ABSTRACT
Evolutionarily conserved E2F family transcription factors regulate the cell cycle via controlling gene expression in a wide range of eukaryotes. We previously demonstrated that the meiosis-specific transcription factor E2fl1 had an important role in meiosis in the model ciliate Tetrahymena thermophila. Here, we report that expression of another E2F family transcription factor gene DPL2 correlates highly with that of E2FL1. Similar to e2fl1Δ cells, dpl2Δ cells undergo meiotic arrest prior to anaphase I, with the five chromosomes adopting an abnormal tandem arrangement. Immunofluorescence staining and immunoprecipitation experiments demonstrate that Dpl2 and E2fl1 form a complex during meiosis. We previously identified several meiotic regulatory proteins in T. thermophila. Cyc2 and Tcdk3 may cooperate to initiate meiosis and Cyc17 is essential for initiating meiotic anaphase. We investigate the relationship of these regulators with Dpl2 and E2fl1, and then construct a meiotic regulatory network by measuring changes in meiotic genes expression in knockout cells. We conclude that the E2fl1/Dpl2 complex plays a central role in meiosis in T. thermophila.
Abbreviations
DAPI | = | 4’, 6-diamidino-2-phenylindole |
DBD | = | DNA-binding domain |
DSBs | = | double-strand breaks |
dpl2Δ | = | DPL2 knockout |
e2fl1Δ | = | E2FL1 knockout |
ELISA | = | enzyme linked immunosorbent assay |
FITC | = | fluorescein isothiocyanate |
FPKM | = | fragments per kilobase of exon per million fragments mapped |
IgG | = | immunoglobulin |
GO | = | gene ontology |
MAC | = | macronucleus |
MIC | = | micronucleus |
MS | = | mass spectrometry |
MuvB | = | multivulva class B |
WT | = | wild-type |
Introduction
Meiosis is an important process in eukaryotic sexual reproduction. To form haploid gametes from diploid cells, a precise reduction in chromosome number is necessary. This occurs through a series of steps: DNA duplication, DNA double-strand breaks (DSBs) formation, homologous chromosome pairing, synaptonemal complex formation, and completion of meiotic recombination repair, leading to chiasmata formation between homologs. Meiotic progression is almost completely conserved among eukaryotes, and has been extensively studied in several model species, including Tetrahymena thermophila [Citation1,Citation2].
T. thermophila is a unicellular ciliate with two functionally distinct nuclei: a diploid (2n) germline micronucleus (MIC) and a polyploid (∼45n) somatic macronucleus (MAC) [Citation3]. As meiosis and the function of many meiotic genes have been extensively studied in T. thermophila [Citation4–14], T. thermophila is an excellent eukaryotic model for studying meiosis regulation. Given the importance of achieving accurate chromosome separation during meiosis, this process must be strictly regulated by a number of factors, including transcription factors. E2F family members have important functions during the cell cycle [Citation15,Citation16]. We previously reported that the E2FL1 transcription factor played an important role during meiosis in T. thermophila. E2FL1 knockout (e2fl1Δ) cells had abnormal meiosis and genes involved in DNA metabolism and homologous chromosome segregation were downregulated [Citation17]. Both E2F and E2F dimerization partner (DP) proteins belong to E2F family in eukaryotes. Therefore, to further understand the transcriptional regulation of meiosis, more transcription factors including DP proteins need to be investgated in T. thermophila.
The first DP transcription factor was identified at 1993 in human cells [Citation18,Citation19]. DP proteins were subsequently found in several eukaryotes: three in Homo sapiens and Mus musculus, two in Arabidopsis thaliana, one in Caenorhabditis elegans and one DP protein in Drosophila melanogaster [Citation16,Citation20,Citation21]. The DNA-binding domain (DBD) of DP is similar to E2F and they can recognize the same DNA consensus sequence [Citation18]. And, DP and E2F could form a heterodimerization which enhances both DNA binding and transcriptional activity [Citation19,Citation22]. The function of DP and E2F proteins that regulate DNA replication and DNA repair are conserved in eukaryotes [Citation21,Citation23–25].
In this paper, we report the identification of the DP transcription factor, DPL2 (TTHERM_00047010), in T. thermophila and its coexpression with E2FL1. Cytological experiments show that disrupting the DPL2 gene leads to arrested meiosis, a phenotype similar to that of E2FL1 knockout cells. Moreover, Dpl2 and E2fl1 form a complex during meiosis. Transcriptome analysis reveal that Dpl2 and E2fl1 cooperate in regulating meiotic progression.
Results
The Dpl2 transcription factor regulates meiosis in T. thermophila
Microarray data from the Tetrahymena Functional Genomics Database (TetraFGD: http://tfgd.ihb.ac.cn/) revealed that in T. thermophila most meiotic genes were strongly transcribed during conjugation, with the highest level of gene expression at early stage of the process [Citation26]. Therefore, meiotic transcription factors are likely to have a similar expression profile. Seven E2F family members (E2FL1, E2FL2, E2FL3, E2FL4, DPL1, DPL2, and DPL3) have been identified in T. thermophila, and all contain a highly conserved winged-helix DBD [Citation17]. Microarray data showed that of these only E2FL1 and DPL2 were highly expressed during conjugation. Consistent with this, transcriptome analysis showed that DPL2 expression was very low under vegetative growth and starvation conditions, but was induced by conjugation, peaking at 3 h post mixing ((A)).
Figure 1. T. thermophila Dpl2 is a transcription factor that regulates meiosis. (A) Microarray and transcriptome sequencing analysis of DPL2 expression. G, vegetative growth; S, starvation; numbers 0–18, number of hours post mixing. (B) Localization of Dpl2-HA (green) throughout the T. thermophila cell cycle. Scale bar: 10 μm. (C) DAPI staining shows the progress of meiosis in WT and dpl2Δ cells. The time post mixing is indicated. Scale bar: 10 μm. (D) GO enrichment analysis of downregulated DEGs in dpl2Δ cells at 3–7 h post mixing. GO terms at each time point post mixing include DNA replication and DNA repair.
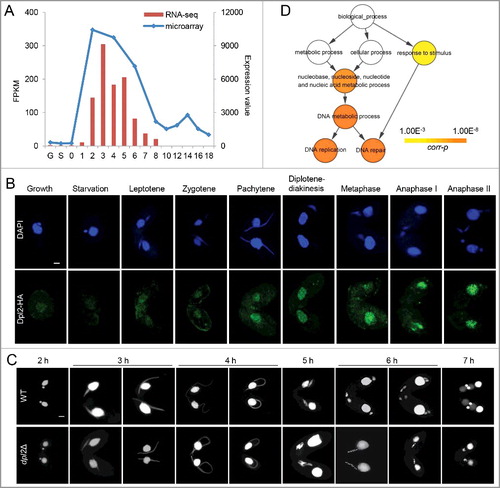
To investigate the function of Dpl2, a C-terminal hemagglutinin (HA)-tagged Dpl2 fusion protein was expressed in T. thermophila. Granular HA signals were detected in MACs under all conditions: these were very weak in vegetatively growing and starved cells but strong in meiotic cells. The strongest HA signals were seen at the chromosome condensation stage, indicating that the endogenous levels of Dpl2 protein should be highest at this time point ((B)).
To study Dpl2 function, DPL2 knockout (dpl2Δ) strains of different mating types were constructed. Transcriptome sequencing analysis showed that DPL2 expression was mostly abolished during meiosis. DPL2 gene knockout cells had no effect on vegetative growth. Comparison of meiosis MIC development in wild-type (WT) and dpl2Δ cells showed that early meiotic MIC elongation and shortening were similar before anaphase. At 6 and 7 h post mixing, WT cells had mostly reached meiotic anaphase and the post-meiotic stage. However, meiotic progression was abnormal at 6 h post mixing in dpl2Δ cells: cells arrested at a stage prior to anaphase I with five dense chromatin masses in an orderly arrangement. The chromatin masses began to condense and by 7 h post mixing some chromatin had condensed into a single MIC ((C) and S1).
To further explore Dpl2 function, differentially expressed genes (DEGs) in WT and dpl2Δ cells were analyzed at 3, 4, 5, 6, and 7 h post mixing using a twofold cutoff. More genes were differentially expresses at later than at early time points (Figure S2), indicating that Dpl2 had an important role in the later stages of meiosis. Gene ontology (GO) enrichment analysis of downregulated DEGs showed that GO terms related to DNA metabolism (which included DNA replication and DNA repair) were significantly enriched at all time points post mixing ((D)).
T. thermophila Dpl2 and E2fl1 form a transcription factor complex
According to TetraFGD, DPL2 and E2FL1 expression levels were highly correlated (r = 0.89). Moreover, our cytological analyses indicated that Dpl2 and E2fl1 proteins colocalized to the MAC and that the phenotype of dpl2Δ cells was similar to that of e2fl1Δ cells. Transcriptome sequencing analysis also showed that GO enrichment profiles for downregulated DEGs were similar in dpl2Δ and e2fl1Δ cells at each time point post mixing. Therefore, we speculated that the Dpl2 may form a complex with E2fl1 during meiosis in T. thermophila.
We used immunofluorescence (IF) and immunoprecipition (IP) analyses to test this hypothesis. Immunoblotting with polyclonal anti-Dpl2 and anti-E2fl1 antibodies clearly documented the presence of Dpl2 and E2fl1 proteins in meiotic protein extracts, corresponding to the sizes of Dpl2-HA and E2fl1-HA proteins bands ((A)). Comparison of IF with HA antibody and polyclonal anti-E2fl1 (or anti-Dpl2) antibody showed similar staining pattern, with both Dpl2 and E2fl1 localizing to the MAC during meiosis. Merged images showed overlapping staining patterns for E2fl1 and Dpl2 ((B)), suggesting that these proteins may interact.
Figure 2. Dpl2 and E2fl1 form a transcription factor complex in T. thermophila. (A) Western blotting analysis of polyclonal anti-Dpl2 (left) or anti-E2fl1 (right) and anti-HA antibody to measure Dpl2 or E2fl1 protein levels during starvation and meiosis. C4, at 4 h post mixing. (B) Immunofluorescence analysis of temporal and spatial expression of endogenous and HA-tagged Dpl2 and E2fl1 protein. Scale bar: 10 μm. (C) Silver-stained Dpl2-HA and E2fl1-HA IP products. Red arrows indicate Dpl2 protein; blue arrows indicate E2fl1 protein. Western blotting analysis using anti-HA monoclonal antibody and anti-E2fl1 and anti-Dpl2 polyclonal antibodies. (D) Venn diagram of IP MS data of E2fl1-HA and Dpl2-HA.
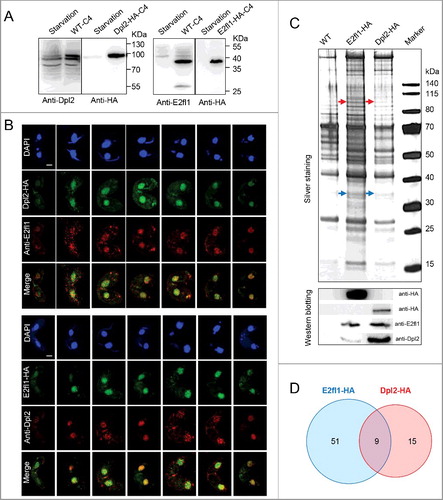
We next analyzed in vivo E2fl1-HA and Dpl2-HA interactomes by IP coupled to silver staining and immunoblotting. Preliminary analysis of the silver staining pattern revealed that several distinct bands were specifically pulled down with Dpl2-HA and E2fl1-HA; immunoblotting identified the Dpl2 and E2fl1 proteins, respectively ((C)). Tandem mass spectrometry (MS) analysis of co-eluted proteins from reciprocal IPs showed that E2fl1 and Dpl2 proteins were included within nine proteins shared by E2fl1-HA and Dpl2-HA in (D). These results provided evidence that Dpl2 and E2fl1 interacted to form a complex during meiosis in Tetrahymena.
The E2fl1/Dpl2 complex is an important meiotic regulator in T. thermophila
We previously identified other important meiotic regulators in T. thermophila, including the Cyc2 and Cyc17 cyclin and the Tcdk3 cyclin-dependent kinase [Citation27,Citation28]. To investigate the function of E2fl1/Dpl2 complex during meiosis regulation, we tracked the expression of meiotic genes in dpl2Δ and e2fl1Δ cells ((A)) and used these data to construct a meiotic regulatory network ((B)). These data revealed a central role for the E2fl1/Dpl2 complex during meiosis.
Figure 3. The E2fl1/Dpl2 complex regulates meiosis in T. thermophila. (A) Heatmap representation of fold changes in the expression of meiotic genes in cyc2Δ, tcdk3Δ, e2fl1Δ, dpl2Δ and cyc17Δ cells compared with WT cells. Upregulated or downregulated genes in mutant cells are colored in red and green, respectively. Values for cyc2Δ, tcdk3Δ and cyc17Δ expression levels were retrieved from NCBI (accession numbers GSE79286, GSE80977, GSE77585, respectively). Euclidean distance was used for hierarchical clustering analysis. The analysis was based on the time to phenotype appearance in knockout cells. (B) Construction of a meiotic regulatory network in T. thermophila. Tcxl1 may form a complex with E2fl1. There is a functional relationship between cell cycle regulators (Cyc2, Tcdk3, E2fl1, Dpl2, and Cyc17) and meiotic proteins.
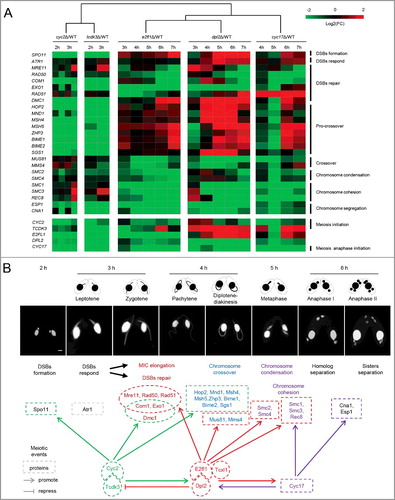
In both dpl2Δ and e2fl1Δ cells, DNA repair synthesis did not take place and meiosis process was arrested before anaphase. Consistent with this, transcriptome analysis showed that genes required for DNA repair (Com1, Exo1, Mre11, Rad50, and Rad51) and chromosome segregation (Cna1, Esp1, Rec8, Smc1, Smc2, Smc3, and Smc4) were downregulated in both knockout cell lines. Genes involved in chromosome crossover (Mms4 and Mus81) were also downregulated ((A), Table S1). These transcriptomics data suggested that Dpl2 and E2fl1 regulated meiosis from late prophase (crossover formation) to anaphase, consistent with the phenotype of dpl2Δ and e2fl1Δ cells.
We also explored the relationship between E2fl1/Dpl2, Cyc2/Tcdk3, and Cyc17. Cyc2 and Tcdk3 may act together to initiate meiosis. They regulated the expression of genes important for early prophase that functioned in DSBs formation and repair [Citation28]. Cyc17 was essential for initiating meiotic anaphase and mainly regulated the genes involved in meiotic anaphase, including those that functioned in chromosome condensation and segregation [Citation27]. (A) showed a heatmap representation of the fold change in expression for these genes. DPL2 downregulation in e2fl1Δ cells suggested that Dpl2 protein levels may be regulated by E2fl1. Therefore, E2fl1 may regulate Dpl2 function at both the transcriptional and post-transcriptional levels. CYC2 and TCDK3 expression was increased in both e2fl1Δ and dpl2Δ cells. Conversely, E2FL1 and DPL2 were downregulated in both cyc2Δ and tcdk3Δ cells. These results indicated the presence of a negative feedback loop between E2fl1/Dpl2 and Cyc2/Tcdk3. CYC17 was downregulated in both e2fl1Δ and dpl2Δ cells, and both E2FL1 and DPL2 were also downregulated in cyc17Δ cell. These results showed the presence of a positive feedback loop between E2fl1/Dpl2 and Cyc17 (). Therefore, Dpl2 complex formation with E2fl1 regulated meiosis in T. thermophila.
Discussion
DNA binding by the E2F/DP heterodimer is conserved in eukaryotes
We previously showed that all members of the eukaryotic E2F transcription factor family contain a highly conserved DBD. In addition, the classic E2F transcription factors (H. sapiens E2Fs 1–6, A. thaliana E2F a-c, C. elegans EFL-1/2) also contain a coiled coil-marked box domains that are necessary to form a heterodimer with the corresponding domains of DP transcription factors [Citation16]. The atypical E2F transcription factors (H. sapiens E2Fs 7 and 8, A. thaliana E2Fs d-f) have duplicate DBDs containing the residues needed for DNA binding and dimerization but cannot form complexes with DPs [Citation29]. Furthermore, in multicellular eukaryotes, DP proteins have a dimerization domain that is necessary for binding to E2F proteins [Citation16]. In T. thermophila, E2FL1/2 are classical E2F proteins and E2FL3/4 are atypical. However, a dimerization domain has not been identified in all T. thermophila DP proteins [Citation17], so it is necessary to determine how T. thermophila E2F and DP proteins interact.
It had been demonstrated that the DP dimerization domain was necessary to form E2F/DP heterodimers in vitro [Citation19]. However, the crystal structural of DNA-bound E2F4-DP2 complex also showed that the DBD contributed to the specificity of DNA binding by the E2F/DP heterodimer. It was therefore possible that residues within the DBD mediated E2F/DP heterodimerization [Citation30]. Sequence alignment of DBDs in T. thermophila and H. sapiens E2F family members identified the conserved DNA-binding motif and the residues essential for E2fl1 and Dpl2 dimerization (Figure S3). Interaction between E2fl1 and Dpl2 was demonstrated by IF and IP in T. thermophila. These data showed that E2fl1/Dpl2 heterodimerization may depend on specific conserved residues within the DBD.
It is therefore possible that E2FL1/2 heterodimerize with DPs to control gene expression and E2FL3/4 recognize and bind consensus E2F-binding sites in target genes in a DP-independent manner in T. thermophila. Based on these results, there was a speculation that the coiled coil-marked box domain may be essential for E2F/DP heterodimerization in both unicellular and multicellular eukaryotes.
Identification of a DREAM-like complex in T. thermophila
The E2F/DP transcription factor complex might regulate the cell cycle by interacting with different proteins. The DREAM complex, consisting of DP, retinoblastoma (RB), E2F proteins, and the multivulva class B (MuvB) complex, regulates cell cycle gene expression [Citation31,Citation32]. E2F, DP, and RB proteins and their evolution have been studied in detail in different eukaryotes [Citation33]. The MuvB complex had been identified in a range of eukaryotes including D. melanogaster, C. elegans, A. thaliana and H. sapiens [Citation16,Citation31,Citation34,Citation35]. The MuvB complex also associates with the Myb transcriptional activator during cell cycle [Citation31]. We identified homologs of some DREAM-like complex components in T. thermophila. Components of some eukaryotic DREAM and DREAM-like are shown in Table S2. Similar DREAM complex has been identified in D. melanogaste, C. elegans, and H. sapiens, suggesting that complex assembly and function may be evolutionarily conserved. There are some differences in complex components in A. thaliana and T. thermophila compared with animals, suggesting that the former may use alternative mechanisms to regulate gene expression.
Tcxl1 (encoded by TCXL1; TTHERM_00600840), a TSO1 CXC-domain protein family member, was one of fifty one E2fl1-HA interacting candidates ((D)). Tcxl1 is a homolog of LIN54, one of MuvB complex components, and gene expression analysis showed that the encoding gene is a meiotic. Tcxl1 binds to DNA via a cysteine-rich CXC domain [Citation36]. The related protein Tomb, is a component of the testis meiotic arrest complex in D. melanogaster. Tomb gene disruption fails to express some meiotic genes [Citation37]. Therefore, we suspected that Tcxl1 may form a complex with E2fl1 in T. thermophila to control meiotic gene expression and thus regulate meiosis. To better understand meiotic regulation of the E2fl1/Dpl2 complex, further efforts will focus on analyzing the function of E2fl1/Dpl2 complex and other components of the DREAM-like complex.
Meiosis regulation by E2fl1/Dpl2 is complex in T. thermophila
In T. thermophila, meiotic DSBs formation are induced by the conserved endonuclease Spo11 [Citation4]. DSBs trigger nuclear elongation via ATM signaling [Citation5]. DSBs end processing requires Mre11, Rad50, Com1, and Exo1 [Citation6,Citation7]. Rad51 and Dmc1 mark DSBs for repair and promote DNA strand exchange [Citation8]. Hop2 and its partner Mnd1 promote recombination repair to form crossovers [Citation4,Citation9]. Due to lack of synaptonemal complexes during meiosis, crossover formation depends on the class II pathway, which is largely independent of ZMM (Zip1/2/3/4, Msh4/5, and Mer3) [Citation38,Citation39]. Therefore, the Mus81 resolvase, its partner Mms4, and the Sgs1 helicase have a prominent role in promoting crossover formation [Citation10]. However, Msh4/5, Zhp3 (a Zip3 homolog) and its partner Bime1, and Bime2 also promote crossover formation [Citation11–13]. Condensation of nuclear DNA into five bivalent chromosomes is controlled by the condensin complex (Smc2 and Smc4). After, the Esp1 separase removes the cohesin complex (Smc1, Smc3, and Rec8) from chromosome arms, homologous chromosomes and sister chromatids are separated [Citation14].
We found that Cyc2/Tcdk3 controls the expression of genes involved in DSBs formation (SPO11), DNA repair (COM1, EXO1, and DMC1) and pro-crossover (HOP2, MND1, MSH4/5, ZHP3, BIME1, and BIME2). Microarray analysis revealed that expression of these genes is mainly restricted to meiosis. However, most meiotic genes regulated by E2fl1/Dpl2 (MRE11, COM1, EXO1, RAD50, RAD51, SMC2, SMC4, SMC1, SMC3, REC8, ESP1, and CNA1) showed some expression under vegetative growth and/or starvation conditions. Therefore, Cyc2/Tcdk3 controls the expression of meiosis-specific genes, whereas E2fl1/Dpl2 controls the expression of meiosis-related genes. These mechanisms may cooperate to ensure that meiosis is tightly regulated in T. thermophila.
The E2fl1/Dpl2 complex regulates meiosis by controlling the expression of DNA repair genes. Although the function of the E2F/DP complex is conserved in eukaryotes, its regulation during meiosis is highly complex and many questions remain. For example, the cause of the meiotic phenotype of Dpl2 and E2fl1 knockout cells is unclear. Therefore, further investigation into meiotic regulation of the E2fl1/Dpl2 complex in T. thermophila is needed.
Materials and methods
Strains and culture conditions
WT T. thermophila strains CU427 (mating type VI) and CU428 (mating type VII) were obtained from the Tetrahymena Stock Center, Cornell University (http://tetrahymena.vet.cornell.edu/) and grown in SPP medium (1% proteose peptone, 0.2% glucose, 0.1% yeast extract, 0.003% Sequestrene) on a rotary shaker at 135 rpm at 30°C [Citation40]. For conjugation, cells of complementary mating types were starved for 20–24 h at 30°C in 10 mM Tris-HCl buffer (pH 7.4) and then mixed at equal densities (to a final density of about 2 × 105 cells/ml). Samples of conjugating cells were harvested at the indicated time points and prepared for RNA sequencing and immunostainings.
Somatic gene knockout and protein HA-tagging
Constructs for somatic gene knockout and protein C-terminal HA-tagging were generated as previously described [Citation41]. Briefly, for somatic gene knockout, the open reading frame of the DPL2 gene was replaced by the coding sequence for the HA epitope tag, and the Neo4 cassette was introduced into the 3ʹ-UTR region to confer paromomycin resistance [Citation42]. For C-terminal tagging, the HA tag was inserted to the open reading frame of the DPL2 gene before the stop codon at its target genomic locus, and the Neo4 cassette was cloned into the 3ʹ-UTR region. The relevant plasmid was then introduced into starved CU427 and CU428 cells via biolistic transformation. Paromomycin-resistant transformants were selected in SPP medium containing paromomycin (Sigma-Aldrich, St Louis, MO, USA) until all MAC copies were replaced via phenotypic assortment [Citation43]. Primers used for amplification were shown in Table S3. Somatic gene knockout was confirmed by transcriptome sequencing, and HA-tagging was confirmed by immunostaining and western blotting.
Production of polyclonal anti-E2fl1 and anti-Dpl2 antibodies
Highly hydrophilic peptides corresponding to the first 140 amino acids (21-160) of Dpl2 and the last 94 amino acids (213-306) of E2fl1 in T. thermophila were codon optimized and synthesized in Escherichia coli (sequences are listed in Table S4). To generate the corresponding recombinant proteins, codon optimized sequences were cloned into the pET-SUMO expression vector and transformed into the E. coli Rosetta strain. Recombinant proteins were purified by Ni-NTA agarose affinity and injected into Japanese white rabbits to generate polyclonal antibodies. The antibody titer was measured by enzyme linked immunosorbent assay (ELISA). The specificity of anti-E2fl1 and anti-Dpl2 polyclonal antibodies (1:2000) was checked in T. thermophila cell extracts by western blotting.
Immunofluorescence staining
For 4’, 6-diamidino-2-phenylindole (DAPI) staining, 5 ml conjugating Tetrahymena cells were fixed at different times post mixing in 250 ul 10% Triton X-100 and 500 ul 37% formaldehyde for 30 min at room temperature (RT, 25°C). Cells were then harvested and resuspended in 500 ul fixative solution (4% paraformaldehyde and 3.4% sucrose in water). Cell suspension (80 ul) was then spread onto a slide and air-dried in a fume hood. Before staining, slides were washed twice with phosphate-buffered saline (PBS) and once with PBS containing 0.05% Triton X-100 (5 min each). For HA immunostaining, the same fixation method was used, but slides were incubated with mouse anti-HA monoclonal antibody (1:100 dilution; clone 16B12, Covance, Berkeley, CA, USA) under a coverslip overnight at 4°C. After washing, cells were incubated with fluorescein isothiocyanate (FITC)-conjugated goat anti-mouse immunoglobulin (IgG) (H+L, 1:1000 dilution, SA00003-1, Proteintech Group, Chicago, IL, USA) for 1 h at RT. After washing, slides mounted with anti-fading agent (Vector Laboratories, Burlingame, CA, USA) containing 0.5 mg/ml DAPI [Citation44].
For E2fl1/Dpl2 co-staining, conjugating cells expressing HA-tagged proteins were prepared as described previously with the following modifications [Citation45]. After overnight incubation with mouse anti-HA monoclonal antibody (1:100 dilution, Covance, Berkeley, CA, USA) at 4°C. cells were washed and incubated in rabbit anti-E2fl1 or anti-Dpl2 polyclonal antibody (1:100 dilution) for about 12 h at 4°C. After washing, cells were incubated with FITC-conjugated goat anti-mouse IgG (H+L, 1:1000 dilution) for 1 h at RT. After washing, cells were then incubated with Alexa Fluor 594-conjugated goat anti-rabbit IgG (H+L, 1:1000 dilution; SA00006-4, Proteintech Group, Chicago, IL, USA) for 1 h at RT. After washing, cells were stained with anti-fading agent containing 0.5 mg/ml DAPI.
Fluorescence staining was observed at 40 × magnification and images were recorded with a Leica TCS SP8 laser scanning confocal microscope (Leica Microsystems, Mannheim, Germany) and Leica LAS AF lite software (http://downloads.informer.com/leica-las-af-lite/).
Data analysis for IP-coupled mass spectrometry (MS)
For IP, to pull down HA-tagged proteins from Tetrahymena cell extracts, cells were harvested after conjugation 3.5–4 h when most paired cells were at pachytene stage. Cells were resuspended in ice-cold Tris buffer (50 mM Tris, 100 mM NaCl, 100 mM EDTA) and resedimented. Cells pellets were resuspended in ice-cold lysis buffer (30 mM Tris-HCl, 20 mM KCl, 2 mM MgCl2, 1 mM phenylmethylsulfonyl fluoride, 0.1% Triton X-100) containing cOmplete proteinase inhibitor (Roche Diagnostics, Indianapolis, IN, USA) and ground in a Dounce homogenizer on ice. NaCl (final concentration of 150 mM) was added to the lysate and cell debris was removed by centrifugation. After filtering the supernatant, soluble proteins were incubated with EZview anti-HA agarose beads (Sigma-Aldrich, St Louis, MO, USA) for 2 h at 4°C. After stringent washing, HA-tagged proteins were eluted with HA peptides (Sigma-Aldrich, St Louis, MO, USA) according to the manufacturer's instructions. IP products were analyzed by silver staining and immunoblotted with an anti-HA antibody.
Co-immunoprecipitating protein were tryptic digested with trypsin and analyzed with a Triple TOF® 5600 tandem mass spectrometer (AB Sciex, Framingham, MA, USA). The MS dataset was analyzed with ProteinPilot software (version 5.0.1, https://sciex.com/products/software/proteinpilot-software) against Tetrahymena protein sequences (downloaded from the TGD; http://ciliate.org/index.php/home/downloads). MS raw data are listed in Table S5. Confidence scores for protein interactions were set at a Bayesian false discovery rate (FDR) value of <0.1.
Gene and domain identification
To identify homolog for DREAM complex components in Tetrehymena, BLASTP was used to compare protein similarity in model eukaryotes [Citation46]. Protein domain prediction was based on the NCBI conserved domain database and protein structure diagrams were generated using ENDscript 2.0 [Citation47,Citation48].
Transcriptome sequencing and data analysis
Total RNA was extracted from WT and dpl2Δ mating cells at 3, 4, 5, 6, and 7 h post mixing using the RNeasy Protect Cell Mini Kit (Qiagen, Valencia, CA, USA), as previously described (TetraFGD: http://tfgd.ihb.ac.cn/index/smphelp) [Citation49]. Pair-end Illumina sequencing libraries were constructed using the manufacturer's protocols and analyzed with a HiSeq 4000 sequencer (Illumina, San Diego, CA, USA). Raw read adaptors were trimmed with Trim-Galore (version 0.4.0) and mapped to the T. thermophila MAC genome (version 2014: http:// ciliate.org/index.php/home/downloads) using TopHat (version 2.0.9) [Citation50,Citation51]. Differential gene expression analyses was assessed using Cuffdiff (version 2.1.1) [Citation52]. The following criteria were used to identify DEGs between WT and dpl2Δ: after normalizing expression levels to fragments per kilobase of exon per million fragments mapped (FPKM), the lower FPKM value (< 20) were excluded for controlling false positives; and a twofold cutoff was used. GO enrichment analysis was carried out using BiNGO (version 3.0.2) [Citation53,Citation54]. FDR correction was used in multiple testing to control for false positives, and significantly enriched GO terms were defined by a corrected p-value of <0.001.
All RNA sequence data have been submitted to GenBank databases under accession number GSE104524.
Disclosure of potential conflicts of interest
No potential conflicts of interest were disclosed.
sup_mat_1431595_KCCY.zip
Download Zip (509.9 KB)Additional information
Funding
References
- Kohl KP, Sekelsky J. Meiotic and mitotic recombination in meiosis. Genetics. 2013;194(2):327–334. doi:10.1534/genetics.113.150581. PMID:23733849
- Chi JY, Mahe F, Loidl J, et al. Meiosis gene inventory of four ciliates reveals the prevalence of a synaptonemal complex-independent crossover pathway. Mol Biol Evol. 2014;31(3):660–672. doi:10.1093/molbev/mst258. PMID:24336924
- Orias E, Cervantes MD, Hamilton EP. Tetrahymena thermophila, a unicellular eukaryote with separate germline and somatic genomes. Res Microbiol. 2011;162(6):578–586. doi:10.1016/j.resmic.2011.05.001. PMID:21624459
- Mochizuki K, Novatchkova M, Loidl J. DNA double-strand breaks, but not crossovers, are required for the reorganization of meiotic nuclei in Tetrahymena. J Cell Sci. 2008;121(13):2148–2158. doi:10.1242/jcs.031799. PMID:18522989
- Loidl J, Lukaszewicz A, Howard-Till RA, et al. The Tetrahymena meiotic chromosome bouquet is organized by centromeres and promotes interhomolog recombination. J Cell Sci. 2012;125(23):5873–5880. doi:10.1242/jcs.112664. PMID:22976299
- Lukaszewicz A, Howard-Till RA, Novatchkova M, et al. Mre11 and Com1/Sae2 are required for double-strand break repair and efficient chromosome pairing during meiosis of the protist Tetrahymena. Chromosoma. 2010;119(5):505–518. doi:10.1007/s00412-010-0274-9. PMID:20422424
- Lukaszewicz A, Shodhan A, Loidl J. Exo1 and Mre11 execute meiotic DSB end resection in the protist Tetrahymena. DNA Repair. 2015;35:137–143. doi:10.1016/j.dnarep.2015.08.005. PMID:26519827
- Howard-Till RA, Lukaszewicz A, Loidl J. The recombinases Rad51 and Dmc1 play distinct roles in DNA break repair and recombination partner choice in the meiosis of Tetrahymena. Plos Genet. 2011;7(3):e1001359. doi:10.1371/journal.pgen.1001359. PMID:21483758
- Loidl J, Lorenz A. DNA double-strand break formation and repair in Tetrahymena meiosis. Semin Cell Dev Biol. 2016;54:126–134. doi:10.1016/j.semcdb.2016.02.021. PMID:26899715
- Lukaszewicz A, Howard-Till RA, Loidl J. Mus81 nuclease and Sgs1 helicase are essential for meiotic recombination in a protist lacking a synaptonemal complex. Nucleic Acids Res. 2013;41(20):9296–9309. doi:10.1093/nar/gkt703. PMID:23935123
- Shodhan A, Lukaszewicz A, Novatchkova M, et al. Msh4 and Msh5 function in SC-independent chiasma formation during the streamlined meiosis of Tetrahymena. Genetics. 2014;198(3):983–993. doi:10.1534/genetics.114.169698. PMID:25217051
- Shodhan A, Kataoka K, Mochizuki K, et al. A Zip3-like protein plays a role in crossover formation in the SC-less meiosis of the protist Tetrahymena. Mol Biol Cell. 2017;28(6):825–833. doi:10.1091/mbc.E16-09-0678. PMID:28100637
- Shodhan A, Novatchkova M, Loidl J. BIME2, a novel gene required for interhomolog meiotic recombination in the protist model organism Tetrahymena. Chromosome Res. 2017;25(3–4):291–298. doi:10.1007/s10577-017-9563-y. PMID:28803330
- Howard-Till RA, Lukaszewicz A, Novatchkova M, et al. A single cohesin complex performs mitotic and meiotic functions in the protist Tetrahymena. Plos Genet. 2013;9(3):e1003418. doi:10.1371/journal.pgen.1003418. PMID:23555314
- Weinberg RA. The retinoblastoma protein and cell cycle control. Cell. 1995;81(3):323–330. doi:http://www.ncbi.nlm.nih.gov/pubmed/7736585. PMID:7736585
- van den Heuvel S, Dyson NJ. Conserved functions of the pRB and E2F families. Nat Rev Mol Cell Biol. 2008;9(9):713–724. doi:10.1038/nrm2469. PMID:18719710
- Zhang J, Tian M, Yan GX, et al. E2fl1 is a meiosis-specific transcription factor in the protist Tetrahymena thermophila. Cell Cycle. 2017;16(1):123–135. doi:10.1080/15384101.2016.1259779. PMID:27892792
- Girling R, Partridge JF, Bandara LR, et al. A new component of the transcription factor DRTF1/E2F. Nature. 1993;365(6445):468. doi:10.1038/365468d0. PMID:8413592
- Helin K, Wu CL, Fattaey AR, et al. Heterodimerization of the transcription factors E2F-1 and DP-1 leads to cooperative trans-activation. Genes Dev. 1993;7(10):1850–1861. doi:http://www.ncbi.nlm.nih.gov/pubmed/8405995. PMID:8405995
- Dynlacht BD, Brook A, Dembski M, et al. DNA-binding and trans-activation properties of Drosophila E2F and DP proteins. Proc Natl Acad Sci USA. 1994;91(14):6359–6363. doi:http://www.ncbi.nlm.nih.gov/pubmed/8022787. PMID:8022787
- Mariconti L, Pellegrini B, Cantoni R, et al. The E2F family of transcription factors from Arabidopsis thaliana. Novel and conserved components of the retinoblastoma/E2F pathway in plants. J Biol Chem. 2002;277(12):9911–9919. doi:10.1074/jbc.M110616200. PMID:11786543
- Krek W, Livingston DM, Shirodkar S. Binding to DNA and the retinoblastoma gene product promoted by complex formation of different E2F family members. Science. 1993;262(5139):1557–1560. doi:http://www.ncbi.nlm.nih.gov/pubmed/8248803. PMID:8248803
- Dyson N. The regulation of E2F by pRB-family proteins. Gene Dev. 1998;12(15):2245–2262. doi:10.1101/gad.12.15.2245. PMID:9694791
- Bracken AP, Ciro M, Cocito A, et al. E2F target genes: unraveling the biology. Trends Biochem Sci. 2004;29(8):409–417. doi:10.1016/j.tibs.2004.06.006. PMID:15362224
- Hao XF, Alphey L, Bandara LR, et al. Functional conservation of the cell cycle-regulating transcription factor DRTF1/E2F and its pathway of control in Drosophila melanogaster. J Cell Sci. 1995;108(Pt 9):2945–2954. doi:http://www.ncbi.nlm.nih.gov/pubmed/8537434. PMID:8537434
- Miao W, Xiong J, Bowen J, et al. Microarray analyses of gene expression during the Tetrahymena thermophila life cycle. Plos One. 2009;4(2):e4429. doi:10.1371/Journal.Pone.0004429. PMID:19204800
- Yan GX, Dang H, Tian M, et al. Cyc17, a meiosis-specific cyclin, is essential for anaphase initiation and chromosome segregation in Tetrahymena thermophila. Cell Cycle. 2016;15(14):1855–1864. doi:10.1080/15384101.2016.1188238. PMID:27192402
- Yan GX, Zhang J, Shodhan A, et al. Cdk3, a conjugation-specific cyclin-dependent kinase, is essential for the initiation of meiosis in Tetrahymena thermophila. Cell Cycle. 2016;15(18):2506–2514. doi:10.1080/15384101.2016.1207838. PMID:27420775
- Lammens T, Li J, Leone G, et al. Atypical E2Fs: new players in the E2F transcription factor family. Trends Cell Biol. 2009;19(3):111–118. doi:10.1016/j.tcb.2009.01.002. PMID:19201609
- Zheng N, Fraenkel E, Pabo CO, et al. Structural basis of DNA recognition by the heterodimeric cell cycle transcription factor E2F-DP. Genes Dev. 1999;13(6):666–674. doi:http://www.ncbi.nlm.nih.gov/pubmed/10090723. PMID:10090723
- Fischer M, Muller GA. Cell cycle transcription control: DREAM/MuvB and RB-E2F complexes. Crit Rev Biochem Mol Biol. 2017;52(6):638–662. doi:10.1080/10409238.2017.1360836.
- Lee H, Ragusano L, Martinez A, et al. A dual role for the dREAM/MMB complex in the regulation of differentiation-specific E2F/RB target genes. Mol Cell Biol. 2012;32(11):2110–2120. doi:10.1128/MCB.06314-11. PMID:22451490
- Cao L, Peng B, Yao L, et al. The ancient function of RB-E2F pathway: insights from its evolutionary history. Biol Direct. 2010;5(1):55. doi:10.1186/1745-6150-5-55. PMID:20849664
- Flowers S, Beck GR Jr., Moran E. Tissue-specific gene targeting by the multiprotein mammalian DREAM complex. J Biol Chem. 2011;286(32):27867–27871. doi:10.1074/jbc.C111.255091. PMID:21685383
- Magyar Z, Bogre L, Ito M. DREAMs make plant cells to cycle or to become quiescent. Curr Opin Plant Biol. 2016;34:100–106. doi:10.1016/j.pbi.2016.10.002. PMID:27816815
- Marceau AH, Felthousen JG, Goetsch PD, et al. Structural basis for LIN54 recognition of CHR elements in cell cycle-regulated promoters. Nat Commun. 2016;7:12301. doi:10.1038/ncomms12301. PMID:27465258
- Jiang J, Benson E, Bausek N, et al. Tombola, a tesmin/TSO1-family protein, regulates transcriptional activation in the Drosophila male germline and physically interacts with always early. Development. 2007;134(8):1549–1559. doi:10.1242/dev.000521. PMID:17360778
- Bishop DK, Zickler D. Early decision: meiotic crossover interference prior to stable strand exchange and synapsis. Cell. 2004;117(1):9–15. doi:10.1016/S0092-8674(04)00297-1. PMID:15066278
- Borner GV, Kleckner N, Hunter N. Crossover/noncrossover differentiation, synaptonemal complex formation, and regulatory surveillance at the leptotene/zygotene transition of meiosis. Cell. 2004;117(1):29–45. doi:10.1016/S0092-8674(04)00292-2. PMID:15066280
- Orias E, Hamilton EP, Orias JD. Tetrahymena as a laboratory organism: useful strains, cell culture, and cell line maintenance. Method Cell Biol. 2000;62(62):189–211. doi:https://doi.org/10.1016/S0091-679X(08)61530-7.
- Tian M, Yang W, Zhang J, et al. Nonsense-mediated mRNA decay in Tetrahymena is EJC independent and requires a protozoa-specific nuclease. Nucleic Acids Res. 2017;45(11):6848–6863. doi:10.1093/nar/gkx256. PMID:28402567
- Mochizuki K. High efficiency transformation of Tetrahymena using a codon-optimized neomycin resistance gene. Gene. 2008;425(1-2):79–83. doi:10.1016/j.gene.2008.08.007. PMID:18775482
- Cassidyhanley D, Bowen J, Lee JH, et al. Germline and somatic transformation of mating Tetrahymena thermophila by particle bombardment. Genetics. 1997;146(1):135–147. doi:http://www.genetics.org/content/genetics/146/1/135. PMID:9136007
- Loidl J, Scherthan H. Organization and pairing of meiotic chromosomes in the ciliate Tetrahymena thermophila. J Cell Sci. 2004;117(24):5791–5801. doi:10.1242/jcs.01504. PMID:15522890
- Liu Y, Taverna SD, Muratore TL, et al. RNAi-dependent H3K27 methylation is required for heterochromatin formation and DNA elimination in Tetrahymena. Genes Dev. 2007;21(12):1530–1545. doi:10.1101/gad.1544207. PMID:17575054
- Altschul SF, Madden TL, Schaffer AA, et al. Gapped BLAST and PSI-BLAST: a new generation of protein database search programs. Nucleic Acids Res. 1997;25(17):3389–3402. doi:10.1093/nar/25.17.3389. PMID:9254694
- Marchler-Bauer A, Derbyshire MK, Gonzales NR, et al. CDD: NCBI's conserved domain database. Nucleic Acids Res. 2015;43(D1):D222–D226. doi:10.1093/nar/gku1221. PMID:25414356
- Robert X, Gouet P. Deciphering key features in protein structures with the new ENDscript server. Nucleic Acids Res. 2014;42(Web Server issue):W320–W324. doi:10.1093/nar/gku316. PMID:24753421
- Xiong J, Lu XY, Zhou ZM, et al. Transcriptome analysis of the model protozoan, Tetrahymena thermophila, using deep RNA sequencing. Plos One. 2012;7(2):e30630. doi:10.1371/journal.pone.0030630. PMID:22347391
- Wu ZP, Wang X, Zhang XG. Using non-uniform read distribution models to improve isoform expression inference in RNA-Seq. Bioinformatics. 2011;27(4):502–508. doi:10.1093/bioinformatics/btq696. PMID:21169371
- Kim D, Salzberg SL. TopHat-Fusion: an algorithm for discovery of novel fusion transcripts. Genome Biol. 2011;12(8):R72. doi:10.1186/Gb-2011-12-8-R72. PMID:21835007
- Trapnell C, Roberts A, Goff L, et al. Differential gene and transcript expression analysis of RNA-seq experiments with TopHat and Cufflinks. Nat Protoc. 2012;7(3):562–578. doi:10.1038/nprot.2012.016. PMID:22383036
- Ashburner M, Ball CA, Blake JA, et al. Gene ontology: tool for the unification of biology. The Gene Ontology Consortium Nat Genet. 2000;25(1):25–29. doi:10.1038/75556.
- Maere S, Heymans K, Kuiper M. BiNGO: a Cytoscape plugin to assess overrepresentation of gene ontology categories in biological networks. Bioinformatics. 2005;21(16):3448–3449. doi:10.1093/bioinformatics/bti551. PMID:15972284