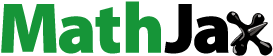
ABSTRACT
The transitions between phases of the cell cycle have evolved to be robust and switch-like, which ensures temporal separation of DNA replication, sister chromatid separation, and cell division. Mathematical models describing the biochemical interaction networks of cell cycle regulators attribute these properties to underlying bistable switches, which inherently generate robust, switch-like, and irreversible transitions between states. We have recently presented new mathematical models for two control systems that regulate crucial transitions in the cell cycle: mitotic entry and exit,Citation1 and the mitotic checkpoint.Citation2 Each of the two control systems is characterized by two interlinked bistable switches. In the case of mitotic checkpoint control, these switches are mutually activating, whereas in the case of the mitotic entry/exit network, the switches are mutually inhibiting. In this Perspective we describe the qualitative features of these regulatory motifs and show that having two interlinked bistable mechanisms further enhances robustness and irreversibility. We speculate that these network motifs also underlie other cell cycle transitions and cellular transitions between distinct biochemical states.
Introduction
During mitosis, cells undergo dramatic architectural changes that facilitate the correct division of the genetic material among the nascent daughter cells. The chromatin condenses, the cell rounds up, the nuclear envelope breaks down and the mitotic spindle assembles. Once all the chromosomes are aligned on the metaphase plate, the sister chromatids separate and move to opposite poles of the cell, and the opening steps are reversed as the cell divides in two.Citation3 Precise control of the activation and inactivation of agents that drive these processes is necessary for cells to successfully complete mitosis.
In light of the complexity of mitosis, it may seem surprising that ordering of events can be understood in terms of relatively simple feedback regulation. The canonical regulatory ensemble consists of cyclin B:Cdk1 and a handful of other regulators such as the Polo and Aurora kinases, and the PPP-family phosphatases (such as protein phosphatase 2A).Citation3 The focus of this Perspective is presented in . Cyclin B:Cdk1 and PP2A-B55 (B55) act on downstream targets (S) and influence each other's activity by engaging in feedback regulation. The sister chromatid attachment state to the mitotic spindle, controlled by an error correction (EC) mechanism, is also involved in feedback regulation with Cyclin B:Cdk1. These interactions give rise to a characteristic profile of specific phosphorylation and dephosphorylation activity throughout mitosis (, time courses).
Figure 1. Bistable modules of mitotic progression. (A, left) Influence diagram of the main players involved in mitotic entry and exit and the mitotic checkpoint. Cdk1 phosphorylates substrates, promoting mitosis, and B55 dephosphorylates substrates, promoting mitotic exit. B55 and Cdk1 also (indirectly) mutually inhibit each other's activity. Once in mitosis, Cdk1 activity is maintained with the help of the error correction (EC) module, which removes incorrect kinetochore-microtubule attachments. Cdk1 also promotes error correction through activation and localisation of error correction proteins (see text for more details). Abbreviations: EC, error correction; B55, PP2A-B55; Cdk1, cyclin-dependent kinase 1; S, mitotic substrate. (A, right) Time course depicting the activity of the main players and cell cytology during mitosis. Cdk1 activity promotes mitotic entry and inhibits PP2A-B55. The nuclear envelope breaks down and chromosomes condense, and the mitotic spindle forms. Attachments between the mitotic spindle and chromosomes form stochastically, and error correction activity removes incorrect attachments until all chromosomes are bi-oriented. Chromosomes align along the equator of the cell at metaphase. The anaphase-promoting complex/cyclosome is liberated from its inhibition and targets securin and cyclin B for degradation, which induces sister chromatid separation in anaphase. Cdk1 activity falls, and PP2A-B55 becomes reactivated. The nuclear envelope reforms around the segregated genetic material in telophase, and division is completed by separation of the cytoplasm during cytokinesis. From there, G1 phase is established with de-condensed genetic material, high PP2A-B55 activity, and low Cdk1 activity. (B) Influence diagram of mitotic entry and exit. Cdk1 promotes its own activity due to the positive feedback with Cdc25 and double-negative feedback with Wee1. B55 promotes its own activity due to the double-negative feedback with Gwl. Cdk1 inhibits B55 self-promotion by phosphorylating Gwl; B55 inhibits Cdk1 self-promotion by dephosphorylating Wee1 and Cdc25. (C) Influence diagram of the mitotic checkpoint. The error correction module self-promotes, with Aurora B promoting unattached kinetochores, and unattached kinetochores promoting Aurora B through decreased kinetochore tension. The spindle assembly checkpoint, with output here of Cdk1 activity, self-promotes, with Cdk1 promoting MCC formation, and MCC promoting Cdk1 activity by inhibiting Cyclin B degradation through inhibition of the APC/C. Error correction promotes Cdk1 activity by generating unattached kinetochores that produce MCC, and Cdk1 promotes error correction by localising Aurora B to centromeres. (D) Phase plane of mitotic entry and exit. The B55 balance curve (red) shows the values of B55 where for a given Cdk1 value, and vice versa for the Cdk1 balance curve (purple). There are three intersections of the balance curves, which are steady states of the system; two are stable (solid circles), one is unstable (open circle). When Cdk1 activity is high, B55 activity is low and vice versa. (E) Phase plane of the mitotic checkpoint. The Cdk1 balance curve (purple) shows the values of Cdk1 where
for a given error correction activity, and vice versa for the error correction activity (blue). The three intersections of the balance curves depict steady states of the system, two of which are stable (solid circles) and one unstable (open circle). Either both Cdk1 and error correction have high activity or that both have low activity. The equations and parameters used for the phase planes can be found online at https://github.com/novakgroupoxford/2017_Hutter_et_al.
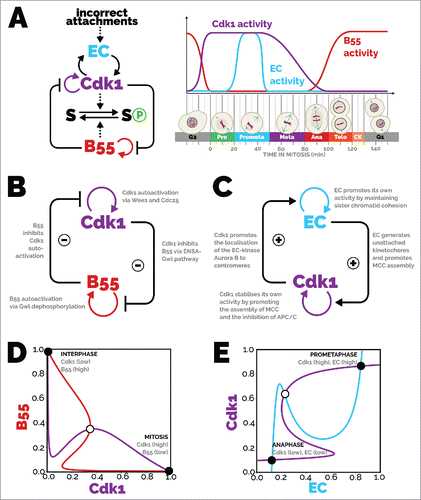
Beyond this familiar set of regulatory enzymes, regulatory motifs present another recurring theme. Self-promotion via positive feedback cycles is common among virtually all regulators of mitosis.Citation4 The mechanistic basis for this kind of self-reinforcement can include: autophosphorylation, the inhibition of an inhibitor (double-negative feedback), and the activation of an activator (positive feedback). These feedback mechanisms form the basis of biochemical switches that oversee complex decision making problems during mitosis and ensure correct ordering of events.
Here, we focus on two recent advancesCitation1,Citation2 in our understanding of the control of mitosis that generate desirable switch-like transitions. Firstly, we study mitotic entry and exit, and secondly we look at the mitotic checkpoint.
Primer Figure: Signal-response diagram showing steady state signal for a given response. (A) A simple hyperbole represents a graded response, which plateaus as the signal is increased. There is no clear threshold between low and high responses. (B) Ultrasensitive signal-response. The steady state response is sigmoidal, with a clear signal threshold at which the response goes from low to high. Starting at high signal and high response and lowering the signal causes the response to go from high to low at the same threshold. (C) Bistable signal-response. This is fundamentally different to the other signal response diagrams. There are two stable steady state response regimes, corresponding to low and high responses. The transition between low and high response occurs at a particular activation threshold of the signal. Transitioning from high response to low response occurs at a lower inactivation threshold of the signal. For signal values between the inactivation and activation thresholds, there exist two stable state response values; the state of the system depends on its history. The stable steady states are separated by an unstable steady state, which can be thought of as a maintain ridge between two valleys (D). If a ball is dropped on the mountain it will end-up in either of the valleys (stable steady states), which one depending on which side of the ridge (unstable steady state) it started.
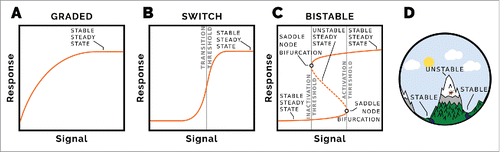
Dynamical systems primer
Mathematical models are powerful tools to complement biological experiments. One way to build a mathematical model describing a biochemical system is to employ nonlinear ordinary differential equations, which describe the time rate of change of each component. A typical equation describing the rate of change of the activity of an enzyme expresses its synthesis, degradation, activation, and inactivation. These are given by their respective expressions
,
,
, and
, where the ‘k's are rate constants (parameters), yielding the equation
. Generally, systems consisting of several such equations are solved numerically based on a set of initial conditions and parameter values, and the output can be compared to experimental data, with dynamic variables plotted against time.
In addition to looking at how a variable changes in time, we can plot the steady state values of the variable (response) as a function of one of the system's parameters (signal). Along the balance curve of a signal-response diagram, the production and consumption of the component are balanced with each other. Signal response diagrams can be used to assess the qualitative nature of the steady states as the value of a parameter changes as shown by a few examples in the Box panels. Panel A shows a graded, hyperbolic, response as the signal is increased, with the response reaching a plateau.
Panel B shows an ultrasensitive signal-response curve. This represents a biochemical switch with a distinct threshold at which a state transition occurs. But the switch is reversible. Going from the low to the high response occurs at the same signal threshold as going from the high to the low response.
Panel C depicts a bistable signal-response curve, which is fundamentally different to the other balance curves. Starting from a low response at steady state and increasing the signal, there is an activation threshold where the low response steady state ceases to exist and the system ‘jumps’ to the high response steady state. Now starting at the high response and lowering the signal, the high response steady state ceases to exist at an inactivation threshold. At this point the system ‘jumps’ to the low response steady state. Going from the high response to the low response happens at a lower signal strength to the activation threshold. It is this hysteresis that imparts robustness and can render the transition irreversible.
As well as stable steady states, a bistable system has an unstable steady state at signal levels between the inactivation and activation thresholds, depicted by the dashed line in Panel C. This can be thought of as a mountain ridge (unstable steady state) between two valleys (stable steady states), as shown in Panel D. Between the inactivation and activation signal thresholds, the system can be in either of the two stable steady states. Which stable state is occupied depends on the history of the system and whether it started above or below the dashed line.
Signal-response curves are useful for observing the output of the whole system, but we can use what is known as a phase plane to assess how two components interact with each other to give a system response. Two signal-response diagrams are plotted in the same coordinate system by changing over the role of the signal and the response. After plotting the first balance curve, the variable on the y-axis is used as a signal for the response being on the x-axis. Two examples are shown in and . Where these two curves intersect, both dynamic variables comprising the system are in steady state, and so these are steady states of the system. For a bistable system there are three intersection points, two representing stable steady states and one unstable steady state.
Mitotic entry and exit
Entry into mitosis requires the phosphorylation of hundreds of substrates by cyclin-dependent kinase 1 (Cdk1) in complex with its regulatory subunit, cyclin B.Citation5 Cyclin B:Cdk1 must overcome the activity of its counteracting phosphatase, protein phosphatase 2A (PP2A), in complex with its regulatory B55 subunit.Citation6 The ratio of the activities of Cdk1 and PP2A-B55 determines the level of their substrates’ phosphorylation, and both contribute to ordered cell-cycle substrate phosphorylation.Citation7,Citation8
As their names suggest, the level of cyclins fluctuate, or cycle, with the cell cycle.Citation9 Beyond the regulation of the rate-limiting cyclin B subunit level, the activity of cyclin B:Cdk1 is further regulated through phosphorylation. When in complex with cyclin B, Cdk1 can be phosphorylated on Tyr15 by Wee1 kinaseCitation10, and on both Thr14 and Tyr15 by Myt1 kinaseCitation11 (we refer only to Wee1 for brevity). These phosphorylations inhibit binding of substrates to Cdk1, and thereby inhibit its activity.Citation12 Crucially, Wee1 is phosphorylated and thereby inactivated by cyclin B:Cdk1, which creates a double negative feedback loop.Citation13 The phosphatase that removes the inhibitory phosphorylations on Cdk1 is Cdc25.Citation14 Like Wee1, Cdc25 is subject to phosphorylation by cyclin B:Cdk1, however this activates the Cdc25 phosphatase, resulting in a positive feedback loop.Citation15 In 1993, Novak and Tyson constructed a mathematical model of these two feedback loopsCitation16 ( and
), depicted as the self-promoting loop on cyclin B:Cdk1 of , and predicted a bistable cyclin B:Cdk1 activity with respect to total cyclin B level, which was confirmed experimentally a decade later.Citation17,Citation18 This makes the mitotic state robust, as once a cell has committed to mitotic entry it will not revert until the cyclin B level has dropped three-fold below that which caused entry. PP2A-B55 dephosphorylates the cyclin B:Cdk1 phosphorylation sites of Wee1 and Cdc25,Citation19 so steady state Cdk1 activity can be plotted as a function of PP2A-B55 activity (purple balance curve of ). This shows that for certain values of input (PP2A-B55) two possible steady states of Cdk1 activity can coexist.
At the time when the Novak and TysonCitation16 model was constructed, the regulation of the cyclin B:Cdk1 counter-acting phosphatase was unknown, and so was modelled as a constitutive activity (shown as a horizontal line in the PP2A-B55 balance curve of ). In their model interphase and mitosis are different in terms of Cdk1 activity only. Recently, the attention of the field has turned to the regulation of PP2A-B55, a major Cdk1-counteracting phosphatase.Citation20 PP2A-B55 was found to be inhibited in M phase and active during interphase, and the inhibition required the phosphorylated form of ENSA and/or ARPP19.Citation21,Citation22 These two small unstructured peptides are phosphorylated by Gwl kinase, which is itself phosphosphorylated by cyclin B:Cdk1.Citation23 S67-phosphorylated ENSA binds to PP2A-B55 with high affinity and is, crucially, a substrate of PP2A-B55 but with a relatively low (two to three orders of magnitude lower) rate of catalysis.Citation24 This so-called ‘unfair competition’ mechanismCitation24 results in a PP2A-B55 activity that is high in interphase – when little phosphorylated ENSA is present – and low during mitosis, when the Gwl kinase is active and continuously replenishing the pool of phosphorylated ENSACitation21, as shown in – the right inhibitory arm. In this way, Gwl promotes the mitotic state alongside the activation of cyclin B:Cdk1.Citation25,Citation26 Inhibition of PP2A-B55 in mitosis also helps to prevent futile cycling of Cdk1 substrates’ phosphorylation/dephosphorylations and for a higher degree of substrate phosphorylation to be achieved. Whereas the Novak and Tyson model relied solely on kinase activity changing, now both kinase and phosphatase activities change to influence the kinase/phosphatase ratio (as shown in ), and the effects of this are discussed further below.
Figure 2. Phase plane of PP2A-B55 activity against cyclin B:Cdk1 activity for three different model scenarios. The effects of different levels of cyclin B are shown using logarithmic scales. The transition of interphase to mitosis occurs when the three intersection points cease to exist, which occurs when the unstable steady state and the stable steady state corresponding to interphase come together. Similarly for the mitosis to interphase transition, when the stable steady state corresponding to mitosis comes together with the unstable steady state, lowering the cyclin B total further results in only one stable state, corresponding to interphase, existing. The PP2A-B55 balance curve is a horizontal straight line in the Novak-Tyson model. When PP2A-B55 is inhibited by cyclin B:Cdk1 (B), the Cdk1 activity balance curves are the same as in A, but now the PP2A-B55 balance curve is hyperbolic. The difference between the thresholds of cyclin B total when mitotic entry and exit occur is greater than for A. In the case of double-negative feedback between PP2A-B55 and Gwl (C), the PP2A-B55 balance curve is Z-shaped, which shows that the regulation of cyclin B:Cdk1 can be removed and the system would still be bistable. The difference between the thresholds of cyclin B total when mitotic entry and exit occur is greater than for B, which shows greater distinction between interphase and mitosis. The equations and parameters used for the phase planes can be found online at https://github.com/novakgroupoxford/2017_Hutter_et_al.
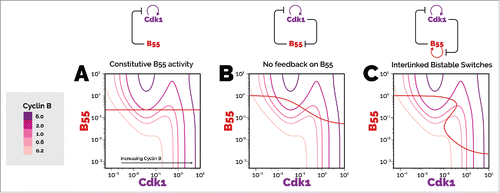
Exit from mitosis, which is triggered by the activation of the anaphase-promoting complex/cyclosome (APC/C) under the control of the mitotic checkpoint (as we will discuss below), requires not only the degradation of cyclin B and securin, but also the reactivation of PP2A-B55 by the timely inactivation of the Greatwall-ENSA pathway.Citation27 This poses the question: what is the phosphatase acting on cyclin B:Cdk1 sites of Gwl? Our group predicted one of these to be PP2A-B55 itself. This would give rise to another feedback loop (), and support an additional bistable switch – that of PP2A-B55 activity with respect to cyclin B:Cdk1 activity.Citation28 The double negative feedback between PP2A-B55 and Gwl in this scenario imparts an auto-activating feature whereby PP2A-B55 inhibits its own inhibitor. This is shown as the loop of B55 promoting itself in , and it was recently demonstrated to be the case with the use of biochemical reconstitution and Xenopus egg extract.Citation1 It also poses a bootstrapping problem: how does PP2A-B55 inactivate Gwl if PP2A-B55 itself is inhibited? The solution is that PP1 kick-starts Gwl dephosphorylation, and then PP2A-B55 finishes the job.Citation29,Citation30 A representative balance curve (red) of PP2A-B55 activity is shown in the phase plane in . For certain values of input (cyclin B:Cdk1) to the positive feedback, there exist two possible stable steady states of PP2A-B55, mirroring the corresponding case discussed above, where PP2A-B55 activity was used to calculate the steady states of cyclin B:Cdk1 activity (purple balance curve).
Notably, the mechanisms giving rise to a bistable response of cyclin B:Cdk1 with respect to PP2A-B55 activity, and of PP2A-B55 with respect to cyclin B:Cdk1 activity, are intimately interlinked. Cyclin B:Cdk1 phosphorylates and thereby activates GwlCitation23 (right inhibitory arm in ), and B55 dephosphorylates Wee1 and Cdc2519 (left inhibitory arm in ), activating and inactivating them, respectively. Thus, the state of one switch dramatically influences the state of the other. Seen in isolation, each balance curve in shows an incomplete picture of the dynamics of the overall system. Only when considered together do the consequences of the coupling become fully evident. Each balance curve depicts the steady state response of one protein to changes in the other. The intersections of the balance curve highlight steady states of the overall system, and allow us to infer the qualitative behaviour of the system. As the connections between the two switches are inhibitory in both cases, the activities of PP2A-B55 and cyclin B:Cdk1 are inversely related (indicated by the two filled circles in corresponding to stable steady states of the overall system). High activity of PP2A-B55 will keep cyclin B:Cdk1 activity down and vice versa. Moreover this type of coupling gives rise to a mutual inhibition network that can switch with maximum theoretical efficiency.Citation31
The phase plane in is representative of a cell with intermediate amounts of cyclin B, where the cell could either be in mitosis or interphase depending on its history. In order to illustrate how the transitions between interphase and mitosis occur, we change the cyclin B level as a single input parameter and analyse how the phase planes change as a result. We have to mention that during a real interphase/mitosis transition the network integrates numerous inputs (activities of Gwl, Wee1, Cdc25 etc.) controlled by upstream components (e.g. cyclin A) as well. We vary the cyclin B level for three different scenarios. Firstly we look at the original Novak and Tyson model with constitutive phosphatase activity (), then the model with PP2A-B55 inhibition at high Cdk1 activity (), and finally the complete mutual inhibition network ().
The differences between the Novak and Tyson model and our current understanding of mitotic entry and exit are apparent. Whereas the PP2A-B55 balance curve in is a horizontal line (because the phosphatase is unregulated), the balance curve in shows PP2A-B55 becoming inhibited as Cdk1 activity increases. This results in a greater difference in the threshold cyclin B totals of mitotic entry and exit, as well as a larger difference in the kinase/phosphatase ratio between interphase and mitosis.
The model with PP2A-B55 being regulated with positive feedback has a ‘Z’-shaped PP2A-B55 balance curve (), showing that phosphatase regulation alone in this case can generate bistability, even if the kinase had constitutive activity (a vertical line on the phase plane could intersect the PP2A-B55 balance curve in three places). When both the kinase and phosphatase are regulated by positive feedback, as in , the possibility of more than three intersections of the balance curves arises. This would yield an interesting intermediate stable steady state, and a tristable system. The existence of this normally unrealized state is worth investigating experimentally.
The mutual inhibition network generates robustness on two fronts. There is a failsafe mechanism; should one bistable mechanism malfunction, there is another one that can takeover. Secondly, when both bistable mechanisms are functioning, the difference between input thresholds at which transitions between the mutually exclusive states occur is greater than with one bistable mechanism. The difference in the responses (kinase/phosphatase ratio) between the states is also greater with both bistable mechanisms, and these differences create states that are more distinct. This helps the cell to commit to a particular decision even with internal or external fluctuations, but at the expense of the aforementioned bootstrapping problems.
Mitotic checkpoint
Once the mitotic state has been established, cells face the challenge of equally distributing a complete set of chromatids to the nascent daughter cells. The mechanism that drives this process is the assembly of a bipolar mitotic spindle, where corresponding sister chromatids, which are held together by ring-shaped cohesin complexes, become attached to opposite poles of the mitotic spindle. Once correct attachment of all sister chromatids is achieved, a cohesin sub-unit is cleaved and the mitotic spindle promotes the separation of sister chromatids to opposite poles.Citation32
Attachments between kinetochores (large centromeric protein complexes on each chromatid) and the microtubules (that constitute the mitotic spindle) are formed stochastically, but are aided by an active error correction mechanism (EC). This mechanism detects attachment configurations that would lead to incorrect separation of sister chromatids, such as when both sister chromatids are attached to microtubules emanating from the same pole, and destabilises them. It thereby biases the attachment process towards the formation of correct attachments. This is achieved through a tension-sensitive error detection and correction mechanism.Citation33,Citation34 A sharp spatial activity gradient of the kinase Aurora B is coupled with structural deformations of the kinetochores. The effect is to move Aurora B's phosphorylation target out of the Aurora B volume of activity only when correct attachments are formed.
To accommodate the biased yet still intrinsically stochastic attachment process, cells employ a surveillance mechanism, usually known as the spindle assembly checkpoint, which delays the cleavage of cohesin and progression into anaphase until all the chromatids have become correctly attached to the mitotic spindle.Citation35 This delay is achieved through a specific inhibitor of the anaphase-promoting complex/cyclosome (APC/C) called the mitotic checkpoint complex (MCC), which is assembled catalytically by unattached kinetochores. APC/C drives the progression into anaphase by targeting two key substrates for proteasomal degradation: securin, an inhibitor of the specific protease separase, which cleaves cohesin; and cyclin B, thereby promoting the initiation of mitotic exit by reducing the activity of cyclin B:Cdk1.Citation36
To establish efficient control it is necessary that the error correction mechanism is irreversibly inactivated prior to, or synchronously with, the activation of separase. If not, cleavage of cohesin following APC/C activation would induce loss of tension sufficient to cause the untimely destabilisation of attachments, and cause the re-inhibition of APC/C through re-activation of the checkpoint mechanism.Citation37,Citation38 Efficient assembly of MCC has been shown to require the presence of both unattached kinetochores and the activity of cyclin B:Cdk1. Thus, by promoting the inhibition of APC/C through the assembly of MCC in the presence of unattached kinetochores, cyclin B:Cdk1 stabilises its own activity.
In a recent study on Drosophila neuroblastsCitation2 we were able to show that the behaviour of the mitotic checkpoint at a systems-level is best explained by a mutual activation between the checkpoint mechanism and the error correction mechanism. In the checkpoint mechanism, Cdk1 promotes the inhibition of its inhibitor (), and in the error correction mechanism the tensionless kinetochore state is self-promoting (
). Error correction promotes the spindle assembly checkpoint, as error correction creates unattached kinetochores from kinetochores lacking tension, and thereby provides a key signal to the checkpoint mechanism to assemble MCC. The checkpoint mechanism in turn promotes error correction, as Cdk1 activity is required to sustain high activity of Aurora B at kinetochores.Citation39–42
The framework of interlinked switches allows us to reframe this situation (). The mitotic checkpoint works by interlinking two self-promoting subsystems: error correction and the spindle assembly checkpoint mechanism. In contrast to the mitotic entry and exit network, the interconnections between the subsystems establishing mitotic checkpoint control are activating. As a consequence, the corresponding profiles of the activity of error correction and Cdk1 overlap throughout prometaphase (). At the onset of metaphase, all chromosomes have become bioriented, and are now characterised by tension-bearing attachments. With the key signal for error correction gone, its activity drops and makes way for the degradation of cyclin B and the initiation of mitotic exit. Notably, error correction still retains the capacity to respond to detachments of chromosomes from the spindle throughout metaphase.Citation43 This is consistent with the mutually activating nature of our model. This transition is illustrated by the phase plane in . The intersection of the two balance curves for error correction and checkpoint mechanism (Cdk1) illustrate two stable steady states corresponding to high activity in both subsystems and low activity in both subsystems. The transition is driven by the gradual formation of tension-bearing attachments, which result in the inactivation of the error correction mechanism while Cdk1 activity remains high. Yet, with all kinetochores stably attached, the assembly of MCC stops and APC/C becomes activated, which effects the inactivation of Cdk1 via cyclin B degradation.
Conclusions and future directions
Biochemical switches underlie the transitions between different phases of the cell cycle. In this Perspective we have highlighted recent findings on two cell cycle switches, the mitotic checkpointCitation2 and mitotic entry and exit,Citation1 and illustrated the qualitative similarities and differences in the underlying regulatory networks. Cdk1 and PP2A-B55 are both regulated by positive feedback loops, as are the error correction and spindle assembly checkpoint modules of the mitotic checkpoint. In both cases, these bistable mechanisms are interlinked but in opposite ways. Cdk1 and PP2A-B55 have antagonistic effects on the mitotic state, and in turn indirectly inhibit each other's activity. The error correction and spindle assembly checkpoint modules, meanwhile, promote each other's activities, so that both modules are in either the high or low activity states at the same time. In each of the network motifs (mutually inhibiting and mutually activating) just one bistable mechanism would be sufficient to generate a bistable system response. In the phase plane analyses of and this is apparent: just one curve having an ‘S’ or ‘Z’ shape would permit the other curve to intersect it in three places without being ‘Z’ or ‘S’ shaped in turn. By having two interlinked positive loops each capable of generating a bistable system response by themselves the system not only has increased robustness in a fail-safe manner but has increased separation of the stable steady states.Citation44 This leads to more switch-like and defined cell cycle transitions. The expectations of the combined switches await further experimental validation.
Due to the properties that these network motifs impart on the system, we expect them to be apparent in other cell cycle transitions and other cellular transitions between distinct biochemical states. Wherever there are two regulated proteins that are either antagonistic or synergistic in their effects, the mutually inhibiting or mutually activating network motifs could enhance their behaviour.
Acknowledgments
S.R. is supported by the EPSRC with a studentship (EP/G03706X/1). The groups of B.N. and H.H. are supported by a BBRSC strategic LoLa grant (BB/M00354X/1).
References
- Mochida S, Rata S, Hino H, Nagai T, Novák B. Two Bistable Switches Govern M Phase Entry. Curr Biol. 2016;26:3361-3367. doi:10.1016/j.cub.2016.10.022. PMID:27889260
- Mirkovic M, Hutter LH, Novák B, Oliveira RA. Premature sister chromatid separation is poorly detected by the spindle assembly checkpoint as a result of system-level feedback. Cell Rep. 2015;13:469-478. doi:10.1016/j.celrep.2015.09.020
- Morgan D. The cell cycle: Principles of control. London (UK): New Science Press, 2007.
- Tyson JJ, Novak B. Irreversible transitions, bistability and checkpoint controls in the eukaryotic cell cycle: A systems-level understanding. In: Walhout M, Vidal M, Dekker J, editors. Handbook of systems biology. Amsterdam: Elsevier; 2013 p.265-285.
- Errico A, Deshmukh K, Tanaka Y, Pozniakovsky A, Hunt T. Identification of substrates for cyclin dependent kinases. Adv Enzyme Regul. 2010;50:375-399. doi:10.1016/j.advenzreg.2009.12.001. PMID:20045433
- Schmitz M, Held M, Janssens V, Hutchins JR, Hudecz O, Ivanova E, Goris J, Trinkle-Mulcahy L, Lamond AI, Poser I, et al. Live-cell imaging RNAi screen identifies PP2A-B55alpha and importin-beta1 as key mitotic exit regulators in human cells. Nat Cell Biol. 2010;12:886-93. doi:10.1038/ncb2092. PMID:20711181
- Godfrey M, Touati SA, Kataria M, Jones A, Snijders AP, Uhlmann F. PP2ACdc55 Phosphatase imposes ordered cell-cycle phosphorylation by opposing threonine phosphorylation. Mol Cell. 2017;65:393-402. e3. doi:10.1016/j.molcel.2016.12.018. PMID:28132839
- Cundell M., Hutter LH, Nunes Bastos R, Poser E, Holder J, Mohammed S, Novak B, Barr FA. A PP2A-B55 recognition signal controls substrate dephosphorylation kinetics during mitotic exit. J Cell Biol. 2016;214:539-54. doi:10.1083/jcb.201606033. PMID:27551054
- Evans T, Rosenthal E, Youngblom J, Distel D, Hunt T. Cyclin: a protein specified by maternal mRNA in sea urchin eggs that is destroyed at each cleavage division. Cell. 1983;33:389-96. doi:10.1016/0092-8674(83)90420-8. PMID:6134587
- Parker L, Piwnica-Worms H. Inactivation of the p34cdc2-cyclin B complex by the human WEE1 tyrosine kinase. Science. 1992;257:1955-7. doi:10.1126/science.1384126. PMID:1384126
- Mueller PR, Coleman TR, Kumagai A, Dunphy WG. Myt1: a membrane-associated inhibitory kinase that phosphorylates Cdc2 on both threonine-14 and tyrosine-15. Science. 1995;270:86-90. doi:10.1126/science.270.5233.86. PMID:7569953
- Welburn J, Tucker JA, Johnson T, Lindert L, Morgan M, Willis A, Noble ME, Endicott JA. How tyrosine 15 phosphorylation inhibits the activity of cyclin-dependent kinase 2-cyclin A. J Biol Chem. 2007;282:3173-81. doi:10.1074/jbc.M609151200. PMID:17095507
- Tang Z, Coleman T, Dunphy W. Two distinct mechanisms for negative regulation of the Wee1 protein kinase. EMBO J. 1993;12:3427-36 PMID:7504624
- Strausfeld U, Labbé JC, Fesquet D, Cavadore JC, Picard A, Sadhu K, Russell P, Dorée M. Dephosphorylation and activation of a p34cdc2/cyclin B complex in vitro by human CDC25 protein. Nature. 1991;351:242-5. doi:10.1038/351242a0. PMID:1828290
- Izumi T, Maller J. Elimination of cdc2 phosphorylation sites in the cdc25 phosphatase blocks initiation of M-phase. Mol Biol Cell. 1993;4:1337-50. doi:10.1091/mbc.4.12.1337. PMID:7513216
- Novak B, Tyson J. Numerical analysis of a comprehensive model of M-phase control in Xenopus oocyte extracts and intact embryos. J Cell Sci. 1993;106:1153-68 PMID:8126097
- Sha W, Moore J, Chen K, Lassaletta AD, Yi CS, Tyson JJ, Sible JC. Hysteresis drives cell-cycle transitions in Xenopus laevis egg extracts. Proc Natl Acad Sci U A 2003;100:975-80. doi:10.1073/pnas.0235349100.
- Pomerening J, Sontag E, Ferrell JJ. Building a cell cycle oscillator: hysteresis and bistability in the activation of Cdc2. Nat Cell Biol 2003;5:346-51. doi:10.1038/ncb954. PMID:12629549
- Mochida S, Ikeo S, Gannon J, Hunt T. Regulated activity of PP2A-B55 delta is crucial for controlling entry into and exit from mitosis in Xenopus egg extracts. EMBO J. 2009;28:2777-85. doi:10.1038/emboj.2009.238. PMID:19696736
- Hunt T. On the regulation of protein phosphatase 2A and its role in controlling entry into and exit from mitosis. Adv Biol Regul. 2013;53:173-8. doi:10.1016/j.jbior.2013.04.001. PMID:23672858
- Mochida S, Maslen S, Skehel M, Hunt T. Greatwall phosphorylates an inhibitor of protein phosphatase 2A that is essential for mitosis. Science. 2010;330:1670-3. doi:10.1126/science.1195689. PMID:21164013
- Gharbi-Ayachi A, Labbé JC, Burgess A, Vigneron S, Strub JM, Brioudes E, Van-Dorsselaer A, Castro A, Lorca T. The substrate of greatwall kinase, Arpp19, controls mitosis by inhibiting protein phosphatase 2A. Science. 2010;330:1673-7. doi:10.1126/science.1197048. PMID:21164014
- Yu J, Zhao Y, Li Z, Galas S Goldberg ML. Greatwall kinase participates in the Cdc2 autoregulatory loop in Xenopus egg extracts. Mol Cell 2006;22:83-91. doi:10.1016/j.molcel.2006.02.022. PMID:16600872
- Williams BC, Filter JJ, Blake-Hodek KA, Wadzinski BE, Fuda NJ, Shalloway D, Goldberg ML. Greatwall-phosphorylated Endosulfine is both an inhibitor and a substrate of PP2A-B55 heterotrimers. eLife 2014;3:e01695. doi:10.7554/eLife.01695. PMID:24618897
- Kishimoto T. Entry into mitosis: a solution to the decades-long enigma of MPF. Chromosoma. 2015;124:417-28. doi:10.1007/s00412-015-0508-y. PMID:25712366
- Vigneron S, Brioudes E, Burgess A, Labbé JC, Lorca T, Castro A. Greatwall maintains mitosis through regulation of PP2A. EMBO J. 2009;28:2786-2793. doi:10.1038/emboj.2009.228. PMID:19680222
- Cundell MJ, Bastos RN, Zhang T, Holder J, Gruneberg U, Novak B, Barr FA. The BEG (PP2A-B55/ENSA/Greatwall) pathway ensures cytokinesis follows chromosome separation. Mol Cell. 2013;52:393-405. doi:10.1016/j.molcel.2013.09.005. PMID:24120663
- Vinod P, Novak B. Model scenarios for switch-like mitotic transitions. FEBS Lett. 2015;589:667-71. doi:10.1016/j.febslet.2015.02.007. PMID:25683003
- Rogers S, Fey D, McCloy RA, Parker BL, Mitchell NJ, Payne RJ, Daly RJ, James DE, Caldon CE, Watkins DN, et al. PP1 initiates the dephosphorylation of MASTL, triggering mitotic exit and bistability in human cells. J Cell Sci. 2016;129:1340-1354. doi:10.1242/jcs.179754. PMID:26872783
- Ma S, Vigneron S, Robert P, Strub JM, Cianferani S, Castro A, Lorca T. Greatwall dephosphorylation and inactivation upon mitotic exit is triggered by PP1. J. Cell Sci. 2016;129:1329-1339. doi:10.1242/jcs.178855. PMID:26906418
- Cardelli L, Csikász-Nagy A. The cell cycle switch computes approximate majority. Sci Rep. 2012;2:656. doi:10.1038/srep00656. PMID:22977731
- Uhlmann F, Lottspeich F, Nasmyth K. Sister-chromatid separation at anaphase onset is promoted by cleavage of the cohesin subunit Scc1. Nature. 1999;400:37-42. doi:10.1038/21831. PMID:10403247
- Uchida KS, Takagaki K, Kumada K, Hirayama Y, Noda T, Hirota T. Kinetochore stretching inactivates the spindle assembly checkpoint. J. Cell Biol. 2009;184:383-390. doi:10.1083/jcb.200811028. PMID:19188492
- Maresca TJ, Salmon ED. Intrakinetochore stretch is associated with changes in kinetochore phosphorylation and spindle assembly checkpoint activity. J Cell Biol. 2009;184:373-381. doi:10.1083/jcb.200808130. PMID:19193623
- Rieder CL. The checkpoint delaying anaphase in response to chromosome monoorientation is mediated by an inhibitory signal produced by unattached kinetochores. J Cell Biol. 1995;130:941-948. doi:10.1083/jcb.130.4.941. PMID:7642709
- Musacchio A. The molecular biology of spindle assembly checkpoint signaling dynamics. Curr Biol. 2015;25:R1002-18. doi:10.1016/j.cub.2015.08.051. PMID:26485365
- Mirchenko L, Uhlmann F. Sli15INCENP Dephosphorylation prevents mitotic checkpoint reengagement due to loss of tension at anaphase onset. Curr Biol. 2010;20:1396-1401. doi:10.1016/j.cub.2010.06.023. PMID:20619650
- Oliveira RA, Hamilton RS, Pauli A, Davis I, Nasmyth K. Cohesin cleavage and Cdk inhibition trigger formation of daughter nuclei. Nat Cell Biol. 2010;12:185-192. doi:10.1038/ncb2018. PMID:20081838
- Tsukahara T, Tanno Y, Watanabe Y. Phosphorylation of the CPC by Cdk1 promotes chromosome bi-orientation. Nature. 2010;467:719-723. doi:10.1038/nature09390. PMID:20739936
- Vázquez-Novelle MD, Petronczki M. Relocation of the chromosomal passenger complex prevents mitotic checkpoint engagement at anaphase. Curr Biol. 2010;20:1402-1407. doi:10.1016/j.cub.2010.06.036. PMID:20619651
- Rattani A, Vinod PK, Godwin J, Tachibana-Konwalski K, Wolna M, Malumbres M, Novák B, Nasmyth K. Dependency of the spindle assembly checkpoint on Cdk1 renders the anaphase transition irreversible. Curr Biol. 2014;24:630-637. doi:10.1016/j.cub.2014.01.033. PMID:24583015
- Vázquez-Novelle MD, Sansregret L, Dick AE, Smith CA, McAinsh AD, Gerlich DW, Petronczki M. Cdk1 inactivation terminates mitotic checkpoint surveillance and stabilizes kinetochore attachments in anaphase. Curr Biol. 2014;24:638-645. doi:10.1016/j.cub.2014.01.034. PMID:24583019
- Dick AE, Gerlich DW. Kinetic framework of spindle assembly checkpoint signalling. Nat Cell Biol. 2013;15:1370-1377. doi:10.1038/ncb2842. PMID:24096243
- Ferrell JE. Feedback regulation of opposing enzymes generates robust all-or-none bistable responses. Curr Biol. 2008;18:R244-R245. doi:10.1016/j.cub.2008.02.035. PMID:18364225