ABSTRACT
TEAD4 (TEA domain family member 4) was recently revealed as an oncogenic character in tumorigenesis. However, its role remains unclear in colorectal tumorigenesis. Here, we firstly found that the expression level of TEAD4 was significantly elevated in clinical samples of colorectal adenomas (CRA) and correlated with the size and histological type of CRA. Moreover, patients with higher TEAD4 expression in normal colon mucosa are more prone to be recurrent after polypectomy. TEAD4 knockdown significantly inhibited colorectal cell proliferation in vitro and suppressed tumor growth in vivo. RNA-seq and GSEA analysis reveals TEAD4 can probably regulate Hippo pathway and further experiment confirm the downstream target gene YAP1. The subsequent ChIP-qPCR and luciferase report assay indicated that TEAD4 regulated YAP1 by direct binding and transcriptional activation. In summary, our study reveals that TEAD4 plays an important tumor-promoting role in colorectal cancer by directly targeting the YAP1, thus we suggests TEAD4 may be used as a novel biomarker in colorectal tumorigenesis and provides TEAD4/YAP1 axis as a potential therapeutic option for colorectal cancer.
Introduction
Colorectal cancer (CRC) ranks the third in morbidity rate and fourth in mortality of all human cancers globally Citation[1]. Despite strong hereditary components, in most cases colorectal cancer are sporadic and develop slowly over several years through the adenoma-carcinoma sequence Citation[2].
In developed countries, sporadic adenomatous colorectal polyps are found in roughly one third of asymptomatic adults below the age of 50 who undergo colonoscopy. Depending on their characteristics (multiplicity, size, histologic features, and degree of dysplasia), these lesions can be associated with a substantial risk of recurrence (up to 60% at 3 years) and the development of advanced neoplastic disease Citation[3]. It has been estimated that 15% of all adenomas measuring >1 cm will progress to carcinomas within 10 years of their detection Citation[4].
Two decades of studies in multiple model organisms have established the Hippo pathway as a key regulator of organ size and tissue homeostasis. By inhibiting YAP and TAZ transcription co-activators, the Hippo pathway regulates cell proliferation, apoptosis, and stemness in response to a wide range of extracellular and intracellular signals, including cell-cell contact, cell polarity, mechanical cues, ligands of G-protein-coupled receptors, and cellular energy status. Dysregulation of the Hippo pathway exerts a significant impact on cancer development Citation[5].
TEA domain (TEAD) proteins compose a family of transcription factors that regulate the expression of diverse genes associated with cell proliferation, differentiation and apoptosis Citation[6]. In mammalian cells, the TEAD family transcription factors (TEAD1–4, orthologs of Sd) are key partners for YAP Citation[7]. Recently, the emerging role of TEAD proteins in tumorigenesis has been clearly evidenced, and the oncogenic function of TEADs in various malignancies was lately summarized Citation[8]. TEAD4 is the downstream transcription factor of the evolutionarily conserved Hippo signaling pathway. TEAD4 occupied almost same regions with YAP1 in the mammal genome Citation[9-11]. Lim reported that the multilevel perturbations of TEAD4 at epigenetic, transcriptional and posttranslational levels may contribute to gastric cancer development Citation[12]. Wang reported that TEAD4 and KLF5, in collaboration, promoted triple negative breast cancer cell proliferation and tumor growth in part by inhibiting p27 gene transcription and TEAD4 is a potential target and biomarker for the development of novel therapeutics for breast cancer Citation[13]. Teng reported that TEF3-1 localization to the nucleus stimulates the cell cycle progression in HUVECs and specifically contributes to tumor angiogenesis Citation[14]. Liu reported that TEAD4 nuclear expression can serve as a biomarker for CRC progression and poor prognosis and the transcription factor TEAD4 regulates a pro-metastasis transcription program in a YAP-independent manner in CRC Citation[15]. However, the role of TEAD4 in initiation of CRA or CRC is poorly understood.
Here, we found that TEAD4 expression levels was dramatically elevated in CRA tissues compared with normal colonic tissues from patients. TEAD4 promotes proliferation of colorectal cancer cell in vitro and tumor growth in vivo. TEAD4 may directly targeting YAP1 which was validated by luciferase report assay and ChIP-qPCR.
Results
Expression pattern of TEAD4 in clinical samples of colorectal adenomas
To assess the role of TEAD4 in colorectal adenoma (CRA), we examined expression of TEAD4 in CRA tissues and paired adjacent normal colorectal tissues by real-time PCR. Results showed that TEAD4 expression was significantly higher in CRA tissues than in their normal counterparts (). This phenomenon could also be validated by the published gene chip microarray data GSE4183 and GSE8671 (Fig. S1). To evaluate the relationship between TEAD4 expression level and the clinicopathological features, immunohistochemical analysis of CRA tissue arrays were carried out using a specific anti-TEAD4 antibody. We found that normal colorectal tissues exhibited low TEAD4 expression level calculated by TEAD4 staining score, whereas a substantial part of CRA tissues exhibited a medium or high level of TEAD4 ( and ). Moreover, notably higher expression level of TEAD4 was observed in patients with larger tumor size (P<0.01) or poor pathological type (P<0.05, ). Intriguingly, it was TEAD4 expression levels in normal colorectal tissues but not CRA tissues correlates with adenoma recurrence (). These data suggested TEAD4 performed an oncogenic feature in CRA tissues and might play a role in progression of colorectal cancer.
Figure 1. TEAD4 expression is upregulated in CRA. (a) Analysis of TEAD4 expression (RT-PCR, mRNA level) in CRA and their matched adjacent mucosa in Renji dataset. N = 48, paired sample t test. (b) Immunohistochemical analysis of TEAD4 in CRA tissues and paired normal colorectal mucosa (original magnification × 400). (c) Analysis of TEAD4 expression (Immunohistochemical analysis, protein level) in CRA and their matched adjacent mucosa in Renji dataset. N = 48, t test.
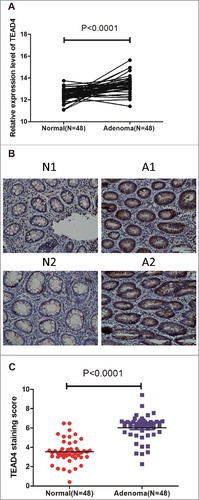
Table 1. The association of TEAD4 expression with clinicopathological characteristics.
Table 2. The association of TEAD4 expression with CRA recurrence.
Knockdown TEAD4 inhibits colorectal cancer cell proliferation in vitro
To investigate the role of TEAD4, we performed a siRNA-mediated knockdown TEAD4 in HCT116 and SW480 CRC cells. The mRNA and protein level of TEAD4 were dramatically reduced after transfection of TEAD4 siRNA in both HCT116 and SW480 cells ( and ). TEAD4 knockdown lead to significantly inhibited cell proliferation compared with control groups as assessed by CCK8 cell viability assay and colony formation assay ( and ). These data indicated that TEAD4 played a critical role in CRC cell proliferation.
Figure 2. Roles of TEAD4 in cell proliferation in vitro. (a,b) qPCR and western blot analysis of TEAD4 expression level in the HCT116 and SW480 cells transduced with control siRNA or TEAD4 siRNA. (b) Cell viability of HCT116 and SW480 cells was quantified at the indicated time by using CCK-8 assay. (c) Colony formation assay of HCT116 and SW480 cells transduced with control shRNA or TEAD4 shRNA were performed, stained and examined at 10 days later.
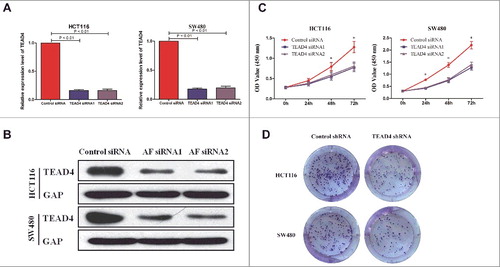
TEAD4 knockdown suppresses tumorigenesis in vivo
To explore the possibility of targeting TEAD4 as a therapeutic measure to suppress colorectal cancer, a xenograft tumor model was established in BALB/c nude mice. HCT116 was transfected pGFP- shRNA plasmid with TEAD4 siRNA sequence (shTEAD4) or with a control siRNA sequence (shControl), and stable cell lines were screened. Then HCT116 stable cell line with control shRNA or TEAD4 shRNA were implanted subcutaneously into left armpits of BALB/c nude mice to allow tumor formation, then tumor growth was monitored and examined. After three weeks, the xenograft tumor formation of HCT116/shTEAD4 group were significantly smaller in size ( and ) and lighter in tumor weight (), compared with those in the HCT116/shControl group (P<0.001), indicating that knockdown of TEAD4 markedly suppressed tumor growth in vivo.
Figure 3. TEAD4 promotes tumor growth in nude mice. (a) Knockdown of TEAD4 inhibits growth of xenografts in nude mice. Stably transfected human CRC HCT116 cells (HCT116/Control shRNA or HCT116/TEAD4 shRNA) were injected subcutaneously into the right forelimb axilla to establish a xenograft model. The sizes of resected tumors arising from cells transfected with/without the TEAD4 knockdown plasmid (at 20 days post-injection) is shown. (b) Tumor growth curves in the TEAD4 knockdown and control groups. (c) Comparison of excised tumor weight in two groups.
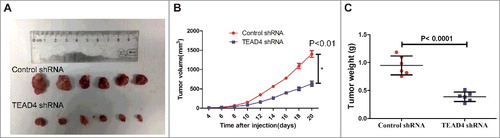
TEAD4 regulated YAP1 downstream Hippo signaling via direct binding and transcriptional activation
To explore the mechanism how TEAD4 regulates colorectal cancer cell proliferation. We performed RNA-seq to analyze the gene expression profile of HCT116 transfected with control or TEAD4 siRNA, seeking a comprehensive understanding of the role of TEAD4 in CRC cells. Compared with HCT116/siControl cells, 640 genes were found downregulated and 513 genes were upregulated in HCT116/siTEAD4 cells based on a >1.5-fold signal change being regarded as significant difference (). Pathway analysis revealed that among the signaling pathways involved in colorectal carcinogenesis, Hippo signaling was significantly downregulated by TEAD4 silencing (). Expression of some important genes downstream Hippo pathway was examined by real-time PCR and immunoblotting analyses in HCT116 and SW480 following TEAD4 silencing. Results confirmed that in both two CRC cell lines, expression of two specific genes, YAP1 and CTGF, were dramatically suppressed upon TEAD4 silencing in mRNA () and protein level (). What's more, in patients with colorectal adenomas, through spearman correlation assay we found a significant positive correlation between TEAD4 expression and YAP1 expression (p< 0.001) ( and F, Fig. S2). As a transcription factor, we firstly wondered whether TEAD4 regulates YAP1 expression by directly binding and transcriptional activation. A luciferase reporter assay was designed with reporter containing the YAP1 promoter region or YAP1 promoter region with mutation on TEAD4-binding motif (, Fig. S3). In HCT116 or SW480 cells transfected with reporter containing the YAP1 promoter region, the YAP1 luciferase activity was significantly lower in TEAD4-knockdown cells compared with control cells, and these differences became diminished when the TEAD4-binding sites on YAP1 promoter was mutated (). We then use chromatin immunoprecipitation-qPCR experiment with anti-TEAD4 antibody to assess whether TEAD4 could directly bind to the endogenous YAP1 promoter region in HCT116 and SW480 cells. Results revealed that compared with anti-IgG control, a significant enrichment of TEAD4 binding in the proximal region (P1) containing TEAD4-binding motif but relatively low enrichment in the distant region (P2, P3, P4, P5) and 3′UTR region (). These data demonstrated that the important oncogene YAP1 downstream Hippo signaling was a direct target of the transcription factor TEAD4 (Fig. S4).
Figure 4. Hippo pathway is regulated by TEAD4 in colon cancer. (a) Heatmap showing the differentially regulated mRNAs in the TEAD4 knockdown HCT116 cells from RNA-seq data analysis. (b) GSEA comparing TEAD4 normal expression group (red) against TEAD4 knockdown expression group (blue) of HCT116 cells. (c) HCT116 and SW480 cells were transfected by TEAD4 siRNA or control siRNA, followed by RT-PCR analysis using specific primers for TEAD4, YAP1 or CTGF. (d) HCT116 and SW480 cells were transfected by TEAD4 siRNA or control siRNA, followed by western blot analysis using specific antibodys for TEAD4, YAP1 or CTGF. (e) Correlation between the mRNA levels of TEAD4 and YAP1 as determined by RT-PCR of CRA tissues. (f) Correlation between the protein levels of TEAD4 and YAP1 as determined by Immunohistochemical staining of CRA tissues.
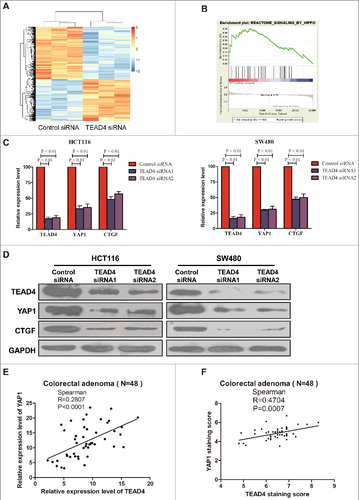
Figure 5. TEAD4 transcriptionally activates YAP1. (a) The predicted TEAD4-binding sequence (−178 to −169) in YAP1 and its mutated variant (both sequences indicated) were respectively inserted into a luciferase reporter and analyzed for their responses to TEAD4. (b) Relative expression of WT and TEAD4-binding motif mutant YAP1 promoter-driven luciferase reporters in HCT116 or SW480 cells transfected with control siRNA or TEAD4 siRNA. (c) ChIP analysis of TEAD4 binding to the YAP1 promoter in the HCT116 or SW480 cells. qPCR was performed with primer specific to regions round the TEAD4-binding motifs (P1) and four control region (P2, P3, P4, P5 and YAP1 3′UTR).
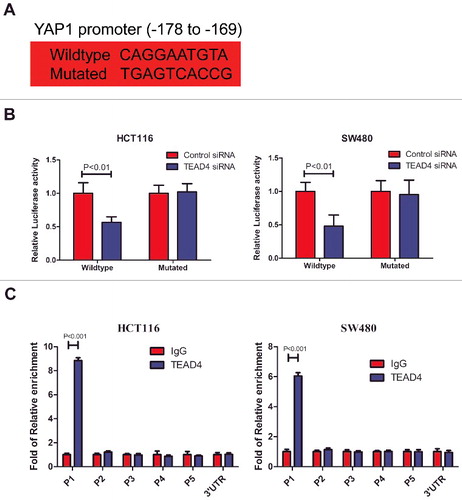
Discussion
Increasing evidence suggests that TEAD4 plays a critical role in carcinogenesis and cancer progression. Here we found that TEAD4 expression was elevated in clinical samples of CRA, which is precancerous lesions of CRC. Interestingly, the expression of TEAD4 is higher in villous adenoma than tubular adenoma. There's a curious question, that is, whether the expression of TEAD4 increases when the malignant degree of colorectal tumors increases gradually? So far, there is no answer to this question, which is subject to sample size and follow-up time.
Our study indicates that TEAD4 expression in normal colonic tissue but not CRA tissue from patients underwent polypectomy are prone to recurrence. The two-hits theory may be the possible explanation for this phenomenon Citation[16]. APC mutation is the most common genetics hit in CRA initiation. High expression of TEAD4 could be viewed as another hit. GSEA analysis also indicated that TEAD4-regulated transcriptome in CRC cells is also rich in genes participating in colon cancer recurrence. These results indicate that TEAD4 is a biomarker for CRA recurrence and might play an important role in the initiation and progression of colorectal adenoma.
In this study, we found that TEAD4 expression was associated with the size and histological type of CRA. Suijkerbuijk reported that Tumor-host interactions play an increasingly recognized role in modulating tumor growth, which APC-/- cells display higher Yorkie (YAP) activity than host cells and promotes tumor growth, in part via cell competition, they also found that relative, rather than absolute, Hippo activity determines adenoma growth Citation[17]. Camargo also reported that YAP1 increases organ size and expands undifferentiated progenitor cells Citation[18]. And our study uncovered the mechanism how TEAD4 promotes colorectal tumorigenesis, we revealed that TEAD4 regulated YAP1 downstream Hippo signaling by direct binding and transcriptional activation, which greatly improved current understanding.
Materials and methods
Patients and specimens
Fresh samples of 48 colorectal adenoma tissues and paired 48 normal colorectal mucosa were obtained from the patients who underwent EMR (endoscopic mucosal resection) or ESD (Endoscopic submucosal dissection) in Renji Hospital. The study protocol was approved by the ethics committee of Renji Hospital, School of Medicine, Shanghai Jiao Tong University. Written informed consents were obtained from all participants in this study. All the research was carried out in accordance with the provisions of the Helsinki Declaration of 1975.
Immunohistochemistry
Immunohistochemistry (IHC) was performed using a standard streptavidin-biotin-peroxidase complex method. Briefly paraffin-embeded section were deparaffinized and rehydrated. Endogenous peroxidase activity was blocked by incubated in 0.3% hydrogen peroxide for 15 min, then the slides were microwaved and boiled in 10 mm citrate buffer (pH 6.0) for antigen retrieval. Nonspecific antigens were blocked by incubation in sheep serum for 30 min. Slides were incubated overnight at 4°C with TEAD4 antibody (1:200, ab97460, Abcam) and YAP1 antibody (1:200, ab52771, Abcam). Sample incubated with PBS instead of primary antibody were used as negative control. TEAD4 and YAP1 staining in the tumor and normal tissues was scored according to the following standards: staining intensity was classified as 0 (lack of staining), 1 (mild staining), 2 (moderate staining) or 3 (strong staining); the percentage of staining was designated as 1 (<25%), 2 (25–50%), 3 (51–75%) or 4 (>75%). For each section, the semiquantitative score was calculated by multiplying these two values (which ranged from 0 to 12). Two histopathologists were blindly assigned to review the slides and score the staining.
RNA sequencing
For RNA-seq, libraries were generated from total RNA using TruSeq RNASample Preparation v2 according to the manufacturer's protocol. Samples were sequenced on Illumina HiScanSQ platform (Illumina, San Diego, CA, USA). Reads were mapped on Hg19 using TopHat v2.0.6 (Johns-HopkinsUniversity, Baltimore, MD, USA), 43 and mRNA quantification was performed using Cuffdiff v2.0.2 (University of Maryland, College Park, MD, USA).
Western Blot
60 μg of protein was electropharesed through 10% SDS polyacrylamide gels and were then transferred to a PVDF membrane (Millipone). The membrane was incubated into TEAD4 antibody (1:500, ab97460, Abcam), YAP1 antibody (1:10000, ab52771, Abcam) and CTGF (1:1000, ab6992, Abcam). GAPDH was used as an endogenous reference.
CCK-8 assay
Cell proliferation was assessed by the CCK-8 cell viability assay (Cell Counting Kit-8, Dojindo, Japan). Briefly, control and treated colon cancer cells were seeded onto the 96-well plates at an initial density of 1 × 103 cells per well. CCK-8 solution (10 ul/well) was added to the cells at specified time points. After incubating for 2 hours, the reaction product was quantified according to manufacturer's instructions.
Colony formation assay
500 cells were seeded in 6-well plates. After 10 days, cells were stained with Crystal Violet Staining Solution (Beyotime C0121), and colonies contained ≥20 cells were counted.
Cell culture, transfection and transduction
Two human colon cancer cell lines, HCT116 and SW480 (purchased from ATCC), were cultureed in McCoy's 5A and 1640 medium (Gibco), supplemented with 10% fetal bovine serum. Cells were then plated in fibronectin- and collagen type I- coated T-25 flasks. All the cells were incubated at 37°C in humidified 5% CO2 atmosphere. TEAD4 siRNA-1 (5′-CCACGAAGGUCUGCUCUUUTT-3′, 5′-AAAGAGCAGACCUUCGUGGTT-3′), TEAD4 siRNA-2 (5′-CGCUCUGUGAGUACAUGAUTT-3′, 5′-AUCAUGUACUCACAGAGCGTT-3′) and control siRNA (5′-UUCUCCGAACGUGUCACGUTT-3′, 5′-ACGUGACACGUUCGGAGAATT-3′) were purchased from Dharmacon RNA Technology (Lafayette, CO, USA). 24 h before transfection at 30–40% confluence, cells were transferred to 6-well plates. Transfection of siRNA was carried out with LipofectamineTM 2000 (Invitrogen) according to the manufacturer's instructions. Cells were collected for analysis 24 h or 48 h after transfection.
Plasmid construction and stable transfection
The pGFP-TEAD4-shRNA plasmid was purchased from Shanghai GeneChem (China) and verified by DNA sequencing. The plasmid encodes a red-shifted variant of wild-type green fluorescent protein (GFP) that has been optimized for brighter fluorescence and greater expression in mammalian cells. For plasmid transfection, HCT116 cells were seeded into 6-well plates 24 h before transfection with plasmids (4 mg/well) using Lipofectamine 2000 Reagent (Invitrogenn), according to the manufacturer's instructions. The pGFP-Control-shRNA construct was used as an empty vector control. Transfected HCT116 were selected using G418 (500 mg/ml and 800 mg/ml, respectively) to generate two stably transfected monoclonal cell lines: one TEAD4 knockdown stable cell lines (HCT116/TEAD4 shRNA) and one control stable cell lines (HCT116/Control shRNA).
In vivo tumorigenesis models
All animals were kept and the experiments were performed in accordance with the European Community guidelines for the use of experimental animals (86/609/EEC). Six pairs of five-week-old male Nu/Nu mice, obtained from the Experimental Animal Center of Shanghai Institute for Biological Sciences (SIBS), were injected subcutaneously (right forelimb axilla) with 5.0 × 106 HCT116/Control shRNA or HCT116/TEAD4 shRNA stable monoclonal cells to establish a CRC xenograft model. After three weeks, all mice were sacrificed, and tumors were isolated for examination of their weight. Our study was approved by the Animal Care and Use Committee of the Renji Hospital, School of Medicine, Shanghai Jiao Tong University, Shanghai, China. All animal procedures were performed according to the guidelines developed by the China Council on Animal Care and the protocol was approved by the Renji Hospital, School of Medicine, Shanghai Jiao Tong University.
RNA extraction and quantitative real-time PCR
Total RNA was extracted by Trizol reagent (Invitrogen), and 1 μg of total RNA from cultured cells was reverse transcribed using the PrimeScriptP RT Reagent Kit (Perfect Real Time, Takara) according to manufacturer's instructions. Quantitative real-time PCR was performed on an Applied Biosystem 7900 quantitative PCR system (Applied Biosystems, Foster City, CA). Quantitative real-time PCR for TEAD4, YAP1 and CTGF was performed according to the manufacturer's instructions and specific primers designed by Shenggong Company (China). The amplified transcript level of each specific gene was normalized to that of GAPDH. The primers used were:
Luciferase report assay
For analysis of luciferase activity, HCT116 and SW480 cell lines were seeded in a 24-well culture plate 24 h before transfection. TEAD4 siRNA were cotransfected with the reporter vectors (YAP1 wildtype-motif or mutant-motif constructs). Renilla control (pRL) was used as an internal control and cotransfected with the described constructs at a ratio of 1:100. Activities of Firefly and Renilla luciferases were determined 48 h after transfection using the Dual-Luciferase Reporter Assay System (Promega) with the BD Monolight 3010 luminometer (BD Biosciences). The Firefly reporter is measured first by adding Luciferase Assay Reagent II to generate a luminescent signal. After quantifying the Firefly luminescence, this reaction is quenched, and the Renilla luciferase reaction is simultaneously initiated by adding Stop & Glo Reagent to the same tube. Relative reporter activity was obtained by normalization to Renilla luciferase activity (ratio of Firefly luciferase to Renilla luciferase). Each experimental condition was measured in triplicate for each luciferase assay, and each luciferase assay was performed three times.
ChIP-qPCR
Chromatin was sonicated and immunoprecipitated with TEAD4 antibody (1:50, ab58310, Abcam). Real-time PCR was performed in triplicates. Each PCR assay was carried out in a 20 ul reaction volume by using the eluted immunoprecipitated DNA. The primers of YAP1 regulatory region were:
Statistical analysis
The data were analyzed by one-way analysis of variance and the Student's t-test to determine statistical significance using SPSS 20.0 statistic software (SPSS, Armonk, NY, USA). Each experiment was repeated at least three times. The results were expressed as MEAN±SEM. Outcomes were considered statistically significant with two-tailed P < 0.05.
Disclosure of potential conflicts of interest
No potential conflicts of interest were disclosed.
Supplementary-1403687.rar
Download (1 MB)Acknowledgments
We thank our colleagues for their critical comments and kind help.
Additional information
Funding
References
- Jemal A, Bray F, Center MM, et al. Global cancer statistics. CA Cancer J Clin. 2011;61:69-90. https://doi.org/10.3322/caac.20107. PMID:21296855
- Brenner H, Kloor M, Pox CP. Colorectal cancer. Lancet. 2014;383:1490-1502. https://doi.org/10.1016/S0140-6736(13)61649-9. PMID:24225001
- Winawer SJ, Zauber AG, Fletcher RH, et al. Guidelines for colonoscopy surveillance after polypectomy: a consensus update by the US Multi-Society Task Force on Colorectal Cancer and the American Cancer Society. Gastroenterology. 2006;130:1872-1885. https://doi.org/10.1053/j.gastro.2006.03.012. PMID:16697750
- Stryker SJ, Wolff BG, Culp CE, et al. Natural history of untreated colonic polyps. Gastroenterology. 1987;93:1009-1013. https://doi.org/10.1016/0016-5085(87)90563-4. PMID:3653628
- Yu FX, Zhao B, Guan KL. Hippo pathway in organ size control, tissue homeostasis, and Cancer. Cell. 2015;163:811-828. https://doi.org/10.1016/j.cell.2015.10.044. PMID:26544935
- Pobbati AV, Hong W. Emerging roles of TEAD transcription factors and its coactivators in cancers. Cancer Biol Ther. 2013;14:390-398. https://doi.org/10.4161/cbt.23788. PMID:23380592
- Zhao B, Ye X, Yu J, et al. TEAD mediates YAP-dependent gene induction and growth control. Genes Dev. 2008;22:1962-1971. https://doi.org/10.1101/gad.1664408. PMID:18579750
- Zhou Y, Huang T, Cheng AS, et al. The TEAD family and Its oncogenic role in promoting tumorigenesis. Int J Mol Sci. 2016;17:138. https://doi.org/10.3390/ijms17010138. PMID:26805820.
- Stein C, Bardet AF, Roma G, et al. YAP1 exerts its transcriptional control via TEAD-Mediated activation of enhancers. PLoS Genet. 2015;11:e1005465. https://doi.org/10.1371/journal.pgen.1005465. PMID:26295846
- Zanconato F, Forcato M, Battilana G, et al. Genome-wide association between YAP/TAZ/TEAD and AP-1 at enhancers drives oncogenic growth. Nat Cell Biol. 2015;17:1218-1227. https://doi.org/10.1038/ncb3216. PMID:26258633
- Dupont S, Morsut L, Aragona M, et al. Role of YAP/TAZ in mechanotransduction. Nature. 2011;474:179-183. https://doi.org/10.1038/nature10137. PMID:21654799
- Lim B, Park JL, Kim HJ, et al. Integrative genomics analysis reveals the multilevel dysregulation and oncogenic characteristics of TEAD4 in gastric cancer. Carcinogenesis. 2014;35:1020-1027. https://doi.org/10.1093/carcin/bgt409. PMID:24325916
- Wang C, Nie Z, Zhou Z, et al. The interplay between TEAD4 and KLF5 promotes breast cancer partially through inhibiting the transcription of p27Kip1. Oncotarget. 2015;6:17685-17697. https://doi.org/10.18632/oncotarget.3779. PMID:25970772
- Liu X, Zhao D, James L, et al. Requirement of the nuclear localization of transcription enhancer factor 3 for proliferation, migration, tube formation, and angiogenesis induced by vascular endothelial growth factor. FASEB J. 2011;25:1188-1197. https://doi.org/10.1096/fj.10-167619. PMID:21169383
- Liu Y, Wang G, Yang Y, et al. Increased TEAD4 expression and nuclear localization in colorectal cancer promote epithelial-mesenchymal transition and metastasis in a YAP-independent manner. Oncogene. 2016;35:2789-2800. https://doi.org/10.1038/onc.2015.342. PMID:26387538
- Knudson AG. Two genetic hits (more or less) to cancer. Nat Rev Cancer. 2001;1:157-162. https://doi.org/10.1038/35101031. PMID:11905807
- Suijkerbuijk SJ, Kolahgar G, Kucinski I, et al. Cell competition drives the growth of intestinal adenomas in Drosophila. Curr Biol. 2016;26:428-438. https://doi.org/10.1016/j.cub.2015.12.043. PMID:26853366
- Camargo FD, Gokhale S, Johnnidis JB, et al. YAP1 increases organ size and expands undifferentiated progenitor cells. Curr Biol. 2007;17:2054-2060. https://doi.org/10.1016/j.cub.2007.10.039. PMID:17980593