ABSTRACT
The treatment of oral mucosa defect such as autologous oral mucosa caused by resection of oral mucosa carcinoma is still not ideal in clinical practice. However, Tissue engineering gives us the possibility to solve this problem. As we all know, Human embryonic stem cells (hESCs) have the ability to give rise to various cell types. We can take advantage of the totipotency of human embryonic stem cells to acquire keratinocytes. Directing the epithelial differentiation of hESCs can provide seed cells for the construction of epithelium tissue by tissue engineering. But, how to get high purity keratinocytes by induced stem cells then Applied to tissue engineering mucosa is an important challenge. We described a novel method to directly induce hESCs to differentiate into keratinocytes. Retinoic acid, ascorbic acid, and bone morphogenetic protein induced hESCs to differentiate into cells that highly expressed cytokeratin (CK)14. Our findings suggest that the retinoic acid, ascorbic acid and bone morphogenetic proteins induced hESCs to form high purity keratinocyte cell populations. In addition, we found that the highly pure keratinocyte populations reconstructed artificial tissue resembling epithelial tissue when inoculated in vitro on a biological scaffold.
Introduction
As a barrier against exogenous substances, pathogens, Oral mucosa play an important role in our physical conditions, Shortage of clinical injury of mucosa transplantation has been bothering us for many years. A potential benefit of tissue engineering is the in vitro generation of tissues that mimic the form and function of their in vivo counterparts and can be used to replace damaged or diseased human oral mucosa tissues. Human embryonic stem cells (hESCs) have significant advantages over adult stem cells as an unlimited, pluripotent source of the multiple cell types needed to fabricate complex tissues [Citation1–Citation3], The direct differentiation of hESCs provides seed cells for engineering a variety of tissues.
The development of substrates that provide uniform growth environments, such as Matrigel [Citation4,Citation5] or gelatin [Citation6,Citation7], and feeder layers [Citation8] has facilitated the efficient, directed differentiation of hESCs into mature cell types that derive from all embryonic layers. The differentiation of hESCs can thus be directed toward derivation of ectodermal tissues including neural lineages [Citation9]. Recent reports have described the derivation of definitive ectoderm, including keratinocytes [Citation10] and corneal epithelial cells [Citation11,Citation12]. However, the cells derived in those studies demonstrated limited expansion potential, which highlights the need for differentiation techniques that more closely mimic the microenvironment found during normal development. That should allow derivation of increasingly pure populations of proliferative progenitor cells, which is essential to optimize the supply of hESC-derived cells for tissue engineering applications in the future.
Keratinocytes cannot reproduce indefinitely outside the body, Embryonic stem cells can reproduce indefinitely in vitro and play an important role in the generation of keratinocytes as well as in the in tissue engineering mucosa [Citation13].We developed a method to derive multiple cell lineages needed for construction of stratified epithelial tissues from an H9 hESC line using retinoic acid, (RA), bone morphogenetic protein 4 (BMP4), and ascorbic acid (AA). A Matrigel feeder layer significantly facilitated the induction of hESC differentiation into keratinocytes, and promoted the proliferation of epidermal keratinocytes (EKCs). We constructed common epithelial tissues using the keratinocyte lineage cells induced from hESCs.
Material and methods
The method for high-purity keratinocytes induction and epithelial tissue construction has been applied for a national patent (Patent Number: 20150501770.9) in China.
Human embryonic stem cells and culture medium
We obtained H9 human embryonic stem cells from WiCell Research Institute (Madison, WI, USA; Agreement no. 12-W0328). hESCs were cultured on a layer of on Matrigel-coated plates (BD Biosciences, Franklin Lake, NJ, USA) in the mTeSR™1 medium (Stemcell Technologies Inc., Vancouver, Canada). We mixed RA 1 μM, BMP4 25 ng/ml, and AA 0.3 mmol/l as the inducing reagents. After we culture the hESCs about 4 d and we got the logarithmic phase of growth, the H9 human embryonic stem cells were cultured in the induction reagent for 7 d and then transferred to keratinocyte serum-free medium (KSFM, Gibco, Gaithersburg, MD, USA) for 4 wk of growth. The morphology of the induced cells was observed, and epidermal keratinocytes (EKCs) expressing CK14 were identified and sorted by flow cytometry.
Flow cytometry
We obtained the cells which induced with RA, BMP4, and AA to compare with the cells without inductive agent. Paraformaldehyde (4%, 1 ml) fix cells for 30 minutes under the condition of 4°C, centrifugal cells at 1500 rpm for 5 min. Triton-100(0.1%) treated the cells at room temperature for 10 min, cells was incubated with anti-CK14 antibody (Abcam, Cambridge, MA, USA) 10 µl under the condition of 4°C for 30 minutes.
Scaffold for tissue engineering
The scaffold used for tissue engineering was a 10 mm x 10 mm x 1 mm fetal bovine acellular dermal matrix (FB-ADM) it was obtained from Yantai Zhenghai Biotech (Authorized No. 20,153,460,386; Shandong, China).
Reconstruction of epithelial tissue
FB-ADMs were immersed in phosphate buffered saline (PBS) with penicillin and streptomycin (100 μ/ml) for 5 minutes, immersed in Hams F-12 nutrient medium with Matrigel for 30 minutes, and then in FB-ADM in KSFM for 12 hours. Three methods were used to inoculate cells on the biological scaffold [Citation14]. EKCs were floated onto the surfaces of biological scaffold [Citation1]. EKCs were injected into the biological scaffold [Citation3]. EKCs were sandwiched between two slices of biological scaffold. Biological scaffolds with EKCs were cultured in KSFM at 37°C in a CO2 incubator (Rs Biotech, Ayrshire, UK) for 7 d. The culture medium was changed every 2 d, and the conglutination of the EKCs and the biological scaffold was monitored by hematoxylin–eosin staining. We choose the method with the greatest extent of conglutination for reconstruction of epithelial tissue.
Immunohistochemistry
Reconstructed epithelial tissue was fixed for antigen staining, immersed in 3% hydrogen peroxide solution for 5 minutes, and washed in 1×PBS buffer for 5 minutes. After blocking nonspecific staining with goat anti-mouse IgG (Abcam, Cambridge, MA, USA) 20 µl, the tissue was incubated with anti-CK14 antibody (Abcam) 20 µl in a humid box at 37°C or room temperature for 30 minutes in the dark. The tissues were processed for staining and observed by microscopy.
Immunofluorescence
Paraffin-embedded tissue specimens were sectioned at 5 µm, deparaffinized in xylene, rehydrated in ethyl alcohol, and mounted on slides. The tissue was blocked in goat serum 20 µl, incubated with anti-cytokeratin 15 and rabbit-anti-human p63 for 1 hour at 37°C, and then incubated with fluorescent-labelled secondary antibody. Following nuclear counterstaining with 4ʹ,6-diamidino-2-phenylindole (DAPI, blue fluorescence), sections were washed 3× in PBS, and the slides were cover slipped. Stained tissue was immediately observed by fluorescence microscopy.
Scanning electron microscopy
The Reconstructed tissue was first fixed with 2.5% glutaraldehyde in phosphate buffer (pH7.0) for more than 4 hours; washed tissue three times in the phosphate buffer; then postfixed with 1% OsO4 in phosphate buffer (pH7.0) for 1 hour and washed three times in the phosphate buffer. The specimen was first dehydrated by a graded series of ethanol (30%,50%, 70%, 80%, 90%, 95% and 100%) for about 15–20 minutes at each step, transferred to the mixture of alcohol and iso-amyl acetate (v: v = 1:1) for about 30 minutes, then transferred to pure iso-amyl acetate for about 1 hour. In the end, the specimen was dehydrated in Hitachi Model HCP-2 critical point dryer with liquid CO2. The dehydrated specimen was coated with gold-palladium and observed in Philips Model TM-1000 SEM. The ultrastructure of the links between the cells and the biological scaffold can been observed by scanning electron microscopy.
Results
The differentiation of human embryonic stem cells
RA and AA induced differentiation of human embryonic stem cells into keratinocytes
CK14 is usually expressed in basal cells of stratified squamous epithelia. hESCs cultured in the induction medium generated large populations of cells that expressed CK14+. Flow cytometry determined that RA, BMP4, and AA induced the development of cells that strongly expressed CK14 ()), however the cells that grew in hESC cultures without added RA, BMP4, and AA weakly expressed CK14 ()). Populations of cells with strong CK14 expression formed polygonal arrays of cells of uniform size and had the appearance of paving stones sample, much like keratinocytes ()). Cells with weak CK14 expression were fusiform in appearance ()).
The construction of tissue engineering
Sandwich method promoted adhesion of EKCs to biological scaffolds
Hematoxylin–eosin staining found that the sandwich method was the most likely, and therefore the most useful, method to promote linking of EKCs and the biological scaffolds (A3)). With the other two methods, only a small number of cells adhered to the biological scaffolds (A1, A2)).
Figure 3. Hematoxylin–eosin staining of EKCs and biological scaffolds shows the in vitro structural organization. In A1, the cell nucleus is not clearly visible and a paving-stone morphology is not apparent. In A2, the number of adherent cells is decrease, nuclei are not apparent, and a paving-stone morphology is absent. A3 shows that cells of uniform size have adhered to the biological scaffold in a polygonal array that has a paving-stone appearance. Panel B shows the biological scaffold without any adherent cells.
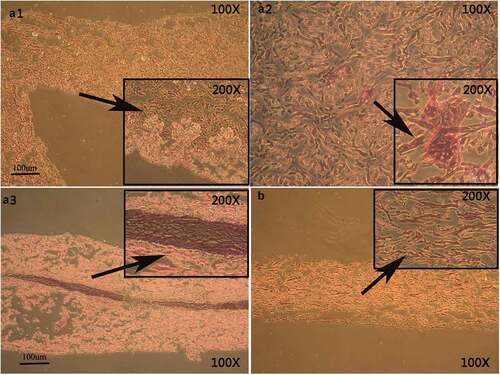
CK14+cells were arranged in the basal layer of reconstructed tissue
Immunohistochemical staining of the reconstructed found that CK14+cells were in close contact with the biological scaffold () much as in the basal cell layer of normal oral mucosal epithelium ().
P63 was expressed in cells of in vitro reconstructed tissue
P63+cells were scattered within each layer of normal epithelial tissue, and their distribution was different from that of CK14. In the in vitro reconstructed tissue, we found P63+cells scattered within each layer () and with a distribution pattern similar to that in normal oral mucosa epithelium ().
Ck15+cells occurred in the in vitro reconstructed tissue
CK15+cells are found dispersed within every layer of the normal epithelial tissue. Immunofluorescence staining revealed that CK15+cells were scattered within the in vitro reconstructed tissue (, and had a distribution pattern similar to that of normal oral mucosal epithelium ().
Desmosomes formed between cells of reconstructed tissue
In normal oral mucosa epithelial tissue, desmosomes are visible between cells, and half-desmosomes form between cells and the basal lamina. By scanning electron microscopy, we found that desmosomes appeared between EKCs, but no half-desmosome were visible ().
Figure 7. Scanning electron micrographs show intercellular junctions. In the reconstructed tissue, many desmosomes formed between cells (A, B), but no half-desmosomes formed between the cells and the basal layer (C). Desmosomes are shown between cells in normal oral mucosal epithelium (D, E), and half-desmosomes occur between cells and the basal lamina (F).
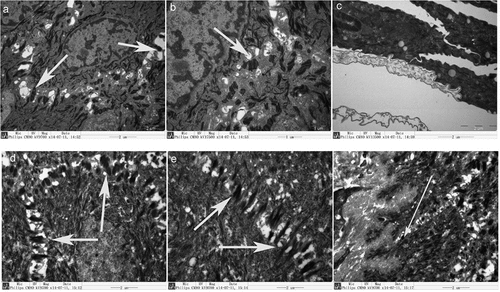
Discussion
The reconstruction of epithelial tissue requires relatively pure populations of keratinocytes, but this is challenging, and has been difficult to accomplish by inducing hESC differentiation. To take advantage of the expansion potential of hESCs, a cell culture method must be developed to efficiently generate lineage-restricted progenitors of high purity cells. hESCs can differentiate into keratinocytes [Citation15]. But the currently available methods are inefficient [Citation16]. In addition, the identification of efficient differentiation protocols to generate functional somatic cell lineages in hESCs is a major challenge.
RA signaling participates in many developmental processes during embryogenesis, including pattern formation and limb development [Citation17–Citation19]. RA has often been used to induce the development of neurons from hESCs [Citation20–Citation22]. Recently, BMP4 has been used to induce keratinocyte differentiation of murine ESCs and to induce apoptosis of neural precursors through the Smad pathway [Citation23]. Studies using murine ESCs have identified BMP-4 as an inducer of epidermal differentiation [Citation24,Citation25]. The role of BMP4 in human ectodermal development is currently unclear, as this factor has mainly been used to direct differentiation of hESC into nonectodermal lineages. RA has been shown to inhibit the terminal differentiation of keratinocytes in vitro and has a caudalizing effect, promoting ESC-derived neuroepithelia to become motor neurons [Citation10]. Given the ethical problems associated with in vivo human embryo research, hESCs offer an attractive in vitro model system to study the effects of RA and other chemicals on early human developmental processes. Metallo et al. [Citation10] reported that RA- and BMP-signaling synergistically direct epithelial differentiation of hESCs. They generated relatively pure keratinocyte cultures capable of terminal differentiation to form coherent epithelial sheets. As a free-radical scavenger, AA has the ability to promote mesenchymal proliferation, Fibroblast differentiation, collagen synthesis, and keratinocyte survival. Combining BMP4 and AA improves hESC differentiation [Citation16,Citation26]. In this study, we used RA, BMP4, and AA [Citation27] to induce early differentiation of hESCs and achieved a higher purity of keratinocytes.
In transplantation studies, hESCs were capable of differentiating within biological scaffolds to form a variety of cell types with the characteristics of normal tissues. However, the cells also formed teratomas [Citation28], which limits their use in transplantation [Citation29]. The biological scaffold material provides a good environment for adhesion, growth, migration, proliferation, and differentiation. To date, various biological scaffolds have been used in tissue engineering studies including a dermal matrix, bacterial cellulose, and collagen. We choose a decellularized fetal bovine dermal matrix, which lacks antigenicity. Adherence of EKCs to the biological scaffold was promoted most effectively with the sandwich method, and the cells were of uniform size and formed polygonal arrays that had a paving-stone appearance. The study results showed that fetal bovine decellularized dermal matrix can be used in the reconstruction of epithelial tissue in vitro.
The differentiation of hESC into keratinocytes is marked by expression of CK14, an intermediate filament present in the basal layer of stratified squamous epithelia [Citation30,Citation31]. The initial studies to generate keratinocytes from hESCs successfully produced CK14-expressing cells, but those cells had limited expansion potential [Citation2]. We induced hESCs in culture medium containing AA, RA, and BMP4, and obtained cells that strongly expressed CK14+ and had other characteristics of keratinocytes. The hESCs induced by RA, BMP4, and AA highly expressed CK14, but hESCs cultured in medium lacking the three inducing agents only expressed CK14 weakly. CK14+EKCs were of uniform size, had a polygonal morphology, and formed arrays with a paving-stone appearance. Those cells were thus similar to keratinocytes. Immunohistochemical analysis confirmed that CK14+cells occurred in the in vitro reconstructed tissue, and were in close contact with the biological scaffold (), similar to cells in the basal layer of normal oral mucosal epithelium.
CK15 is an epidermal stem-cell marker that appears in the early stages of epidermal stem-cell differentiation, and CK15 expression occurs earlier than other keratin expression marker proteins. CK15+cells are scattered within every layer of normal epithelial tissue. In this study, immunofluorescence staining revealed CK15+cells in vitro reconstructed tissue with a distribution pattern similar to that in normal oral mucosal ().
The P63 protein is a marker of the differentiation of normal epithelium and is highly expressed in the normal oral mucosal epithelial cells. We found P63+cells scattered in each layer of in vitro reconstructed tissue (), similar to its distribution in normal oral mucosal epithelium.
It is well known that normal oral mucosal epithelium includes desmosomes between cells and half-desmosomes between cells and the basement lamina. SEM confirmed () that the desmosomes were present between EKCs, but there were no half-desmosomes between the cells and biological scaffold, which needs an in-depth study.
Conclusion
We optimized a cell culture system using RA, AA, and BMPS to induce differentiation of hESCs into keratinocytes and obtain a high purity keratinocyte population. We inoculated biological scaffolds with EKCs, which resulted in in vitro reconstruction of artificial tissue that was like epithelial tissue. Such tissue-engineered skin might be used for the repair of clinical skin tissue defects in the future, and our findings add to what is known of epithelial tissue engineering. However, how to obtain more purity of differentiated cell populations is need the further research and the way to reconstruction of artificial tissue in vitro is diffcult to find. Nevertheless, we believe that artificial epithelial tissue will be widely put into clinic practice in the future.
Acknowledgments
We would like to thank Cao Tong (Department of Oral and Maxillofacial Surgery, Faculty of Dentistry, National University of Singapore), and Xiao Ran and Fu Xin (Research Center of Plastic Surgery, Plastic Surgery Hospital, Chinese Academy of Medical Sciences). This research was supported by the National Natural Science Foundation of China (grant No. 81170968).
Disclosure statement
No potential conflict of interest was reported by the authors.
Additional information
Funding
References
- Coraux C, Hilmi C, Rouleau M, et al. Reconstituted skin from murine embryonic stem cells. Curr Biol. 2003;13(10):849–853.
- Green H, Easley K, Iuchi S. Marker succession during the development of keratinocytes from cultured human embryonic stem cells. Proc Natl Acad Sci USA. 2003;100(26):15625–15630.
- Sugaya K, Vaidya M. Stem cell therapies for neurodegenerative diseases. Adv Exp Med Biol. 2018;1056:61–84.
- Kleinman HK, Martin GR. Matrigel: basement membrane matrix with biological activity. Semin Cancer Biol. 2005;15(5):378–386.
- Perny M, Ting CC, Kleinlogel S, et al. Generation of otic sensory neurons from mouse embryonic stem cells in 3D culture. Front Cell Neurosci. 2017;19(11):409.
- Chong EJ, Phan TT, Lim IJ, et al. Evaluation of electrospun PCL/gelatin nanofibrous scaffold for wound healing and layered dermal reconstitution. Acta Biomater. 2007;3(3):321–330.
- Wang C, Yue H, Feng Q, et al. Injectable nanoreinforced shape-memory hydrogel system for regenerating spinal cord tissue from traumatic injury. ACS Appl Mater Interfaces. 2018;10(35):29299–29307.
- Correia A, Anisimov SV, Jia-Yi L, et al. Growth Factors and feeder cells promote differentiation of human embryonic stem cells into dopaminergic neurons: a novel role for fibroblast growth factor-20. Front Neurosci. 2008;2(1):26–34.
- Trounson A. Human embryonic stem cells: mother of all cell and tissue types. Reprod Biomed Online. 2002;4(1):58–63.
- Metallo CM, Ji L, de Pablo JJ, et al. Retinoic acid and bone morphogenetic protein signaling synergize to efficiently direct epithelial differentiation of human embryonic stem cells. Stem Cells. 2008 Feb;26(2):372–380.
- Ahmad S, Stewart R, Yung S, et al. Differentiation of human embryonic stem cells into corneal epithelial like cells by in vitro replication of the corneal epithelial stem cell niche. Stem Cells. 2007;25(5):1145–1155.
- Hanson C, Hardarson T, Ellerström C, et al. Transplantation of human embryonic stem cells onto a partially wounded human cornea in vitro. Acta Ophthalmol. 2013;91(2):127–130.
- Movahednia MM, Kidwai FK, Jokhun DS, et al. Potential applications of keratinocytes derived from human embryonic stem cells. Biotechnol J. 2016;11(1):58–70.
- Thomson JA, Itskovitz-Eldor J, Shapiro SS, et al. Embryonic stem cell lines derived from human blastocysts. Science. 1998;282(5391):1145–1147.
- Metallo CM, Mohr JC, Detzel CJ, et al. Engineering the stem cell microenvironment. Biotechnol Prog. 2007;23(1):18–23.
- Guenou H, Nissan X, Larcher F, et al. Human embryonic stem-cell derivatives for full reconstruction of the pluristratified epidermis: a preclinical study. Lancet. 2009;374(9703):1745–1753.
- Kawakami Y, Raya A, Raya RM, et al. Retinoic acid signalling links left-right asymmetric patterning and bilaterally symmetric somitogenesis in the zebrafish embryo. Nature. 2005;435(7039):165–171.
- Thaller C, Eichele G. Identification and spatial distribution of retinoids in the developing chick limb bud. Nature. 1987;327(6123):625–628.
- Cunningham TJ, Zhao X, Sandell LL, et al. Antagonism between retinoic acid and fibroblast growth factor signaling during limb development. Cell Rep. 2013;3(5):1503–1511.
- Li XJ, Du ZW, Zarnowska ED, et al. Specification of motoneurons from human embryonic stem cells. Nat Biotechnol. 2005;23(2):215–221.
- Wichterle H, Lieberam I, Porter JA, et al. Directed differentiation of embryonic stem cells into motor neurons. Cell. 2002;110(3):385–397.
- Boisvert EM, Engle SJ, Hallowell SE, et al. Maturation of nociceptive neurons from human embryonic stem cells. Sci Rep. 2015;19(5):16821.
- Gambaro K, Aberdam E, Virolle T, et al. BMP-4 induces a smaddependent apoptotic cell death of mouse embryonic stem cell-derived neural precursors. Cell Death Differ. 2006;13(7):1075–1087.
- Xu RH, Chen X, Li DS, et al. BMP4 initiates human embryonic stem cell differentiation to trophoblast. Nat Biotechnol. 2002;20(12):1261–1264.
- Schlüter H, Kaur P. Bioengineered human skin from embryonic stem cells. Lancet. 2009;374(9703):1725–1726.
- Hewitt KJ, Shamis Y, Carlson MW, et al. Three-dimensional epithelial tissues generated from human embryonic stem cells. Tissue Eng Part A. 2009;15(11):3417–3426.
- Li H, Zhou H, Xin F, et al. Directed differentiation of human embryonic stem cells into keratinocyte progenitors in vitro: an attempt with promise of clinical use. In Vitro Cell Dev Biol Animal. 2016;52(8):885–893.
- Lin YT, Wang CK, Yang SC, et al. Elimination of undifferentiated human embryonic stem cells by cardiac glycosides. Sci Rep. 2017;7(1):5289.
- Lees JG, Lim SA, Croll T, et al. Transplantation of 3D scaffolds seeded with human embryonic stem cells: biological features of surrogate tissue and teratoma-forming potential. Regen Med. 2007;2(3):289–300.
- TroyTC TK. In vitro characteristics of early epidermal progenitors isolated from keratin14(K14)—deficient mice: insightsinto the role of keratin 17 in mousekeratinocytes. J Cell Physiol. 1999;180(3):409–421.
- Liu Y, Zhong L, Liu D, et al. Differential miRNA expression profiles in human keratinocytes in response to protein kinase C inhibitor. Mol Med Rep. 2017 Nov;16(5):6608–6619.