ABSTRACT
Apolipoprotein CIII (ApoCIII) has been shown to be associated with the inflammatory response, but the mechanism of its inflammatory effects remains unclear. Because vascular endothelial cells (VECs) play a key role in the development of inflammation, the present study was performed to investigate inflammatory mechanisms induced by ApoCIII in VECs. In this study, we screened differentially expressed genes (DEGs) using RNA-sequencing. The results identified 390 up-regulated genes and 257 down-regulated genes. We performed GO functional classification and KEGG pathway analysis for DEGs. Analysis of sequencing data showed that 21 genes were related to the MAPK pathway. Finally, we investigated whether ApoCIII regulates the expression of pro-inflammatory cytokines via MAPK signaling pathway. The results showed that ApoCIII increased the expression levels of IL-6, TNF-α, VCAM-1 and ICAM-1 in VECs. ApoCIII activated the phosphorylation of ERK1/2 and p38 MAPK. An inhibitor of ERK1/2 and p38 MAPK decreased the protein levels of IL-6 and TNF-α. Our findings demonstrate that ApoCIII induces pro-inflammatory cytokine production in VECs via activation of ERK1/2 and p38 MAPK phosphorylation.
Introduction
Atherosclerosis is an inflammatory disease associated with hypertriglyceridemia and vascular endothelial cell dysfunction.Citation1,Citation2 VECs are the main component of the vascular endothelium. VECs maintain the integrity of the vascular intima and the function of blood vessels. Under normal physiological conditions, VECs regulate the relaxation and contraction of blood vessels, blood coagulation and anticoagulation, cell adhesion, and the proliferation of vascular smooth muscle cells.Citation3 Under pathological conditions, VECs become activated. The activation of VECs contributes to inflammation and formation of the thrombosis. Activated VECs release large amounts of inflammatory cytokines and adhesion molecules, such as IL-6, TNF-α, VCAM-1 and ICAM-1.Citation4,Citation5 These cytokines play a key role in arterial inflammation, leading to endothelial dysfunction, thereby resulting in a variety of diseases.Citation6
Apolipoprotein CIII (ApoCIII), a 79-amino acid peptide, is synthesized by the liver and intestine. ApoCIII mainly resides on the surface of very-low-density lipoprotein (VLDL) and high-density lipoprotein (HDL).Citation7,Citation8 ApoCIII inhibits the activity of lipoprotein lipase and the metabolism and clearance of hepatic triglyceride-rich lipoproteins.Citation9,Citation10 One important role of ApoCIII is to modulate plasma lipid concentrations. The plasma concentration of ApoCIII is elevated in patients with hypertriglyceridemia and coronary heart disease.Citation11,Citation12 Many studies have demonstrated that ApoCIII contributes to atherosclerosis. Overexpression of ApoCIII in mice and rabbits causes hypertriglyceridemia, which is related to atherosclerosis.Citation13,Citation14 ApoCIII induces apoptosis of INS-IE cells by activating the p38 MAPK and ERK1/2 pathways.Citation15 Although ApoCIII regulates the expression of VCAM-1 and ICAM-1 in VECs, the mechanism of ApoCIII-induced inflammation in porcine VECs is unclear.
In this study, we simulated the process of ApoCIII-induced inflammation in VECs by adding ApoCIII to cultured VECs. We then explored the key inflammatory cytokines and molecular pathways of ApoCIII-induced cellular inflammation by deep-sequencing. According to the sequencing analysis, the MAPK pathway was determined to be related to porcine vascular endothelial cell inflammation. We identified that ApoCIII induces the expression of pro-inflammatory cytokines via the ERK1/2 and p38 MAPK signaling pathways. These findings may provide a theoretical basis for further understanding the molecular mechanisms of vascular endothelial cell inflammation by ApoCIII.
Results
Isolation, culture and identification of VECs
The morphology of porcine VECs is polygonal with a short spindle shape. After two days, the cells formed clusters (). Cells were fused into a single layer, which showed typical cobblestone-like features after eight days in culture (). VECs were identified by immunofluorescence staining. Green fluorescence was observed in the cytoplasm around the nucleus (). The purity of cells reached > 99%. shows that the morphology of VECs changed from the regular polygonal shape to the spindle shape after ApoCIII treatment.
Figure 1. The isolation, culture and identification of porcine vascular endothelial cells. (a) The morphology of porcine vascular endothelial cells after two days cultured. (b) The morphology of cells after eight days cultured. (c) The vascular endothelial cells were identified by immunofluorescent staining. (d) The morphology of vascular endothelial cells without ApoCIII treatment. (e) The morphology of vascular endothelial cells changed after ApoCIII treatment.
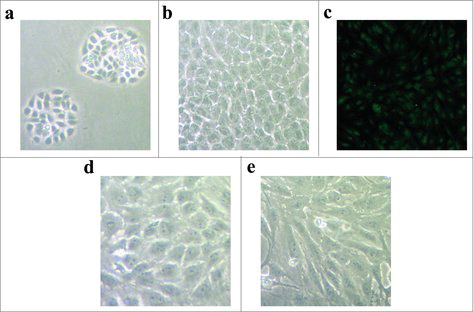
Effect of ApoCIII on IL-6 and TNF-α expression in VECs
Pro-inflammatory cytokines (including IL-6 and TNF-α) play key roles in the inflammatory process. After treatment with different concentrations of ApoCIII, we detected the expression levels of IL-6 and TNF-α by ELISA. shows that ApoCIII increased the protein levels of IL-6 () and TNF-α () in a dose-dependent manner in VECs. The levels of IL-6 and TNF-α increased significantly at concentrations of 50–100 μg/ml ApoCIII.
Figure 2. ApoCIII induces pro-inflammatory cytokines IL-6 and TNF-α expression in vascular endothelial cells. Vascular endothelial cells were treated with 0–100μg/ml ApoCIII. (a-b) The expression of IL-6 and TNF-α were detected by ELISA (n = 3). Error bars represent mean± s.e.m. *P < 0.05, **P < 0.01.
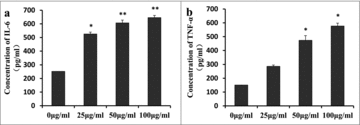
Effect of ApoCIII on VCAM-1 and ICAM-1 expression in VECs
Adhesion molecules (including VCAM-1 and ICAM-1) play important roles in the occurrence of inflammation by mediating the adhesion of mononuclear leucocytes to the intima of the blood vessel wall. To identify whether ApoCIII effects on the expression of adhesion molecules, we measured the levels of VCAM-1 and ICAM-1 using RT-qPCR. shows that the mRNA levels of VCAM-1 () and ICAM-1 () increased significantly at concentrations of 50–100 μg/ml ApoCIII. A concentration of 50 μg/ml ApoCIII was chosen as the concentration for subsequent experiments.
RNA-Seq analysis
To characterize the whole transcriptome of VECs following ApoCIII activation, we stimulated VECs with ApoCIII (50 μg/ml) for 16 h. And then we used high-throughput sequencing system to sequence the gene expression libraries in VECs. We obtained almost the same number of clean reads. These clean reads were mapped to genome reference, the mapping rates was 80.54% with ApoCIII (−) library and 80.30% with ApoCIII (+) library (). The higher mapping rates indicating that the closer the genetic relationship the samples and the genome reference.
Table 1. Primers of real-time PCR.
Table 2. Summary statistics of the sequencing.
Gene ontology analysis of DEGs
To understand the regulatory mechanism of inflammation induced by ApoCIII, we screened for DEGs induced by ApoCIII. The results showed 647 significant differential expression genes between the treatment and control groups, including 390 up-regulated genes and 257 down-regulated genes. To recognize the main biological functions of DEGs in VECs following ApoCIII treatment, we used 647 significant DEGs to GO functional classification The GO functional classification divided the DEGs into three categories (biological processes, cellular components and molecular functions, respectively), within these categories, the metabolic process and immune system process were distributed in the biological process category, binding was distributed in molecular function category, and cell and cell part were distributed in the cellular component category (). Following GO annotation on the DEGs, we found that many DEGs were related to the function of the cytokine activity and immune response, etc. Among these DEGs, we found several genes related to inflammation, cell adhesion and metabolic, including PTX3, IL1A, CD14, CCL2, HSP70, PTGS2, VEGFA, WIF1, CTNNAL1 and T GFB2 ().
Figure 4. GO functional classification of DEGs. GO functional classification for DEGs was analyzed by WEGO software.
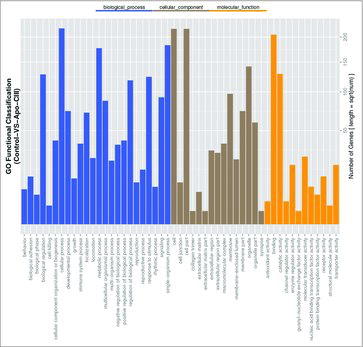
Table 3. Expression levels of DEGs in two groups.
KEGG pathway analysis of DEGs
To confirm the significantly enriched signal transduction pathways, we performed pathway enrichment analysis of DEGs. The top 20 enriched pathways are shown in . showed several enriched pathway terms. The ‘Focal adhesion’ pathway was enriched the most DEGs, followed by ‘cytokine-cytokine receptor interaction’ and ‘MAPK signaling pathway’. Among these pathways, a total of 21 DEGs were enriched in the MAPK signaling pathway. These results indicate the MAPK pathway might play a key role in the effects of ApoCIII.
Figure 5. KEGG pathway enrichments of DEGs. The x-axis represents the number of DEGs, and the y-axis is the p-value.
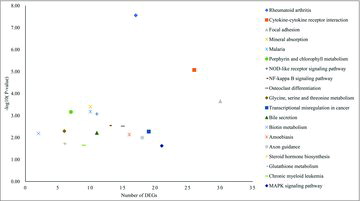
Table 4. Pathway enrichment analysis of the DEGs.
Validation of DEGs data by RT-qPCR
To validate the DEGs identified by sequencing, we randomly selected 9 genes from the significant DEGs including 7 up-regulated genes and 2 down-regulated genes. The seven up-regulated genes were as follows: toll-like receptor 10 (TLR10), IL1A, CXCL2, CCL2, PTX3, PIK3CG, and NF-κB. The two down-regulated genes were catenin CTNNAL1 and TGFB2. The data are presented as fold changes (log2) in gene expression. indicates that the gene expression levels based on sequencing and RT-qPCR data are consistent. It is confirmed that the sequencing data are reliable.
ApoCIII-regulated expression of pro-inflammatory cytokines via ERK1/2 and p38 MAPK pathways in VECs
The MAPK signaling pathways are closely related to the regulation of the inflammatory response. Therefore, we investigated the effects of ApoCIII on the activation of phospho-ERK and phospho-p38 MAPK. ApoCIII induced the phosphorylation of ERK1/2 and p38 MAPK and up-regulated the levels of pro-inflammatory cytokines. To investigate whether ApoCIII regulates the expression of pro-inflammatory cytokines via ERK1/2 and p38 MAPK, we pretreated the cells with the ERK1/2 inhibitor PD98059 and the p38 MAPK inhibitor SB203580 prior to stimulation with ApoCIII. These inhibitors completely blocked the increase in pro-inflammatory cytokine expression induced by ApoCIII (). These results suggest that the ERK1/2 and p38 MAPK pathways mediate the up-regulation of pro-inflammatory cytokine expression induced by ApoCIII.
Figure 7. ApoCIII induces pro-inflammatory cytokines expression is regulated by ERK1/2 and p38 MAPK pathways. Vascular endothelial cells were pretreated with PD98059 and SB203580 for 1h prior to incubation of ApoCIII for 16h. (a) The levels of ERK1/2, p38 MAPK and their phosphorylated counterparts were determined by western blotting (n = 3). (b-c) ELISA was used to determine the levels of IL-6 and TNF-α (n = 3). Error bars represent mean± s.e.m. *P < 0.05, **P < 0.01.
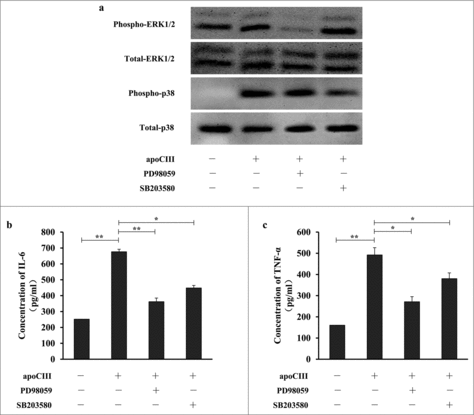
Discussion
ApoCIII is a small protein that increases the expression of adhesion molecules in VECs via the NF-κB pathway and enhances the adhesion of monocytes to endothelial cells, thereby causing the occurrence of atherosclerosis.Citation16,Citation17 Recent studies have shown that ApoCIII is associated with the inflammatory response, but the mechanism of its effects remains unclear. To provide a new insight into the genes and pathways associated with ApoCIII in VECs, we performed RNA-seq analysis to investigate the DEGs expression profile following ApoCIII treatment.
It has demonstrated that the activation of VECs causes the release of adhesion molecules and pro-inflammatory cytokines, including IL-6 and TNF-α. IL-6 and TNF-α contribute to inflammation in many types of cells and are involved in some cardiovascular diseases, such as atherosclerosis.Citation18 IL-6 is involved in the inflammatory reaction.Citation19 TNF-α promotes the production of chemokines, pro-inflammatory cytokines and adhesion molecules in VECs.Citation20 Our data suggest that ApoCIII significantly increases the levels of adhesion molecules and inflammatory cytokines in a dose-dependent manner in VECs, suggesting an effect of ApoCIII via the enhancement of vascular endothelial cell activation and the production of inflammatory mediators.Citation17 Wilms Kretschmer et al. demonstrated that VECs are activated during allergic reactions, accompanied by changes in the morphological features of VECs.Citation21 After ApoCIII treatment, the morphology of VECs changed from a polygonal to a spindle shape. The reason for this might be due to ApoCIII activating VECs.
Subsequently, RNA-seq analysis was used to screening the DEGs. Furthermore, GO functional classification and KEGG pathway analysis were performed to recognize the main biological functions of DEGs. GO analysis indicated that many DEGs are associated with the function of immune response and cytokine activity, etc. Several genes were found related to inflammation, including PTX3, IL1A, CD14, HSP70, CTNNAL1 and TGFB2, etc. PTX3 have been reported as acute inflammatory response protein, and the expression level of PTX3 increased with the cardiovascular damage, endotoxin shock and other diseases.Citation22,Citation23 CTNNAL1 play a key role in vascular smooth muscle cells mitotic and anti-apoptosis.Citation24 Kreiseder demonstrated that the activity of NF-κB, MAPK and AP-1 decreased with the down regulation of CTNNAL1.Citation25 These results suggest that ApoCIII may regulate inflammation in VECs by regulating these DEGs.
KEGG pathway analysis showed that the significant DEGs are related to the immune response and inflammatory pathways and that 21 DEGs are enriched in the MAPK signaling pathway. MAPKs are a family of serine/threonine protein kinases that are crucial for the development of inflammation.Citation26,Citation27 MAPK pathways regulate the processes of proliferation, differentiation, apoptosis and inflammation. Many studies have indicated that MAPKs are involved in the release of pro-inflammation cytokines such as TNF-α and IL-6.Citation28,Citation29 MAPK pathways are activated by transcription factors, cytoskeletal proteins, kinases and other enzymes.Citation27,Citation30 In this study, IL1A, CD14, HSP70 and TGFB2 were found to be related to MAPK pathway, suggesting that ApoCIII may induce inflammation via MAPK pathway Therefore, we focused on the MAPK pathway for the subsequent in-depth study.
Eri M Sol et al. demonstrated that ApoCIII regulates apoptosis of INS-IE cells via the phosphorylation of ERK1/2 and p38 MAPK.Citation15 To determine whether ApoCIII activates VECs via the MAPK pathway, we investigated the effects of ApoCIII on the phosphorylation of ERK1/2 and p38 MAPK in VECs. The results show that ApoCIII increased the phosphorylation of ERK1/2 and p38 MAPK. Researches have shown that the expression of heat stress protein (HSP27) and muscle cell enhancement factor 2 (MEF2) are up-regulated by p38 MAPK activation, which plays a key role in asthma, autoimmune disorders, inflammation, etc..Citation31,Citation32 These results suggest that the p38 MAPK pathway may play a key role in the activation of VECs and the release of inflammatory cytokines. The key function of the ERK1/2 pathway is to regulate cellular proliferation, differentiation, the inflammatory reaction, etc.Citation33 In this study, treatment with the ERK1/2 and p38 MAPK inhibitors completely blocked the increase in pro-inflammatory cytokine expression. Our results demonstrate that ApoCIII increases the expression of pro-inflammatory cytokines through the ERK1/2 and p38 MAPK pathways. There are reports showing that ApoCIII increases Lp-PLA2 mRNA and protein levels in human THP-1 cells,Citation34 but whether ApoCIII induces inflammation via Lp-PLA2 in VECs need to be investigated.
Taken together, the results of this study indicate that ApoCIII induce the inflammation in porcine VECs through a different mechanism, such as the MAPK pathway. These findings provide new insight into the role of ApoCIII in the inflammatory pathway.
Materials and methods
Experimental animals
Junmu-1 pigs were provided by the Protospecies Pig Farm of Jilin University. All animal experiments were conducted according to the guidelines of the Jilin University Institutional Animal Care and Use committee.
Cell isolation and culture
Porcine aorta was isolated under sterile conditions. The aorta was then repeatedly washed with PBS containing penicillin and streptomycin to remove impurities. The intima of vascular was turned outward. The two vascular ends were ligated with sterile cotton. The vascular endocardium was then digested with type I collagen enzyme (1 g/L) at 37°C for 30 min. The digested lysate was placed in a centrifuge tube and centrifuged at 1000 rpm for 10 min. The precipitation was resuspended in DMEM/F12 medium (Gibco, USA) containing 10% fetal bovine serum (Bioind, Israel). Suspended cells were seeded onto 12-well plates and incubated at 37°C with 5% CO2. When cells had grown to 80% confluency, the medium was removed, and the medium containing 0 μg/ml, 25 μg/ml, 50 μg/ml or 100 μg/ml ApoCIII (Academy Bio-medical, USA) was added and then incubated at 37°C with 5% CO2 for 16 h.
Immunofluorescence
Porcine VECs were cultured in 24-well plates for 24 h and washed three times with PBS. Cells were then fixed with 4% paraformaldehyde solution at room temperature for 15 min and rinsed twice with PBS. After permeabilizing with 0.1% Triton X-100 in PBS for 15 min on ice, cells were incubated with blocking buffer (1% BSA diluted with PBS) at 37°C for 2 h. The cells were then incubated with rabbit anti-Factor VIII-related antigen (1:100; Boster, China) overnight at 4°C in a dark humidity chamber. Cells were washed with PBS and incubated with fluorescein-conjugated secondary antibodies in a dark humidity chamber at 4°C for 1 h. The FITC-labeled goat anti-rabbit IgG (1:50; Boster, China) was used as the secondary antibody. After washing with PBS, cells were counterstained with DAPI for nuclear visualization. Cells were observed using a fluorescence microscope, and photomicrograph images were captured using the same exposure.
RT-PCR and Real-Time quantitative PCR
Total RNA was extracted from cells using TRIzol® (Roche, Germany). The concentration of total RNA was determined using a Nanodrop 2000 spectrophotometer. RNA was treated with DNase, and 0.5-1 μg of total RNA was reverse-transcribed into cDNA using the FastQuant RT Kit (Tiangen, China). The mRNA levels of various genes were determined by real-time quantitative PCR (RT-qPCR) using SuperReal PreMix Plus (Tiangen, China). Relative expression levels were calculated using the 2−ΔΔCT method. All values were normalized to the expression level of GAPDH, and each experiment was repeated three times. The primers used are listed in .
Quantification of pro-inflammatory cytokines by ELISA
The levels of pro-inflammatory cytokines in the culture medium (IL-6 and TNF-α) were determined using an ELISA kit (mlbio, China) according to the manufacturer's instructions.
RNA-seq and sequencing data analysis
Total RNA was isolated from VECs treated with 0 μg/ml and 50 μg/ml ApoCIII using TRIzol®. RNA was treated with DNase I to eliminate potential DNA contamination. To construct an RNA library and for deep-sequencing, equal quantities of RNA from either the three parallel control or the three parallel ApoCIII-treated vascular endothelial cell samples were pooled. Library products were sent for sequencing on an Illumina HiSeq™ 2000 sequencing system. The sequencing data were analyzed to screen for differentially expressed genes (DEGs) and for deep-sequencing analysis based on DEGs including Gene Ontology (GO) and KEGG pathway enrichment analyses.
Western blot
Proteins were extracted in cell lysis buffer (Beyotime, China) for 30 min on ice, and then centrifuged at 13,000 rpm for 5 min at 4°C. Protein concentration was determined by a BCA protein assay kit (Beyotime, China). 30 μg protein were separated by 12% SDS-PAGE and then transferred to polyvinylidene difluoride (PVDF) membranes (Millipore, USA). The membranes were blocked with 5% BSA at room temperature and incubated with the following primary antibodies: ERK1/2 (1:1000), phospho-ERK1/2 (1:1000), p38 MAPK (1:1000), or phospho-p38 MAPK (1:1000) (Beyotime, China). The membranes were washed in TBS-T and then incubated with horseradish peroxidase (HRP)-conjugated secondary goat anti-rabbit (1:2000; EarthOx Life Sciences, USA) or goat anti-mouse (1:2000; EarthOx Life Sciences, USA) antibodies for 2 h. The membranes were washed three additional times in TBS-T. Immunoreactive bands were detected as chemiluminescence on X-ray film using the Immobilon Western Chemiluminescent HRP Substrate Kit (Millipore, USA).
Abbreviations
IL6 | = | interleukin-6 |
TNF-α | = | tumor necrosis factor-α |
VCAM-1 | = | vascular cell adhesion molecule 1 |
ICAM-1 | = | intercellular cell adhesion molecule-1 |
Disclosure of potential conflicts of interest
No potential conflicts of interest were disclosed.
Conflict of interest
No authors in this manuscript declare any conflict of interest.
Grant sponsor
National Natural Science Foundation of China (31201761), Jilin Province Science and Technology Development Project (20170623033TC).
Additional information
Funding
References
- Galkina E, Ley K Immune and inflammatory mechanisms of atherosclerosis (*). Annu Rev Immunol. 2009;27:165-97. doi:10.1146/annurev.immunol.021908.132620. PMID:19302038
- Caron S, Staels B Apolipoprotein CIII: a link between hypertriglyceridemia and vascular dysfunction? Circ Res. 2008;103(12):1348-50. doi:10.1161/CIRCRESAHA.108.189860. PMID:19059836
- Furchgott RF, Zawadzki JV. The obligatory role of endothelial cells in the relaxation of arterial smooth muscle by acetylcholine. Nature. 1980;288(5789):373-6. doi:10.1038/288373a0. PMID:6253831
- Keller TT, Mairuhu AT, de Kruif MD, Klein SK, Gerdes VE, ten Cate H, Brandjes DP, Levi M, van Gorp EC. Infections and endothelial cells. Cardiovasc Res. 2003;60(1):40-8. doi:10.1016/S0008-6363(03)00354-7. PMID:14522405
- Wang X, Sun Z, Borjesson A, Andersson R. Inhibition of platelet-activating factor, intercellular adhesion molecule 1 and platelet endothelial cell adhesion molecule 1 reduces experimental pancreatitis-associated gut endothelial barrier dysfunction. Br J Surg. 1999;86(3):411-6. doi:10.1046/j.1365-2168.1999.01028.x. PMID:10201790
- Aikawa M, Sugiyama S, Hill CC, Voglic SJ, Rabkin E, Fukumoto Y, Schoen FJ, Witztum JL, Libby P. Lipid lowering reduces oxidative stress and endothelial cell activation in rabbit atheroma. Circulation. 2002;106(11):1390-6. doi:10.1161/01.CIR.0000028465.52694.9B. PMID:12221058
- Brewer HB, Jr., Shulman R, Herbert P, Ronan R, Wehrly K. The complete amino acid sequence of alanine apolipoprotein (apoC-3), and apolipoprotein from human plasma very low density lipoproteins. J Biol Chem. 1974;249(15):4975-84. PMID:4846755
- Jong MC, Hofker MH, Havekes LM. Role of ApoCs in lipoprotein metabolism: functional differences between ApoC1, ApoC2, and ApoC3. Arterioscler Thromb Vasc Biol. 1999;19(3):472-84. doi:10.1161/01.ATV.19.3.472. PMID:10073946
- Wang CS, McConathy WJ, Kloer HU, Alaupovic P. Modulation of lipoprotein lipase activity by apolipoproteins. Effect of apolipoprotein C-III. J Clin Invest. 1985;75(2):384-90. doi:10.1172/JCI111711. PMID:3973011
- Mann CJ, Troussard AA, Yen FT, Hannouche N, Najib J, Fruchart JC, Lotteau V, Andre P, Bihain BE. Inhibitory effects of specific apolipoprotein C-III isoforms on the binding of triglyceride-rich lipoproteins to the lipolysis-stimulated receptor. J Biol Chem. 1997;272(50):31348-54. doi:10.1074/jbc.272.50.31348. PMID:9395464
- Lee SJ, Moye LA, Campos H, Williams GH, Sacks FM. Hypertriglyceridemia but not diabetes status is associated with VLDL containing apolipoprotein CIII in patients with coronary heart disease. Atherosclerosis. 2003;167(2):293-302. doi:10.1016/S0021-9150(03)00007-8. PMID:12818412
- Mendivil CO, Rimm EB, Furtado J, Chiuve SE, Sacks FM. Low-density lipoproteins containing apolipoprotein C-III and the risk of coronary heart disease. Circulation. 2011;124(19):2065-72. doi:10.1161/CIRCULATIONAHA.111.056986. PMID:21986282
- Ito Y, Azrolan N, O'Connell A, Walsh A, Breslow JL. Hypertriglyceridemia as a result of human apo CIII gene expression in transgenic mice. Science. 1990;249(4970):790-3. doi:10.1126/science.2167514. PMID:2167514
- Ding Y, Wang Y, Zhu H, Fan J, Yu L, Liu G, Liu E. Hypertriglyceridemia and delayed clearance of fat load in transgenic rabbits expressing human apolipoprotein CIII. Transgenic Res. 2011;20(4):867-75. doi:10.1007/s11248-010-9467-5. PMID:21120692
- Sol EM, Sundsten T, Bergsten P. Role of MAPK in apolipoprotein CIII-induced apoptosis in INS-1E cells. Lipids Health Dis. 2009;8:3. doi:10.1186/1476-511X-8-3. PMID:19196457
- Kawakami A, Aikawa M, Alcaide P, Luscinskas FW, Libby P, Sacks FM. Apolipoprotein CIII induces expression of vascular cell adhesion molecule-1 in vascular endothelial cells and increases adhesion of monocytic cells. Circulation. 2006;114(7):681-7. doi:10.1161/CIRCULATIONAHA.106.622514. PMID:16894036
- Kawakami A, Aikawa M, Nitta N, Yoshida M, Libby P, Sacks FM. Apolipoprotein CIII-induced THP-1 cell adhesion to endothelial cells involves pertussis toxin-sensitive G protein- and protein kinase C alpha-mediated nuclear factor-kappaB activation. Arterioscler Thromb Vasc Biol. 2007;27(1):219-25. doi:10.1161/01.ATV.0000249620.68705.0d. PMID:17038637
- Feve B, Bastard JP. The role of interleukins in insulin resistance and type 2 diabetes mellitus. Nat Rev Endocrinol. 2009;5(6):305-11. doi:10.1038/nrendo.2009.62. PMID:19399017
- Heinrich PC, Castell JV, Andus T. Interleukin-6 and the acute phase response. Biochem J. 1990;265(3):621-361133681. doi:10.1042/bj2650621. PMID:1689567
- Old LJ Tumor necrosis factor (TNF). Science. 1985;230(4726):630-2. doi:10.1126/science.2413547. PMID:2413547
- Willms-Kretschmer K, Flax MH, Cotran RS. The fine structure of the vascular response in hapten-specific delayed hypersensitivity and contact dermatitis. Lab Invest. 1967;17(3):334-49. PMID:6051014
- Suliman ME, Yilmaz MI, Carrero JJ, Qureshi AR, Saglam M, Ipcioglu OM, Yenicesu M, Tong M, Heimburger O, Barany P, et al. Novel links between the long pentraxin 3, endothelial dysfunction, and albuminuria in early and advanced chronic kidney disease. Clin J Am Soc Nephrol. 2008;3(4):976-85. doi:10.2215/CJN.03960907. PMID:18417746
- Dubin R, Shlipak M, Li Y, Ix J, de Boer IH, Jenny N, Peralta CA. Racial differences in the association of pentraxin-3 with kidney dysfunction: the Multi-Ethnic Study of Atherosclerosis. Nephrol Dial Transplant. 2011;26(6):1903-8. doi:10.1093/ndt/gfq648. PMID:21079193
- Bear MD, Li M, Liu Y, Giel-Moloney MA, Fanburg BL, Toksoz D. The Lbc Rho guanine nucleotide exchange factor alpha-catulin axis functions in serotonin-induced vascular smooth muscle cell mitogenesis and RhoA/ROCK activation. J Biol Chem. 2010;285(43):32919-26. doi:10.1074/jbc.M109.062513. PMID:20696764
- Kreiseder B, Holper-Schichl YM, Muellauer B, Jacobi N, Pretsch A, Schmid JA, de Martin R, Hundsberger H, Eger A, Wiesner C. Alpha-catulin contributes to drug-resistance of melanoma by activating NF-kappaB and AP-1. PLoS One. 2015;10(3):e0119402. doi:10.1371/journal.pone.0119402. PMID:25793618
- Widmann C, Gibson S, Jarpe MB, Johnson GL. Mitogen-activated protein kinase: conservation of a three-kinase module from yeast to human. Physiol Rev. 1999;79(1):143-80. PMID:9922370
- Fecher LA, Amaravadi RK, Flaherty KT. The MAPK pathway in melanoma. Curr Opin Oncol. 2008;20(2):183-9. doi:10.1097/CCO.0b013e3282f5271c. PMID:18300768
- Dai JN, Zong Y, Zhong LM, Li YM, Zhang W, Bian LG, Ai QL, Liu YD, Sun J, Lu. D Gastrodin inhibits expression of inducible NO synthase, cyclooxygenase-2 and proinflammatory cytokines in cultured LPS-stimulated microglia via MAPK pathways. PLoS One. 2011;6(7):e21891. doi:10.1371/journal.pone.0021891. PMID:21765922
- Ajmone-Cat MA, De Simone R, Nicolini A, Minghetti L. Effects of phosphatidylserine on p38 mitogen activated protein kinase, cyclic AMP responding element binding protein and nuclear factor-kappaB activation in resting and activated microglial cells. J Neurochem. 2003;84(2):413-6. doi:10.1046/j.1471-4159.2003.01562.x. PMID:12559004
- Qi M, Elion EA. MAP kinase pathways. J Cell Sci. 2005;118(Pt 16):3569-72. doi: 10.1242/jcs.02470 PMID:16105880
- Navas TA, Nguyen AN, Hideshima T, Reddy M, Ma JY, Haghnazari E, Henson M, Stebbins EG, Kerr I, O'Young G, et al. Inhibition of p38alpha MAPK enhances proteasome inhibitor-induced apoptosis of myeloma cells by modulating Hsp27, Bcl-X(L), Mcl-1 and p53 levels in vitro and inhibits tumor growth in vivo. Leukemia. 2006;20(6):1017-27. doi:10.1038/sj.leu.2404200. PMID:16617327
- Obata T, Brown GE¸ Yaffe MB. MAP kinase pathways activated by stress: the p38 MAPK pathway. Crit Care Med. 2000;28(4 Suppl):N67−77. doi:10.1097/00003246-200004001-00008. PMID:10807318
- Roskoski R, Jr. ERK1/2 MAP kinases: structure, function, and regulation. Pharmacol Res. 2012;66(2):105-43. doi:10.1016/j.phrs.2012.04.005. PMID:22569528
- Han X, Wang T, Zhang J, Liu X, Li Z, Wang G, Song Q, Pang D, Ouyang H, Tang X. Apolipoprotein CIII regulates lipoprotein-associated phospholipase A2 expression via the MAPK and NFkappaB pathways. Biol Open. 2015;4(5):661-5. doi:10.1242/bio.201410900. PMID:25836672