ABSTRACT
Ionizing radiation causes not only targeted effects in cells that have been directly irradiated but also non-targeted effects in several cell generations after initial exposure. Recent studies suggest that radiation can enrich for a population of stem cells, derived from differentiated cells, through cellular reprogramming. Here, we elucidate the effect of irradiation on reprogramming, subjected to two different responses, using an induced pluripotent stem cell (iPSC) model. iPSCs were generated from non-irradiated cells, directly-irradiated cells, or cells subsequently generated after initial radiation exposure. We found that direct irradiation negatively affected iPSC induction in a dose-dependent manner. However, in the post-irradiated group, after five subsequent generations, cells became increasingly sensitive to the induction of reprogramming compared to that in non-irradiated cells as observed by an increased number of Tra1-81-stained colonies as well as enhanced alkaline phosphatase and Oct4 promoter activity. Comparative analysis, based on reducing the number of defined factors utilized for reprogramming, also revealed enhanced efficiency of iPSC generation in post-irradiated cells. Furthermore, the phenotypic acquisition of characteristics of pluripotent stem cells was observed in all resulting iPSC lines, as shown by morphology, the expression of pluripotent markers, DNA methylation patterns of pluripotency genes, a normal diploid karyotype, and teratoma formation. Overall, these results suggested that reprogramming capability might be differentially modulated by altered radiation-induced responses. Our findings provide that susceptibility to reprogramming in somatic cells might be improved by the delayed effects of non-targeted response, and contribute to a better understanding of the biological effects of radiation exposure.
Introduction
Ionizing radiation (IR) can induce not only targeted effects, primarily affecting DNA, but also non-targeted effects. The latter event, specifically, the induction of biological changes in non-irradiated cells that are similar to the responses observed after direct irradiation, has emerged as a new paradigm in radiobiology.Citation1 Non-targeted response is considered to amplify a variety of radiation-induced biological effects including adaptive response, genomic instability, premature differentiation of cells, and bystander effect. These responses can be observed in either cells exposed to signaling molecules released by irradiated cells, which activate non-irradiated cells or in subsequent generations of irradiated cells.Citation2,Citation3 Among radiation-induced non-targeted response, the delayed effect such as delayed reproductive death and chromosomal instability have been described in the descendants of irradiated cells. Its persistence over many generations after irradiation has been considered relevant for the human health risk associated with radiation-induced carcinogenesis.Citation4-6
The reprogramming of somatic cells to induced pluripotent stem cells (iPSCs) has been accomplished by the ectopic expression of transcription factors such as Oct4, Sox2, Klf4, and c-Myc (OSKM). iPSCs have been shown to have similar characteristics to embryonic stem cells (ESCs), such as self-renewal and pluripotency, indicating that iPSCs could be applied for cell therapy and tissue engineering in regenerative medicine.Citation7-9 To make iPSCs more amenable for therapeutic application, the original methodology has been modified through the use of several strategies including a reduction in the required number of factors in attempt to avoid the use of oncogenes such as c-Myc,Citation10 the inclusion of cell permeable proteins to trigger reprogramming,Citation11 or the use of a number of small molecules to overcome low efficiency.Citation12 Moreover, there is growing evidence that environmental agents including nutrients,Citation13 hyperosmosis,Citation14 antioxidants,Citation15 and low-frequency electromagnetic fieldsCitation16 can modulate reprogramming to a pluripotent state.
Radiation is a ubiquitous environmental agent occurring naturally in terrestrial rocks and the atmosphere, and is also utilized in clinically for medical applications such as X-rays. Studies have suggested that radiation-enriched stem cell populations, derived from differentiated cells, can be induced through cellular reprogramming in breast cancerCitation17 and in the non-tumorigenic mammary epithelium.Citation18 It has also been reported that a positive effect of radiation on reprogramming of mouse embryonic fibroblasts (MEF) to pluripotency is mediated by IR-induced cytokine production.Citation19 Therefore, it is of particular interest to study the effect of radiation on pluripotency reprogramming of human cells, including that based on both responses, namely, targeted and non-targeted effects of radiation.
In this study, to address the effect of both radiation responses on pluripotency reprogramming, we investigated iPSCs induction using non-irradiated cells, directly irradiated cells, and cells subsequently generated after initial radiation exposure. We used human fibroblasts as a reliable experimental system, with a well-described radiation response, in association with the gold standard to induce pluripotency reprogramming.Citation8-11 By comparing reprogramming efficiency and characterizing the resulting iPSCs, we found that post-irradiated cells, but not directly irradiated cells, were sensitive to reprogramming, resulting in the generation of bona fide iPSCs. Therefore, our study suggests that susceptibility to pluripotency reprogramming can be induced by the delayed effect of non-targeted response, and differentially affected by both radiation responses.
Results
Direct exposure to radiation attenuates pluripotency induction in human fibroblasts
It has been reported that human induced pluripotent stem cells (hiPSCs) can be generated by reprogramming somatic cells via the ectopic expression of four defined factors including Oct4, Sox2, Klf4, and cMyc (OSKM).Citation8,Citation9 IR causes not only targeted effects but also non-targeted effects several cell generations after initial exposure.Citation1-6 Therefore, we asked whether radiation effects somatic cell reprogramming with respect to the two different radiation responses. To assess the effect of targeted radiation on the reprogramming of somatic cells to pluripotency, as shown in , we transduced retroviral vectors expressing OSKM into human fibroblasts, as the radiation response in these cells has been, and because these cells are commonly used for reprogramming.Citation8,Citation9 After radiation exposure, the induction of pluripotent stem cells was achieved by using TesR-E7 medium, as this results in superior hiPSC formation when compared to that with various feeder-free reprogramming media, as observed by human embryonic stem cell (hESC)-like morphology and the expression of SSEA4 and Tra1-81,Citation20 a known surface marker of pluripotent stem cells (Supplementary Fig. S1). Prior to hiPSC formation, the analysis of GFP and OSKM levels suggested similar transduction efficiency and expression patterns, respectively, between non-irradiated and irradiated cells, as determined by FACS and immunoblotting (). In contrast, the number of Tra1-81-stained colonies was significantly reduced, in a dose dependent manner, after hiPSC induction. Moreover, we could not detect any colonies after irradiating cells with 10 Gy during reprogramming. Therefore, results suggested that somatic cell reprogramming efficiency was negatively affected after exposure to direct radiation.
Figure 1. Reduced generation of induced pluripotent stem (iPS) cells from fibroblasts after direct radiation exposure. Fibroblasts (passage 12∼13) were transduced with retro-GFP or retro-4F (OSKM) twice sequentially, which was followed by irradiation exposure at the indicated doses. After further culture for 3 d, transduced cells were transferred to Matrigel-coated plates to induce reprogramming as shown in (A). (B) Efficiency of transduction in non-irradiated or irradiated fibroblasts using retroviral GFP. Quantification of GFP expression in irradiated fibroblasts before reprogramming by FACS analysis. Scale bar = 200 μm. (C) Immunoblotting of OSKM overexpression in non-irradiated or irradiated fibroblasts before reprogramming. (D) Morphology and live staining of Tra1-81 (upper panel) and numbers (lower panel) of Tra1-81 stained colonies in reprogrammed cells derived from non-irradiated or irradiated fibroblasts after OSKM-transduction. Staining of Tra1-81 was performed at two weeks during reprogramming. Scale bar = 100 μm. The data in the lower panel are shown as mean ± SD from three independent experiments (**P < 0.01, one-way ANOVA analysis with Scheffe pairwise post-hoc test).
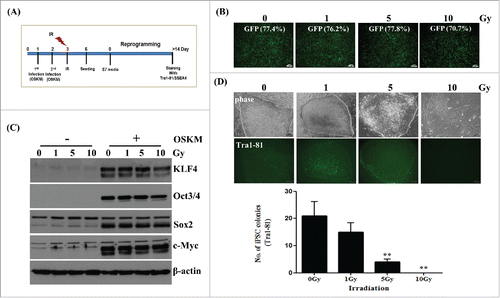
Post-irradiation enhances four (4F)- or three factor (3F)-induced hiPSC reprogramming efficiency
To investigate the non-targeted effects of radiation on somatic cell reprogramming, we designed our experimental scheme in accordance with a previous report in which irradiated fibroblasts were continuously sub-cultured after initial exposure.Citation21 Thus, non-irradiated (control) and irradiated fibroblasts sub-cultured for the indicated number of days were transduced with four factors (OSKM) in feeder-free conditions for iPSC induction (). At 3 d (D3) post-irradiation, the frequency of hiPSC induction decreased in a radiation dose-dependent manner; this was very similar to the results observed in . Interestingly, hiPSC formation steadily increased in fibroblasts cultured for 14 d (D14) post-radiation exposure, with the exception of those cultured at a dose of 10 Gy. Maximum induction occurred in post-irradiated cells exposed to a dose of 5 Gy (referred to as 5Gy-D14), when compared to that in non-irradiated cells (OGy-D14), as assessed by enumerating Tra1-81 stained-colonies, a known surface marker of pluripotent stem cells ( and Supplemental Fig. S2C). Using traditional condition of hiPSC generation on murine-derived feeder cells,Citation8 the similar results were also obtained (Supplemental Fig. S2D). Consistent with this, 5Gy-D14 cells also exhibited considerably stronger hiPSC induction, as shown by alkaline phosphatase (ALP) assays; levels significantly increased by more than two-fold, when compared to those with 0Gy-D14 (non-irradiated) cells (). We further confirmed the enhanced efficiency of hiPSC reprogramming using 5Gy-D14 cells harboring an eGFP reporter at the endogenous Oct4 locus.Citation22 Here, an approximate two-fold increase in the number of Oct4-eGFP positive cells was observed post-irradiation by FACS analysis (). In contrast, there was no significant difference in the expression of retro-GFP and OSKM between control cells (0Gy-D14) and 5Gy-D14 cells (Supplemental Fig. S2A and B).
Figure 2. Enhanced generation of induced pluripotent stem (iPS) cells from fibroblasts on day 14 post-irradiation using four factors (4F) for reprogramming. Fibroblasts were exposed to irradiation and were further sub-cultured for the indicated number of days; this was followed by generation of iPS cells using retrovirus encoding four factors (OSKM). (A) Overall scheme of this study. (B and C) The numbers of Tra1-81- or alkaline phosphatase (ALP)-stained colonies induced from OSKM-transduced fibroblasts at 3 d (D3) or 14 d (D14) post-irradiation. Staining for Tra1-81 and ALP was performed at 2 or 3 weeks, respectively, during reprogramming. The data are shown as mean ± SD from three independent experiments (**P < 0.01, one-way ANOVA analysis with Scheffe pairwise post-hoc test). (D) Morphology and GFP expression in Oct4-promoter EGFP expressing 5Gy-D14 cells during reprogramming (via OSKM). Post-irradiated fibroblasts were transduced with Oct4 promoter-EGFP for 1 d before OSKM infection. Scale bar = 100 μm. Oct4-promoter EGFP expressing colony numbers were counted at 2 weeks during reprogramming. The data are shown as mean ± SD from three independent experiments (**P < 0.01, one-way ANOVA analysis with Scheffe pairwise post-hoc test). (E) Quantification of GFP expression by flow cytometry analysis. Representative image of Oct4-promoter EGFP expressing colonies during reprogramming. FITC represents the intensity of GFP expression, and the y-axis represents cell counts. 0Gy-D14: non-irradiated fibroblasts sub-cultured for 14 d (control), 5Gy-D14: fibroblasts at 14 d post-irradiation with 5 Gy.
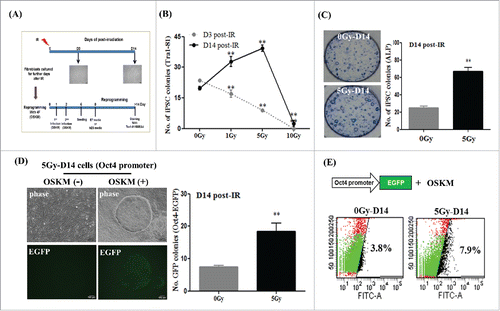
To clarify whether post-irradiation facilitates reprogramming, we attempted to reprogram post-irradiated cells (5Gy-D14) with a reduced number of factors (), as performed in previous reports that iPSCs can also be induced by three factors (OSK) at very low efficiency.Citation7,Citation10,Citation15 Consistent with this, we observed that three factors (3F) including Oct4, Sox2, and Klf4, but not other combinations of the three factors, could induce iPSC generation from post-irradiated cells (Supplemental Fig. S3). Interestingly, comparative analysis of 3F-induced reprogramming revealed the enhanced reprogramming of post-irradiated cells (5Gy-D14), as exhibited by Tra1-81-stained colonies and ALP assays during reprogramming (). Unexpectedly, we also found that TesR-E7, feeder-free reprogramming media, yielded high efficiency of iPSC reprogramming from fibroblasts (approximately 0.02–0.03%), compared to a previous report demonstrating very low hiPSC reprogramming efficiency from fibroblasts using mouse embryonic fibroblasts as feeder cells in conjunction with 3F (Oct4, Sox2, and Klf4)-mediated reprogramming.Citation10 Furthermore, RT-PCR, using genomic DNA from 3F-iPS clones derived from 5Gy-D14 cells, confirmed the specific integration of the indicated retroviral factors (). Therefore, all results suggest that a delayed radiation response can improve the efficiency of hiPSC reprogramming when using human fibroblasts.
Figure 3. Efficiency of induced pluripotent stem cells (iPSC) reprogramming from post-irradiated fibroblasts using three factors (OSK). (A) The overall scheme to generate iPSCs derived from post-irradiated fibroblasts with 3F (Oct4, Sox2, and Klf4). (B and C) The numbers of Tra1-81- or alkaline phosphatase (ALP)-stained colonies induced from OSK-transduced 0Gy-D14 cells and 5Gy-D14 cells. Staining for Tra1-81 and ALP was performed at 3 or 4 weeks during reprogramming, respectively. The data are shown as mean ± SD from three independent experiments (*P < 0.05, **P < 0.01, one-way ANOVA analysis with Scheffe pairwise post-hoc test). (D) PCR analysis to confirm the absence of c-Myc chromosomal integration in 3F-iPSCs generated from 5Gy-D14 cells. 0Gy-D14: non-irradiated fibroblasts cultured for 14 d as a control, 5Gy-D14: fibroblasts at 14 d post-irradiation with 5Gy, 4F-iPSC (5Gy-D14): iPSCs derived from fibroblasts at 14 d post-irradiation with 5 Gy using four factors (OSKM), 3F-iPSC (5Gy-D14): iPSCs derived from fibroblasts at 14 d post-irradiation with 5 Gy using three factors (OSK).
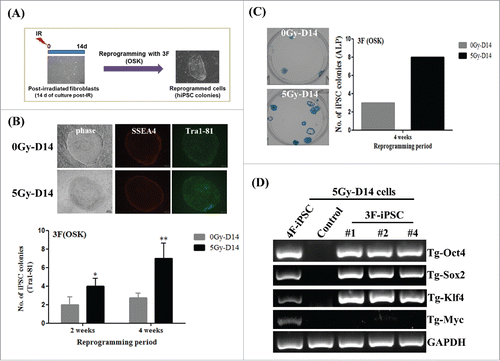
Characterization of human iPSCs generated from fibroblasts at 14 d post-irradiation
To assess whether the enhanced reprogramming efficiency obtained by post-irradiation could eventually generate bona fide iPSCs,Citation20,Citation23 we generated a series of iPSC lines from post-irradiated fibroblasts (5Gy-D14 cells) using 4F (OSKM) or 3F (OSK) combinations of factors. After several passages, all clones exhibited typical morphology of hESCs such as a flat shape, large nucleoli, and scant cytoplasm. The characteristics of these iPSC clones (5Gy-D14), based on the expression of pluripotent markers such as ALP, Oct4, Sox2, and Nanog, were examined by immunostaining and real-time PCR (, other clones are shown in Supplemental Fig. S4A and B). DNA methylation analysis of Oct4 and Nanog promoters revealed that demethylation levels in 3F and 4F iPSCs (5Gy-D14) were similar. In contrast, the Oct4 and Nanog promoters of parental cells (fibroblasts) were hypermethylated (). Further analysis was performed using the clones exhibiting a normal karyotype (). The pluripotency of selected 4F-hiPSCs (5Gy-D14) were confirmed by embryonic body (EB) formation in vitro and by assessing the development of teratomas containing tissues derived from all three germ layers including primitive neuron structures (ectoderm), cartilage structures (mesoderm), and gut-like structures (endoderm) upon subcutaneous transplantation into SCID mice (). We also confirmed the pluripotency of 3F-hiPSCs (5Gy-D14) by investigating EB formation and in vitro tri-lineage differentiation (). Similarities between reprogrammed cells from non-irradiated fibroblasts (control) and true iPSCs were further demonstrated by real-time PCR, immunohistochemistry, and in vitro tri-lineage differentiation analysis (Supplemental Fig. S5).
Figure 4. Characterization of induced pluripotent stem cells (iPSCs) generated from post-irradiated fibroblasts using four factors (OSKM) or three factors (OSK). (A) Morphology, immunostaining for pluripotent markers (Oct4, Sox2, and Nanog), and alkaline phosphatase (ALP) assay in 4F- or 3F-iPSCs (passage 7) derived from 5Gy-D14 cells. DAPI was used for counting nuclear staining. Scale bar = 100 μm. (B) Quantitative RT-PCR analysis of expression of endogenous pluripotent markers (Nanog, Oct4, and Sox2) in 4F- or 3F-iPSCs (5Gy-D14). mRNA expression levels of pluripotent markers was calculated relative to that of GAPDH and normalized to the parental cells as the control. The data are shown as means ± SDs from triplicate experiments (**P < 0.01, one-way ANOVA analysis with Scheffe pairwise post-hoc test) (C) DNA methylation analysis of several CpG sites in Oct4 and Nanog promoters, indicating demethylation of Oct4 and Nanog promoters in 4F- or 3F-iPSCs generated from 5Gy-D14 cells. Oct4 and Nanog promoters of parental cells were hyper-methylated. (D) Karyotyping of 4F- or 3F-iPSCs generated from 5Gy-D14 cells. NUFF: newborn human foreskin fibroblasts as donor cells. 4F-iPSC (5Gy-D14): iPSCs derived from fibroblasts at 14 d post-irradiation with 5 Gy using four factors (OSKM). 3F-iPSC (5Gy-D14): iPSCs derived from fibroblasts at 14 d post-irradiation with 5 Gy using three factors (OSK).
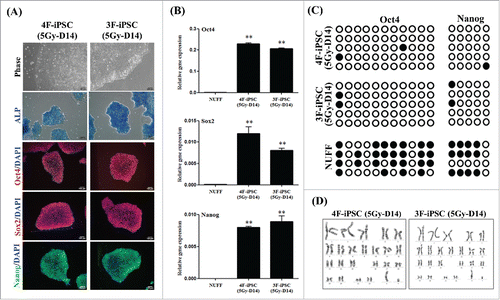
Discussion
IR causes not only targeted effects on irradiated cells, but also non-targeted effects, several cell generations after initial exposure.Citation1-6 Recent studies suggested that the radiation-enriched stem cell population, derived from differentiated cells, is associated with cellular reprogramming.Citation17-19 In this study, we extended these studies to demonstrate for the first time that susceptibility to pluripotency reprogramming can depend on the delayed effect of non-targeted rather than targeted response of radiation. Using human fibroblasts as a well-defined experimental model for reprogramming somatic cells into pluripotent state,Citation8-11,Citation20,Citation22 we found that capacity of somatic cell reprogramming can depend on the type of radiation response; we also confirmed that these reprogrammed cells are bona fide iPSCs.
We established iPSC lines from non-irradiated cells, directly irradiated cells, and post-irradiated cells (five generations after initial exposure), through the retroviral expression of Oct4, Sox2, Klf4, and c-Myc (OSKM) using a standard protocol of reprogramming.Citation8 Our data demonstrated reduced induction of iPSCs after direct irradiation, whereas iPSC induction was significantly enhanced in post-irradiated cells, when compared to that in non-irradiated cells (, and ). This indicated that pluripotency reprogramming could be differentially modulated by the type of radiation response such as targeted- or non-targeted effects. We confirmed that established iPSC lines acquired similar characteristics to those of pluripotent stem cells through the analysis SSEA4, Tra1-81, Oct4, Sox2, and Nanog expression, known as major pluripotency markers. The ability to differentiate into cells from all three germ layers in vivo, known as a teratoma, is representative evidence of pluripotency.Citation23 Indeed, reprogrammed cells from non-irradiated and post-irradiated cells showed the capacity to differentiate into cells derived from endoderm, mesoderm, and ectoderm ( and Supplemental Fig. S5).
Figure 5. The pluripotency of induced pluripotent stem (iPS) cells generated from post-irradiated fibroblasts. (A) Formation of embryonic bodies and the development of teratomas derived from three germ layers, as shown by hematoxylin and eosin (HE) staining of 4F-iPSCs generated from 5Gy-D14 cells. Each panel shows ectoderm (primitive neural cells), mesoderm (cartridge), and endoderm (epithelium). Scale bar = 200 and 50 μm. (B) The formation of embryonic bodies and evidence of differentiation potential from 3F-iPSCs into tri-lineage type cells in vitro. Immunostaining was performed using antibodies specific for the three germ layers as follows: Otx2 (red, ectoderm), GATA4 (green, endoderm), and Brachyury (red, mesoderm). Nuclei were double stained with DAPI (blue). Scale bar = 50 μm. In vitro tri-lineage differentiation from 3F-iPSCs (5Gy-D14) was performed as indicated in the materials and methods. 4F-iPSC (5Gy-D14): iPSCs derived from fibroblasts at 14 d post-irradiation with 5 Gy using four factors (OSKM), 3F-iPSC (5Gy-D14): iPSCs derived from fibroblasts at 14 d post-irradiation with 5 Gy using three factors (OSK).
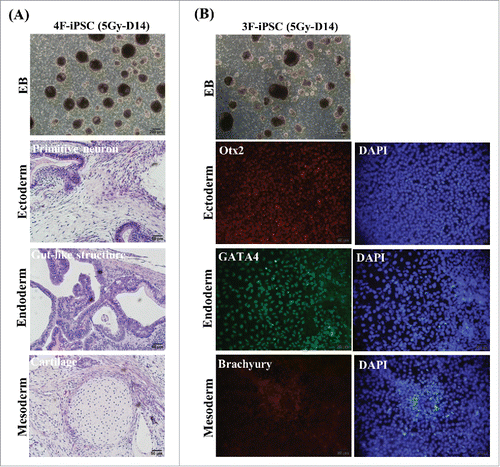
A variety of phenotypes persist during the delayed effect of radiation exposure, including delayed reproductive death and chromosomal instability.Citation4,Citation5 One type of damage that is transmitted over many generations following irradiation has been referred to as genomic instability, which results from DNA double strand breaks (DSB) induced by exposure to high doses of irradiation.Citation24 To confirm this, we previously observed increased phosphorylation of γ-H2AX and 53BP1, known DSB indicators,Citation25,Citation26 in cells at 0.5 h and 1 d post-irradiation with 5 Gy. However, when cells were cultured for five generations after initial irradiation at 5 Gy (5Gy-D14 cells), DSBs could not be detected, suggesting that prolonged cell cultivations results in recovery from radiation-induced genomic instability (unpublished data). Indeed, we did not observe beta-galactosidase (SA-βgal) activity, a biomarker of senescent cellsCitation27 in 5Gy-D14 cells, whereas strong SA-βgal activity was detected in 10Gy-D14 cells (unpublished data). These results were consistent with a previous report suggesting that exposure to high-dose radiation induces persistent genomic instability, leading to cellular senescence.Citation28 Thus, the limited or lack of iPSC induction in 10 Gy-exposed cells might have been due to radiation-induced senescence, which is hurdle for pluripotency reprogramming from somatic cells.Citation29 Interestingly, established iPSCs from 5Gy-D14 cells using OSKM (4F) and OSK (3F) exhibited a normal karyotype (). Given that iPSCs derived from patient cells harbor the same genetic aberrations,Citation30 post-irradiated cells exposed to 5 Gy were likely to be chromosomally similar to normal cells, at least in part. Therefore, enhanced reprogramming with post-irradiation is likely to novel type of delayed effects, because the event in our condition seems to be less related with genomic instability which is known as a major phenotype to delayed effects of irradiation, although we cannot exclude the possibility of minor genetic alterations that could not be detected by karyotype analyses.
The mechanism through which factors are involved in susceptibility to reprogramming in post-irradiated cells remains elusive. By analysis for the impact of secretome of IR-induced senescent cells on pluripotency reprogramming of mouse embryonic fibroblasts (MEF), Mosteiro et al.Citation19 reported that cytokine production associated with IR-mediated senescent cells facilitates OSKM-driven reprogramming of MEF, indicating that reprogramming capability can be enhanced by the bystander effect of non-targeted radiation response. This notion is further supported by our study showing the enhanced pluripotency reprogramming of descendants of irradiated cells as the delayed effect of non-targeted radiation response. Considering the five generations after radiation exposure and no observation of elevated SA-βgal activity (unpublished data) in cells used for the present study, enhanced reprogramming with post-irradiation seems less related to the cytokine released from senescent cells after irradiation. In another report by Lagadec et al., radiation-induced reprogramming is associated with reactivation of major pluripotent transcription factors, especially including Oct4 and Sox2 in breast cancer cells.Citation17 However, with the conditions used herein, we did not observe upregulation of these factors in directly-irradiated or post-irradiated fibroblasts ( and Supplemental Fig S2B). A recent study reported that the process involved in reprogramming is modulated through telomerase activity,Citation31 restructuring of mitochondriaCitation32 or histone post-translational modification.Citation33 Among them, chromatin remodeling has emerged as a major mediator of non-targeted effects associated with radiation and pluripotency reprogramming.Citation33,Citation34 It is well characterized that histone H3 acetylation (AcH3) and methylation of histone H3 at lysine 4 (H3K4me3) are associated with transcript activation and are critical during the early phase of reprogramming.Citation33 Consistently, we observed a significant increase in AcH3 and H3K4me3 in 5G-D14 post-irradiated cells, compared to that in 0Gy-D14 cells, as assessed by western blotting, and based on preliminary results (unpublished data), suggesting the possibility that post-irradiation might induce pre-modulation of the epigenetic state in cells that are primed to undergo cellular reprogramming. Further studies are required to determine the functional relationship between epigenetic regulators and reprogramming in post-irradiated cells. It also remains to be explored whether these finding are relevant for other cell types after radiation exposure.
Materials and methods
Antibodies and plasmids
The antibodies used in this study were anti-OCT3/4 (Santa Cruz Biotechnology, cat. No sc-5279), anti-Sox2 (Cell Signaling Technology, cat. No #4900), anti-KLF4 (Santa Cruz Biotechnology, cat. No sc-20691), anti-c-Myc (Santa Cruz Biotechnology, cat. No sc-764), anti-Nanog (Cosmo Bio, cat. No REC-RCAB0004PF), and anti-β-actin (Santa Cruz Biotechnology, cat. No sc-47778). The plasmids used in this study were purchased from Addgene for pMXs-hKlf4, pMXs-hOct4, pMXs-hSox2, pMXs-c-hMyc, pCMV-VSVG, and PL-SIN-EOS-C(3+)-EiP and from Cell Biolabs for pMXs-IRES-GFP.
Animals, cell culture, and irradiation
All animal experiments were approved by the Animal Investigation Committee of the Korea Institute of Radiological and Medical Sciences in Korea and were performed according to the institutional guidelines and national animal protection laws. SCID male mice, 8∼10-weeks of age (n = 4, Central Laboratory Animals) were used for teratoma assays. Newborn human foreskin fibroblasts (passage 9) were purchased from Globalstem Inc. and maintained in DMEM containing 10% FBS. Reprogrammed cells were grown on Matrigel-coated plates with mTeSR1 medium (STEMCELL Technology Inc) and were passaged using ReLeSR (STEMCELL Technology Inc). The medium was changed every day. For irradiation treatments, fibroblasts were seeded at 5 × 105 in 100-mm dishes and incubated overnight; cells were then exposed to 137Cs γ-rays from a Gamma Cell-3000 irradiator (MDS Nordion International). Non-irradiated or irradiated cells were further cultured by passaging every 3 d (5 × 105 cells/dish), and cells were reprogrammed on the indicated days.
Generation of OSKM retrovirus and reprogramming of irradiated human fibroblasts
We previously described the generation of retroviral particles encoding reprogramming factors, as well as the reprogramming of somatic cells.Citation35,Citation36 In brief, gp293 cells were seeded and transfected with each retroviral vector (Klf4, Oct4, Sox2, c-Myc) plus the virus packaging plasmid (VSVG) using Lipofectamine 2000 (Invitrogen). For virus infection, non-irradiated and post-irradiated fibroblasts were seeded in 6-well plates. The next day, attached cells were infected with viral particles containing polybrene for 8 h. After 5 d, transduced cells were seeded at 5 × 104 cells/well in 6-well plates coated with Matrigel, and incubated until the appearance of ES-like cells by changing feeder-free reprogramming medium for human iPS cell induction (TeSR-E7 media, STEMCELL Technology) every day. To monitor the efficiency of retrovirus infection, the cells transduced with retrovirus-GFP were resuspended in 0.5 ml of FACS buffer (1% BSA in PBS). The efficiency of cell infection was analyzed by FACS using a FACSCanto (BD Biosciences) and the CellQuest program (BD Biosciences).
Live cell staining and ALP assay
Live staining for Tra1-81 and SSEA4 was performed using StainAlive anti-human TRA1-81, DyLight 488 and anti-human SSEA-4, DyLight 550 (STEMGENT, cat. No 09–0069 and 09–0097, respectively). ALP activity was determined using an ALP detection kit (System Biosciences) according to the manufacturer's protocol.
Western blotting
Cells were lysed in buffer containing 50 mM tris-HCl (pH 7.4), 5 mM EDTA, 150 mM NaCl, 1% Nonidet P-40, and a protease inhibitor mixture. Equal amounts of the protein (20 μg) were separated by SDS-PAGE and then electrotransferred to Immobilon membranes (Millipore, Bedford, MA), which were subsequently blotted using the indicated antibodies and visualized using chemiluminescence (Amersham ECL; GE Healthcare Life Sciences).
RNA preparation and quantitative real-time PCR (qPCR)
Total RNA was prepared from cells as indicated using the RNase mini kit (Qiagen), according to the manufacturer's protocol. A total of 1 µg of RNA was reverse transcribed using the AccuPower RT PreMix kit (Bioneer). Quantitative PCR was performed using FastStart Essential DNA Green Master (Roche, Indianapolis, IN, USA). All reactions were performed in triplicate. mRNA expression was normalized to that of endogenous glyceraldehyde 3-phosphate dehydrogenase (GAPDH) and expressed relative to control cells. Primer sequences are listed in Supplemental Table S1.
Genomic DNA purification and DNA methylation
Genomic DNA was isolated and purified from cells using the QIAamp DNA micro kit (Qiagen) according to the manufacturer's protocol. To analyze the methylation status of the target sites, each sample was then bisulfite-modified using a Methyl Detector kit (Active Motif) according to the manufacturer's protocol and stored at −80 °C until use. Modified DNA was amplified by PCR using Platinum® Taq DNA Polymerase (Invitrogen). Primer sequences and conditions for PCR are listed in Supplemental Table S1. Each PCR product was purified using a gel extraction kit (Real Biotech Corp.). The purified products were cloned into the T&A vector using the T&A cloning vector kit according to the manufacturer's instructions (Real Biotech Corp). The clones, including purified DNA, were introduced into DH5α competent cells by transformation following the manufacturer's instructions (iNtRON). Plasmid DNA was isolated using a plasmid mini-prep kit and were sequenced using the M13 universal primer by the Macrogen service center (Macrogen). Primer sequences are listed in Supplemental Table S2.
Immunocytochemistry
Cells were fixed with 4% paraformaldehyde in PBS for 30 min at room temperature. After washing with PBS, cells were incubated with PBS containing 1% BSA and 0.1% triton X-100 for 1 h at room temperature; this was followed by incubation with primary antibodies including hOCT3/4 (1:50), hSox2 (1:100), hNanog (1:200). Alexa Fluor 488 goat anti-rabbit IgG (1:500, Invitrogen, cat. No A11008) or Alexa Fluor 594 goat anti-mouse IgG (1:500, Invitrogen, cat. No A11005) was used as the secondary antibody. Images were acquired using an immunofluorescence microscope (Olympus).
Karyotyping of hiPSC lines
For post-irradiated fibroblast-derived iPSCs (passage 5), chromosomal analysis after GTG-banding was performed. For each line, 20 metaphase spreads were analyzed and normal lines, as defined in this study, were euploid in 20 of 20 cells.
EB formation and teratoma assay
The differentiation potential of hiPSCs was investigated by the induction of EB formation in vitro and teratoma development in vivo. For EB formation, dissociated iPSC colonies (at passage 6∼7) in ReLeSR (STEMCELL Technology) were transferred to ultra-low attachment 6-well plates, and incubated overnight in suspension. Suspended cells were then cultured with hESC media without bFGF for 5 d to form spheroids. Media was changed every day. The shape of EBs was analyzed using an inverted light microscope (Olympus). hESC medium contained DMEM/F12, 20% knockout serum, 2 mM GlutaMAX, 0.1 mM nonessential amino acids, 0.1 mM beta-mercaptoethanol, 50 U/ml penicillin, 50 g/ml streptomycin, and 10 ng/ml bFGF as previously reported.Citation8 For teratoma assays, hiPSCs were collected using accutase (BD Biosciences), resuspended in 50% Matrigel in HBSS buffer (Invitrogen), and subcutaneously inoculated into SCID mice (2 × 106 cells/mouse). Tumors were dissected 8–10 weeks after transplantation and embedded in paraffin for routine histology using hematoxylin and eosin (H&E).
Tri-lineage differentiation in vitro
The direct differentiation of 3F-iPSC (5Gy-D14) into three germ layers in vitro was induced using a human pluripotent stem cell functional identification kit (R&D Systems, cat. No SC027). In brief, 2 × 105 cells were seeded on Matrigel-coated slide chambers and cultured in media containing 10 μM of the ROCK inhibitor Y27632 (STEMCELL Technologies Inc). Next day, culture medium was changed to differentiation medium, and each of the three germ layers was induced according to the manufacturer's instructions.
Supporting information
The supporting information includes the Supplemental Materials and Methods, Table, Figure legends, and Figures.
Disclosure of potential conflict of interest
The authors indicate no potential conflicts of interest.
KCCY_A_1371887_Supplement.doc
Download MS Word (98 KB)Acknowledgments
This study was supported by a grant of the Korea Institute of Radiological and Medical Sciences (KIRAMS), funded by the Ministry of Science, ICT & Future Planning, Republic of Korea (1711045573;1711045551/50535-2017).
Funding
This study was supported by a grant of the Korea Institute of Radiological and Medical Sciences (KIRAMS), funded by Ministry of Science, ICT and Future Planning, Republic of Korea (No. 1711045573;1711045551/50535-2017)
References
- Baverstock K, Belyakov OV. Classical radiation biology, the bystander effect and paradigms: a reply. Hum Exp Toxicol. 2005;24(10):537-42. doi:10.1191/0960327105ht562oa. PMID:16270755
- Morgan WF. Is there a common mechanism underlying genomic instability, bystander effects and other nontargeted effects of exposure to ionizing radiation? Oncogene. 2003;22(45):7094-9. doi:10.1038/sj.onc.1206992. PMID:14557815
- Widel M. Radiation induced bystander effect: From in vitro studies to clinical application. Int J Med Phys Clin Eng Radiat Oncol. 2016;5:1-17. doi:10.4236/ijmpcero.2016.51001
- Suzuki K, Ojima M, Kodama S, Watanabe M. Radiation-induced DNA damage and delayed induced genomic instability. Oncogene. 2003;22(45):6988-93. doi:10.1038/sj.onc.1206881. PMID:14557802
- Han W, Yu KN. Response of cells to ionizing radiation. S C Tjong (Ed.). Advances in Biomedical Sciences and Engineering. Bentham Science Publishers, 2009, p. 204-262.
- Morgan WF, Sowa MB. Non-targeted effects induced by ionizing radiation: Mechanisms and potential impact on radiation induced health effects. Cancer Lett. 2015;356:17-21. doi:10.1016/j.canlet.2013.09.009. PMID:24041870
- Takahashi K, Yamanaka S. Induction of pluripotent stem cells from mouse embryonic and adult fibroblast cultures by defined factors. Cell. 2006;126:663-76. doi:10.1016/j.cell.2006.07.024. PMID:16904174
- Takahashi K, Tanabe K, Ohnuki M, Narita M, Ichisaka T, Tomoda K, Yamanaka S. Induction of pluripotent stem cells from adult human fibroblasts by defined factors. Cell. 2007;131:861-72. doi:10.1016/j.cell.2007.11.019. PMID:18035408
- Li Y, Shen Z, Shelat H, Geng YJ. Reprogramming somatic cells to pluripotency: a fresh look at Yamanaka's model. Cell Cycle. 2013;23:3594-8. doi:10.4161/cc.26952
- Nakagawa M, Koyanagi M, Tanabe K, Takahashi K, Ichisaka T, Aoi T, Okita K, Mochiduki Y, Takizawa N, Yamanaka S. Generation of induced pluripotent stem cells without Myc from mouse and humanfibroblasts. Nat Biotechnol. 2008;1:101-6.
- Zhou H, Wu S, Joo JY, Zhu S, Han DW, Lin T, Trauger S, Bien G, Yao S, Zhu Y, et al. Generation of induced pluripotent stem cells using recombinant proteins. Cell Stem Cell. 2009;5:381-4. doi:10.1016/j.stem.2009.04.005
- Anwar MA, Kim S, Choi S. The triumph of chemically enhanced cellular reprogramming: a patent review. Expert Opin Ther Pat. 2016;26(2):265-80. doi:10.1517/13543776.2016.1118058. PMID:26593376
- Esteban MA, Wang T, Qin B, Yang J, Qin D, Cai J, Li W, Weng Z, Chen J, Ni S, et al. Vitamin C enhances the generation of mouse and human induced pluripotent stem cells. Cell Stem Cell. 2010;6(1):71-9. doi:10.1016/j.stem.2009.12.001. PMID:20036631
- Xu X, Wang Q, Long Y, Zhang R, Wei X, Xing M, Gu H, Xie X. Stress-mediated p38 activation promotes somatic cell reprogramming. Cell Res. 2013;23(1):131-41. doi:10.1038/cr.2012.143. PMID:23044805
- Wang Q, Xu X, Li J, Liu J, Gu H, Zhang R, Chen J, Kuang Y, Fei J, Jiang C, et al. Lithium, an anti-psychotic drug, greatly enhances the generation of induced pluripotent stem cells. Cell Res. 2011;21(10):1424-35. doi:10.1038/cr.2011.108. PMID:21727907
- Baek S, Quan X, Kim S, Lengner C, Park JK, Kim J. Electromagnetic fields mediate efficient cell reprogramming into a pluripotent state. ACS Nano. 2014;8(10):10125-38. doi:10.1021/nn502923s. PMID:25248035
- Lagadec C, Vlashi E, Della Donna L, Dekmezian C, Pajonk F. Radiation-induced reprogramming of breast cancer cells. Stem Cells. 2012;30(5):833-44 doi:10.1002/stem.1058. PMID:22489015
- Gao X, Sishc BJ, Nelson CB, Hahnfeldt P, Bailey SM, Hlatky L. Radiation-induced reprogramming of pre-senescent mammary epithelial cells enriches putative CD44(+)/CD24(-/low) stem cell phenotype. Front Oncol. 2016;6:138 doi:10.3389/fonc.2016.00138. PMID:27379202
- Mosteiro L, Pantoja C, Alcazar N, Marión RM, Chondronasiou D, Rovira M, Fernandez-Marcos PJ, Muñoz-Martin M, Blanco-Aparicio C, Pastor J, Gómez-López G, De Martino A, Blasco MA, Abad M, Serrano M. Tissue damage and senescence provide critical signals for cellular reprogramming in vivo. Science. 2016;25:354(6315):aaf4445
- Chan EM, Ratanasirintrawoot S, Park IH, Manos PD, Loh YH, Huo H, Miller JD, Hartung O, Rho J, Ince TA, et al. Live cell imaging distinguishes bona fide human iPS cells from partially reprogrammed cells. Nat Biotechnol. 2009;27:1033-1037. doi:10.1038/nbt.1580. PMID:19826408
- Cho YH, Kim SY, Woo HD, Kim YJ, Ha SW, Chung HW. Delayed numerical chromosome aberrations in human fibroblasts by low dose of radiation. Int J Environ Res Public Health. 2015;12(12):15162-72. doi:10.3390/ijerph121214979. PMID:26633443
- Hotta A, Cheung AY, Farra N, Vijayaragavan K, Séguin CA, Draper JS, Pasceri P, Maksakova IA, Mager DL, Rossant J, et al. Isolation of human iPS cells using EOS lentiviral vectors to select for pluripotency. Nat Methods. 2009;6(5):370-6. doi:10.1038/nmeth.1325. PMID:19404254
- Martí M, Mulero L, Pardo C, Morera C, Carrió M, Laricchia-Robbio L, Esteban CR, Izpisua Belmonte JC. Characterization of pluripotent stem cells. Nat Protoc. 2013;8:223-53. doi:10.1038/nprot.2012.154. PMID:23306458
- Little JB. Radiation-induced genomic instability. Int J Radiat Biol. 1998;74:663-71. doi:10.1080/095530098140925. PMID:9881710
- Rogakou EP, Pilch DR, Orr AH, Ivanova VS, Bonner WM. DNA double-stranded breaks induce histone H2AX phosphorylation on serine 139. J Biol Chem. 1998;273:5858-68. doi:10.1074/jbc.273.10.5858. PMID:9488723
- Tartier L, Gilchrist S, Burdak-Rothkamm S, Folkard M, Prise KM. Cytoplasmic irradiation induces mitochondrial-dependent 53BP1 protein relocalization in irradiated and bystandercells. Cancer Res. 2007;67:5872-9. doi:10.1158/0008-5472.CAN-07-0188. PMID:17575156
- Debacq-Chainiaux F, Erusalimsky JD, Campisi J, Toussaint O. Protocols to detect senescence-associated beta-galactosidase (SA-betagal) activity, a biomarker of senescent cells in culture and in vivo. Nat Protoc. 2009;4(12):1798-806. doi:10.1038/nprot.2009.191. PMID:20010931
- Rodier F, Coppé JP, Patil CK, Hoeijmakers WA, Muñoz DP, Raza SR, Freund A, Campeau E, Davalos AR, Campisi J. Persistent DNA damage signalling triggers senescence-associated inflammatory cytokinesecretion. Nat Cell Biol. 2009;11(8):973-9. doi:10.1038/ncb1909. PMID:19597488
- Banito A, Rashid ST, Acosta JC, Li S, Pereira CF, Geti I, Pinho S, Silva JC, Azuara V, Walsh M, et al. Senescence impairs successful reprogramming to pluripotent stem cells. Genes Dev. 2009;23(18):2134-9. PMID:19696146
- Adiwinata Pawitan J. Prospect of induced pluripotent stem cell genetic repair to cure genetic diseases. Stem Cells Int. 2012;2012:498197. PMID:22448173
- George AL, Boulanger CA, Smith GH. Telomerase and estrogen-sensing activities are essential for continued mammary growth in vivo but dispensable for “reprogramming” neural stem cells. Aging. 2016;8(7):1353-63. PMID:27347776
- Hung SS, Van Bergen NJ, Jackson S, Liang H, Mackey DA, Hernandez D, Lim SY, Hewitt AW, Trounce I, Pebay A, et al. Study of mitochondrial respiratory defects on reprogramming to human induced pluripotent stem cells. Aging. 2016;8(5):945-57. PMID:27127184
- Buganim Y, Faddah DA, Jaenisch R. Mechanisms and models of somatic cell reprogramming. Nat Rev Genet. 2013;14:427-439. PMID:23681063
- Mothersill C, Seymour CB. Are epigenetic mechanisms involved in radiation-induced bystander effect? Front Genet. 2012;3:74. PMID:22629281
- Kim HJ, Jeong J, Park S, Jin YW, Lee SS, Lee SB, Choi D. Establishment of hepatocellular cancer induced pluripotent stem cells using a reprogramming technique. Gut Liver. 2017;11(2):261-9
- Lee SB, Seo D, Choi D, Park KY, Holczbauer A, Marquardt JU, Conner EA, Factor VM, Thorgeirsson SS. Contribution of hepatic lineage stage-specific donor memory to the differential potential of induced mouse pluripotent stem cells. Stem Cells. 2012;30(5):997-1007