ABSTRACT
Inflammatory bowel disease (IBD) results from a chronic intestinal inflammation and tissue destruction via an aberrant immune-driven inflammatory response towards an altered gut microbiota. Dietary intervention is becoming an attractive avenue for the therapy of colitis because diet is a key determinant of the mucosal immune response. Quercetin (QCN) is the most common in nature and the major representative of dietary antioxidant flavonoids, which has been demonstrated to influence the progression of colitis. However, the underlying mechanism of QCN on intestinal immunomodulation remains unclear. Here, our study demonstrated dietary QCN could ameliorate experimental colitis in part by modulating the anti-inflammatory effects and bactericidal capacity of macrophages via Heme oxygenase-1 (Hmox1, HO-1) dependent pathway. It suggested that QCN might restore the proper intestinal host-microbe relationship to ameliorate the colitis via rebalancing the pro-inflammatory, anti-inflammatory and bactericidal function of enteric macrophages. Hence, modulating the function of intestinal macrophages with dietary administration of QCN to restore the immunological hemostasis and rebalance the enteric commensal flora is a potential and promising strategy for IBD therapy.
Introduction
Inflammatory bowel disease (IBD), comprising Crohn's disease and ulcerative colitis, is a chronic intestinal inflammatory condition caused by multiple factors. The etiology and pathogenesis of IBD are poorly understood, but immune dysregulation, barrier dysfunction, and a loss of immune tolerance toward enteric flora are thought to lead to an exacerbated immune response with an imbalance of inflammatory cytokines [Citation1,2]. In active IBD, innate immune cells, such as neutrophils, macrophages, and dendritic cells, are the main protagonists [Citation3]. Migration and activation of these cells into the target mucosal tissues depend on the expression of Th-1 and Th-2 cytokines, chemokines, and adhesion molecules [Citation4–6]. Macrophages are plastic cells whose phenotypes depend on the anatomical location of the cells, as well as on the physiological or pathological context [Citation7]. An imbalance in the functional subsets of macrophages was found to contribute to the development of colitis in mice [Citation8–11]. In dextran sodium sulfate (DSS) and interleukin (IL)-10 KO models of colitis, the intestinal macrophages were found to play opposing roles in experimental models of intestinal inflammation [Citation12,13]. Classically activated type (M1) macrophages are involved in intestinal tissue destruction during the pathogenesis of colitis, primarily by secreting proinflammatory cytokines such as interleukin (IL)-6, tumor necrosis factor (TNF)-α, and interferon (IFN)-γ. However, alternatively activated type (M2) macrophages, which mediate anti-inflammatory function and tissue reparation, are essential for the resolution of colitis by primarily expressing low levels of proinflammatory cytokines, but high levels of arginase 1 (Arg-1), FIZZ1, YM-1, and IL-10 [Citation14,15]. Thus, macrophage polarization is implicated in the pathogenesis of human and experimental colitis, suggesting that restoration of the proper function of macrophages might be a major requisite for the resolution of chronic intestinal inflammation [Citation16].
Microbial colonization is vital for the development and homeostasis of the intestinal immune system [Citation17]. Accumulating evidence revealed the profound changes in gut microbial ecology in IBD patients and mouse models. The intestinal microbiota has been identified as a key contributor to the development of IBD Citation18–20]. In the intestine, immune reactivity toward commensal flora must be restrained to prevent pathological inflammation [Citation21]. Of the immunological parameters involved, macrophages cooperate to promote tolerance toward commensal flora, while they permit reactivity to pathogens. An imbalance of immune defense and tolerance between intestinal microbiota and macrophages might initiate and promote the pathological process underlying IBD [Citation22]; hence, rebalancing the commensal microbiota and remodeling macrophage functions may serve as an effective strategy for IBD treatment.
Dietary antioxidant flavonoids that are ubiquitously present in vegetables and fruits are a family of polyphenolic compounds. Their potential prophylactic and therapeutic activity has been intensively studied in the last decade [Citation23,24]. The bioactivity of the antioxidation and inflammation alleviation in various organs [Citation25] partly account for the potential and promising therapeutic options for inflammatory bowel disease [Citation26,27]. Among the flavonoids, QCN (3,3′,4′,5,7-pentahydroxyflavone; quercetin) is the most commonly found in nature and it is the major representative of the flavonol subclass [Citation28], exerting both antioxidative and anti-inflammatory functions. When administered orally, QCN exhibits protective and beneficial effects on chronic intestinal inflammation [Citation29–31]. Moreover, the therapeutic effects of QCN have been confirmed in inflammatory disorders such as asthma, arthritis, and lung injury [Citation32,33]; however, the mechanism of dietary QCN involved in a chronic colitis model remains undetermined. Furthermore, the cell types involved in QCN-mediated protection have not yet been thoroughly investigated. Previously, the anti-inflammatory effects of QCN were speculated to be related to the expansion of CD4+ T cells in the murine colon [Citation34]. Here, we assessed the effect of QCN in the inflamed murine intestinal tract in a naïve T cell transfer model of colitis, as well as in an oral DSS colitis model. We showed that treatment with QCN led to a reduction in inflammation and to alterations in the composition of gut microbiota; these effects were dependent on HO-1 activity in intestinal macrophages.
Results
Administration of QCN reduces gut inflammation in colitic mice
To determine the effect of QCN on a T cell-dependent colitis model induced by the adoptive transfer of naive T cells into Rag1-deficient mice (Rag1–/– mice), QCN was orally administered at a dose of 10 mg/kg body weight for 7 weeks after transfer. As expected, 7 weeks after T cell reconstitution, the mice manifested clinical signs of colitis (). Systemic delivery of QCN significantly reduced the severity of chronic intestinal inflammation evidenced by histological inflammation scores, body weight/colon length ratios, mucosal wall thickness, and a loss of colonic goblet cells (). Flow cytometric analysis showed that QCN significantly reduced the percentage of CD4+ T cells (Supplementary Figure 1A) and the percentages of IFN-γ and TNF-α-positive CD4+ T cells in colonic lamina propria lymphocytes (cLP) and mesenteric lymph nodes (MLN) () at 45 days after T-cell transfer. We also found that QCN inhibited the expression of the T-cell activation markers CD69, CD40L, and 4-1BB, whereas it upregulated the expression of IL-10 (Supplementary Figure 1B). Real-time polymerase chain reaction (PCR) results confirmed that the expression of TNF-α, IFN-γ, IL-17A, and IL-6 were decreased and that IL-10 was enhanced in the colon of QCN-treated mice compared with vehicle-treated mice (). The enzyme-linked immunosorbent assay (ELISA) data demonstrated that the concentrations of TNF-α, IFN-γ, and IL-6 in the colon were significantly reduced after the administration of QCN compared with controls (Supplementary Figure 1C). The data suggested that QCN treatment could affect the profile of proinflammatory and anti-inflammatory cytokines.
Figure 1. QCN administration reduces gut inflammation in T-cell mediated colitic mice. Rag1–/– mice were given QCN orally at a dose of 10 mg/kg/body weight for 7 weeks following the adoptive transfer of naïve CD4+ T cells (0.5 × 106, injected intraperitoneally). (A) Representative histology staining of colon and histologic score. (B) Ratio of colon weight to length. (C) Colonic mucosal thickness. (D) Alcian blue staining of the colon. (E) Representative FACS plots of intracellular staining of IFN-γ and TNF-α in CD3+CD4+ T cells from colonic cLP and MLN. (F) Real-time PCR analysis of the expression of genes encoding pro- or anti-inflammatory cytokines in the colon from PBS- and QCN-treated mice with colitis. Data are represented as the mean ± SEM. *P<0.05, **P<0.01 (Student's t-test).
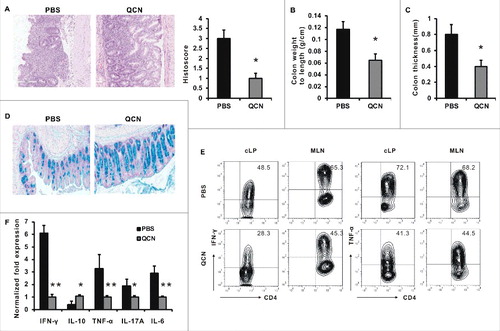
QCN alters the enteric flora and restores its hemostasis in colitic mice
To verify the effects of QCN on the commensal bacterium-mediated inflammation in T-cell-dependent colitis, we used 16S rDNA real-time PCR to examine four major intestinal bacteria: Bacteroidetes, Firmicutes, Actinobacteria, and Proteobacteria. Real-time PCR analysis revealed a significant decrease of 16S rDNA in the luminal bacterial flora of colons in QCN-treated mice compared to that in PBS-treated mice at day 45 of the CD4+ T-cell transfer ). We also observed that 5 weeks of QCN feeding increased the Bacteroidetes/Firmicutes ratio and led to a proportional decrease in the gram-negative Proteobacteria and gram-positive Actinobacteria ). Specifically, we observed an increase in the Bacteroides (BACT) and decreases in the segmented filamentous bacteria (SFB) groups in QCN-treated mice, as compared to phosphate buffered saline (PBS)-treated mice ). Interestingly, the number of E. coli was also decreased in the intestinal luminal specimens of QCN-treated mice ). To confirm whether QCN suppresses the translocation of microbiota to the basolateral surface of intestinal epithelial cells (IECs), we performed colon tissue staining with the antibody against lipopolysaccharide (LPS), which exists in gram-negative bacteria such as E. coli. A reduced LPS signal was observed in the basolateral side of the IECs after CD4+ T cell-mediated epithelial damage in QCN-treated mice compared with PBS-treated mice ).
Figure 2. QCN alters enteric flora in mice with T-cell-mediated colitis. (A) Real-time PCR analysis of 16S rDNA in the feces of colon at day 45 in PBS or QCN-treated Rag1–/– mice after T-cell transfer. (B) The relative abundance of fecal bacteria phylum (the exact percentage is indicated by the color bars) of colitic Rag1–/– mice with or without QCN treatment. (C) Real-time PCR analysis of 16s rDNA of indicated bacteria in feces of the colon at day 45 in PBS- or QCN-treated Rag1–/– mice after T-cell transfer. (D) LPS staining of the representative colons from colitic Rag1–/– mice with or without QCN treatment. Data are represented as the mean ± SEM. *P<0.05, **P<0.01 (Student's t-test).
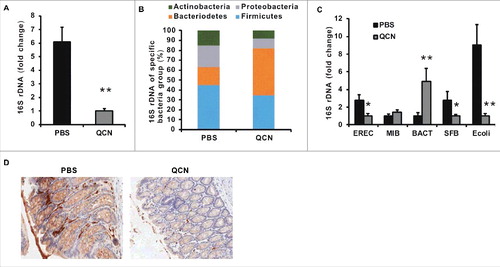
QCN suppresses colitis by inducing the anti-inflammatory effects of macrophages
Given the phagocytic nature of lamina propria (LP) macrophages [Citation35], we hypothesized that LP macrophages might contribute to restricting the access of commensal bacteria to the LP during an inflammatory condition of the intestines. To assess the influence of QCN on macrophage phenotypes in colitic mice, we compared our in vivo-derived colonic macrophages from QCN-treated animals with those from untreated mice, both in the inflammatory phase of colitis. In the T-cell transfer model of colitis, fluorescence-activated cell sorting (FACS) analysis of the pan-macrophage marker F4/80 and CD11b revealed a significant increase in the percentage of macrophages in both the small and large intestines of QCN-treated mice compared to that of PBS-treated mice ). The absolute number of CD11b+ macrophages isolated from the inflamed intestine of QCN-treated mice increased significantly when compared with that from the PBS-treated mice ). Flow cytometry phenotypic analysis further revealed that QCN treatment inhibited the expression of maturation markers CD86, CD80, CD40, and 4-1BBL ), which are molecules associated with T-cell activation and oral tolerance development. To gain insight into the CD11b+ macrophage genes induced as a result of QCN treatment, we performed gene expression profiling by real-time PCR. We found that QCN-derived CD11b+ macrophages expressed a higher level of genes associated with regulatory M2 macrophage signatures, such as Ym1, Fizz1, Arg1, TGF-β1, and IL-10 ), and there was also a concomitant increase in the mRNA associated with the antioxidation substrates, including GCLC, GCLM, HO-1, SRXN1, and NQO1 ). Collectively, these data showed that QCN is important for inducing the expression of mRNAs that encode the proteins required for anti-oxidation and anti-inflammation. Compared with the colitic mice that only received PBS, the secretion of proinflammatory cytokines TNF-α, IL-23, and IL-12 from the colonic macrophages of QCN-treated colitic mice was greatly reduced, whereas anti-inflammatory molecule IL-10 was increased ).
Figure 3. QCN induces the functional alteration of enteric macrophages in experimental colitis. (A–B) The frequency (A) and cell number (B) of CD11b+ macrophages isolated from the large intestine (LI) and small intestine (SI) in naïve CD4+ T-cell-mediated colitis. (C) Phenotypic characterization of macrophages from the colon in CD4+ T-cell-mediated colitis. (D–E) Real-time PCR for the expression of genes in CD11b+ F4/80+ sorted from pooled colonic LPL in CD4+ T-cell-mediated colitis. (F) Cytokine levels in the LPS-stimulated colonic macrophages that were collected at day 45 following T-cell transfer. (G–H) CFSE-labeled OT-II T cells were co-cultured with PBS or QCN-pretreated lamina propria macrophages and OVA peptide (5 µg/mL). Proliferation of CFSE-labeled CD4+ T cells (G) and the level of IFN-γ and TNF-α (H) in the supernatant of co-cultured cells were examined. T-cell activation molecules (I) were analyzed by FACS after 4 days in culture. Data (B–D, H) are presented as the mean ± SEM. *P<0.05, **P<0.01 (Student's t-test).
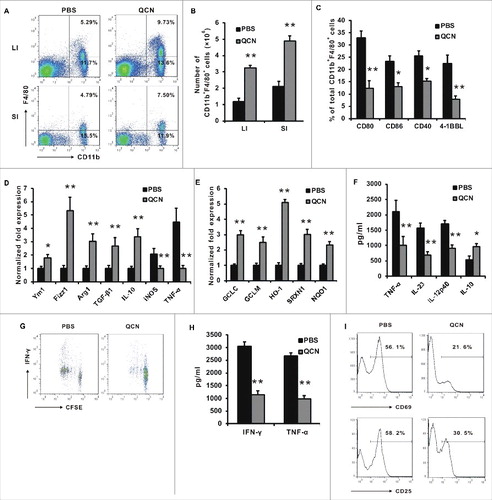
To assess the biological effects of QCN-treated macrophages to CD4+ T cells, naive CD4+ T cells from the MHC class II-restricted T-cell receptor (TCR) transgenic line specific for ovalbumin (OT-II) mice, loaded with carboxyfluorescein succinimidyl ester (CFSE), were co-cultured with colonic macrophages pretreated with QCN or PBS in the presence of ovalbumin (OVA). A significant decrease in the proliferation and secretion of TNF-α and IFN-γ ) by CD4+ T cells was detected in the QCN-pretreated group when compared with the PBS-pretreated group. FACS assay showed that CD69 and CD25, the T-cell activation markers, exhibited reduced expression on the CD4+ T cells of the QCN-pretreated group when compared with the PBS-pretreated group ). Collectively, it suggested that QCN could induce the regulatory phenotype of CD11b+ macrophages, which favor the inhibition of T-cell activation.
To demonstrate the protective effects of QCN on colitis that result from regulating the immunological function of macrophages, we depleted macrophages in mouse colons by injecting clodronate-containing liposomes (CDLs). The in vivo depletion of macrophages partially counteracted the therapeutic effects of QCN in a T-cell-induced colitic mouse model in terms of the colon weight/length ratio ), mucosal wall thickness ), and histological score ).
Figure 4. Macrophage depletion reduces the therapeutic effects of QCN on colitic mice. Mice transferred with CD4+ T cells were administrated with PBS or QCN and intrarectally administered CDLs or PBS. (A) Ratio of colon weight to length. (B) Colonic mucosal thickness. (C) Histologic score. Data (A–C) are presented as the mean ± SEM. *P < 0.05 (one-way ANOVA).
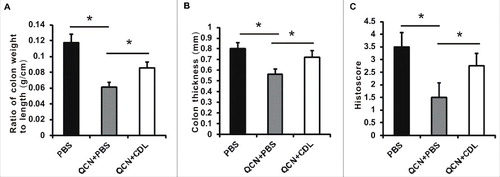
QCN enhances the anti-inflammatory and bactericidal effects of macrophages via the Nrf2/HO-1 pathway
It is well established that the expression of antioxidant-related genes is regulated by nuclear factor-erythroid 2-related factor 2 (Nrf2) [Citation36], including HO-1. HO-1 has attracted particular interest since it is finely upregulated by enteric microbiota and generates metabolites to enhance macrophage bactericidal activity and bacterial clearance in vivo [Citation37]. Our studies indicated that HO-1 induction in intestinal macrophages during QCN treatment is associated with decreased iNOS and M1 cytokine expression and increased IL-10 expression ) in mice with colitis. Hence, we speculated that QCN may directly affect macrophage responses via Nrf2/HO-1. Consistently, our data showed that QCN increased the expression of Nrf2 and HO-1 in the colon of mice with colitis ). In addition, stimulation with QCN also led to the accumulation of Nrf2 in the nucleus and enhanced the expression of HO-1 in macrophages ).
Figure 5. The HO-1 pathway involved in the effects of QCN on the immune regulation and bactericidal capacity of macrophages. (A) T-cell-transferred mice were administrated with PBS or QCN. Colonic lysates were prepared and analyzed for the expression of Nrf2 and HO-1 by Western blotting. (B) QCN induction of HO-1 protein expression in a time-dependent manner. BMDMs were treated with QCN (100 μM) for 8 hours, 12 hours, and 24 hours, and the expression of HO-1 and the nuclear Nrf2 protein was detected by Western blotting. (C) Hmox1 siRNA or Scr-transfected BMDMs were treated by PBS or QCN for 6 hours and stimulated by LPS (50 ng/mL) and IFN-γ (10 ng/mL) for 4 hours. Gene expression was examined with real-time PCR analysis. (D–E) Hmox1 siRNA or Scr-transfected BMDMs (D) or SnPP (30 μM)-treated BMDMs (E) were analyzed for their macrophage bactericidal activity against E. coli in the presence of PBS or QCN using a gentamicin protection assay. (F) Hmox1 siRNA or Scr-transfected BMDMs were incubated with PBS or QCN; the cells were then treated with LysoTracker (100 nmol/L) and exposed to E. coli. The cells were subsequently fixed and the nuclei were stained with 5 nmol/L of Topro-3 (Invitrogen, Carlsbad, CA, USA). LysoTracker-positive cells were calculated as a percentage of total cells. The data are presented as the mean ± SEM. *P<0.05, **P<0.01 (Student's t-test). (G) Bacterial titers in MLN were determined in PBS- or QCN-treated mice with T-cell-mediated colitis.
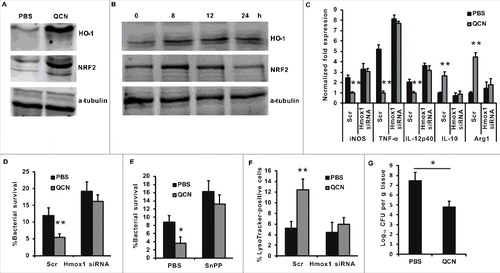
To clarify the role of macrophage HO-1 on QCN-induced anti-inflammation effects, bone marrow-derived macrophages (BMDMs) were infected with retroviral Hmox1 siRNA (named BMDMHmox1siRNA) or control scrambled siRNA (named BMDMScr), and they then induced M1 polarization with IFN-γ and LPS in the presence of QCN. The genes encoding M1 markers iNOS, TNF-α, and IL-12p40 were significantly suppressed and M2 markers IL-10 and Arg1 were significantly enhanced by QCN in BMDMScr cells ). Such effects of QCN on macrophage polarization were counteracted in BMDMHmox1siRNA cells ).
The gasotransmitter carbon monoxide (CO) is generated by the stress-responsive enzyme HO-1, which is highly induced in macrophages in response to bacterial infection [Citation38]. Based on our in vivo findings, we speculated that the HO-1/CO pathway might be involved in the QCN-enhanced bacteria clearance capacity of macrophages. Our data demonstrated that QCN increased bactericidal activity by erasing intracellular E. coli in the BMDMScr cell group, whereas it failed to do so in the BMDMHmox1siRNA cell group ). The fact that the QCN-strengthened bactericidal capacity of BMDMs was neutralized by Sn-protoporphyrin (SnPP), which could prevent endogenous CO formation, confirmed the participation of CO in the QCN-induced HO-1 pathway ).
Phagolysosomal maturation is an indicator of the bactericidal activity of macrophages. To further verify the ability of HO-1 to mediate the QCN-enhanced bacterial clearance of macrophages, BMDMs were incubated with LysoTracker® Red DND-99, a weak base that permeates cell membranes and fluoresces upon protonation in low-pH environments, to detect phagolysosome. Compared with PBS control, QCN significantly increased phagolysosomal formation in BMDMScr cells that were challenged with E. coli, but they failed in BMDMHmox1siRNA cells challenged with E. coli ).
Given the phagocytic nature of LP macrophages, and their significant induction in QCN-treated mice, we hypothesized that QCN-treated macrophages will enhance the clearance of commensal bacteria that gain access to MLNs. To test this, we analyzed the MLNs from these mice to assess them for signs of bacterial translocation. The total MLN cell suspensions cultured on LB agar plates revealed the presence of lower numbers of bacteria in mice after QCN treatment ).
Collectively, these data revealed that HO-1 and its bioactive product, CO, are involved in the QCN-induced bacterial clearance of macrophages.
QCN-treated macrophages suppress DSS-induced colitis via the HO-1 pathway
To further confirm that the HO-1 pathway is involved in the protective effects of QCN on colitis, QCN or PBS-treated BMDMs were transferred into mice at day 1 and day 4 after DSS administration. At day 6, mice transferred with QCN-treated BMDMs were significantly protected from colitis compared with those transferred with PBS-treated BMDMs ). In contrast to BMDMScr, the transfer of BMDMHmox1siRNA showed no detectable effect on modulating DSS-induced colitis ). The expression of proinflammatory cytokines IFN-γ, IL-17A, and TNF-α in the colon were downregulated following the transfer of QCN-treated BMDMScr than PBS-treated BMDMScr, while cytokine downregulations could not be detected when silencing HO-1 expression ). In addition, the number of E. coli was less pronounced in the colon from mice transferred with QCN-treated BMDMScr than in that of the PBS-treated BMDMScr transfer group. However, there is no significant difference between the number of E. coli between QCN-treated and PBS-treated BMDMHmox1siRNA transfer groups ).
Figure 6. QCN-treated macrophages suppress DSS-induced colitis via the HO-1 pathway. Hmox1 siRNA or Scr-transfected BMDMs were treated with a gut bacteria antigen (20 µg/mL) in the presence of PBS or QCN for 24–48 hours. Then, 3 × 106 cells were injected intravenously into C57BL/6 mice at days 1 and 4 with 2.5% DSS administration. (A) Body weight was measured at the indicated time points following the transfer of BMDMs. (B) Representative histology staining of the colon from BMDM recipient mice with DSS-induced colitis. (C) The colonic length of colitic mice. (D) The real-time PCR analysis of the expression of genes encoding cytokines in the colon from PBS- and QCN-treated mice with colitis was examined. (E) Real-time PCR analysis of 16S rDNA in the feces of colon in PBS- or QCN-treated mice after DSS was orally administered. Data are presented as the mean ± SEM. *P<0.05, **P < 0.01 (Student's t-test).
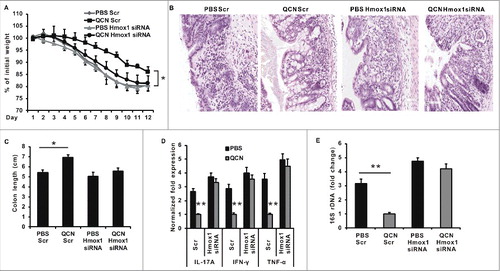
Discussion
In this study, we report that the treatment of QCN limits experimental chronic intestinal inflammation in mice by modifying the tissue cytokine milieu and inducing macrophage polarization and bacterial killing. The incidence of IBD has been growing over the past few decades and new therapeutic strategies are needed. A number of studies have investigated the possibility of antioxidant compounds, such as flavonoids, to restore and maintain intestinal epithelial health in several models of experimental colitis [Citation39,40]. Among these flavonoids, QCN reportedly exerts intestinal anti-inflammatory effects associated with the downregulation of the production of different inflammatory mediators, including cytokines (TNF-α, IL-1β), as well as reactive oxygen and nitrogen metabolites [Citation39–41]. Immune cells involved in inflammatory responses, such as monocytes [Citation42], macrophages [Citation43], and T cells [Citation34], are modulated by QCN through its inhibition of the proinflammatory transcription factors activating protein 1 (AP-1) and nuclear factor kB (NF-kB) [Citation43,44], or through the inhibition of IL-12 signaling, including the tyrosine phosphorylation of JAK2 and STAT3 [Citation34]. Recent work by Mascaraque et al [Citation45] demonstrated that one important target of rutin/QCN in colitis may be IFN-γ-producing cells, probably lymphocytes of the Th1 phenotype [Citation45]. However, the biologic impact of QCN on macrophages during intestinal inflammation has never been investigated. In this report, we found that QCN treatment enhanced the bacterial clearance and anti-inflammatory capacity of macrophages in the mouse colitis model of Rag1–/– mice transferred with CD4+-naïve T cells (). Depletion of macrophages with CDLs partially abolished these therapeutic effects of QCN ), suggesting that macrophages are the target cells of QCN.
Activated macrophages can be functionally divided into M1 and M2 in response to the different stimuli of the microenvironment [Citation46]. M1 macrophages mediate the host's defense from a variety of bacteria, protozoa, and viruses, whereas M2 macrophages regulate anti-inflammatory function and wound healing [Citation47]. Therefore, balancing the immune defense and the tolerance of macrophages is essential for intestinal hemostasis [Citation48]. Intestinal macrophages have long been considered an important, destructive force behind IBD inflammation through the production of proinflammatory cytokines and chemokines [Citation49,50]. Our data revealed that QCN induced the macrophages' preference for a M2 phenotype with low levels of co-stimulatory molecules ), a high expression of M2 signature genes ), and genes encoding antioxidation proteins ), the reduced secretion of proinflammatory cytokines, and a higher production of anti-inflammatory molecule IL-10 ). During the immune response, macrophages are vital regulators for helper CD4+ T cells. Our data showed that QCN-pretreated colonic macrophages could inhibit CD4+ T-cell activation ) and proliferation ), as well as inflammatory cytokine TNF-α and IFN-γ secretion ). QCN administration lessened the enteric bacteria flora ), increased the Bacteriodetes/Firmicutes ratio with a proportional decrease in the Gram-negative Proteobacteria and Gram-positive Actinobacteria ), increased the Bacteroides (Bact), decreased segmented filamentous bacteria (SFB) groups and E. coli ), and reduced the LPS distribution in the basolateral side of IECs ). These findings indicated that QCN were not only able to clear the harmful bacteria, but it restored the hemostasis of the enteric commensal flora. This interesting effect of QCN might partially result from the macrophages ). Increasing evidence revealed that intestinal microbes influence the host's immune development, immune responses, and susceptibility to human IBD, diabetes mellitus, and obesity. Conversely, host factors can affect microbes which, in turn, modulate disease susceptibility. Dysregulation of host–microbe interactions breaks the balance of the host defense and tolerance, leading to the development of intestinal inflammation and IBD[ Citation22]. Thus, our data suggested that QCN might restore the proper intestinal host-microbe relationship to ameliorate colitis by adjusting the balancing between the anti-inflammatory and bactericidal functions of enteric macrophages.
QCN administration enhanced Nrf2 and HO-1 expression in the colon tissues of colitic mice ), similar to what was found in a previous report where QCN was potent in the stimulation of Nrf2-induced HO-1 protein expression in murine microglial cells [Citation51]. The accumulation of NRF2 in the nucleus and the enhanced expression of HO-1 in macrophages treated with QCN ) suggested that the Nrf2/HO-1 pathway is involved in the biological effects of QCN on colitis. Silencing HO-1 expression resulted in decreased anti-inflammatory effects ), M2 polarization ), bactericidal capacity ), and phagolysosome formation ) of the QCN-treated macrophages; it also inhibited the aberrant bacterial translocation ). These results confirmed that HO-1 is critical for the signal transduction of QCN stimulation in intestinal macrophages. CO, downstream molecular of HO-1 pathway, mediated bacterial clearance and anti-inflammation cytokine production in macrophages [Citation38]. Inhibition of endogenous CO production with SnPP significantly blocks the bactericidal ability of LP macrophages from QCN-treated mice ), demonstrating that CO is a critical effector molecule of QCN-induced protection for colitic mice. Amelioration of DSS-induced colitis with QCN-treated BMDMs ) verified that macrophages mediate the protective effect of QCN. Silencing HO-1 in BMDMs impairs the protective function of QCN ), further demonstrating the role of the HO-1 pathway in QCN-mediated protection for colitis.
Hence, our study demonstrated that QCN could ameliorate experimental colitis, in part, by modulating the anti-inflammatory effects and bactericidal activity of macrophages via an HO-1 dependent pathway. The dietary administration of QCN to restore intestinal immune hemostasis and enteric commensal flora balance is a potential and promising strategy for IBD therapy.
Materials and methods
Animals and treatments
OT-II mice and Rag1–/– mice in a C57BL/6 background and C57BL/6 mice were purchased from Nanjing Biomedical Research Institute of Nanjing University (Nanjing, People's Republic of China). All animal protocols were approved by the Institutional Laboratory Animal Care and Use Committee at Soochow University (Suzhou, People's Republic of China).
Induction of colitis
Adoptive T-cell transfer model of chronic colitis. T-cell-mediated colitis was induced in Rag1–/– mice by adoptively transferring CD4+CD25−CD62L+ T cells that were sorted using a FACSAria II flow cytometer (BD Biosciences, San Jose, CA, USA). The Rag1−/– recipients were given 5 × 105 CD4+CD25−CD62L+ T cells via intraperitoneal injection, and the mice were euthanized at 6–7 weeks after transfer. Some recipient mice also orally received 10 mg/kg of body weight of QCN or PBS once every 3 days for 7 weeks after the transfer of CD4+CD25−CD62L+ T cells. The Disease Activity Index (DAI) and histology scores were determined as previously described [Citation52–55].
Chemically induced colitis model. Colitis was induced in 8- to 12-week-old C57BL/6J mice by the addition of 2.5% (wt/vol) DSS (36–50 KD molecular weight; MP Biomedicals, Solon, OH, USA) in their drinking water. For the transfer of BMDMs, BMDMHmox1siRNA or BMDMScr were treated with a gut bacterial antigen (20 µg/mL) for 48 hours in the presence of PBS or QCN. BMDMs were injected intravenously (3 × 106) into each recipient mouse at days 1 and 4 of DSS treatment. Body weights, stool consistency, and GI bleeding were monitored daily. Clinical scores and colonic damage scores were estimated, as detailed previously [Citation56]. Colons were collected immediately after sacrifice, and the mucosa was scraped to isolate total RNA or proteins.
Reagents, antibodies, and flow cytometry
QCN and LPS were purchased from Sigma-Aldrich Co. (St Louis, MO, USA). SnPP (30 μM) were purchased from Frontier Scientific Inc. (Logan, UT, USA). Fluorochrome-labelled antibodies were purchased from Biolegend, unless otherwise noted: IL-17A (TC11–18H10.1; eBioscience, San Diego, CA, USA); anti-IL-4 (11B11); anti-IL-10 (JES5–16E3, eBioscience); anti-IFN-γ (XMG1.2), anti-Foxp3 (FJK-16s, eBioscience); anti-CD11b (M1/70); anti-CD4 (RM4-5); anti-CD3 (145-2C11); anti-Gr-1 (RB6-8C5); anti-Ly6G (1A8); and anti-CD45 (30-F11). For the analysis of surface markers, the cells were stained in PBS containing 2% (wt/vol) BSA. The cells were stained with the appropriate mixture of antibodies. For the detection of intracellular cytokines, cells were first stimulated for 4 hours with 50 ng/mL of PMA and 1 µg/mL of ionomycin in the presence of Brefeldin A (5 µg/mL; all obtained from Sigma-Aldrich Co.), followed by staining for surface markers. The cells were then fixed and permeabilized using the Foxp3 Fix/Perm Buffer Set (eBioscience) and stained for intracellular cytokines. Flow cytometry data were acquired on FACSCalibur (BD Biosciences) and analyzed using FlowJo software (Tree Star, Inc., Ashland, OR, USA). Cell sorting was performed using FACSAria II.
Histology and immunohistochemistry
Tissue specimens were fixed in 10% formalin, dehydrated, and then embedded in paraffin; 2–4 μm-thick sections were stained with hematoxylin and eosin. For the immunofluorescence analysis, tissue sections were subjected to antigen retrieval by boiling the slides in Antigen Unmasking Solution (Vector Laboratories, Burlingame, CA, USA) for 10 minutes, according to the manufacturer's instructions. Sections were then blocked for 1 hour at 22°C with 5% BSA in PBS and incubated with antibodies against Ki67 (eBioscience), mouse monoclonal anti-E-cadherin, anti-CD11c, F4/80 and CD11b (BD Biosciences), and they were used at a dilution of 1/250, followed by Alexa488-conjugated anti-goat immunoglobulin (Ig)G or Alexa594-labelled anti-rabbit IgG (1:600; Invitrogen, Carlsbad, CA, USA). Tissues were counterstained with DAPI and images were captured on a Leica SP5 II confocal microscope (Leica Microsystems, Wetzlar, Germany). For the immunohistochemical analysis of dendritic cells or macrophages, OCT (Sakura Finetek)-embedded tissue cryosections (5 μm thick) were stained with CD11c or F4/80 (BM8; eBioscience).
16S rDNA genetic analysis of bacteria
DNA was extracted from the colon contents of QCN-treated and untreated mice, and analyzed by quantitative real-time PCR using general 16S rDNA primers (ACTCCTACGGGAGGCAGCAGT and ATTACCGCGGCTGCTGGC). The relative abundance of 16S rDNA for each targeted bacterial group was calculated by the ΔCT method and normalized to the amount of total 16S rDNA in the sample.
RNA extraction and PCR
Total RNA was prepared from isolated small intestinal epithelial cells using the RNeasy Mini Kit (Qiagen, Valencia, CA, USA) in accordance with the manufacturer's instructions. Total RNA (1 μg) was subjected to reverse transcription using random hexamers and Superscript II (Invitrogen), followed by quantitative PCR analysis. To quantify the genes of interest, cDNA samples were amplified in a LightCycler 480 Real-Time PCR System (Roche Diagnostics, Mannheim, Germany) with SYBR Green Master Mix (Invitrogen) and specific primers (Supplemental Table 1), according to the manufacturer's instructions. Fold changes in mRNA expression between treatments and controls were determined by the ΔCT method, as described [Citation57]. Error bars on the plots represent ± standard error of the mean (SEM), unless otherwise noted. The data were normalized to a GAPDH reference. All primers were purchased from Sangon Biotech (Shanghai, People's Republic of China).
ELISA
The quantity of IL-17A, IL-6, TNF-α, IL-10, and IFN-γ (eBioscience) were determined in culture supernatants, the small intestine, and colon using ELISA kits, according to the manufacturer's instructions. The sensitivity of the assay was <20 pg/mL.
Cells and cell culture conditions
Cells were maintained in DMEM supplemented with 10% fetal bovine serum (FBS) and 100 U/mL of penicillin/streptomycin. All cells were grown in a humidified atmosphere of 5% CO2 at 37°C. For macrophage–T-cell co-cultures, the macrophages were treated by PBS or QCN (100 µM) for 24 hours and washed. Then, 2 × 105 macrophages and 8 × 105 OT-II T cells were further mixed in the presence of cognate peptide (5 μg/mL; OVA). After 4 days of culture, live T cells were collected and stimulated with PMA (phorbol 12-myristate 13-acetate; 50 ng/mL) and ionomycin (1 µg/mL; Sigma-Aldrich Co.) plus brefeldin A (10 µg/mL; Sigma-Aldrich Co.) for intracellular cytokine staining or for mRNA analysis. mRNA was assessed by real-time PCR and supernatants were used for cytokine measurement by ELISA.
Isolation of Lamina propria lymphocytes (LPLs)
The method used to isolate LPLs has been previously described [Citation58]. In brief, fat tissues and PPs were removed from the small intestine. The intestine was opened and cut into pieces 1 cm long and incubated in an HBSS solution containing 5 mM EDTA and 10 mM Hepes for 30 minutes at 37°C with slow rotation (180 rpm min−1). Pieces were then further cut and incubated in an HBSS solution containing 0.5 mg ml−1 DNase I (Roche, Basel, Switzerland) and 1 mg/ml−1 of Collagenase type IV (Worthington Biochemical, Lakewood, NJ, USA). Finally, the solution containing digested tissue was passed through a 100 μm cell strainer and LPLs were recovered at the interface of the 40% and 80% Percoll (GE Healthcare, Pittsburgh, PA, USA) solutions. For flow cytometry analysis, the cells were labelled using standard procedures, as described above.
Isolation of gut bacteria antigens for in vitro cell culture
Fresh fecal content was collected and carefully resuspended in PBS (0.01 g/mL). The obtained suspension was centrifuged at 400 × g for 5 minutes to remove the larger particle from the bacteria. Bacterial suspensions were then lysed by physical disruption through sonication. The protein concentration of the lysate was quantified with the Bradford protein assay. We used 20 µg/mL of total GBA for in vitro stimulation.
Western blot analysis
Western blotting was performed with standard protocols using BMDMs or colon tissue. Primary antibodies reactive to rabbit anti-mouse HO-1 (Santa Cruz Biotechnology, Santa Cruz, CA, USA), rabbit anti-mouse Nrf2 polyclonal antibody (Santa Cruz Biotechnology), rabbit monoclonal anti-GAPDH antibody (D16H11, Cell Signaling Technology, Danvers, MA, USA), and β-actin (Sigma-Aldrich Co., St Louis, MO, USA) were used. Blots were washed three times in TBST for 30 minutes, incubated with horseradish peroxidase-conjugated secondary antibodies (1:5,000 dilution; Jackson ImmunoResearch, West Grove, PA, USA) for 20 minutes, washed three times in TBST, and visualized with enhanced chemiluminescence.
Generation of BMDMs
For the generation of mouse macrophages, bone marrow cells were harvested from the femurs and tibias of mice and cultured in six-well tissue culture plates (Costar) for 8 days in complete medium supplemented with 20 ng/mL of M-CSF. PBS or QCN was added to some wells from day 5.
Small interfering RNA transfection
BMDMs were transfected with Hmox1 small interfering RNA (siRNA) (Thermo Fisher Scientific, Waltham, MA, USA) using lipofectamine RNAiMAX Transfection Reagent (Thermo Fisher Scientific). Control scrambled siRNA was purchased from Thermo Fisher Scientific.
Phagolysosomal acidification assay
BMDMs from WT mice were incubated with SnPP (30 μM) for 1 hour. Cells then were treated with LysoTracker Red DND-99 (Invitrogen, Carlsbad, CA, USA) at a concentration of 100 nmol/L for 30 minutes, as well as with E. coli for 1 hour, fixed in 4% paraformaldehyde, and stained with nuclear stain Topro-3 (5 nmol/L; Invitrogen). Cells were visualized using confocal microscopy with a Leica SP5 II (Leica Microsystems) confocal microscope. At least 10 high-powered fields and 100 cells were counted.
Gentamicin protection assay
BMDMs (1 × 106) were cultured in the presence of PBS or QCN and then incubated with E. coli at a 10:1 ratio in antibiotic-free medium for 1 hour. Cells then were washed with PBS plus gentamicin (200 μg/mL) and incubated in medium supplemented with gentamicin (200 μg/mL) at 37°C for an additional hour to eliminate extracellular bacteria. New medium supplemented with gentamicin (100 μg/mL) was added to the cells. In some experiments, SnPP (30 μM) was added to the cells following elimination of the extracellular bacteria. Cells were lysed with 1% Triton X-100, diluted, plated on brain heart infusion agar plates, and incubated at 37°C. Colony-forming units (CFUs) calculated at 1 hour reflected the total uptake of bacteria (phagocytosis). CFUs recovered at 12 hours represent the percentage survival of total bacteria phagocytosed at the 1-hour time point.
Statistical analysis
Values are shown as the SEM, except where otherwise indicated. A comparison of multiple experimental groups was performed by two-way analysis of variance. A t-test was used to compare the means of two groups. P-values <0.05 were considered statistically significant. Sample sizes were calculated to ensure statistical power.
Acknowledgments
This work was supported by grants from National Natural Science Foundation of China (81373149, 31370887), Nature Science Foundation of Jiangsu Province (BK20151195), and Nature Science Foundation of Suzhou City (SYS201363). Fund for Digestive Diseases and Nutrition Research Key Laboratory of Suzhou (SZS201620). Fund of 333 Project of Jiangsu Province (BRA2015106) . Fund of Medical Youth Talent project of Jiangsu Province (QNRC2016241). Nanjing Medical Science and Technique Development Foundation (QRX17205). English-language editing of this manuscript was provided by Dr. Christina Gallucci of Journal Prep.
Additional information
Funding
References
- MacDonald TT, Monteleone I, Fantini MC, et al. Regulation of homeostasis and inflammation in the intestine. Gastroenterology. 2011;140(6):1768–1775. doi:10.1053/j.gastro.2011.02.047.
- Izcue A, Coombes JL, Powrie F. Regulatory lymphocytes and intestinal inflammation. Annu Rev Immunol. 2009;27:313–338. doi:10.1146/annurev.immunol.021908.132657.
- Bain CC, Mowat AM. Macrophages in intestinal homeostasis and inflammation. Immunol Rev. 2014;260(1):102–117. doi:10.1111/imr.12192.
- Iboshi Y, Nakamura K, Ihara E, et al. Multigene analysis unveils distinctive expression profiles of helper T-cell-related genes in the intestinal mucosa that discriminate between ulcerative colitis and Crohn's disease. Inflamm Bowel Dis. 2014;20(6):967–977.
- Heller F, Florian P, Bojarski C, et al. Interleukin-13 is the key effector Th2 cytokine in ulcerative colitis that affects epithelial tight junctions, apoptosis, and cell restitution. Gastroenterology. 2005;129(2):550–564. doi:10.1016/j.gastro.2005.05.002.
- Fuss IJ, Heller F, Boirivant M, et al. Nonclassical CD1d-restricted NK T cells that produce IL-13 characterize an atypical Th2 response in ulcerative colitis. J Clin Invest. 2004;113(10):1490–1497. doi:10.1172/JCI19836.
- Hagemann T, Lawrence T, McNeish I, et al. “Re-educating” tumor-associated macrophages by targeting NF-kappaB. J Exp Med. 2008;205(6):1261–1268. doi:10.1084/jem.20080108.
- Manicassamy S, Reizis B, Ravindran R, et al. Activation of beta-Catenin in Dendritic Cells Regulates Immunity Versus Tolerance in the Intestine. Science. 2010;329(5993):849–853. doi:10.1126/science.1188510.
- Ahern PP, Schiering C, Buonocore S, et al. Interleukin-23 Drives Intestinal Inflammation through Direct Activity on T Cells. Immunity. 2010;33(2):279–288. doi:10.1016/j.immuni.2010.08.010.
- D'Alessio S, Correale C, Tacconi C, et al. VEGF-C-dependent stimulation of lymphatic function ameliorates experimental inflammatory bowel disease. J Clin Invest. 2014;124(9):3863–3878.
- Arranz A, Doxaki C, Vergadi E, et al. Akt1 and Akt2 protein kinases differentially contribute to macrophage polarization. Proc Natl Acad Sci U S A. 2012;109(24):9517–9522. doi:10.1073/pnas.1119038109.
- Bain CC, Scott CL, Mowat AM. Resident and pro-inflammatory macrophages in the colon represent alternative context dependent fates of the same Ly6Chi monocyte precursors. Immunology. 2012;137:218–218.
- Watanabe N, Ikuta K, Okazaki K, et al. Elimination of local macrophages in intestine prevents chronic colitis in interleukin-10-deficient mice. Dig Dis Sci. 2003;48(2):408–414. doi:10.1023/A:1021960401290.
- Hunter MM, Wang A, Parhar KS, et al. In Vitro-Derived Alternatively Activated Macrophages Reduce Colonic Inflammation in Mice. Gastroenterology. 2010;138(4):1395–1405. doi:10.1053/j.gastro.2009.12.041.
- Weisser SB, Brugger HK, Voglmaier NS, et al. SHIP-deficient, alternatively activated macrophages protect mice during DSS-induced colitis. J Leukoc Biol. 2011;90(3):483–492. doi:10.1189/jlb.0311124.
- Denning TL, Wang YC, Patel SR, et al. Lamina propria macrophages and dendritic cells differentially induce regulatory and interleukin 17-producing T cell responses. Nat Immunol. 2007;8(10):1086–1094. doi:10.1038/ni1511.
- Danese S, Sans M, Fiocchi C. Inflammatory bowel disease: the role of environmental factors. Autoimmun Rev. 2004;3(5):394–400. doi:10.1016/j.autrev.2004.03.002.
- Mow WS, Vasiliauskas EA, Lin YC, et al. Association of antibody responses to microbial antigens and complications of small bowel Crohn's disease. Gastroenterology. 2004;126(2):414–424. doi:10.1053/j.gastro.2003.11.015.
- Lodes MJ, Cong Y, Elson CO, et al. Bacterial flagellin is a dominant antigen in Crohn disease. J Clin Invest. 2004;113(9):1296–1306. doi:10.1172/JCI200420295.
- Arthur JC, Perez-Chanona E, Muhlbauer M, et al. Intestinal inflammation targets cancer-inducing activity of the microbiota. Science. 2012;338(6103):120–123. doi:10.1126/science.1224820.
- Duchmann R, Kaiser I, Hermann E, et al. Tolerance exists towards resident intestinal flora but is broken in active inflammatory bowel disease (IBD). Clin Exp Immunol. 1995;102(3):448–455.
- Abraham C, Medzhitov R. Interactions between the host innate immune system and microbes in inflammatory bowel disease. Gastroenterology. 2011;140(6):1729–1737. doi:10.1053/j.gastro.2011.02.012.
- Vezza T, Rodriguez-Nogales A, Algieri F, et al. Flavonoids in Inflammatory Bowel Disease: A Review. Nutrients. 2016;8(4). doi:10.3390/nu8040211. PMID:27070642
- Martin DA, Bolling BW. A review of the efficacy of dietary polyphenols in experimental models of inflammatory bowel diseases. Food Funct. 2015;6(6):1773–1786. doi:10.1039/C5FO00202H.
- Tian H, Zhang X, Wu C, et al. Effects of Baicalin and Octreotide on the serum TNF-alpha level and apoptosis in multiple organs of rats with severe acute pancreatitis. Inflammation. 2009;32(3):191–201.
- Dryden GW, Lam A, Beatty K, et al. A pilot study to evaluate the safety and efficacy of an oral dose of (-)-epigallocatechin-3-gallate-rich polyphenon E in patients with mild to moderate ulcerative colitis. Inflamm Bowel Dis. 2013;19(9):1904–1912.
- Azuma T, Shigeshiro M, Kodama M, et al. Supplemental naringenin prevents intestinal barrier defects and inflammation in colitic mice. J Nutr. 2013;143(6):827–834. doi:10.3945/jn.113.174508.
- Guazelli CFS, Fattori V, Colombo BB, et al. Quercetin-Loaded Microcapsules Ameliorate Experimental Colitis in Mice by Anti-inflammatory and Antioxidant Mechanisms. J Nat Prod. 2013;76(2):200–208. doi:10.1021/np300670w.
- Comalada M, Camuesco D, Sierra S, et al. In vivo quercitrin anti-inflammatory effect involves release of quercetin, which inhibits inflammation through down-regulation of the NF-kappaB pathway. Eur J Immunol. 2005;35(2):584–592. doi:10.1002/eji.200425778.
- Caddeo C, Nacher A, Diez-Sales O, et al. Chitosan-xanthan gum microparticle-based oral tablet for colon-targeted and sustained delivery of quercetin. J Microencapsul. 2014;31(7):694–699. doi:10.3109/02652048.2014.913726.
- Cavalcanti E, Vadrucci E, Delvecchio FR, et al. Administration of reconstituted polyphenol oil bodies efficiently suppresses dendritic cell inflammatory pathways and acute intestinal inflammation. Plos One. 2014;9(2):e88898. doi:10.1371/journal.pone.0088898. PMID:24558444
- Townsend EA, Emala CW, Sr. Quercetin acutely relaxes airway smooth muscle and potentiates beta-agonist-induced relaxation via dual phosphodiesterase inhibition of PLCbeta and PDE4. Am J Physiol Lung Cell Mol Physiol. 2013;305(5):L396–403. doi:10.1152/ajplung.00125.2013. PMID:23873842
- Gardi C, Bauerova K, Stringa B, et al. Quercetin reduced inflammation and increased antioxidant defense in rat adjuvant arthritis. Arch Biochem Biophy. 2015;583:150–157. doi:10.1016/j.abb.2015.08.008.
- Mascaraque C, Aranda C, Ocon B, et al. Rutin has intestinal antiinflammatory effects in the CD4+CD62L+T cell transfer model of colitis. Pharmacol Res. 2014;90:48–57. doi:10.1016/j.phrs.2014.09.005.
- Smythies LE, Sellers M, Clements RH, et al. Human intestinal macrophages display profound inflammatory anergy despite avid phagocytic and bacteriocidal activity. J Clin Invest. 2005;115(1):66–75. doi:10.1172/JCI200519229.
- Deng ZB, Pardi R, Cheadle W, et al. Plant homologue constitutive photomorphogenesis 9 (COP9) signalosome subunit CSN5 regulates innate immune responses in macrophages. Blood. 2011;117(18):4796–4804. doi:10.1182/blood-2010-10-314526.
- Motterlini R, Otterbein LE. The therapeutic potential of carbon monoxide. Nat Rev Drug Discov. 2010;9(9):728–U724. doi:10.1038/nrd3228. PMID:20811383
- Wegiel B, Larsen R, Gallo D, et al. Macrophages sense and kill bacteria through carbon monoxide-dependent inflammasome activation. J Clin Invest. 2014;124(11):4926–4940. doi:10.1172/JCI72853.
- Camuesco D, Comalada M, Rodriguez-Cabezas ME, et al. The intestinal anti-inflammatory effect of quercitrin is associated with an inhibition in iNOS expression. Brit J Pharmacol. 2004;143(7):908–918. doi:10.1038/sj.bjp.0705941.
- Camuesco D, Comalada M, Concha A, et al. Intestinal anti-inflammatory activity of combined quercitrin and dietary olive oil supplemented with fish oil, rich in EPA and DHA (n-3) polyunsaturated fatty acids, in rats with DSS-induced colitis. Clin Nutr. 2006;25(3):466–476. doi:10.1016/j.clnu.2005.12.009.
- Ramyaa P, Krishnaswamy R, Padma VV. Quercetin modulates OTA-induced oxidative stress and redox signalling in HepG2 cells – up regulation of Nrf2 expression and down regulation of NF-kappa B and COX-2. Bba-Gen Subjects. 2014;1840(1):681–692. doi:10.1016/j.bbagen.2013.10.024.
- Bhaskar S, Kumar KS, Krishnan K, et al. Quercetin alleviates hypercholesterolemic diet induced inflammation during progression and regression of atherosclerosis in rabbits. Nutrition. 2013;29(1):219–229. doi:10.1016/j.nut.2012.01.019.
- Comalada M, Camuesco D, Sierra S, et al. In vivo quercitrin anti-inflammatory effect involves release of quercetin, which inhibits inflammation through down-regulation of the NF-kappa B pathway. Eur J Immunol. 2005;35(2):584–592. doi:10.1002/eji.200425778.
- Endale M, Park SC, Kim S, et al. Quercetin disrupts tyrosine-phosphorylated phosphatidylinositol 3-kinase and myeloid differentiation factor-88 association, and inhibits MAPK/AP-1 and IKK/NF-kappa B-induced inflammatory mediators production in RAW 264.7 cells. Immunobiology. 2013;218(12):1452–1467. doi:10.1016/j.imbio.2013.04.019.
- Mascaraque C, Aranda C, Ocon B, et al. Rutin has intestinal antiinflammatory effects in the CD4+ CD62L+ T cell transfer model of colitis. Pharmacol Res. 2014;90:48–57. doi:10.1016/j.phrs.2014.09.005.
- Gordon S, Martinez FO. Alternative Activation of Macrophages: Mechanism and Functions. Immunity. 2010;32(5):593–604. doi:10.1016/j.immuni.2010.05.007.
- Mowat AM. Mucosal Macrophages in Intestinal Homeostasis and Inflammation. J Innate Immun. 2011;3(6):550–564. doi:10.1159/000329099.
- Bain CC, Scott CL, Uronen-Hansson H, et al. Resident and pro-inflammatory macrophages in the colon represent alternative context-dependent fates of the same Ly6Chi monocyte precursors. Mucosal Immunol. 2013;6(3):498–510. doi:10.1038/mi.2012.89.
- Banks C, Bateman A, Payne R, et al. Chemokine expression in IBD. Mucosal chemokine expression is unselectively increased in both ulcerative colitis and Crohn's disease. J Pathol. 2003;199(1):28–35. doi:10.1002/path.1245.
- Biancheri P, Curciarello R, Powell N, et al. Down-Modulation of Intestinal Production of Pro-Inflammatory Cytokines in Experimental Colitis and in Inflammatory Bowel Disease by a Narrow Spectrum Kinase Inhibitor. Gastroenterology. 2013;144(5):S820–S820. doi:10.1016/S0016-5085(13)63044-9.
- Yao P, Nussler A, Liu L, et al. Quercetin protects human hepatocytes from ethanol-derived oxidative stress by inducing heme oxygenase-1 via the MAPK/Nrf2 pathways. J Hepatol. 2007;47(2):253–261. doi:10.1016/j.jhep.2007.02.008.
- Dieleman LA, Pena AS, Meuwissen SG, et al. Role of animal models for the pathogenesis and treatment of inflammatory bowel disease. Scand J Gastroenterol Suppl. 1997;223:99–104.
- Read S, Malmstrom V, Powrie F. Cytotoxic T lymphocyte-associated antigen 4 plays an essential role in the function of CD25(+)CD4(+) regulatory cells that control intestinal inflammation. J Exp Med. 2000;192(2):295–302. doi:10.1084/jem.192.2.295.
- Ju SW, Mu JY, Dokland T, et al. Grape Exosome-like Nanoparticles Induce Intestinal Stem Cells and Protect Mice From DSS-Induced Colitis. Mol Ther. 2013;21(7):1345–1357. doi:10.1038/mt.2013.64.
- Qiu W, Wu B, Wang X, et al. PUMA-mediated intestinal epithelial apoptosis contributes to ulcerative colitis in humans and mice. J Clin Invest. 2011;121(5):1722–1732. doi:10.1172/JCI42917.
- Cooper HS, Murthy SN, Shah RS, et al. Clinicopathologic study of dextran sulfate sodium experimental murine colitis. Lab Invest. 1993;69(2):238–249.
- Xiang Y, Ma N, Wang D, et al. MiR-152 and miR-185 co-contribute to ovarian cancer cells cisplatin sensitivity by targeting DNMT1 directly: a novel epigenetic therapy independent of decitabine. Oncogene. 2014;33(3):378–386. doi:10.1038/onc.2012.575.
- Deng Z, Mu J, Tseng M, et al. Enterobacteria-secreted particles induce production of exosome-like S1P-containing particles by intestinal epithelium to drive Th17-mediated tumorigenesis. Nat Commun. 2015;6:6956. doi:10.1038/ncomms7956. PMID:25907800