, and hormones, it acts on ECs by providing mechanical forces. ECs sense mainly 2 types of mechanical stimuli from blood flow: one is shear stress, the frictional force tangential to ECs; the other is mechanical strain, the force perpendicular to the direction of flow, related to rhythmic heart beating.Citation1-4 Flow-mediated mechanical stimuli regulate various aspects of vascular functions including blood vessel formation, maintenance, and remodeling by acting on ECs and smooth muscle cells (SMCs). Vascular remodeling, which includes alterations of the distribution and diameter of blood vessels, allows them to meet the demand of tissues so that they receive appropriate perfusion with maximal efficiency.Citation5,6 Although our knowledge about mechanical strain- or hydrostatic pressure-mediated mechanotransduction in ECs is limited, extensive studies provide much information about shear stress-induced multiple mechanotransduction signals.Citation1,2
Shear stress is a weak force that is less than 1/100 of mechanical strain.Citation7 Therefore, highly sensitive sensing mechanisms is necessary to sense shear stress. Because flow is directly in touch with apical surfaces of ECs, the structures located on the apical side, including primary cilia, glycocalyx, ion channels, and lipid bilayer membranes, have been proposed as direct shear stress sensors.Citation8-12,13 A mechanosensory complex at cell-cell junctions, consisting of PECAM-1, VE-cadherin, and VEGFR2,Citation14 and a mechanosensitive cationic channel, Piezo1,Citation15 have been characterized as sensor molecules responding to flow. In addition, caveolae and vimentin intermediate filaments are also proposed as shear stress sensors,Citation16,17 although they are not mainly located on the apical surfaces of ECs. It still remains a central topic how sear stress is sensed by ECs in vivo. These sensing mechanisms might act individually depending on the situations. Alternatively, multiple sensing mechanisms might function simultaneously in a coordinated manner.
ECs differentially respond to different patterns of flow. In straight portions of a blood vessel, ECs are exposed to uniform laminar shear stress (). In contrast, the flow pattern is variously altered dependently on vessel curvatures, stenosis, and branching (). In those regions, the flow becoming reversed and separated gives low shear stress to ECs.Citation1,18-20 High laminar shear stress promotes EC survival and renders ECs quiescent with low proliferation rate (). Laminar shear stress suppresses cell cycle progression by upregulating cyclin-dependent kinase inhibitor 1 (p21) and reduced phosphorylation of retinoblastoma protein (Rb).Citation21,22 On the contrary, disturbed flow enhances apoptosis and proliferation of ECs, thereby leading to increased EC turnover ().Citation23-26 Furthermore, disturbed flow enhances vascular permeability and promotes inflammatory gene expression that induces accumulation of monocytes.Citation27,28 It also promotes endothelial senescence via a p53 dependent signaling pathway.Citation29
Figure 1. Different endothelial responses to different patterns of flow. (A) ECs lining straight blood vessels are exposed to laminar shear stress, whereas those linging branching or curved blood vessels are exposed to disturbed flow. (B) High laminar flow induces quiesent phenotypes in ECs including low proliferation rate, reduced cell death, and anti-inflammatory gene expression (left panels), while low or disturbed flow induces atheroprone phenotypes in ECs including high proliferation rate, increased cell death, and inflammatory gene expression (right panels). High laminar flow induces the expression of KLF2 and KLF4 via the MEK5/ERK5/MEF2 signaling pathway. KLF2 promotes the activation of Nrf2 and both KLF2 and Nrf2 promote quiescent phenotypes of ECs. Low or disturbed flow upregulates the expression of NFκB through JNK and its downstream ATF2. Flow-driven NFκB activation is mediated by integrin-dependent signaling upon binding to fibronectin. AP-1-, EGR1-, and YAP-dependent gene expression is also induced by disturbed flow. RhoA and F-actin are responsible for determinging the localization of YAP in sheared ECs, although it is not determined whether disturbed flow regulates the canonical Hippo pathway.
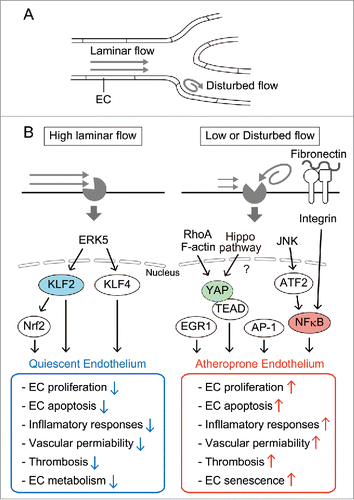
These disturbed flow-dependent regulation of ECs is involved in the onset and progression of atherosclerosis. High steady laminar flow or pulsatile flow is atheroprotective, while low or disturbed flow creates an atheroprone environment for blood vessels.Citation1,18-20 Indeed, atherosclerotic lesions develop primarily at bifurcations of medium-sized arteries in which disturbed flow is observed.Citation30 However, it is unknown how ECs can distinguish the different flow patterns. Distinct shear stress sensors might be used according to flow patterns.
Transcriptional regulators responsible for EC responses to flow
Shear stress induces mechanotransduction signals inside of ECs, although the surface mechanosensory systems of ECs are yet unclear. The mechanotransduction signals are also differentially regulated by different flow patterns.Citation1,18,20 It is clear that shear stress affects EC functions mainly through global changes in gene expression mediated by flow-regulated transcriptional factors (TFs). Kruppel-like factor 2 (KLF2) is a major flow-regulated transcription factor, because KLF2 regulates ∼46% of highly flow-responsive genes in ECs.Citation31,32 Atheroprotective laminar flow, but not disturbed flow, upregulates KLF2 and KLF4, another member of KLF family TFs.Citation32-35 KLF2 promotes the activation of antioxident TF, Nrf2. Both KLF2 and Nrf2 induce quiescence of ECs characterized by the reduction of inflammatory responses ().Citation36-38 Nuclear factor-κb (NFκB) is a representative TF that is enhanced by disturbed flow.Citation39,40 Whereas expression of NFκB is driven by disturbed flow in a sustained manner, longer laminar shear stress reduces the activity of NFκB below the base line. Similar to NFκB, activator protein-1 (AP-1) and early growth response-1 (EGR1) are also induced by disturbed flow ().Citation40 Through the coordinated expression of these TFs, disturbed flow upregulates pro-atherosclerotic genes.Citation1,18 In lymphatic endothelial cells (LECs), disturbed flow induces FOXC2, a forkhead TF important for lymphatic vessel development and lymphatic valve formation.Citation41
Recently, we and other group have identified Yes-associated protein (YAP), a transcriptional cofactor downstream of the Hippo signaling pathway, as a novel mechanotransducer in ECs.Citation42-44 Endothelial YAP responds to flow-mediated mechanical stimuli both in vitro and in vivo and is important for vessel maintenance and atherosclerosis.
KLF2 – a transcription factor responsible for atheroprotective flow-induced regulation of vascular tones
KLF2 is a member of the zinc finger family of TFs and is expressed in ECs in vivo.Citation45,46 High laminar shear stress and unidirectional pulsatile shear stress, both of which are atheroprotective flow, induce the expression of KLF2 and its paralog KLF4 in a sustained manner via the MEK5/ERK5/MEF2 signaling pathway.Citation32-35 KLF2 expression in ECs is dependent on blood flow in vivo, because endothelial KLF2 expression is lost without blood flow.Citation32,47 KLF2 expression is increased in the region exposed to high shear stress in developing chick embryos.Citation48 In mouse embryo, endothelial KLF2 expression is higher in arteries than in veins.Citation49 Consistently, higher KLF2 expression is induced by unidirectional pulsatile flow mimicking arterial flow than by steady laminar flow in cultured ECs.Citation35 In human, KLF2 expression is relatively low at the outer wall of the bifurcation of the aorta and iliac arteries and at the branch of the common carotid artery from the aortic arch, where blood flow is disturbed and atheroprone.Citation35,50 Similarly in cultured ECs, atheroprone flow does not enhance KLF2 expression.Citation32 Thus, KLF2 responds to atheroprotective flow.
In response to atheroprotective flow, KLF2 functions as a transcriptional integrator for multiple vascular protective functions.Citation31,32,51 KLF2 controls vascular tones by inducing the expression of endothelial nitric oxide (NO) synthase (eNOS) and caveolin1.Citation32,50 Under laminar shear stress, KLF2 reduces vessel permeability, thrombosis, and endothelial metabolism.Citation32,52-54 In addition, KLF2 represses inflammatory responses induced by IL-1β or TNF-αCitation53,55 Hemizygous deficiency of KLF2 increases atherosclerosis in apolipoprotein E (apoE) deficient mice.Citation56 However, the contribution of endothelial KLF2 expression to this phenotype remains to be defined. KLF4 also represses inflammatory responses in ECs.Citation57,58 Endothelial specific loss-of-function of KLF4 results in increased atherosclerosis in apoE deficient mice,Citation58 demonstrating atheroprotective functions of endothelial KLF4.
In addition to the vascular protective role of KLF2, an angiogenic role of KLF2 is reported in zebrafish. Klf2a is important for flow-stimulated angiogenesis during aortic arch development.Citation59 Klf2a induces expression of an EC-specific microRNA, miR-126, to activate VEGFR2 signaling. KLF2 is also responsible for ectopic angiogenesis in cerebral cavernous malformation (CCM) mutant zebrafish.Citation60 The question whether KLF2 is a general regulator of flow-stimulated angiogenesis should be solved in the future. In zebrafish, Klf2a is also important for the maintenance of haematopoietic stem cells (HSCs), another flow-regulated process, because knockdown of Klf2a resulted in the loss of HSC.Citation47 These phenotypes are shown in the morphants of klf2a using morpholino antisense oligonucleotides (MOs). Analyses using klf2 mutant fish would be more beneficial to understand its physiologic role. klf2 mutant zebrafish (klf2a and klf2b) exhibit reduced diameter of the axial blood vessels and increased blood flow velocity,Citation61 indicating an essential function of KLF2 in the vascular tone regulation. These mutant fish also show defects in flow-induced EC polarization.Citation61 Consistently, constitutive and EC-specific KLF2 knockout mice die at embryonic day 10.5 owning to lack of vascular tone, bleeding, and cardiac dysfunction,Citation46,49 although vessel patterning and density appear normal in these knockout mice.Citation46,49,62 KLF2 deficient mice exhibit disorganized SMC due to the failure of SMC migration.Citation62 Thus, endothelial KLF2 might affect smooth muscle tone in vivo.
Gene expression studies using cultured ECs identify numerous KLF2 target genes that could affect vascular smooth muscle tone.Citation32,50 Hergenreider et al. reveal that KLF2 upregulates miR-143/145 controling SMC phenotype.Citation63 In HUVECs, shear stress induces miR-143- and miR-145-containing extracellular vesicles through KLF2. These miRNAs can be transferred to SMCs and regulate SMC differentiation, suggesting that endothelial KLF2 regulates communication between ECs and SMCs under shear stress.
In mice, KLF2 is detected in the endocardium overlying the endocardial cushions and the common outflow tract that are exposed particularly to high shear stress.Citation49 Also, in zebrafish, Klf2a is highly expressed in the endocardium within the atrioventricular canal (AVC) in a manner dependent on oscillatory flow.Citation64,65 klf2a mutant zebrafish exhibit variety of abnormal phenotypes in atrioventricular valve formation, including valve deficiency or thickened valve leaflets.Citation64 In endocardium, KLF2a regulates fibronectin synthesis in the AVC for proper valve formation, suggesting the essential responses of endocardial endothelial cells to high shear stress.
NFκB – a transcription factor involved in inflammatory responses to atheroprpne flow
NFκB is a ubiquitous TF that participates in inflammatory, immune, and developmental processes.Citation67 NFκB family members, p65 (RelA), RelB, c-Rel, p105/p50 (NFκB1), p100/p52 (NFκB2), exist as homo- or heterodimers. Among them, p50/p65 is predominantly expressed in ECs.Citation68 NFκB is inactive when localized in the cytoplasm, where it is retained with inhibitors of NFκB (IκB) proteins.Citation69 After stimulation, IκB proteins are phosphorylated by IκB kinase (IKK) complex, ubiquitinated, and subjected to subsequent degradation in 26S proteasome. NFκB then translocates into the nucleus, thereby promoting the expression of target genes.
In cultured ECs, disturbed flow, and steady or pulsatile low shear stress enhance nuclear NFκB (p65) and its transcriptional activity in a sustained manner.Citation39,40 In contrast, higher laminar shear stress induces NFκB activation in a rapid and transient manner (∼30 min).Citation39 Notably, the extent of NFκB activation in response to laminar shear stress is determined by flow direction relative to the cell axis.Citation70 Whereas laminar flow in a direction which highly diverges from the cell axis induces NFκB activation, laminar flow parallel to the cell axis does not. Therefore, ECs that have already been pre-flowed and aligned to the flow direction do not respond to laminar flow parallel to the cell axis. Besides flow direction, the frequency components of shear stress waveforms are important for effective NFκB activation.Citation71 The IKK-IκB pathway mediates nuclear translocation of NFκB induced by shear stress. Consistently, IKK activation and subsequent IκB degradation are induced by shear stress.Citation72
Nuclear NFκB binds to a shear stress responsive element (SSRE) found in the promoter of atherogenic adhesion molecules, including ICAM-1, VCAM-1, and E-selectin,Citation73 as well as PDGF, a main regulator of SMC development.Citation74 Because expression of ICAM-1 and VCAM-1 is increased in ECs of atherosclerotic-prone regions and atherosclerotic lesions,Citation75,76 NFκB may participate in early events in atherosclerotic progression. Consistently, NFκB is detected in the nucleus of ECs in the atherosclerotic lesions.Citation77
In arterial ECs, NFκB (p65) is highly expressed in atheroprone regions where blood flow is disturbed.Citation27,78 In these ECs, disturbed flow enhances NFκB expression through JNK and its downstream transcription factor ATF2.Citation78 Unexpectedly, in these NFκB-expressing ECs, nuclear translocation of NFκB is detected only in a small population (< 15%) probably because of higher IκB expression in these regions.Citation27,79 Thus, in the ECs experiencing disturbed flow in vivo, NFκB is not fully activated but is primed for the activation to respond to activation stimuli including atherogenic diet. Thus, disturbed shear stress might not be sufficient for full activation of NFκB in vivo. Additional extracellular factors including inflammatory cytokines, eg, TNF-α, and subendothelial ECM may be required for triggering NFκB activation. For example, increased fibronectin deposition is associated with atherosclerotic progression in apoE deficient mice.Citation80,81 Flow triggers NFκB activation in ECs on fibronectin but not on collagen or laminin.Citation80,82 Integrin αvβ3 and α5β1 are activated by shear stress on fibronectin but not on collagenCitation83-85 and mediate shear stress-induced NFκB activation in cultured ECs.Citation86,87 Thus, flow regulates endothelial NFκB activity in concert with surrounding microenvironment and integrin signaling. NFκB mediates an increase in fibronectin expression under disturbed flow, thereby creating a positive feedback loop to maintain inflammatory responses.Citation82
Several lines of evidence using an in vitro model and a mouse retinal neovascularization model suggest an angiogenic role of NFκB.Citation88-90 In zebrafish embryo, NFκB signaling functions in maintaining vessel integrity and stabilization.Citation91 Therefore, flow-regulated NFκB might also functions in normal vascular development besides atherosclerosis.
YAP – a novel transcriptional co-activator responding to blood flow in vivo
YAP, originally known as a final effector molecule of the Hippo signaling pathway,Citation92 has been recently identified as a mediator for mechanical stimuli.Citation93 YAP is a transcription cofactor that shuttles between the cytoplasm and nucleus where it associates with transcriptional factors. Among the TFs YAP potentially binds to including RUNX1, RUNX2, Smad, p73, and Nkx2.5, TEA domain (TEAD) family members are regarded as the main partners of YAP.Citation93-95
The Hippo signaling pathway has emerged as a key negative regulator of tissue growth in Drosophila and mammalian systems.Citation96 The Hippo signaling pathway negatively regulates YAP by inducing nuclear export of YAP. In the Hippo signaling cascade, MST1/2 kinases phosphorylate and activate LATS1/2 kinases, which in turn directly phosphorylate YAP, thereby exporting YAP from the nucleus.Citation92,97 Hippo-mediated YAP phosphorylation is a fundamental input for YAP regulation but not the only one, because nuclear localization of YAP can be often regulated by upstream components independent of the core Hippo signaling cascade or YAP phosphorylation by LATS1/2.Citation42,98-101
The mechanical stimuli, which are generated by extracellular matrix rigidity, cell strain, and cell density, control nuclear translocation of YAP through the modulation of filamentous-actin (F-actin) structures.Citation98,99,102-105 Recently, we and other groups have shown that endothelial YAP responds to shear stress. In cultured ECs, YAP and its paralogue, transcriptional coactivator with PDZ-binding motif (TAZ, also known as WWTR1), translocate into the nucleus and promotes TEAD-dependent transcription by disturbed or oscillatory flow in a sustained manner ().Citation43,44,106 On the contrary, unidirectional laminar shear stress transiently induces nuclear translocation of YAP in acute phase (10–60 min).Citation42 However, because YAP is excluded from the nucleus under longer laminar shear stress (∼6 h), its transcriptional activity is suppressed.Citation44 Thus, the localization of YAP might be controlled by different flow patterns. Similar to NFκB, the response of YAP to flow might vary according to flow direction relative to the cell axis. YAP phosphorylation is reduced by disturbed flow but is not altered by acute laminar shear stress.Citation42-44 It is not determined whether shear stress regulates the canonical Hippo signaling pathway. F-actin appears to be a common regulator of YAP under different patterns of flow. RhoA, a small GTPase involved in assembly and bundling of F-actin, regulates YAP in sheared ECs ().Citation43,44 Consistently, thick cortical actin bundles along cell-cell boundaries are commonly assembled by both transient laminar shear stress and longer (48 h) oscillatory shear stress in ECs, whereby nuclear localization of YAP is enhanced.Citation42,106,107 Angiomotin (AMOT) links actin organization to YAP localization. AMOT sequesters YAP in the cytoplasm via a direct interaction in the static state.Citation42,108-110 Upon acute laminar shear stress, part of AMOT pool preferentially binds to enhanced cortical actin bundles and thereby releases YAP. Subsequently, released YAP enters into the nucleus to promote TEAD-dependent transcription.Citation42 The similar mechanism might also control nuclear localization of YAP under disturbed flow.
Figure 2. Flow-regulated YAP-dependent transcriptional activity, and its targets, and physiologic/pathological functions. While acute laminar shear stress induces nuclear translocation of YAP and enhances TEAD-dependent transcription, longer laminar shear stress reverses them. In contrast, disturbed flow induces nuclear translocation of YAP in a sustained manner. In response to disturbed flow, YAP enhances a variety of genes involved in cell cycle and inflammatory responses. Then, YAP regulates EC proliferation, vascular maintenance, and atherogenesis.
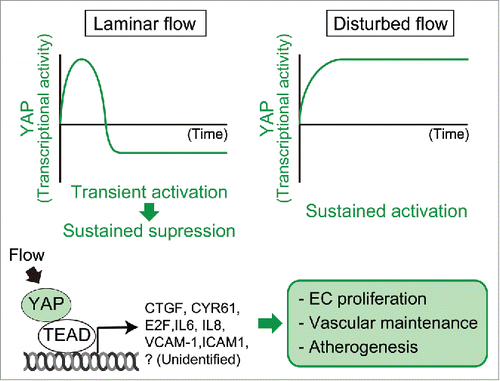
We developed the transgenic (Tg) zebrafish systems that could indirectly monitor spatio-temporal localization and transcriptional activity of Yap1 (zebrafish YAP) in living zebrafish and revealed that endothelial Yap1 responded to blood flow in vivo.Citation42,111,112 In accordance with YAP regulation by different flow patterns in cultured ECs, Yap1-dependent transcription is sustained in the vessels including the dorsal longitudinal anastomotic vessel (DLAV), where blood flow is disturbed, while it is transient in the dorsal aorta (DA), where blood flow is unidirectional.Citation42 Also in mice, in outer curvature of aortic arch and thoracic aorta, where blood flow is unidirectional, YAP is predominantly localized in the cytoplasm, while in the inner curvature of aortic arch, where blood flow is disturbed, YAP is mainly localized in the nucleus.Citation44
In cultured ECs, YAP regulates cell cycle progression.Citation43 YAP promotes hyperphosphorylation of RB protein and controls the expression of cell cycle regulatory genes in ECs exposed to shear stress (). In zebrafish, Yap1 is important for the maintenance of some blood vessels including the DLAV and the central artery (CtA), since yap1 mutant larvae exhibit enhanced lumen stenosis in these vessels ().Citation42 Future studies are needed to elucidate the mechanisms by which Yap1 stabilizes blood vessels in response to blood flow. In addition, nuclear translocation of YAP and TAZ leads to disturbed flow-induced inflammatory responses by increasing JNK ().Citation43,44 Consistently, flow-induced nuclear translocation of YAP/TAZ is related to atherosclerosis. CTGF and CYR61, well-characterized YAP/TAZ target genes, are highly expressed in human atherosclerotic lesions.Citation113-115 In apoE deficient mice, increased atherosclerotic plaques are induced by EC-specific overexpression of YAP, whereas the overall size of atherosclerotic plaques is reduced by EC-specific YAP knockdown or by blockade of YAP/TAZ translation in the partial ligation (PL)-operated left carotid artery (LCAs).Citation43,44 Thus, inhibition of YAP will become a promising therapeutic strategy against atherosclerosis.
We also report flow-independent nuclear translocation of YAP in vessel sprouts of zebrafish brain vessels.Citation42 Nuclear localization of YAP is similarly observed in the angiogenic front of the mouse retina where flow is absent.Citation116 In the developing mouse retina, YAP functions as an important regulator for angiogenesis through the regulation of Angiopoetin-2 expression. Thus, YAP could be regulated in both flow-dependent and flow-independent manners in ECs. It would also be a next challenge to elucidate what extracellular stimuli regulate YAP functions during angiogenesis in vivo.
Crosstalks among shear stress-regulated transcriptional factors
NFκB and KLF2 signaling mutually play antagonistic roles in ECs.Citation117 NFκB reduces KLF2 expression by inhibiting MEF2 transcriptional activity.Citation118 This inhibitory effect is due to the cooperative action of NFκB (p65) and histone deacetylases (HDAC4 and HDAC5). On the other hand, KLF2 and KLF4 inhibit NFκB function and expression of adhesion molecules.Citation55,57 Similarly, MEF2 that can stimulate KLF2 expression also inhibits the cytokine-induced NFκB activation.Citation119 KLF2 alters neither nuclear translocation nor DNA-binding property of NFκB. Instead, KLF2 binds to CBP/p300, a coactivator required for optimal NFκB activity. By competing with NFκB for binding to CBP/p300, KLF2 reduces NFκB-dependent transcriptional activity.Citation55 Furthermore, KLF2 inhibits nuclear activity of ATF2, one of the heterodimeric components of AP-1, in response to laminar shear stress.Citation120 Thus, shear stress-regulated TFs interact with each other in ECs. Therefore, it is assumed that ECs differentially respond to different flow patterns through these crosstalks between TFs. The Hippo-YAP signaling coordinately functions with various other key signaling pathways including TGFβ/SMAD, Delta/Notch, JAK/STAT, and Wnt/β-catenin signaling.Citation95 Therefore, YAP might associate with the flow-regulated TFs in a complex transcriptional network. Because disturbed flow-driven YAP/TAZ activation in ECs promotes the expression of inflammation-related genes by enhancing JNK activity,Citation44 there might be a functional relationship between YAP/TAZ and NFκB signaling in sheared ECs.
Concluding remarks
Transcriptional regulation is a fundamental process in EC responding to blood flow-mediated mechanical stimuli. Multiple expression profiling studies have uncovered shear stress-responsive genes regulated by various flow patterns both in cultured ECs and in vivo.Citation32,35,121-124 Expression of atherosclerosis-related genes is closely related to disturbed flow in vivo.Citation79,125,126 However, it is not fully understood why atherosclerosis-related genes should be enhanced in ECs where flow is disturbed. This might be due to the maladaptation of ECs to disturbed blood flow.Citation20 Alternatively, the genes upregulated by disturbed flow might have some positive functions in normal blood vessels. For example, the inflammatory NFκB signaling is involved in the HSC emergence in the DA and the vessel integrity during normal development of zebrafish.Citation91,127,128 YAP also has dual functions, vessel maintenance and atherosclerosis, in the regions where blood flow is disturbed.Citation42-44 It would be interesting to investigate non-pathological function of disturbed flow in vivo. In addition, shear stress is not a sole mechanical stimulus mediated by blood flow in living animals. Therefore, it is important to understand how shear stress and other mechanical factors including mechanical strain cooperatively regulate gene expression.
Disclosure of potential conflicts of interest
No potential conflicts of interest were disclosed
Acknowledgments
We thank all laboratory members for their help, advice, and encouragement.
Funding
The studies in our laboratory were supported by JSPS KAKENHI (No. 16 H02618 to N.M.; No. 17K08560 to H.N.); AMED-CREST (No. 13414779 to N.M.)
References
- Hahn C, Schwartz MA. Mechanotransduction in vascular physiology and atherogenesis. Nat Rev Mol Cell Biol. 2009;10:53-62. doi:10.1038/nrm2596. PMID:19197332
- Zhou J, Li YS, Chien S. Shear stress-initiated signaling and its regulation of endothelial function. Arterioscler Thromb Vasc Biol. 2014;34:2191-2198. doi:10.1161/ATVBAHA.114.303422
- Ando J, Yamamoto K. Effects of shear stress and stretch on endothelial function. Antioxid Redox Signal. 2011;15:1389-1403. doi:10.1089/ars.2010.3361. PMID:20854012
- Freund JB, Goetz JG, Hill KL, Vermot J. Fluid flows and forces in development. functions, features and biophysical principles. Development. 2012;139:1229-1245. doi:10.1242/dev.073593. PMID:22395739
- Chen Q, Jiang L, Li C, Hu D, Bu JW, Cai D, Du JL. Haemodynamics-driven developmental pruning of brain vasculature in zebrafish. PLoS Biol. 2012;10:e1001374. doi:10.1371/journal.pbio.1001374. PMID:22904685
- Lucitti JL, Jones EA, Huang C, Chen J, Fraser SE, Dickinson ME. Vascular remodeling of the mouse yolk sac requires hemodynamic force. Development. 2007;134:3317-3326. doi:10.1242/dev.02883. PMID:17720695
- Baeyens N, Schwartz MA. Biomechanics of vascular mechanosensation and remodeling. Mol Biol Cell. 2016;27:7-11. doi:10.1091/mbc.E14-11-1522. PMID:26715421
- Ando J, Yamamoto K. Flow detection and calcium signalling in vascular endothelial cells. Cardiovasc Res. 2013;99:260-268. doi:10.1093/cvr/cvt084. PMID:23572234
- Tarbell JM, Simon SI, Curry FR. Mechanosensing at the vascular interface. Annu Rev Biomed Eng. 2014;16:505-532. doi:10.1146/annurev-bioeng-071813-104908. PMID:24905872
- Li J, Hou B, Tumova S, Muraki K, Bruns A, Ludlow MJ, Sedo A, Hyman AJ, McKeown L, Young RS, Yuldasheva NY, et al. Piezo1 integration of vascular architecture with physiological force. Nature. 2014;515:279-282. doi:10.1038/nature13701. PMID:25119035
- Hierck BP, Van der Heiden K, Alkemade FE, Van de Pas S, Van Thienen JV, Groenendijk BC, Bax WH, Van der Laarse A, Deruiter MC, Horrevoets AJ, et al. Primary cilia sensitize endothelial cells for fluid shear stress. Dev Dyn 2008;237:725-735. doi:10.1002/dvdy.21472. PMID:18297727
- Pahakis MY, Kosky JR, Dull RO, Tarbell JM. The role of endothelial glycocalyx components in mechanotransduction of fluid shear stress. Biochem Biophys Res Commun. 2007;355:228-233. doi:10.1016/j.bbrc.2007.01.137
- Goetz JG, Steed E, Ferreira RR, Roth S, Ramspacher C, Boselli F, Charvin G, Liebling M, Wyart C, Schwab Y, et al. Endothelial cilia mediate low flow sensing during zebrafish vascular development. Cell Rep. 2014;6:799-808. doi:10.1016/j.celrep.2014.01.032
- Tzima E, Irani-Tehrani M, Kiosses WB, Dejana E, Schultz DA, Engelhardt B, Cao G, DeLisser H, Schwartz MA. A mechanosensory complex that mediates the endothelial cell response to fluid shear stress. Nature. 2005;437:426-431. doi:10.1038/nature03952. PMID:16163360
- Li J, Hou B, Tumova S, Muraki K, Bruns A, Ludlow MJ, Sedo A, Hyman AJ, McKeown L, Young RS, et al. Piezo1 integration of vascular architecture with physiological force. Nature. 2014;515:279-282. doi:10.1038/nature13701. PMID:25119035
- Helmke BP, Goldman RD, Davies PF. Rapid displacement of vimentin intermediate filaments in living endothelial cells exposed to flow. Circ Res. 2000;6:745-752. doi:10.1161/01.RES.86.7.745
- Yu J, Bergaya S, Murata T, Alp IF, Bauer MP, Lin MI, Drab M, Kurzchalia TV, Stan RV, Sessa WC. Direct evidence for the role of caveolin-1 and caveolae in mechanotransduction and remodeling of blood vessels. J Clin Invest. 2006;116:1284-1291. doi:10.1172/JCI27100. PMID:16670769
- Chiu JJ, Chien S. Effects of disturbed flow on vascular endothelium: Pathophysiological basis and clinical perspectives. Physiol Rev. 2011;91:327-387. doi:10.1152/physrev.00047.2009. PMID:21248169
- Heo KS, Fujiwara K, Abe J. Shear stress and atherosclerosis. Mol Cells. 2014;37:435-440. doi:10.14348/molcells.2014.0078. PMID:24781409
- Schober A, Nazari-Jahantigh M, Weber C. MicroRNA-mediated mechanisms of the cellular stress response in atherosclerosis. Nat Rev Cardiol. 2015;12:361-374. doi:10.1038/nrcardio.2015.38. PMID:25855604
- Akimoto S, Mitsumata M, Sasaguri T, Yoshida Y. Laminar shear stress inhibits vascular endothelial cell proliferation by inducing cyclin-dependent kinase inhibitor p21(Sdi1/Cip1/Waf1). Circ Res. 2000;86:185-190. doi:10.1161/01.RES.86.2.185. PMID:10666414
- Lin K, Hsu PP, Chen BP, Yuan S, Usami S, Shyy JY, Li YS, Chien S. Molecular mechanism of endothelial growth arrest by laminar shear stress. Proc Natl Acad Sci U S A. 2000;97:9385-9389. doi:10.1073/pnas.170282597. PMID:10920209
- Bjorkerud S, Bondjers G. Endothelial integrity and viability in the aorta of the normal rabbit and rat as evaluated with dye exclusion tests and interference contrast microscopy. Atherosclerosis. 1972;15:285-300. doi:10.1016/0021-9150(72)90019-6. PMID:4115209
- Hansson GK, Schwartz SM. Evidence for cell death in the vascular endothelium in vivo and in vitro. Am J Pathol. 1983;112:278-286. PMID:6351628
- Sakao S, Taraseviciene-Stewart L, Lee JD, Wood K, Cool CD, Voelkel NF. Initial apoptosis is followed by increased proliferation of apoptosis-resistant endothelial cells. FASEB J. 2005;19:1178-1180. doi:10.1096/fj.04-3261fje. PMID:15897232
- Tardy Y, Resnick N, Nagel T, Gimbrone MA, Jr., Dewey CF, Jr.. Shear stress gradients remodel endothelial monolayers in vitro via a cell proliferation-migration-loss cycle. Arterioscler Thromb Vasc Biol. 1997;17:3102-3106. doi:10.1161/01.ATV.17.11.3102. PMID:9409299
- Hajra L, Evans AI, Chen M, Hyduk SJ, Collins T, Cybulsky MI. The NF-kappa B signal transduction pathway in aortic endothelial cells is primed for activation in regions predisposed to atherosclerotic lesion formation. Proc Natl Acad Sci U S A. 2000;97:9052-9057. doi:10.1073/pnas.97.16.9052. PMID:10922059
- Jongstra-Bilen J, Haidari M, Zhu SN, Chen M, Guha D, Cybulsky MI. Low-grade chronic inflammation in regions of the normal mouse arterial intima predisposed to atherosclerosis. J Exp Med. 2006;203:2073-2083. doi:10.1084/jem.20060245. PMID:16894012
- Warboys CM, de Luca A, Amini N, Luong L, Duckles H, Hsiao S, White A, Biswas S, Khamis R, Chong CK, et al. Disturbed flow promotes endothelial senescence via a p53-dependent pathway. Arterioscler Thromb Vasc Biol. 2014;34:985-995. doi:10.1161/ATVBAHA.114.303415
- Zarins CK, Giddens DP, Bharadvaj BK, Sottiurai VS, Mabon RF, Glagov S. Carotid bifurcation atherosclerosis. Quantitative correlation of plaque localization with flow velocity profiles and wall shear stress. Circ Res. 1983;53:502-514.
- Atkins GB, Jain MK. Role of Kruppel-like transcription factors in endothelial biology. Circ Res. 2007;100:1686-1695. doi:10.1161/01.RES.0000267856.00713.0a
- Parmar KM, Larman HB, Dai G, Zhang Y, Wang ET, Moorthy SN, Kratz JR, Lin Z, Jain MK, Gimbrone MA, Jr., et al. Integration of flow-dependent endothelial phenotypes by Kruppel-like factor 2. J Clin Invest. 2006;116:49-58. doi:10.1172/JCI24787. PMID:16341264
- Le NT, Heo KS, Takei Y, Lee H, Woo CH, Chang E, McClain C, Hurley C, Wang X, Li F, et al. A crucial role for p90RSK-mediated reduction of ERK5 transcriptional activity in endothelial dysfunction and atherosclerosis. Circulation. 2013;127:486-499. doi:10.1161/CIRCULATIONAHA.112.116988
- Ohnesorge N, Viemann D, Schmidt N, Czymai T, Spiering D, Schmolke M, Ludwig S, Roth J, Goebeler M, Schmidt M. Erk5 activation elicits a vasoprotective endothelial phenotype via induction of Kruppel-like factor 4 (KLF4). J Biol Chem. 2010;285:26199-26210. doi:10.1074/jbc.M110.103127. PMID:20551324
- Dekker RJ, van Soest S, Fontijn RD, Salamanca S, de Groot PG, VanBavel E, Pannekoek H, Horrevoets AJ. Prolonged fluid shear stress induces a distinct set of endothelial cell genes, most specifically lung Kruppel-like factor (KLF2). Blood. 2002;100:1689-1698. doi:10.1182/blood-2002-01-0046. PMID:12176889
- Dai G, Vaughn S, Zhang Y, Wang ET, Garcia-Cardena G, Gimbrone MA, Jr.. Biomechanical forces in atherosclerosis-resistant vascular regions regulate endothelial redox balance via phosphoinositol 3-kinase/Akt-dependent activation of Nrf2. Circ Res. 2007;101:723-733. doi:10.1161/CIRCRESAHA.107.152942
- Zakkar M, Van der Heiden K, Luong lA, Chaudhury H, Cuhlmann S, Hamdulay SS, Krams R, Edirisinghe I, Rahman I, Carlsen H, et al. Activation of Nrf2 in endothelial cells protects arteries from exhibiting a proinflammatory state. Arterioscler Thromb Vasc Biol. 2009;29:1851-1857. doi:10.1161/ATVBAHA.109.193375. PMID:19729611
- Fledderus JO, Boon RA, Volger OL, Hurttila H, Yla-Herttuala S, Pannekoek H, Levonen AL, Horrevoets AJ. KLF2 primes the antioxidant transcription factor Nrf2 for activation in endothelial cells. Arterioscler Thromb Vasc Biol. 2008;28:1339-1346. doi:10.1161/ATVBAHA.108.165811
- Mohan S, Mohan N, Sprague EA. Differential activation of NF-kappa B in human aortic endothelial cells conditioned to specific flow environments. Am J Physiol. 1997;273:C572-C578. PMID:9277354
- Nagel T, Resnick N, Dewey CF, Jr., Gimbrone MA, Jr.. Vascular endothelial cells respond to spatial gradients in fluid shear stress by enhanced activation of transcription factors. Arterioscler Thromb Vasc Biol. 1999;19:1825-1834. doi:10.1161/01.ATV.19.8.1825. PMID:10446060
- Sabine A, Agalarov Y, Maby-El HH, Jaquet M, Hagerling R, Pollmann C, Bebber D, Pfenniger A, Miura N, Dormond O, et al. Mechanotransduction, PROX1, and FOXC2 cooperate to control connexin37 and calcineurin during lymphatic-valve formation. Dev Cell. 2012;22:430-445. doi:10.1016/j.devcel.2011.12.020
- Nakajima H, Yamamoto K, Agarwala S, Terai K, Fukui H, Fukuhara S, Ando K, Miyazaki T, Yokota Y, Schmelzer E, et al. Flow-Dependent Endothelial YAP Regulation Contributes to Vessel Maintenance. Dev Cell. 2017;40:523-536. doi:10.1016/j.devcel.2017.02.019
- Wang KC, Yeh YT, Nguyen P, Limqueco E, Lopez J, Thorossian S, Guan KL, Li YJ, Chien S. Flow-dependent YAP/TAZ activities regulate endothelial phenotypes and atherosclerosis. Proc Natl Acad Sci U S A. 2016;113:11525-11530. doi:10.1073/pnas.1613121113. PMID:27671657
- Wang L, Luo JY, Li B, Tian XY, Chen LJ, Huang Y, Liu J, Deng D, Lau CW, Wan S, et al. Integrin-YAP/TAZ-JNK cascade mediates atheroprotective effect of unidirectional shear flow. Nature. 2016;540:579-582. doi:10.1038/nature20602.
- Anderson KP, Kern CB, Crable SC, Lingrel JB. Isolation of a gene encoding a functional zinc finger protein homologous to erythroid Kruppel-like factor: Identification of a new multigene family. Mol Cell Biol. 1995;15:5957-5965. doi:10.1128/MCB.15.11.5957. PMID:7565748
- Kuo CT, Veselits ML, Barton KP, Lu MM, Clendenin C, Leiden JM. The LKLF transcription factor is required for normal tunica media formation and blood vessel stabilization during murine embryogenesis. Genes Dev. 1997;11:2996-3006. doi:10.1101/gad.11.22.2996. PMID:9367982
- Wang L, Zhang P, Wei Y, Gao Y, Patient R, Liu F. A blood flow-dependent klf2a-NO signaling cascade is required for stabilization of hematopoietic stem cell programming in zebrafish embryos. Blood. 2011;118:4102-4110. doi:10.1182/blood-2011-05-353235. PMID:21849483
- Groenendijk BC, Hierck BP, Gittenberger-De Groot AC, Poelmann RE. Development-related changes in the expression of shear stress responsive genes KLF-2, ET-1, and NOS-3 in the developing cardiovascular system of chicken embryos. Dev Dyn. 2004;230:57-68. doi:10.1002/dvdy.20029. PMID:15108309
- Lee JS, Yu Q, Shin JT, Sebzda E, Bertozzi C, Chen M, Mericko P, Stadtfeld M, Zhou D, Cheng L, et al. Klf2 is an essential regulator of vascular hemodynamic forces in vivo. Dev Cell. 2006;11:845-857. doi:10.1016/j.devcel.2006.09.006
- Dekker RJ, van Thienen JV, Rohlena J, de Jager SC, Elderkamp YW, Seppen J, de Vries CJ, Biessen EA, van Berkel TJ, Pannekoek H, et al. Endothelial KLF2 links local arterial shear stress levels to the expression of vascular tone-regulating genes. Am J Pathol. 2005;167:609-618. doi:10.1016/S0002-9440(10)63002-7. PMID:16049344
- Abe J, Berk BC. Novel mechanisms of endothelial mechanotransduction. Arterioscler Thromb Vasc Biol. 2014;34:2378-2386. doi:10.1161/ATVBAHA.114.303428. PMID:25301843
- Doddaballapur A, Michalik KM, Manavski Y, Lucas T, Houtkooper RH, You X, Chen W, Zeiher AM, Potente M, Dimmeler S, et al. Laminar shear stress inhibits endothelial cell metabolism via KLF2-mediated repression of PFKFB3. Arterioscler Thromb Vasc Biol. 2015;35:137-145. doi:10.1161/ATVBAHA.114.304277
- Lin Z, Kumar A, SenBanerjee S, Staniszewski K, Parmar K, Vaughan DE, Gimbrone MA, Jr., Balasubramanian V, Garcia-Cardena G, Jain MK. Kruppel-like factor 2 (KLF2) regulates endothelial thrombotic function. Circ Res. 2005;96:e48-e57. doi:10.1161/01.RES.0000159707.05637.a1
- Lin Z, Natesan V, Shi H, Dong F, Kawanami D, Mahabeleshwar GH, Atkins GB, Nayak L, Cui Y, Finigan JH, et al. Kruppel-like factor 2 regulates endothelial barrier function. Arterioscler Thromb Vasc Biol. 2010;30:1952-1959. doi:10.1161/ATVBAHA.110.211474
- SenBanerjee S, Lin Z, Atkins GB, Greif DM, Rao RM, Kumar A, Feinberg MW, Chen Z, Simon DI, Luscinskas FW, et al. KLF2 Is a novel transcriptional regulator of endothelial proinflammatory activation. J Exp Med. 2004;199:1305-1315. doi:10.1084/jem.20031132. PMID:15136591
- Atkins GB, Wang Y, Mahabeleshwar GH, Shi H, Gao H, Kawanami D, Natesan V, Lin Z, Simon DI, Jain MK. Hemizygous deficiency of Kruppel-like factor 2 augments experimental atherosclerosis. Circ Res. 2008;103:690-693. doi:10.1161/CIRCRESAHA.108.184663. PMID:18757824
- Hamik A, Lin Z, Kumar A, Balcells M, Sinha S, Katz J, Feinberg MW, Gerzsten RE, Edelman ER, Jain MK. Kruppel-like factor 4 regulates endothelial inflammation. J Biol Chem. 2007;282:13769-13779. doi:10.1074/jbc.M700078200. PMID:17339326
- Zhou G, Hamik A, Nayak L, Tian H, Shi H, Lu Y, Sharma N, Liao X, Hale A, Boerboom L, et al. Endothelial Kruppel-like factor 4 protects against atherothrombosis in mice. J Clin Invest. 2012;122:4727-4731. doi:10.1172/JCI66056. PMID:23160196
- Nicoli S, Standley C, Walker P, Hurlstone A, Fogarty KE, Lawson ND. MicroRNA-mediated integration of haemodynamics and Vegf signalling during angiogenesis. Nature. 2010;464:1196-1200. doi:10.1038/nature08889. PMID:20364122
- Renz M, Otten C, Faurobert E, Rudolph F, Zhu Y, Boulday G, Duchene J, Mickoleit M, Dietrich AC, Ramspacher C, et al. Regulation of beta1 integrin-Klf2-mediated angiogenesis by CCM proteins. Dev Cell. 2015;32:181-190. doi:10.1016/j.devcel.2014.12.016
- Kwon HB, Wang S, Helker CS, Rasouli SJ, Maischein HM, Offermanns S, Herzog W, Stainier DY. In vivo modulation of endothelial polarization by Apelin receptor signalling. Nat Commun. 2016;7:11805. doi:10.1038/ncomms11805
- Wu J, Bohanan CS, Neumann JC, Lingrel JB. KLF2 transcription factor modulates blood vessel maturation through smooth muscle cell migration. J Biol Chem. 2008;283:3942-3950. doi:10.1074/jbc.M707882200. PMID:18063572
- Hergenreider E, Heydt S, Treguer K, Boettger T, Horrevoets AJ, Zeiher AM, Scheffer MP, Frangakis AS, Yin X, Mayr M, et al. Atheroprotective communication between endothelial cells and smooth muscle cells through miRNAs. Nat Cell Biol. 2012;14:249-256. doi:10.1038/ncb2441. PMID:22327366
- Heckel E, Boselli F, Roth S, Krudewig A, Belting HG, Charvin G, Vermot J. Oscillatory Flow Modulates Mechanosensitive klf2a Expression through trpv4 and trpp2 during Heart Valve Development. Curr Biol. 2015;25:1354-1361. doi:10.1016/j.cub.2015.03.038
- Vermot J, Forouhar AS, Liebling M, Wu D, Plummer D, Gharib M, Fraser SE. Reversing blood flows act through klf2a to ensure normal valvulogenesis in the developing heart. PLoS Biol. 2009;7:e1000246. doi:10.1371/journal.pbio.1000246. PMID:19924233
- Steed E, Faggianelli N, Roth S, Ramspacher C, Concordet JP, Vermot J. klf2a couples mechanotransduction and zebrafish valve morphogenesis through fibronectin synthesis. Nat Commun. 2016;7:11646. doi:10.1038/ncomms11646. PMID:27221222
- Hayden MS, Ghosh S. Shared principles in NF-kappaB signaling. Cell. 2008;132:344-362. doi:10.1016/j.cell.2008.01.020
- Read MA, Whitley MZ, Williams AJ, Collins T. NF-kappa B and I kappa B alpha: An inducible regulatory system in endothelial activation. J Exp Med. 1994;179:503-512. doi:10.1084/jem.179.2.503. PMID:7507507
- Karin M. The beginning of the end: IkappaB kinase (IKK) and NF-kappaB activation. J Biol Chem. 1999;274:27339-27342. doi:10.1074/jbc.274.39.27339. PMID:10488062
- Wang C, Baker BM, Chen CS, Schwartz MA. Endothelial cell sensing of flow direction. Arterioscler Thromb Vasc Biol. 2013;33:2130-2136. doi:10.1161/ATVBAHA.113.301826. PMID:23814115
- Feaver RE, Gelfand BD, Blackman BR. Human haemodynamic frequency harmonics regulate the inflammatory phenotype of vascular endothelial cells. Nat Commun. 2013;4:1525. doi:10.1038/ncomms2530. PMID:23443553
- Bhullar IS, Li YS, Miao H, Zandi E, Kim M, Shyy JY, Chien S. Fluid shear stress activation of IkappaB kinase is integrin-dependent. J Biol Chem. 1998;273:30544-30549. doi:10.1074/jbc.273.46.30544. PMID:9804824
- Collins T, Read MA, Neish AS, Whitley MZ, Thanos D, Maniatis T. Transcriptional regulation of endothelial cell adhesion molecules: NF-kappa B and cytokine-inducible enhancers. FASEB J. 1995;9:899-909. PMID:7542214
- Khachigian LM, Resnick N, Gimbrone MA, Jr., Collins T. Nuclear factor-kappa B interacts functionally with the platelet-derived growth factor B-chain shear-stress response element in vascular endothelial cells exposed to fluid shear stress. J Clin Invest. 1995;96:1169-1175. doi:10.1172/JCI118106. PMID:7635955
- Huo Y, Ley K. Adhesion molecules and atherogenesis. Acta Physiol Scand. 2001;173:35-43.doi:10.1046/j.1365-201X.2001.00882.x. PMID:11678724
- Iiyama K, Hajra L, Iiyama M, Li H, DiChiara M, Medoff BD, Cybulsky MI. Patterns of vascular cell adhesion molecule-1 and intercellular adhesion molecule-1 expression in rabbit and mouse atherosclerotic lesions and at sites predisposed to lesion formation. Circ Res. 1999;85:199-207. doi:10.1161/01.RES.85.2.199. PMID:10417402
- Brand K, Page S, Rogler G, Bartsch A, Brandl R, Knuechel R, Page M, Kaltschmidt C, Baeuerle PA, Neumeier D. Activated transcription factor nuclear factor-kappa B is present in the atherosclerotic lesion. J Clin Invest. 1996;97:1715-1722. doi:10.1172/JCI118598. PMID:8601637
- Cuhlmann S, Van der Heiden K, Saliba D, Tremoleda JL, Khalil M, Zakkar M, Chaudhury H, Luong lA, Mason JC, Udalova I, et al. Disturbed blood flow induces RelA expression via c-Jun N-terminal kinase 1: A novel mode of NF-kappaB regulation that promotes arterial inflammation. Circ Res. 2011;108:950-959. doi:10.1161/CIRCRESAHA.110.233841. PMID:21350211
- Passerini AG, Polacek DC, Shi C, Francesco NM, Manduchi E, Grant GR, Pritchard WF, Powell S, Chang GY, Stoeckert CJ, Jr., et al. Coexisting proinflammatory and antioxidative endothelial transcription profiles in a disturbed flow region of the adult porcine aorta. Proc Natl Acad Sci U S A. 2004;101:2482-2487. doi:10.1073/pnas.0305938101. PMID:14983035
- Orr AW, Sanders JM, Bevard M, Coleman E, Sarembock IJ, Schwartz MA. The subendothelial extracellular matrix modulates NF-kappaB activation by flow: A potential role in atherosclerosis. J Cell Biol. 2005;169:191-202. doi:10.1083/jcb.200410073. PMID:15809308
- Shekhonin BV, Domogatsky SP, Idelson GL, Koteliansky VE, Rukosuev VS. Relative distribution of fibronectin and type I, III, IV, V collagens in normal and atherosclerotic intima of human arteries. Atherosclerosis. 1987;67:9-16. doi:10.1016/0021-9150(87)90259-0. PMID:3314885
- Feaver RE, Gelfand BD, Wang C, Schwartz MA, Blackman BR. Atheroprone hemodynamics regulate fibronectin deposition to create positive feedback that sustains endothelial inflammation. Circ Res. 2010;106:1703-1711. doi:10.1161/CIRCRESAHA.109.216283
- Jalali S, del Pozo MA, Chen K, Miao H, Li Y, Schwartz MA, Shyy JY, Chien S. Integrin-mediated mechanotransduction requires its dynamic interaction with specific extracellular matrix (ECM) ligands. Proc Natl Acad Sci U S A. 2001;98:1042-1046. doi:10.1073/pnas.031562998. PMID:11158591
- Orr AW, Ginsberg MH, Shattil SJ, Deckmyn H, Schwartz MA. Matrix-specific suppression of integrin activation in shear stress signaling. Mol Biol Cell. 2006;17:4686-4697. doi:10.1091/mbc.E06-04-0289. PMID:16928957
- Tzima E, del Pozo MA, Shattil SJ, Chien S, Schwartz MA. Activation of integrins in endothelial cells by fluid shear stress mediates Rho-dependent cytoskeletal alignment. EMBO J. 2001;20:4639-4647. doi:10.1093/emboj/20.17.4639
- Chen J, Green J, Yurdagul A, Jr., Albert P, McInnis MC, Orr AW. alphavbeta3 Integrins Mediate Flow-Induced NF-kappaB Activation, Proinflammatory Gene Expression, and Early Atherogenic Inflammation. Am J Pathol. 2015;185:2575-2589. doi:10.1016/j.ajpath.2015.05.013
- Petzold T, Orr AW, Hahn C, Jhaveri KA, Parsons JT, Schwartz MA. Focal adhesion kinase modulates activation of NF-kappaB by flow in endothelial cells. Am J Physiol Cell Physiol. 2009;297:C814-C822. doi:10.1152/ajpcell.00226.2009. PMID:19587216
- Sainson RC, Johnston DA, Chu HC, Holderfield MT, Nakatsu MN, Crampton SP, Davis J, Conn E, Hughes CC. TNF primes endothelial cells for angiogenic sprouting by inducing a tip cell phenotype. Blood. 2008;111:4997-5007. doi:10.1182/blood-2007-08-108597. PMID:18337563
- Shono T, Ono M, Izumi H, Jimi SI, Matsushima K, Okamoto T, Kohno K, Kuwano M. Involvement of the transcription factor NF-kappaB in tubular morphogenesis of human microvascular endothelial cells by oxidative stress. Mol Cell Biol. 1996;16:4231-4239. doi:10.1128/MCB.16.8.4231. PMID:8754823
- Yoshida A, Yoshida S, Ishibashi T, Kuwano M, Inomata H. Suppression of retinal neovascularization by the NF-kappaB inhibitor pyrrolidine dithiocarbamate in mice. Invest Ophthalmol Vis Sci. 1999;40:1624-1629. PMID:10359349
- Santoro MM, Samuel T, Mitchell T, Reed JC, Stainier DY. Birc2 (cIap1) regulates endothelial cell integrity and blood vessel homeostasis. Nat Genet. 2007;39:1397-1402. doi:10.1038/ng.2007.8. PMID:17934460
- Yu FX, Guan KL. The Hippo pathway: Regulators and regulations. Genes Dev. 2013;27:355-371. doi:10.1101/gad.210773.112
- Piccolo S, Dupont S, Cordenonsi M. The biology of YAP/TAZ: Hippo signaling and beyond. Physiol Rev. 2014;94:1287-1312. doi:10.1152/physrev.00005.2014. PMID:25287865
- Bertini E, Oka T, Sudol M, Strano S, Blandino: YAP: at the Crossroad Between Transformation and Tumor Suppression. Cell Cycle. 2009;8:49-57. doi:10.4161/cc.8.1.7259
- Mauviel A, Nallet-Staub F, Varelas X. Integrating developmental signals: A Hippo in the (path)way. Oncogene. 2012;31:1743-1756. doi:10.1038/onc.2011.363. PMID:21874053
- Harvey K, Tapon N: The Salvador-Warts-Hippo pathway – an emerging tumour-suppressor network. Nat Rev Cancer. 2007;7:182-191. doi:10.1038/nrc2070. PMID:17318211
- Avruch J, Zhou D, Fitamant J, Bardeesy N, Mou F, Barrufet LR. Protein kinases of the Hippo pathway: Regulation and substrates. Semin Cell Dev Biol. 2012;23:770-784. doi:10.1016/j.semcdb.2012.07.002
- Aragona M, Panciera T, Manfrin A, Giulitti S, Michielin F, Elvassore N, Dupont S, Piccolo S. A mechanical checkpoint controls multicellular growth through YAP/TAZ regulation by actin-processing factors. Cell. 2013;154:1047-1059. doi:10.1016/j.cell.2013.07.042
- Calvo F, Ege N, Grande-Garcia A, Hooper S, Jenkins RP, Chaudhry SI, Harrington K, Williamson P, Moeendarbary E, Charras G, et al. Mechanotransduction and YAP-dependent matrix remodelling is required for the generation and maintenance of cancer-associated fibroblasts. Nat Cell Biol. 2013;15:637-646. doi:10.1038/ncb2756. PMID:23708000
- Dupont S, Morsut L, Aragona M, Enzo E, Giulitti S, Cordenonsi M, Zanconato F, Le Diqabel J, Forcato M, Bicciato S, et al. Role of YAP/TAZ in mechanotransduction. Nature. 2011;474:179-183. doi:10.1038/nature10137. PMID:21654799
- Feng X, Degese MS, Iglesias-Bartolome R, Vaque JP, Molinolo AA, Rodrigues M, Zaidi MR, Ksander BR, Merlino G, Sodhi A, et al. Hippo-independent activation of YAP by the GNAQ uveal melanoma oncogene through a trio-regulated rho GTPase signaling circuitry. Cancer Cell. 2014;25:831-845. doi:10.1016/j.ccr.2014.04.016
- Benham-Pyle BW, Pruitt BL, Nelson WJ. Cell adhesion. Mechanical strain induces E-cadherin-dependent Yap1 and beta-catenin activation to drive cell cycle entry. Science 2015;348:1024-1027. doi:10.1126/science.aaa4559
- Codelia VA, Sun G, Irvine KD. Regulation of YAP by mechanical strain through Jnk and Hippo signaling. Curr Biol. 2014;24:2012-2017. doi:10.1016/j.cub.2014.07.034
- Cui Y, Hameed FM, Yang B, Lee K, Pan CQ, Park S, Sheetz M. Cyclic stretching of soft substrates induces spreading and growth. Nat Commun. 2015;6:6333. doi:10.1038/ncomms7333. PMID:25704457
- Wada K, Itoga K, Okano T, Yonemura S, Sasaki H. Hippo pathway regulation by cell morphology and stress fibers. Development. 2011;138:3907-3914. doi:10.1242/dev.070987. PMID:21831922
- Sabine A, Bovay E, Demir CS, Kimura W, Jaquet M, Agalarov Y, Zangger N, Scallan JP, Graber W, Gulpinar E, et al. FOXC2 and fluid shear stress stabilize postnatal lymphatic vasculature. J Clin Invest. 2015;125:3861-3877. doi:10.1172/JCI80454. PMID:26389677
- Birukov KG, Birukova AA, Dudek SM, Verin AD, Crow MT, Zhan X, DePaola N, Garcia JG. Shear stress-mediated cytoskeletal remodeling and cortactin translocation in pulmonary endothelial cells. Am J Respir Cell Mol Biol. 2002;26:453-464. doi:10.1165/ajrcmb.26.4.4725
- Chan SW, Lim CJ, Chong YF, Pobbati AV, Huang C, Hong W. Hippo pathway-independent restriction of TAZ and YAP by angiomotin. J Biol Chem. 2011;286:7018-7026. doi:10.1074/jbc.C110.212621. PMID:21224387
- Mana-Capelli S, Paramasivam M, Dutta S, McCollum D. Angiomotins link F-actin architecture to Hippo pathway signaling. Mol Biol Cell. 2014;25:1676-1685. doi:10.1091/mbc.E13-11-0701. PMID:24648494
- Zhao B, Tumaneng K, Guan KL. The Hippo pathway in organ size control, tissue regeneration and stem cell self-renewal. Nat Cell Biol. 2011;13:877-883. doi:10.1038/ncb2303. PMID:21808241
- Fukui H, Terai K, Nakajima H, Chiba A, Fukuhara S, Mochizuki N. S1P-Yap1 signaling regulates endoderm formation required for cardiac precursor cell migration in zebrafish. Dev Cell. 2014;31:128-136. doi:10.1016/j.devcel.2014.08.014
- Uemura M, Nagasawa A, Terai K. Yap/Taz transcriptional activity in endothelial cells promotes intramembranous ossification via the BMP pathway. Sci Rep. 2016;6:27473. doi:10.1038/srep27473. PMID:27273480
- Oemar BS, Werner A, Garnier JM, Do DD, Godoy N, Nauck M, Marz W, Rupp J, Pech M, Luscher TF. Human connective tissue growth factor is expressed in advanced atherosclerotic lesions. Circulation. 1997;95:831-839. doi:10.1161/01.CIR.95.4.831. PMID:9054739
- Cicha I, Yilmaz A, Klein M, Raithel D, Brigstock DR, Daniel WG, Goppelt-Struebe M, Garlichs CD. Connective tissue growth factor is overexpressed in complicated atherosclerotic plaques and induces mononuclear cell chemotaxis in vitro. Arterioscler Thromb Vasc Biol. 2005;25:1008-1013. doi:10.1161/01.ATV.0000162173.27682.7b
- Chaqour B, Goppelt-Struebe M. Mechanical regulation of the Cyr61/CCN1 and CTGF/CCN2 proteins. FEBS J. 2006;273:3639-3649. doi:10.1111/j.1742-4658.2006.05360.x. PMID:16856934
- Choi HJ, Zhang H, Park H, Choi KS, Lee HW, Agrawal V, Kim YM, Kwon YG. Yes-associated protein regulates endothelial cell contact-mediated expression of angiopoietin-2. Nat Commun. 2015;6:6943. doi:10.1038/ncomms7943. PMID:25962877
- Atkins GB, Simon DI. Interplay between NF-kappaB and Kruppel-like factors in vascular inflammation and atherosclerosis: location, location, location. J Am Heart Assoc. 2013;2:e000290. doi:10.1161/JAHA.113.000290
- Kumar A, Lin Z, SenBanerjee S, Jain MK. Tumor necrosis factor alpha-mediated reduction of KLF2 is due to inhibition of MEF2 by NF-kappaB and histone deacetylases. Mol Cell Biol. 2005;25:5893-5903. doi:10.1128/MCB.25.14.5893-5903.2005
- Xu Z, Yoshida T, Wu L, Maiti D, Cebotaru L, Duh EJ. Transcription factor MEF2C suppresses endothelial cell inflammation via regulation of NF-kappaB and KLF2. J Cell Physiol. 2015;230:1310-1320. doi:10.1002/jcp.24870. PMID:25474999
- Fledderus JO, van Thienen JV, Boon RA, Dekker RJ, Rohlena J, Volger OL, Bijnens AP, Daemen MJ, Kuiper J, van Berkel TJ, et al. Prolonged shear stress and KLF2 suppress constitutive proinflammatory transcription through inhibition of ATF2. Blood. 2007;109:4249-4257. doi:10.1182/blood-2006-07-036020. PMID:17244683
- Chu TJ, Peters DG. Serial analysis of the vascular endothelial transcriptome under static and shear stress conditions. Physiol Genomics. 2008;34:185-192. doi:10.1152/physiolgenomics.90201.2008. PMID:18505769
- Kumar S, Kim CW, Son DJ, Ni CW, Jo H. Flow-dependent regulation of genome-wide mRNA and microRNA expression in endothelial cells in vivo. Sci Data. 2014;1:140039. doi:10.1038/sdata.2014.39. PMID:25977794
- McCormick SM, Eskin SG, McIntire LV, Teng CL, Lu CM, Russell CG, Chittur KK. DNA microarray reveals changes in gene expression of shear stressed human umbilical vein endothelial cells. Proc Natl Acad Sci U S A. 2001;98:8955-8960. doi:10.1073/pnas.171259298. PMID:11481467
- Ohura N, Yamamoto K, Ichioka S, Sokabe T, Nakatsuka H, Baba A, Shibata M, Nakatsuka T, Harii K, Wada Y, et al. Global analysis of shear stress-responsive genes in vascular endothelial cells. J Atheroscler Thromb. 2003;10:304-313. doi:10.5551/jat.10.304. PMID:14718748
- Ni CW, Qiu H, Rezvan A, Kwon K, Nam D, Son DJ, Visvader JE, Jo H. Discovery of novel mechanosensitive genes in vivo using mouse carotid artery endothelium exposed to disturbed flow. Blood. 2010;116:e66-e73. doi:10.1182/blood-2010-04-278192. PMID:20551377
- Son DJ, Kumar S, Takabe W, Kim CW, Ni CW, Alberts-Grill N, Jang IH, Kim S, Kim W, Won KS, et al. The atypical mechanosensitive microRNA-712 derived from pre-ribosomal RNA induces endothelial inflammation and atherosclerosis. Nat Commun. 2013;4:3000. doi:10.1038/ncomms4000. PMID:24346612
- Espin-Palazon R, Stachura DL, Campbell CA, Garcia-Moreno D, Del Cid N, Kim AD, Candel S, Meseguer J, Mulero V, Traver D. Proinflammatory signaling regulates hematopoietic stem cell emergence. Cell. 2014;159:1070-1085. doi:10.1016/j.cell.2014.10.031
- He Q, Zhang C, Wang L, Zhang P, Ma D, Lv J, Liu F. Inflammatory signaling regulates hematopoietic stem and progenitor cell emergence in vertebrates. Blood. 2015;125:1098-1106. doi:10.1182/blood-2014-09-601542. PMID:25540193