ABSTRACT
The human immunodeficiency virus-1 (HIV-1) regulatory protein Tat plays an important role during HIV-1-associated neurocognitive disorders (HAND) by inducing neuronal autophagy. In this study, we used immunohistochemistry, immunofluorescence, western blot, qRT-PCR, and RNA interference to elucidate the involvement of Bcl-2-associated athanogene 3 (BAG3) in the pathogenesis of HIV-1 Tat-induced autophagy during HAND. We found that BAG3 expression is elevated in astrocytes in frontal cortex of macaques infected with simian immunodeficiency virus-human immunodeficiency chimeric virus (SHIV). In addition, in human primary glioblastoma cells (U87), HIV-1 Tat upregulated BAG3 in an NF-κB-dependent manner to induce autophagy. Importantly, suppression of BAG3 or inhibition of NF-κB activity reversed the HIV-1 Tat-induced autophagy. These results indicate that HIV-1 Tat induces autophagy by upregulating BAG3 via NF-κB signaling, which suggests BAG3 and NF-κB could potentially serve as novel targets for HAND therapies.
Introduction
As many as 50% of people living with treated human immunodeficiency virus (HIV) infection may be afflicted by HIV brain infection and HIV-associated neurocognitive disorders (HAND), resulting in profound cognitive, behavioral, and motor impairment [Citation1]. The pathological features of HAND have been clarified including microglial nodules, multinucleated giant cells, neuronal loss, and widespread reactive astrocytosis [Citation1], however, the molecular mechanisms involved in HAND remain incompletely understood.
Postmortem brains of patients with HIV-1 encephalitis exhibit an increased expression of autophagy markers, suggesting that dysregulation of autophagy might be important in the pathogenesis of HIV-1 encephalitis [Citation2]. Additionally, in cells of monocyte/macrophage lineage, HIV-1 proteins can both promote and delay autophagy to facilitate different stages of viral replication, which might modulate the pathogenesis of HAND [Citation3]. On the other hand, in human astrocytes, HIV-1 protein Nef blocks the last step of autophagy, which helps HIV-1 virus to avoid autophagic degradation [Citation4]. Unlike Nef, HIV-1 Tat increases autophagy, mitochondrial damage, and oxidative stress in neuronal cell lines and mouse astrocytes [Citation5,Citation6]. However, the specific mechanisms of how HIV-1 Tat induces autophagy in astrocytes are not clear.
According to previous studies, BAG3 participates in autophagosome formation during the initiation process [Citation7,Citation8]. In addition, studies have shown an increased expression of BAG3 in human lymphocytes and fetal microglial cells infected with HIV-1 [Citation9,Citation10], but the responsible mechanisms are not clear.
In this study, we found BAG3 increased in astrocytes in the SHIV-infected macaques HAND animal model. We hypothesized that BAG3 is involved in the HIV-1 Tat-induced autophagy and HAND pathogenesis, and we analyzed the responsible molecular mechanisms in astrocytes using cell experiments.
Materials and methods
SHIV-infected animals
SHIV−162.P4 is a molecularly cloned and a T-lymphocyte-tropic virus, which includes the main genes from HIV-1(SF162) (R5, MT/NSI) in the molecular clone SIV−mac239, such as the tat, env, rev, and vpu genes. The pathogenic properties of SHIV−162.P4 virus have been previously described [Citation11]. This virus could induce immunosuppression and eventually develop to AIDS in macaques. Twelve macaques were screened and found to be seronegative for simian T-lymphotropic virus, SHIV, SIV, B virus, and Type D retroviruses. Eight SHIV-infected macaques (#1-8) were intravenously inoculated with SHIV−162.P4 (provided by Dr. Nancy Miller, at the National Institute of Allergy and Infectious Diseases, National Institutes of Health, Bethesda, MD, USA) at 40 weeks of age, and sacrificed at 68 weeks of age (). Four uninfected macaques (#10-13) served as controls (). The animals were housed in individual cages and maintained according to the rules and guidelines of the Institute of Laboratory Animal Sciences of Chinese Academy of Medical Science and the National Institute for Infectious Diseases and Experimental Animal Welfare. The animals were sacrificed at the same time after infection, when they showed signs of morbidity, such as loss of appetite, unresponsiveness, or sluggishness. Viral RNA loads were analyzed in peripheral blood of infected macaques at the time of autopsy (). The methods used have been previously described [Citation12,Citation13].
Table 1. The information of the macaques in this study
Histopathological examination
The brain tissues were embedded in paraffin and cut into 5-micron thick sections. Immunohistochemistry (IHC) was performed using a streptavidin peroxidase (SP) kit (Maixin Biotechnology, KIT9720) with antibody specific to BAG3 (BBI Life Sciences, D220147) according to instructions.
Double-labeling immunohistochemistry
To analyze the co-localization of BAG3-expressing cells with glial fibrillary acidic protein (GFAP) in the frontal cortex of SHIV-infected macaques, we performed double-labeling IHC using the Envision method. BAG3 antibody (BBI Life Sciences, D220147), AEC/peroxidase (PO) (Maixin Biotechnology, AEC-0037), GFAP antibody (Epitomics, 2301-1) and PermaBlue Plus/alkaline phosphatase (AP) (Diagnostic Biosystems, K058).
Cell culture and transfection
U87 cells were purchased from American Type Culture Collection (Manassas) and cultured in basic Dulbecco’s modified Eagle’s medium (DMEM) (Gibco, C11995500BT) supplemented with 10% fetal bovine serum (FBS) (Gibco, 12657) and 1% Penicillin-Streptomycin Solution (HyClone, SV30010) in a humidified atmosphere of 5% CO2 at 37°C.
Transfection was performed by using Lipofec-tamine® 2000 Transfection Reagent (Invitrogen, 11668019) following the manufacturers protocol. Overexpression plasmid pcDNA3.1-Tat-HA was prepared in the E. coli DH5α strain and extracted using Endofree Maxi Plasmid Kit (TIANGEN, DP117). GV112-BAG3-shRNA and pEGFP-C3-BAG3-GFP plasmids were purchased from Shanghai Genechem Company. U87 cells were transfected with plasmids at 70% confluence.
Western blotting analysis
Cells were harvested by centrifugation, rinsed with PBS and extracted using RIPA lysis buffer (Boster, AR0105-100). The lysate was centrifuged for 20 min at 15,000 × rpm, at 4°C. The proteins from the frontal cortex of SHIV-infected macaques were extracted using Qproteome FFPE Tissue Kit (QIAGEN, 37623). The supernatant was used for protein determination using the BCA Protein Assay Kit (Thermo Scientific Pierce, 23228). The proteins were separated on 8% to 12% SDS-PAGE gels. Immunoblotting was performed using one of the primary antibodies: HIV-1 Tat (Abcam, ab63957), BAG3 (BBI Life Sciences, D220147), Phospho-p65 (Ser311) (BBI Life Sciences, D151215), IκBα (Cell Signaling Technology, #4812), Phospho-IκBα (Ser32) (Cell Signaling Technology, #5209), LC3B (Cell Signaling Technology, #12513), p62 (Cell Signaling Technology, #5114), or GAPDH (Abcam, ab181602), followed with horseradish peroxidase-labeled secondary antibodies (R&D Systems, HAF008 and Thermo Fisher, 62-6520). Chemiluminescent HRP substrate (Millipore, WBKLS0500) was used to visualize the protein bands.
RNA extraction and quantitative real-time PCR (qRT-PCR)
Cell RNA extraction was done using the IBI-Trizol Reagent (Life Technologies, 15596018), and tissue RNA was extracted from the frontal cortex of SHIV-infected macaques using FFPE Kit (OMEGA, R6954-01). Reverse transcription was performed using the ReverTra Ace qRT-PCR Master Mix (Toyobo, FSQ-201). qRT-PCR was carried out using the FastStart Universal SYBR Green Master (ROX) (Toyobo, 11750800). The RNA level was expressed as 2-ΔCt, where ΔCt = Ct (gene)–Ct (β-actin). Additionally, the RNA level of the control was considered as “1”. The forward and reverse primers used for each gene are shown in . Each sample represents a pool of three replicates per experiment. Experiments were done at least three times.
Table 2. Primers for the qRT-PCR Assay
Immunofluorescence
U87 cells were seeded in 24-well plates with glass coverslips and transfection was performed as described above. Cells were fixed 48 hours after transfection with 4% freshly-prepared paraformaldehyde (pH 6.9), permeabilized with 0.5% Triton-X100 in 1× PBS, and blocked with 5% BSA. The cells were then incubated with primary antibodies specific to p65 (Proteintech, 10745-1-AP) and HIV-1 Tat (Abcam, ab63957), in blocking buffer overnight at 4°C, washed three times with 1× PBS, and incubated with fluorescent secondary antibodies (Thermo, #31506) for 1 hour. The sections were stained with DAPI (VECTOR, H-1200) for nuclear staining. Images were collected under a laser scanning confocal microscope (Nikon).
Statistical analysis
All results from at least three independent experiments were expressed as mean ± standard deviation (SD). Data was analyzed by unpaired-samples t-test and one-way ANOVA using GraphPad Prism5.0 statistical software. P < 0.05 was considered statistically significant.
We also performed semi-quantitative BAG3 assessment for the IHC data. BAG3 antibody-positive cells were counted in 5 light microscopic fields (original magnification: 100×) of the frontal cortex. When more than 25 BAG3-positive cells were counted, this was considered evidence of an increase of BAG3. 0 stands for the less than 25 BAG3-positive cells; 1 stands for between 25 and 50 BAG3-positive cells; 2 stands for between 50 and 75 BAG3-positive cells; 3 stands for between 75 to 100 BAG3-positive cells.
Results
The quality of viral RNA in SHIV-infected macaques
Eight macaques were infected with SHIV−162.P4, a molecularly cloned virus. The quantity of viral RNA was measured in peripheral blood at the time of autopsy. As shown in , the infected macaques showed high viral loads, as well as weight loss, prior to death and autopsy. Four uninfected macaques (#10-13) were used as controls.
BAG3 expression is increased in astrocytes in the frontal cortex of SHIV-infected macaques
Expression of BAG3 in the frontal cortex of SHIV-infected macaques was measured by IHC. The BAG3 expression was increased in the frontal cortex of SHIV-infected group compared with control group ((A–C); ). To investigate the expression of BAG3 in astrocytes, we performed double-labeled IHC for BAG3 in combination with GFAP. Increased BAG3 expression was observed in astrocytes of SHIV-infected group compared with control ((D–F)). Then, we randomly selected four SHIV-infected macaques and two control macaques to analyze BAG3 protein and mRNA levels. The BAG3 protein levels were increased in the frontal cortex of SHIV-infected group compared with control group ()), consistent with our IHC observation. However, we did not find any significant changes in BAG3 mRNA levels between SHIV-infected group and control group ()). These results suggested that the protein expression of BAG3 was increased in astrocytes in the frontal cortex of SHIV-infected macaques.
Figure 1. BAG3 is increased in astrocytes in the frontal cortex of SHIV-infected macaques
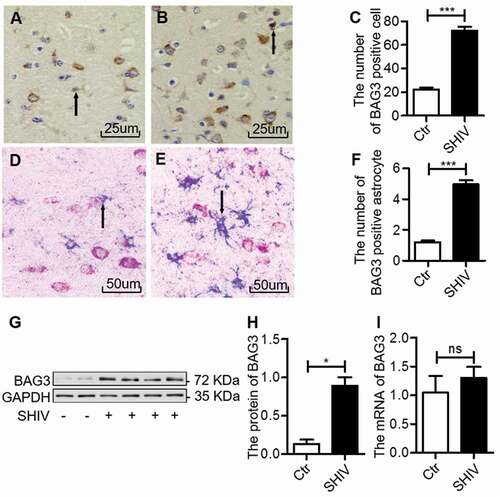
HIV-1 Tat upregulates BAG3 in U87 cells
In order to explore the association between HIV-1 Tat and BAG3, U87 cells were transfected with plasmids pcDNA3.1-HA or pcDNA3.1-Tat-HA for 48 hours, and the expression of HIV-1 Tat was measured by western blotting. Increased HIV-1 Tat expression was only seen in pcDNA3.1-Tat-HA-transfected group (Figure 2(A)). Next, we transfected U87 cells with the above plasmids, and analyzed BAG3 protein and mRNA levels. We observed that HIV-1 Tat upregulated the BAG3 protein levels, which peaked at 48 hours ()). However, we did not find any significant changes in BAG3 mRNA levels ()). These results suggested that HIV-1 Tat could only upregulate the protein level of BAG3 in U87 cells.
Figure 2. HIV-1 Tat upregulates BAG3 in U87 cells
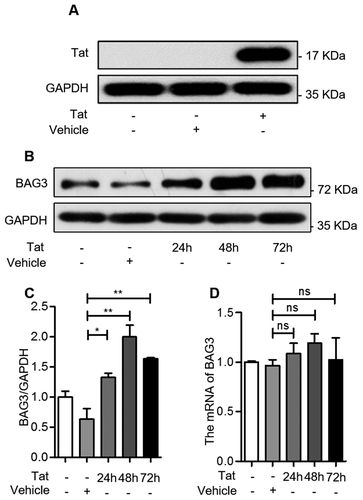
HIV-1 Tat upregulates BAG3 via NF-κB signaling in U87 cells
To address how HIV-1 Tat upregulates BAG3, we transfected U87 cells with pcDNA3.1-Tat-HA or pcDNA3.1-HA for 48 hours, and analyzed IκBα and p65 proteins. As shown in ), HIV-1 Tat induced phosphorylation and degradation of IκBα. In addition, HIV-1 Tat induced p65 nuclear translocation and increased levels of phosphorylated p65 ()), indicating that HIV-1 Tat activates the NF-κB signaling.
Figure 3. HIV-1 Tat activates the NF-κB signaling
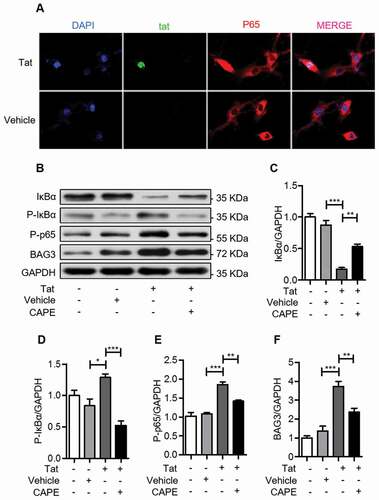
To evaluate the role of NF-κB signaling in HIV-1 Tat-induced upregulation of BAG3, we treated U87 cells with the NF-κB inhibitor CAPE, following transfection with pcDNA3.1-Tat-HA. CAPE treatment increased the levels of IκBα ()), while phosphorylated IκBα and phosphorylated p65 were decreased ()). In addition, CAPE inhibited the HIV-1 Tat-induced upregulation of BAG3 ()), indicating that HIV-1 Tat upregulates BAG3 via the NF-κB signaling.
HIV-1 Tat induces autophagy by BAG3-dependent manner
In order to investigate whether the HIV-1 Tat-induced upregulation of BAG3 was associated with a stimulation of autophagy, U87 cells were transfected with pEGFP-C3-BAG3-GFP, and treated with Baf-A1, an inhibitor of autophagy. BAG3 induced expression of the autophagy marker LC3B-II ()), when the degradation of autophagy-lysosome was inhibited by Baf-A1, suggesting that BAG3 enhances autophagosome formation. Then, we transfected U87 cells with GV112-BAG3-shRNA before pcDNA3.1-Tat-HA. In this experiment, we discovered that HIV-1 Tat could induce LC3B-II and decrease p62 ()), suggesting that autophagy was induced by HIV-1 Tat. In addition, the HIV-1 Tat-induced autophagy was decreased when BAG3 expression was suppressed ((C–F)), suggesting that HIV-1 Tat upregulates BAG3 and induces autophagy. Similar effects were observed in CAPE-treated cells (–J)), further supporting the conclusion that the HIV-1 Tat-induced autophagy is mediated by NF-κB-regulated BAG3 in U87 cells.
Figure 4. HIV-1 Tat induces autophagy by BAG3-dependent manner
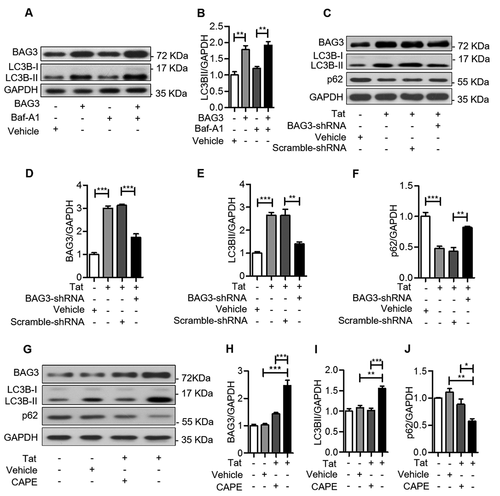
Discussion
We have previously reported that astrocytes in the frontal cortex are involved in the pathogenesis of HAND [Citation14,Citation15]. In this study, we examined the role of BAG3 in astrocytes in the pathogenesis of HIV-1 Tat-induced autophagy during HAND using immun-ohistochemistry, immunofluorescence, western blot, qRT-PCR, and RNA interference.
Firstly, we analyzed the frontal cortex tissues from SHIV-infected macaques. SHIV-infected macaques are considered as the animal model of HAND because there are striking similarities between SHIV-induced disease in macaques and HIV-1-induced disease in humans. For example, SHIV-infected macaques exhibit symptoms that are similar to clinical manifestations of HAND, such as loss of appetite, unresponsiveness, and sluggishness. In addition, previous studies show that increased BAG3 is elicited by HIV-1 infection in human fetal microglial cells, which may provide a new sight for the suppression of HIV-1 gene expression [Citation10]. Our results demonstrate that BAG3 increase in SHIV-infected astrocytes, suggesting that BAG3 might be involved in the pathogenesis of HAND.
In our previous study, we have found that HIV-1 Tat upregulates AEG-1 to inhibit EAAT-2 expression in astrocytes, which might contribute to the development of HAND [Citation15]. Therefore, we speculated that abnormal expression of BAG3 in astrocytes might be also caused by HIV-1 Tat. HIV-1 Tat is one of the most important HIV-encoded proteins, and as a trans-activator of transcription, plays a pivotal role in the viral transcription [Citation10], and development of HAND [Citation15,Citation16]. In transgenic mouse, the expression of HIV-1 Tat in astrocytes causes learning and memory deficits [Citation17], and is associated with thinning of the cerebral cortex [Citation18] and synaptodendritic injury [Citation19]. Our results show that overexpression of HIV-1 Tat upregulates the protein levels of BAG3 in U87 cells that are widely used to study the astrocyte function [Citation15,Citation20,Citation21]. These results are consistent with the findings of Anna Paola Bruno et al that HIV-1 Tat raises BAG3 at the post-transcriptional levels in U87 cells [Citation22].
Previous reports have indicated that LPS induces BAG3 by NF-κB-dependent manner in human monocytic cells, and HIV-1 Tat-induced human umbilical vein endothelial cells (HUVEC) proliferation and microtubule formation depend on NF-κB signaling [Citation23]. Therefore, we surmise that HIV-1 Tat upregulates BAG3 depending on NF-κB signaling. Our results indicate that overexpression of HIV-1 Tat induces phosphorylation and degradation of IκBα, resulting in p65 nuclear translocation and NF-κB-dependent upregulation of BAG3 in U87 cells. The role of NF-κB in the HIV-1 Tat-induced upregulation of BAG3 is further supported by our data demonstrating that CAPE, a potent and specific inhibitor of NF-κB activation [Citation24], inhibits the HIV-1 Tat-induced upregulation of BAG3.
What are the consequences of HIV-1 Tat induced upregulation of BAG3? In recent years, BAG3 has received an increased attention due to its increased expression in several disease models, and its proposed role in autophagy. Moreover, HIV-1 Tat has been demonstrated to disrupt autophagy in immune cells [Citation25]. There is an increasing evidence to suggest that enhanced autophagy leads to neurite degeneration [Citation26]. Autophagy is involved in both neuroprotection and neurodegeneration, and it has been implicated in a number of neurological diseases including Alzheimer’s disease, Parkinson’s disease, Huntington’s disease and HAND [Citation27]. Using pcDNA3.1-Tat-HA, our results demonstrate that HIV-1 Tat upregulates LC3B and downregulates p62. LC3B is a marker of autophagy, and positivity correlates with the level of autophagy [Citation28]. p62 is one of the selective substrates for autophagy [Citation29], the amount of which negativity correlates with the level of autophagy. Thus, our results demonstrate that autophagy is activated after HIV-1 Tat overexpression in U87 cells. Furthermore, we have found that after downregulating BAG3, the ability of HIV-1 Tat to promote autophagy is inhibited, demonstrating that the HIV-1 Tat induced autophagy is mediated by BAG3. Interestingly, our results showed similar trends with other studies, in which increased autophagy is induced by HIV-1 Tat in human glial cells [Citation22]. Of note, from the study of Rodriguez et al, autophagy is involved in the process of HIV-1 Tat-induced inflammatory cytokines in astrocytes [Citation30]. In addition, our previous have showed that IL-1β and TNF-α were detected in astrocytes from postmortem brains of patients with HIV-1 encephalitis [Citation31]. Thus, we speculate that the expression of IL-1β and TNF-α were induced by HIV-1 Tat in astrocytes with HIV-1 encephalitis, in which autophagy might participate.
In conclusion, our results demonstrate for the first time that HIV-1 Tat upregulates BAG3 via NF-κB signaling, and BAG3 mediates autophagy induced by HIV-1 Tat. A better understanding of the effect of HIV-1 Tat induced-autophagy on astrocyte function and HAND progression should provide a new insight into the HAND pathogenesis.
Acknowledgments
We thank Prof. Zhou Q. from the Department of Molecular and Cell Biology at the University of California, Berkeley, CA, USA; Xue Y. from the School of Pharmaceutical Sciences at Xiamen University, Xiamen, Fujian, China; Prof. Zhang J. Prof. Zhang Y. and Qiu J. from the School of Medical College at Xiamen University, Xiamen, Fujian, China for their help.
Disclosure statement
No potential conflict of interest was reported by the authors.
Additional information
Funding
References
- Masliah E, Heaton RK, Marcotte TD, et al. Dendritic injury is a pathological substrate for human immunodeficiency virus-related cognitive disorders. HNRC Group. HIV Neurobehav Res Center Ann Neurol. 1997;42:963–972.
- Zhou D, Masliah E, Spector SA. Autophagy is increased in postmortem brains of persons with HIV-1-associated encephalitis. J Infect Dis. 2011;203:1647–1657.
- Meulendyke KA, Croteau JD, Zink MC. HIV life cycle, innate immunity and autophagy in the central nervous system. Curr Opin HIV AIDS. 2014;9:565–571.
- Sardo L, Iordanskiy S, Klase Z, et al. HIV-1 Nef blocks autophagy in human astrocytes. Cell Cycle. 2015;14:3781–3782.
- Qi L, Gang L, Hang KW, et al. Programmed neuronal cell death induced by HIV-1 tat and methamphetamine. Microsc Res Tech. 2011;74:1139–1144.
- Cai NS, Cadet JL. The combination of methamphetamine and of the HIV protein, Tat, induces death of the human neuroblastoma cell line, SH-SY5Y. Synapse. 2008;62:551–552.
- Behl C. BAG3 and friends: co-chaperones in selective autophagy during aging and disease. Autophagy. 2011;7:795–798.
- Rosati A, Graziano V, De Laurenzi V, et al. BAG3: a multifaceted protein that regulates major cell pathways. Cell Death Dis. 2011;2:e141.
- Rosati A, Khalili K, Deshmane SL, et al. BAG3 protein regulates caspase-3 activation in HIV-1-infected human primary microglial cells. J Cell Physiol. 2009;218:264–267.
- Rosati A, Leone A, Del Valle L, et al. Evidence for BAG3 modulation of HIV-1 gene transcription. J Cell Physiol. 2007;210:676–683.
- Polacino P, Larsen K, Galmin L, et al. Differential pathogenicity of SHIV infection in pig-tailed and rhesus macaques. J Med Primatol. 2008;37(Suppl 2):13–23.
- Xing HQ, Moritoyo T, Mori K, et al. Expression of proinflammatory cytokines and its relationship with virus infection in the brain of macaques inoculated with macrophage-tropic simian immunodeficiency virus. Neuropathology. 2009;29:13–19.
- Xing HQ, Moritoyo T, Mori K, et al. Simian immunodeficiency virus encephalitis in the white matter and degeneration of the cerebral cortex occur independently in simian immunodeficiency virus-infected monkey. J Neurovirol. 2003;9:508–518.
- Xing HQ, Mori K, Sugimoto C, et al. Impaired astrocytes and diffuse activation of microglia in the cerebral cortex in simian immunodeficiency virus-infected macaques without simian immunodeficiency virus encephalitis. J Neuropath Exp Neur. 2008;67:600–611.
- Ye X, Zhang Y, Xu Q, et al. HIV-1 Tat inhibits EAAT-2 through AEG-1 upregulation in models of HIV-associated neurocognitive disorder. Oncotarget. 2017;8:39922–39934.
- Wang J, Zhang Y, Xu Q, et al. Menin mediates Tat-induced neuronal apoptosis in brain frontal cortex of SIV-infected macaques and in Tat-treated cells. Oncotarget. 2017;8:18082–18094.
- Carey AN, Sypek EI, Singh HD, et al. Expression of HIV-Tat protein is associated with learning and memory deficits in the mouse. Behav Brain Res. 2012;229:48–56.
- Carey AN, Liu X, Mintzopoulos D, et al. Conditional Tat protein expression in the GT-tg bigenic mouse brain induces gray matter density reductions. Prog Neuropsychopharmacol Biol Psychiatry. 2013;43:49–54.
- Fitting S, Ignatowska-Jankowska BM, Bull C, et al. Synaptic dysfunction in the hippocampus accompanies learning and memory deficits in human immunodeficiency virus type-1 Tat transgenic mice. Biol Psychiatry. 2013;73:443–453.
- Hu B, Emdad L, Bacolod MD, et al. Astrocyte elevated gene-1 interacts with Akt isoform 2 to control glioma growth, survival, and pathogenesis. Cancer Res. 2014;74:7321–7332.
- Ye ZC, Rothstein JD, Sontheimer H. Compromised glutamate transport in human glioma cells: reduction-mislocalization of sodium-dependent glutamate transporters and enhanced activity of cystine-glutamate exchange. J Neurosci. 1999;19:10767–10777.
- Bruno AP, De Simone FI, Iorio V, et al. HIV-1 Tat protein induces glial cell autophagy through enhancement of BAG3 protein levels. Cell Cycle. 2014;13:3640–3644.
- Yao S, Hu M, Hao T, et al. MiRNA-891a-5p mediates HIV-1 Tat and KSHV Orf-K1 synergistic induction of angiogenesis by activating NF-kappaB signaling. Nucleic Acids Res. 2015;43:9362–9378.
- Natarajan K, Singh S, Burke TR Jr., et al. Caffeic acid phenethyl ester is a potent and specific inhibitor of activation of nuclear transcription factor NF-kappa B. Proc Natl Acad Sci U S A. 1996;93:9090–9095.
- Van Grol J, Subauste C, Andrade RM, et al. HIV-1 inhibits autophagy in bystander macrophage/monocytic cells through Src-Akt and STAT3. PLoS One. 2010;5:e11733.
- Yang Y, Fukui K, Koike T, et al. Induction of autophagy in neurite degeneration of mouse superior cervical ganglion neurons. Eur J Neurosci. 2007;26:2979–2988.
- Cherra SJ III, Chu CT. Autophagy in neuroprotection and neurodegeneration: A question of balance. Future Neurol. 2008;3:309–323.
- Kabeya Y, Mizushima N, Ueno T, et al. LC3, a mammalian homologue of yeast Apg8p, is localized in autophagosome membranes after processing. EMBO J. 2000;19:5720–5728.
- Ichimura Y, Komatsu M. Selective degradation of p62 by autophagy. Semin Immunopathol. 2010;32:431–436.
- Rodriguez M, Lapierre J, Ojha CR, et al. Importance of autophagy in mediating Human Immunodeficiency Virus (HIV) and morphine-induced metabolic dysfunction and inflammation in human astrocytes. Viruses. 2017;9:201.
- Xing HQ, Hayakawa H, Izumo K, et al. In vivo expression of proinflammatory cytokines in HIV encephalitis: an analysis of 11 autopsy cases. Neuropathology. 2009;29:433–442.