ABSTRACT
A tight link has been established between inflammation and cancer. Liver regeneration is a widely used model to study the correlation between inflammation and proliferation. IL-6 is essentially involved in liver regeneration and in cancer. Recently, IL-17A has been shown to regulate not only inflammation, but also cell proliferation. Here, we analyze the role played by IL-17A signaling in liver regeneration by comparing cell proliferation in Wild Type and IL-17RA−/− mice. Partial hepatectomy experiments performed in IL-17RA−/− mice showed a delay in expression of early-genes to prime the residual hepatocyte to proliferate, with subsequent delay in G1/S-phase transition. We demonstrated that IL-17RA regulates, by recruitment of non-parenchymal cell, the expression of IL-6, which in turn triggers the proliferation of residual hepatocytes. Our data indicate an important role played by IL-17RA in liver proliferation via IL-6.
Introduction
The linkage between inflammation and cancer has been extensively studied and cytokines have been ascribed as fundamental in tumor growth [Citation1–Citation4] and metastasis [Citation5–Citation7]. In particular, IL-6 has been described as an important regulatory molecule involved in a number of cellular and molecular processes in cancer and, inter alia, elevated levels of IL-6 are associated with aggressive tumor growth [Citation8–Citation11]. Liver regeneration represents a widely used in vivo proliferation model to study inflammation and proliferation. In mammals, the liver is the most important organ for the homeostasis maintenance of the whole body. Among all the organs, the liver presents the peculiarity to reconstitute the original mass by regeneration. Following injury, such as partial removal of the liver mass, the residual hepatocytes begin to proliferate in a synchronous way until the complete hepatic mass reconstitution. The ability of the residual hepatocytes to begin the proliferative process starting from a quiescent state has been extensively studied as an experimental method for studying cell proliferation in vivo after Partial Hepatectomy (PH) [Citation12]. Particularly, it represents an important tool to study the role played by inflammatory cells and related cytokines, in modulating the priming of residual hepatocytes proliferation [Citation13–Citation16]. Different factors have been identified to play a role in liver regeneration [Citation13,Citation17–Citation22].
With the introduction of the studies on Knock-Out (KO) mice, many authors have indicated a number of genes and pathways that could affect liver regeneration. A significant explanation of the complex mechanisms concerning the liver regeneration has been prospected by Nelson Fausto [Citation15]. He suggested that three different types of pathways, cytokines, growth and metabolic factors act together orchestrating liver functions and proliferation. The key event in liver regeneration is the priming of residual hepatocytes. The hepatic cells transit from G0 to G1 and become responsive to growth factors proliferating to restore the original mass. Many investigations have determined the role played by inflammatory cytokines IL-6 and TNFα, which in turn activate the transcription factor Nuclear Factor-kappa B (NF-κB) and Signal Transducer and Activator of Transcription 3 (Stat3) [Citation23–Citation26]. NF-κB, Stat3 and the second messenger cAMP [Citation27–Citation29] act on the residual hepatocytes to transcribe early-genes (such as c-fos, c-myc, c-jun) that trigger liver regeneration [Citation30]. Indeed, a fundamental role has been ascribed to IL-6 [Citation31]. IL-6 seems to control the transcription of 40% of the early-genes expressed after PH [Citation32]. Notably, an important function seems to be played by the serum concentration of IL-6.
After the priming phase a number of genes and metabolic pathways have been activated [Citation33–Citation36]. IL-17A is considered a key player in regulating inflammatory process [Citation37,Citation38]. It is a pro-inflammatory cytokine, which regulates many inflammatory process and associated cytokines [Citation39]. Recently, it has been described a role played by liver γδT cells during hepatic regeneration, through the increase of IL-17A and IL-22 levels. The authors demonstrated that in mice, the lacking of TCRδ gene is associated with delay in liver regeneration [Citation40]. Indeed, they claim that via IL-22 and IL-17A, sharing a direct mitogenic effect on hepatocytes, γδT cells promote a pro-regenerative phenotype of Kupffer cells, DCs and neutrophils [Citation41,Citation42]. Because the role of IL-17A and IL-22 produced by inflammatory cells in priming residual hepatocytes proliferation, we analyzed the liver regeneration in mice where IL-17 Receptor A (IL-17RA) is deleted [Citation40]. We dissected the role played by IL-17A in liver regeneration and analyzed the ability of IL-17A to prime the residual hepatocytes and the resulting effect on proliferation. The data indicate an involvement of IL-17A alone, in controlling the liver regeneration of residual hepatocytes, even in the absence of IL-22 cooperative effect.
Results
Serum cytokine analysis in wild type and IL-17RA−/−mice
To explore the role of IL-17RA in activating residual hepatocytes by recruiting pro-inflammatory cells to promote liver regeneration, we analyzed the serum level of cytokines involved in the first hours of liver regeneration.
Liver regeneration was induced by PH and studied in Wild Type (WT) and IL-17RA Knock-Out (IL-17RA−/−) mice at 2, 6, 36 and 48 after PH. No significant difference in estimated liver weight/initial body weight in relationship to regenerated liver/final body weight of animals, between the two groups (WT and IL-17RA−/−) examined has been found (Supp. ).
Figure 1. Pro-regenerative inflammatory mediators level in WT and IL-17RA−/− mice sera in liver regeneration after PH.
(a) IL-17 level in serum of WT and IL-17RA−/− mice. (b) IL-22 level in serum of WT and IL-17RA−/− mice. (c) TNFα level in serum of WT and IL-17RA−/− mice. D) IL-6 level in serum of WT and IL-17RA−/− mice. Bars represent means ±SD (n = 3). Experiments were repeated 3 times using 3 mice per group.
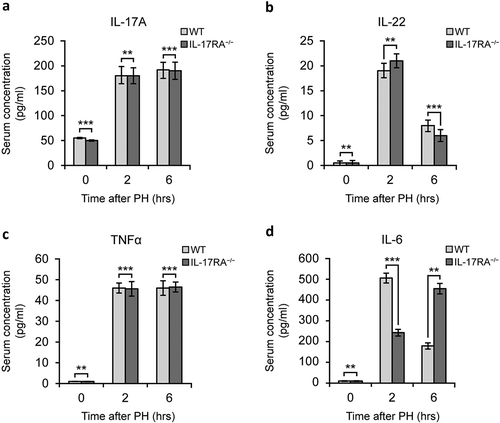
At first, we compared the level of IL-17A in serum of WT and IL-17RA−/− mice (). The analysis of IL-17A was carried out at 0, 2 and 6 hours after PH. The sera analyzed comparing WT and IL-17RA−/− showed no difference between the samples observed. Because the cooperative role of IL-17A and IL-22 in priming liver regeneration, we measured the level of IL-22 in the same samples. Even in this case no difference was appreciated between the two strains of mice (). Similarly, analysis has been performed to evaluate two other pivotal cytokines in priming of residual hepatocytes: TNFα and IL-6. While serum level of TNFα did not show difference in WT and IL-17RA−/− mice ()), surprisingly, IL-6 showed great difference in serum concentration 2 and 6 hours after PH. In particular, we observed a discrepancy between the WT and IL-17RA−/−.
IL-6 evaluation after PH showed an increase in serum levels in both mice strains but an opposite progression. Two hours after PH, we observed in IL-17RA−/− mice serum samples an absolute value of IL-6 of 220 pg/ml, about 50% less than the WT counterpart (500 pg/ml). Generally, 6 hours after PH, when the priming of hepatocytes has occurred, IL-6 serum level drops to low levels, as we observed in WT mice (190 pg/ml, about 60% less). Conversely in IL-17RA−/− we recorded a further increase in IL-6 level rising 50% if compared to 2 hours level and 60% higher than WT samples at the same time point ()).
Early-genes expression analysis
Because specific serum concentration of IL-6 is necessary to induce priming in residual hepatocytes, we asked whether this discrepancy in IL-17RA−/− at 2 hours after PH could in turn affect the expression of early-genes responsible for the trigger of proliferative process. To this aim we analyzed at first whether IL-6 concentration in residual hepatocytes of WT and IL-17RA−/− mice were sufficient to activate Stat3 (). Stat3 activation is critical for the control of expression of the early-genes involved in priming of proliferation of residual hepatocytes. Notably, we observed that while in WT liver the Stat3 phosphorylation follows the canonical activation, we noted a reduced phosphorylation in liver of IL-17RA−/− mice ()). Moreover, we analyzed by immunohistochemistry the Stat3 localization comparing the liver at 0 and at two hours after PH in WT and IL-17RA−/− mice. At 2 hours, in WT liver we observed, as expected, a Stat3 nuclear localization, while a very low amount of Stat3 has been detected in nucleus of IL-17RA−/− cells ()). We performed the analysis of c-Fos, c-Jun and Icer mRNA from samples at 0, 2 and 6 hours after PH. The results showed the classical induction of c-Fos and c-Jun in WT mice, showing an increase at 2 hours followed by a restoration of normal level at 6 hours after PH. The classical raise after PH was displayed in Icer expression. Interestingly, the analysis performed in IL-17RA−/− showed a reduced induction of c-Fos and Icer with respect to WT, while the expression of c-Jun does not seem to be triggered in the first hours after PH in IL-17RA−/− samples ()).
Figure 2. Evaluation of the Inflammatory Factor STAT3, the transcription factors cFos and cJun and the repressor of transcription factors Icer in liver of WT and IL-17RA−/− mice during liver regeneration.
(A) WB analysis of STAT3 protein expression during liver regeneration in WT and IL-17RA−/− mice. The protein amount was normalized to GAPDH protein expression. Representative data and averages of 3 replicates were shown. Experiments were repeated 3 times using 3–5 mice per group (left). Graph represents pSTAT3/STAT3 ratio of immunoblotting signal by densitometric quantification (right). (B) Analysis of STAT expression and localization in WT and IL-17RA−/− mice after PH. Representative micrographs of liver sections, immunostained using monoclonal STAT antibody (red) and DAPI (blue). Representative merge images were shown (right). Bars indicate 10 μm. Magnification, 400X. (C) mRNA levels of cFos, cJun and Icer expression, detected by qPCR in WT and IL-17RA−/− mice during liver regeneration at the indicated times following PH. The expression of each gene was normalized to mouse Gapdh levels. The mRNA amount was expressed in relative fold of expression. Bars represent means ±SD (n = 3). Experiments were repeated 3 times using 3 mice per group.
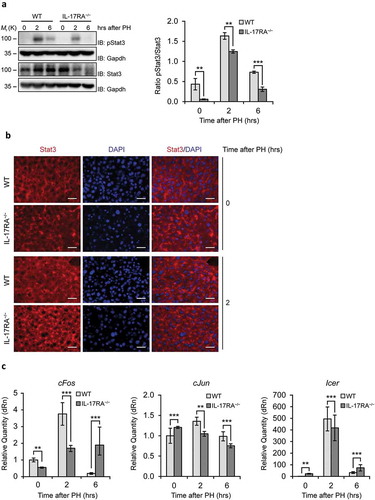
IL-17RA−/− deficiency leads to impaired hepatocyte proliferation
These results open the question whether reduced induction in priming of residual hepatocytes IL-17RA−/− is accompanied with altered liver regeneration.
At first, we investigate DNA synthesis during liver regeneration in WT and IL-17RA−/− mice. In order to evaluate hepatocytes proliferation, DNA synthesis was measured in the nuclei of hepatocytes from WT and IL-17RA−/− mice, as bromodeoxyuridine (BrdU) incorporation, injected 2 hours prior to the sacrifice. The total number of BrdU positive nuclei at all time points analyzed was significantly reduced in IL-17RA−/− compared to WT mice. As expected, in WT mice we observed intense peak of BrdU incorporation at 36 hours after PH, at the onset of S-phase of residual hepatocytes, with a robust reduction at 48 hours after PH. The same analysis performed in IL-17RA−/− mice revealed a negligible amount of BrdU incorporation at 36 hours with a weak increase of BrdU incorporation at 48 hours after PH, suggesting a delay in the regeneration process ()). To better understand the role of IL-17 in triggering proliferation, we analyzed the expression of important molecule related to the G1/S transition during liver regeneration: Cyclin D1. Cyclin D1 mRNA expression in WT mice presented a peak of expression at 36 hours, followed by a slow, progressive decrease. Conversely in IL-17RA−/− mice Cyclin D1 mRNA expression level was maintained low to reach the highest level 48 hours after PH ()). The analysis of an important regulator of cell cycle such as p27, showed in WT a kinetic similar to that previously described [Citation43], with a slight increase in expression during the first hours of liver regeneration and during the G1/S transition. Interestingly, a down-regulation of p27 has been detected in IL-17RA−/− mice through the regenerating liver ()).
Figure 3. Defective proliferation in IL-17RA−/− mice regenerating livers.
(a) Quantitative analysis of BrdU labeling index (% of BrdU-positive hepatocytes) at each time of liver regeneration in WT and IL-17RA−/− mice. (left). Representative micrographs of liver sections collected from WT and IL-17RA−/− mice after PH, immunostained with anti-BrdU antibody (green) and DAPI (blue). Representative merge images were shown (right). The arrows indicate the BrdU-positive cells in a representative way. Bars indicate 10 μm. Magnification, 200X. (b) Expression of markers of G1/S phase during liver regeneration in WT and IL-17RA−/− mice. mRNA levels of Cyclin D1 and p27 were detected by qPCR in WT and IL-17RA−/− mice during liver regeneration following PH. The expression of each gene was normalized to mouse Gapdh levels. The amount of mRNA is expressed in relative fold of expression. Bars represent means ±SD (n = 3). Experiments were repeated 3 times using 3 mice per group. (c) Percentage of pH3-positive cells during liver regeneration in WT and IL-17RA−/− mice (right). Representative micrographs of liver sections, collected from WT and IL-17RA−/− mice after PH, immunostained with monoclonal pH3 antibody (green) and DAPI (blue). Representative merge images were shown (right). Bars indicate 10 μm. Magnification, 400X.
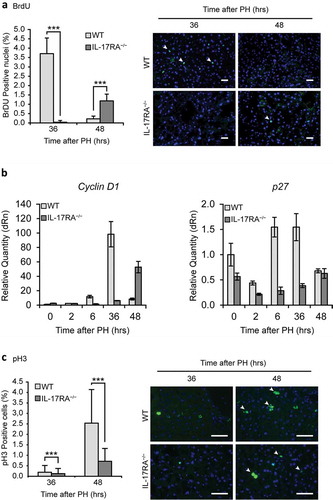
To corroborate the difference in proliferation in IL-17RA−/− with respect to WT mice, we compared the percentage of cells in G2/M phase after of BrdU incorporation.
To this aim, the expression of H3 Histone phosphorylated form (Ser-10, pH3) was analyzed by immunohistochemistry. Fluorescence analysis, by using a polyclonal antibody to pH3, revealed that the percentage of cells in mitotic phase in WT liver were low at 36 hours after PH, showing the classical increase at 48 hours, when parenchymal cells reached the maximum of mitotic figures. In both groups of mice, hepatocytes proliferated and divided at 48 hours after PH, even though in IL-17RA−/− mice the percentage of cells expressing pH3 was remarkably lower with respect to WT mice. The analysis performed in IL-17RA−/− mice showed an improvement in mitotic cell at 48 hours with respect to 36 hours after PH with a significant reduction of about 60% with respect to WT mice ()).
The data obtained by the analyzes of cyclin confirm the histological analysis performed in regenerating liver tissue, suggesting that the proliferation of residual hepatocytes is strongly influenced by IL-17A. All these results indicate that altered priming in the first hours after PH is linked to the delay of the G1/S transition observed in residual hepatocytes of IL-17RA−/− mice.
Involvement of non-parenchymal cells
To evaluate the possible role played by non-parenchymal cells in liver regeneration, at first, we analyzed in WT liver, whether the expression of IL-17RA is modulated after PH. Interestingly, no difference in expression has been detected in liver by qPCR at different times after PH ()). Moreover, we evaluated eventual Il-17A expression in liver of WT and IL-17RA−/−. In both mice population Il-17A was no detected in liver (data not shown).
Figure 4. Gene Expression of IL-17A and downstream genes in liver.
mRNA levels of Il-17RA (a), C/ebpβ (b) and A20 (c) expression, detected by qPCR in WT and IL-17RA−/− mice during liver regeneration at the indicated times following PH. The expression of each gene was normalized to mouse Gapdh levels. The mRNA amount was expressed in relative fold of expression. Bars represent means ±SD (n = 3). Experiments were repeated 3 times using 3 mice per group. (d) Analysis of A20 expression and localization in WT and IL-17RA−/− mice after PH. Representative micrographs of liver sections, immunostained using monoclonal A20 antibody (green) and DAPI (blue). Representative merge images were shown (right). Bars indicate 10 μm. Magnification, 400X. A larger inset of stained areas was shown (bottom). Arrows indicate the nuclear staining of cells in Disse space.
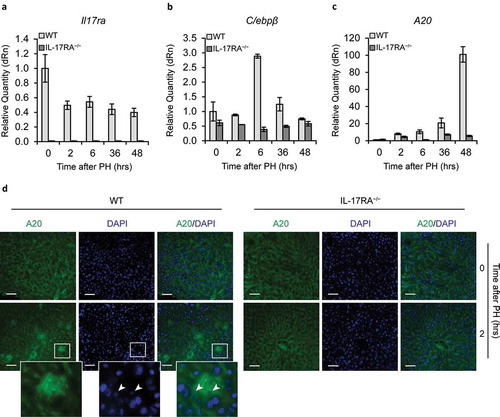
To investigate a potential activation of the liver non-parenchymal cells by IL-17A in proliferative process of liver regeneration, we analyzed in WT and in IL-17RA−/− livers the expression of C/ebpβ and A20 genes specifically activated by IL-17A pathway [Citation44]. Notably, comparing the expression of C/ebpβ ()) and A20 ()) in WT and in IL-17RA−/− mice liver, we found a great difference in expression between the two populations. In particular, we found in WT a rapid activation of both genes at 6 hours, whereas A20 progressively increases its expression in the following hours, with a maximum at 48 hours ()). Recently, a key role has been attributed to A20 in liver regeneration [Citation45]. In IL-17RA−/− mice the expression of C/ebpβ and A20 is not appreciable in all the hours examined. Moreover, to establish the localization of the cells expressing A20 in the liver, an immunohistochemical analysis has been performed comparing the livers at 0 and 2 hours after PH in WT and IL-17RA−/− mice. At 0 hours A20 amount is negligible in both WT and IL-17RA−/− liver. At two hours after PH in WT, a high level of expression has been detected in Disse space, while any increase has been observed in the neighboring hepatocytes. An insignificant A20 expression has been observed in IL-17RA−/− mice ()) [Citation46]. These data indicate a clear involvement of IL-17A in recruitment of liver non-parenchymal cells with impairment in the activation of IL-17A pathway between WT and IL-17RA−/− which in turn could regulate the proliferation of residual hepatocytes after PH.
Discussion
Liver regeneration is an intricate phenomenon characterized by complex and coordinate pathways acting to induce proliferation of residual hepatocytes. Many studies have clarified the role played by inflammatory cytokines to prime the cell proliferation of hepatocytes and non-parenchymal cells.
This study demonstrated for the first time the role played by IL-17RA in liver regeneration and in particular in the priming of the residual hepatocytes to proliferate. In the first hours after PH, lack of IL-17RA showed a reduced recruitment of non-parenchymal cells activation, which in turn was responsible of the observed delay in the increase of IL-6 serum concentration. Indeed, the other analyzed cytokines, such as TNFα, IL-17 and IL-22, did not show difference when comparing WT and IL-17RA−/− mice. Differences in IL-6 concentration in the first 2 hours after PH are crucial for the priming of residual hepatocytes. Many authors have ascertained in different KO mice models, that low concentration of IL-6 or IL-6 pathway inactivation, affected the liver regeneration. They demonstrated that altered IL-6 level are unable to stimulate appropriate proliferation in vivo. In our experimental model, we established that IL-17RA has a pivotal role in triggering proliferation of residual hepatocytes. In fact, in IL-17RA−/− mice, we found low IL-6 serum concentration that in the first hours after PH is not able to activate Stat3, which result hypo-phosphorylated with respect to WT. The reduced activation of Stat3 reflects in turn a low expression of the immediate early-genes that are responsible for the priming of residual hepatocytes proliferation. The lack of the priming determines, as expected, a different activation of the proliferation, identified in the G1/S transition as observed in IL-17RA−/− liver.
Recently, it has been revealed a role played by IL-17A/IL-22–producing T cells in orchestration of hepatic regeneration by regulating the recruitment of various hepatic leukocytes. Indeed, the authors highlighted a double action played by IL-17A and IL-22 in liver regeneration. In our experimental model we adduce an important role for IL-17RA in modulating liver regeneration. The level of IL-17A and IL-22 are normal, while the lack of the IL-17A receptor alone is accompanied by an incorrect proliferation during liver regeneration. Our data let us suppose an important role played by non-parenchymal cells to sustain the liver regeneration. We defined the role of IL-17A as an important cytokine to recruit the liver non-parenchymal cells to express IL-6, which in turn modulate the priming of residual hepatocytes to proliferate. Certainly, the normal serum concentration of TNFα and IL-22, found in IL-17RA−/− with respect to WT, let us assume that the coordination of the first proliferative wave by IL-17A is determined by the critical concentration of IL-6 in serum. Many authors have described the delay in liver regeneration related to altered involvement of inflammatory cells in proliferation. Kupffer cells are undoubtedly one of the most important cells controlling the rate of proliferation of residual hepatocytes by producing cytokines implicated in priming of hepatocytes. Indeed, other components of innate immunity seem to have a crucial role in controlling the liver regeneration. Hepatic γδT cells production of IL-17A and IL-22 cytokines seemed to have a regulatory effect on hepatocytes proliferation, while other authors indicate resident CD4+ lymphocytes in the spleen as the most important cells producing IL-17 in regulating liver regeneration. Certainly, a complex model such as liver regeneration offers a great opportunity to evaluate multifaceted in vivo aspects of proliferation to study the intertwinement of cells proliferation and inflammatory system in liver and to understand how growth factors, cytokines and others molecules orchestrate the liver regeneration ().
Figure 5. IL-17A modulates liver regeneration.
Schematic representation in the liver of putative role played by IL-17A in recruitment of non-parenchymal cells and proliferative control after partial hepatectomy.
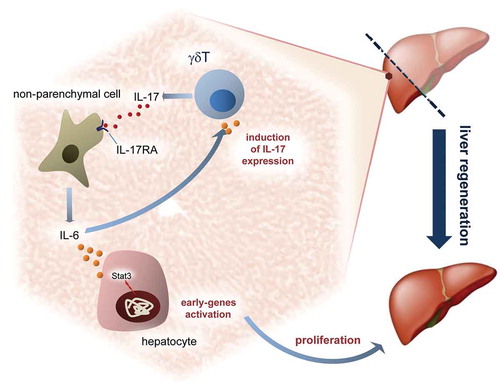
Our data indicate a role played by inflammatory cells in modulating proliferation and points out of the key-role of IL-17A in recruitment of non-parenchymal cells to express IL-6.
High level of IL-6 in serum of patients with cancer are mostly associated with poor prognosis despite to patients with low serum level of IL-6, being it related to a better response to chemotherapy. Additional studies are necessary to understand the role of inflammatory cells and in turn cytokines synthesis in controlling cell proliferation and cancer. A number of studies are now aimed to the use of cytokines and in particular IL-6 as target of specific therapy. Certainly, more investigations need to be performed to shed a light on the fundamental contribute of innate immune system and lymphoid cells on the priming of proliferation in liver regeneration and in cancer.
Materials and methods
Animals
Wild-Type (WT) control C57BL/6, eight- to ten-week old mice, was purchased from Charles River (Calco, Italy). Homozygous IL-17RA knockout (IL-17RA−/−) mice (C57BL/6J) were purchased from Amgen (Amgen Inc., Thousand Oaks, Ca, USA) and were bred under specific pathogen-free conditions at the Animal Facility of the University of Perugia. Animals were housed under standard conditions (12 hrs light/dark cycles) with food and water ad libitum before and after surgery. A number of 3–4 mice for each time point were used for most of the experiments, unless differently specified. All of the animal experiments were carried on in accordance with the National Institutes of Health Guide for the Care and Use of Laboratory Animals. Protocols for the care and use of animals were approved by the University of Perugia Ethical Committee and the European Communities Council Directive 86/609/EEC.
Partial hepatectomy model
Eight- to ten-week-old male IL-17RA−/− and age-matched WT mice were subjected to partial hepatectomy (PH) as previously described [Citation13]. Briefly, PH experiments were performed under deep anesthesia by isoflurane (2-chloro-2-(difluoromethoxy)-1,1,1-trifluoro-ethane) inhalation. Mice were subjected to removal of two-thirds of the liver mass and sacrificed at the indicated times post PH. At the time of sacrifice the regenerating liver was quickly extracted, weighted, and the liver-body weight ratio was calculated. The tissue punches were used for RNA analysis, protein expression study and histological observation.
Histological analysis and immunohistochemistry
Two hours before sacrifice, mice were injected with BrdU as previously described [Citation47]. Subsequently, the regenerated tissue fragments were fixed in 10% formalin and embedded in paraffin after serial dehydration in alcohol for histology, or included in Tissue-Tek® O.C.T.™ Compound (Sakura Finetek Inc, Torrance, CA, USA) and stored at −80°C for immunohistochemistry. Frozen sections were exposed to anti-BrdU (Abcam plc, Cambridge, UK, #Ab8152), anti-pH3 (Cell Signaling Technology, Inc, Danvers, MA, USA #9706), anti-STAT3 (Cell Signaling Technology, Inc, #9139) and anti-A20 (Santa Cruz Biotechnology, Inc, Dallas, Tx, USA, #sc-1666692) antibodies. All antibodies were used according to the manufacturer’s instructions. All the antibodies were counterstained using the appropriate Alexa Fluor® secondary antibodies (Molecular Probes, Inc, Eugene, Or, USA). Slides were examined with a Zeiss Axioplan fluorescence microscope. The images were acquired by using a Spot-2 cooled camera (Diagnostic Instruments, Sterling Heights, MI, USA). Images are representative of 3 independent experiments. Total BrdU and pH3 labeled hepatocytes were determined by counting positively stained versus total cell nuclei in 10 microscope fields (200X and 400X magnification, respectively) per liver section at each experimental point. The hepatocyte proliferation index was calculated as percentage of BrdU positive cell nuclei.
Western blot analysis
Fresh liver tissues and cell samples were homogenized in Laemmli buffer as previously described [Citation47]. The extracts were normalized by Coomassie staining and subsequently separated by SDS-polyacrylamide gel electrophoresis and transferred onto nitrocellulose membrane. After blocking, membranes were incubated with primary antibodies – rabbit anti-phospho-STAT3 (Tyr705, Cell Signaling, #9131), rabbit anti-STAT3, (Cell Signaling, #9139), mouse anti-GAPDH (Sigma-Aldrich Inc., St Louis, Mo, USA, #G8795) – at 4°C overnight, shaking. All antibodies were used according to the manufacturer’s instructions. The membranes were then incubated with horseradish peroxidase-conjugated secondary antibodies (Bio Rad, Hercules, Ca, USA). Detection and quantification of protein bands were performed using the ChemiDoc TMXRS+ System with Image LabTM Software (Bio-Rad).
Quantitative real-time polymerase chain reaction
RNA extraction and qPCR were performed as previously described [Citation48,Citation49]. cDNA was reverse-transcribed from 1µg of RNA using the iScript kit (Bio Rad,). A mix of cDNA from 3–4 mice was used for qPCR experiments. qPCR was performed with SYBR®GreenERTM qPCR SuperMix (Invitrogen, Carlsbad, Ca, USA). The relative amount of mRNA was normalized to Gapdh. The primer sequences are as follows (5ʹ-3ʹ): c-fos: sense: TACTACCATTCCCCAGCCGA; anti-sense: GCTGTCACCGTGGGGATAAA; c-jun: sense: CGATGCCCTCAACGCC, anti-sense: CTTAGGGTTACTGTAGCCGTAGGC; Icer: sense: ATGGCTGTAACTGGAGATGAA; anti-sense: TGGCAAAGCAGTAGTAGGA; Cyclin D1: sense AGAGGCGGATGAGAACAAG; antisense GAGGGTGGGTTGGAAATGA; p27: sense TGACTCGTCAGACAATCCG antisense GCCCTTTTGTTTTGCGAAGAA; Il-17: sense AGGAGGGGCTGAGCTGCA; antisense GGAAGACGGGGTCAGGTTTT; C/ebpβ: sense TTCCTCTCCGACCTCTTC; antisense GCTCACGTAACCGTAGTC; A20: sense GACACTCGGAACTGGAATGAC; antisense TCTGAGGATGTTGCTGAGGA; Gapdh: sense: AGGCCGGTGCTGAGTATGTC, anti-sense: TGCCTGCTTCACCACCTTCT.
All values were relative to those of Gapdh mRNA levels at each time point. Bars represent the mean ±standard deviation (SD) (n = 3 technical replicates).
Enzyme-linked immunosorbent assay
Blood samples were drawn from the portal vein 2 and 6 hours after PH. The sera were collected for the tests. Serum IL-6, IL-17, IL-22 and TNFα levels were measured by enzyme-linked immunosorbent assay (ELISA). Assays were performed following the manufacturer’s instructions (Invitrogen). The detection limits of the assays were <8 pg/ml for IL-17A and IL-22. Absorbance was read at 450 nm and recorded on a microplate reader (Tecan Infinite M200, Tecan Group, Männedorf, Switzerland).
Statistical analysis
All data are presented as mean ±SD or SEM. Statistical significance was determined by the Student t test. P < 0.05 was considered statistically significant (* = P < 0.05; ** = P < 0.01; *** = P < 0.001).
Disclosure of Interest
The authors report no conflict of interest.
Supplemental Material
Download TIFF Image (17.9 MB)Acknowledgments
We wish to thank Damiano Scopetti for the fruitful discussion, Silvano Pagnotta and Maria Luisa Alunni for technical support. The original studies (to G. S.) were supported in part by Associazione Umbra Contro il Cancro (AUCC), PRIN Project n. 20152CB22L_004, Fondazione Cassa di Risparmio di Perugia 2015.0329.021, Comitato Maria Grazia Frasconi. DP, SP, MC and DB are recipient of the AUCC fellowship program.
Supplementary material
Supplementary data for this article can be accessed here.
Additional information
Funding
References
- Coussens LM, Werb Z. Inflammation and cancer. Nature. 2002 Dec 19–26;420(6917):860–867. PubMed PMID: 12490959; PubMed Central PMCID: PMCPMC2803035.
- Palucka AK, Coussens LM. The basis of oncoimmunology. Cell. 2016 Mar 10;164(6):1233–1247. PubMed PMID: 26967289; PubMed Central PMCID: PMCPMC4788788.
- Mantovani A, Barajon I, Garlanda C. IL-1 and IL-1 regulatory pathways in cancer progression and therapy. Immunol Rev. 2018 Jan;281(1):57–61. PubMed PMID: 29247996.
- Ruhland MK, Coussens LM, Stewart SA. Senescence and cancer: an evolving inflammatory paradox. Biochim Biophys Acta. 2016 Jan;1865(1):14–22. PubMed PMID: 26453912; PubMed Central PMCID: PMCPMC4733607.
- Ara T, Declerck YA. Interleukin-6 in bone metastasis and cancer progression. Eur J Cancer. 2010 May;46(7):1223–1231. PubMed PMID: 20335016; PubMed Central PMCID: PMCPMC2917917.
- Kantono M, Guo B. Inflammasomes and cancer: the dynamic role of the inflammasome in tumor development. Front Immunol. 2017;8:1132. PubMed PMID: 28955343; PubMed Central PMCID: PMCPMC5600922.
- Qian BZ. Inflammation fires up cancer metastasis. Semin Cancer Biol. 2017 Dec;47:170–176. PubMed PMID: 28838845.
- Schmidt-Arras D, Rose-John S. IL-6 pathway in the liver: from physiopathology to therapy. J Hepatol. 2016 Jun;64(6):1403–1415. PubMed PMID: 26867490.
- Guo Y, Xu F, Lu T, et al. Interleukin-6 signaling pathway in targeted therapy for cancer. Cancer Treat Rev. 2012 Nov;38(7):904–910. PubMed PMID: 22651903.
- Xu J, Ye Y, Zhang H, et al. Diagnostic and prognostic value of serum interleukin-6 in colorectal cancer. Medicine (Baltimore). 2016 Jan;95(2):e2502. PubMed PMID: 26765465; PubMed Central PMCID: PMCPMC4718291.
- Lippitz BE, Harris RA. Cytokine patterns in cancer patients: A review of the correlation between interleukin 6 and prognosis. Oncoimmunology. 2016 May;5(5):e1093722. PubMed PMID: 27467926; PubMed Central PMCID: PMCPMC4910721.
- Higgins GM, Rogers JC. Effect of radium emanation on the histocyte in the liver of the white rat. Am J Pathol. 1932 May;8(3):355–365 4. PubMed PMID: 19970025; PubMed Central PMCID: PMCPMC2062684.
- Taub R. Liver regeneration: from myth to mechanism. Nat Rev Mol Cell Biol. 2004 Oct;5(10):836–847. PubMed PMID: 15459664.
- Brockmann L, Giannou AD, Gagliani N, et al. Regulation of TH17 cells and associated cytokines in wound healing, tissue regeneration, and carcinogenesis. Int J Mol Sci. 2017 May 11;18(5). PubMed PMID: 28492497; PubMed Central PMCID: PMCPMC5454945. DOI: 10.3390/ijms18051033
- Fausto N, Campbell JS, Riehle KJ. Liver regeneration. Hepatology. 2006 Feb;43(2 Suppl 1):S45–53. PubMed PMID: 16447274.
- Della Fazia MA, Servillo G. Foie gras and liver regeneration: a fat dilemma. Cell Stress. 2018;2:1–14.
- Fausto N. Liver regeneration. J Hepatol. 2000;32(1 Suppl): 19–31. PubMed PMID: 10728791.
- Gilgenkrantz H, Collin de l’Hortet A. New insights into liver regeneration. Clin Res Hepatol Gastroenterol. 2011 Oct;35(10):623–629. PubMed PMID: 21613004.
- Michalopoulos GK. Liver regeneration after partial hepatectomy: critical analysis of mechanistic dilemmas. Am J Pathol. 2010 Jan;176(1):2–13. PubMed PMID: 20019184; PubMed Central PMCID: PMCPMC2797862.
- Michalopoulos GK, DeFrances MC. Liver regeneration. Science. 1997 Apr 4;276(5309):60–66. PubMed PMID: 9082986.
- Riehle KJ, Dan YY, Campbell JS, et al. New concepts in liver regeneration. J Gastroenterol Hepatol. 2011 Jan;26(Suppl 1):203–212. PubMed PMID: 21199532; PubMed Central PMCID: PMCPMC3077908.
- Mangnall D, Bird NC, Majeed AW. The molecular physiology of liver regeneration following partial hepatectomy. Liver Int. 2003 Apr;23(2):124–138. PubMed PMID: 12654135.
- Iwai M, Cui TX, Kitamura H, et al. Increased secretion of tumour necrosis factor and interleukin 6 from isolated, perfused liver of rats after partial hepatectomy. Cytokine. 2001 Jan 7;13(1):60–64. PubMed PMID: 11145844.
- Akerman P, Cote P, Yang SQ, et al. Antibodies to tumor necrosis factor-alpha inhibit liver regeneration after partial hepatectomy. Am J Physiol. 1992 Oct;263(4 Pt 1):G579–85. PubMed PMID: 1415718.
- FitzGerald MJ, Webber EM, Donovan JR, et al. Rapid DNA binding by nuclear factor kappa B in hepatocytes at the start of liver regeneration. Cell Growth Differ. 1995 Apr;6(4):417–427. PubMed PMID: 7794809.
- Trautwein C, Rakemann T, Niehof M, et al. Acute-phase response factor, increased binding, and target gene transcription during liver regeneration. Gastroenterology. 1996 Jun;110(6):1854–1862. PubMed PMID: 8964411.
- Servillo G, Della Fazia MA, Sassone-Corsi P. Transcription factor CREM coordinates the timing of hepatocyte proliferation in the regenerating liver. Genes Dev. 1998 Dec 1;12(23):3639–3643. PubMed PMID: 9851970; PubMed Central PMCID: PMCPMC317249.
- Servillo G, Della Fazia MA, Sassone-Corsi P. Coupling cAMP signaling to transcription in the liver: pivotal role of CREB and CREM. Exp Cell Res. 2002 May 1;275(2):143–154. PubMed PMID: 11969286.
- Servillo G, Penna L, Foulkes NS, et al. Cyclic AMP signalling pathway and cellular proliferation: induction of CREM during liver regeneration. Oncogene. 1997 Apr 3;14(13):1601–1606. PubMed PMID: 9129151.
- Su AI, Guidotti LG, Pezacki JP, et al. Gene expression during the priming phase of liver regeneration after partial hepatectomy in mice. Proc Natl Acad Sci U S A. 2002 Aug 20;99(17):11181–11186. PubMed PMID: 12177410; PubMed Central PMCID: PMCPMC123230.
- Cressman DE, Greenbaum LE, DeAngelis RA, et al. Liver failure and defective hepatocyte regeneration in interleukin-6-deficient mice. Science. 1996 Nov 22;274(5291):1379–1383. PubMed PMID: 8910279.
- White P, Brestelli JE, Kaestner KH, et al. Identification of transcriptional networks during liver regeneration. J Biol Chem. 2005 Feb 4;280(5):3715–3722. PubMed PMID: 15546871.
- Bellet MM, Masri S, Astarita G, et al. Histone deacetylase SIRT1 controls proliferation, circadian rhythm, and lipid metabolism during liver regeneration in mice. J Biol Chem. 2016 Oct 28;291(44):23318–23329. PubMed PMID: 27634039; PubMed Central PMCID: PMCPMC5087747.
- Deng X, Li W, Chen N, et al. Exploring the priming mechanism of liver regeneration: proteins and protein complexes. Proteomics. 2009 Apr;9(8):2202–2216. PubMed PMID: 19322782.
- Liu HX, Fang Y, Hu Y, et al. PPARbeta regulates liver regeneration by modulating akt and E2f signaling. PLoS One. 2013;8(6):e65644. PubMed PMID: 23823620; PubMed Central PMCID: PMCPMC3688817.
- Xu C, Zhao W, Hao Y, et al. Comparative analysis of gene expression profiles of acute hepatic failure and that of liver regeneration in rat. Gene. 2013 Oct 10;528(2):59–66. PubMed PMID: 23916745.
- Amatya N, Garg AV, Gaffen SL. IL-17 signaling: the Yin and the Yang. Trends Immunol. 2017 May;38(5):310–322. PubMed PMID: 28254169; PubMed Central PMCID: PMCPMC5411326.
- Chen K, Kolls JK. Interluekin-17A (IL17A). Gene. 2017 May 30;614:8–14.
- Romani L. Immunity to fungal infections. Nat Rev Immunol. 2011 Apr;11(4):275–288. PubMed PMID: 21394104.
- Rao R, Graffeo CS, Gulati R, et al. Interleukin 17-producing gammadeltaT cells promote hepatic regeneration in mice. Gastroenterology. 2014 Aug;147(2):473–84 e2. PubMed PMID: 24801349; PubMed Central PMCID: PMCPMC4123443.
- Furuya S, Kono H, Hara M, et al. Interleukin-17A plays a pivotal role after partial hepatectomy in mice. J Surg Res. 2013 Oct;184(2):838–846. PubMed PMID: 23590864.
- Sabat R, Ouyang W, Wolk K. Therapeutic opportunities of the IL-22-IL-22R1 system. Nat Rev Drug Discov. 2014 Jan;13(1):21–38. PubMed PMID: 24378801.
- Albrecht JH, Poon RY, Ahonen CL, et al. Involvement of p21 and p27 in the regulation of CDK activity and cell cycle progression in the regenerating liver. Oncogene. 1998 Apr 23;16(16):2141–2150. PubMed PMID: 9572495.
- De Luca A, Pariano M, Cellini B, et al. The IL-17F/IL-17RC axis promotes respiratory allergy in the proximal airways. Cell Rep. 2017 Aug 15;20(7):1667–1680. PubMed PMID: 28813677.
- Studer P, Da Silva CG, Revuelta Cervantes JM, et al. Significant lethality following liver resection in A20 heterozygous knockout mice uncovers a key role for A20 in liver regeneration. Cell Death Differ. 2015 Dec;22(12):2068–2077. PubMed PMID: 25976305; PubMed Central PMCID: PMCPMC4816110.
- Brunt EM, Gouw AS, Hubscher SG, et al. Pathology of the liver sinusoids. Histopathology. 2014 Jun;64(7):907–920. PubMed PMID: 24393125.
- Bartoli D, Piobbico D, Bellet MM, et al. Impaired cell proliferation in regenerating liver of 3 beta-hydroxysterol Delta14-reductase (TM7SF2) knock-out mice. Cell Cycle. 2016 Aug 17;15(16):2164–2173. PubMed PMID: 27341299; PubMed Central PMCID: PMCPMC4993425.
- Bellet MM, Piobbico D, Bartoli D, et al. NEDD4 controls the expression of GUCD1, a protein upregulated in proliferating liver cells. Cell Cycle. 2014;13(12):1902–1911. PubMed PMID: 24743017; PubMed Central PMCID: PMCPMC4111753.
- Piccoli C, Scrima R, Ripoli M, et al. Transformation by retroviral vectors of bone marrow-derived mesenchymal cells induces mitochondria-dependent cAMP-sensitive reactive oxygen species production. Stem Cells. 2008 Nov;26(11):2843–2854. PubMed PMID: 18787213.