ABSTRACT
Non-small cell lung cancer (NSCLC) represents about 85% of all lung cancer cases. Lung cancer is the most frequent non-AIDS-defining malignancies in HIV-infected patients. The mechanism of the increased risk for lung cancer in HIV-1 patients is poorly understood. HIV-1 Nef protein has been suggested to be one of the key players in HIV-related lung disease. In here, we showed the involvement of Nef protein in cell modifications such as fibroblasts (IMR-90) and normal (BEAS-2B) or cancerous (A549) epithelial cells. We demonstrated that Nef protein reprograms initial stages of lung cancer (e.g. changes in the metabolism, improved cell survival and invasion, increase the angiogenesis factor VEGF). Additionally, we showed that Nef is provoking a global decrease of mature miRNA and a decrease of DICER1 and AGO expression in lung cells. MiRNAs play a crucial role in cell signaling and homeostasis, functioning as oncogenes or tumor suppressors, and their dysregulation can contribute to the tumorigenic process. These results showed that HIV-1 Nef protein is directly involved in preventing cell death and contributes to tumor progression.
Introduction
Lung cancer is the most common Non-AIDS-Defining Cancer (NADC) and the leading cause of cancer-related deaths among HIV-infected patients [Citation1,Citation2]. Non-small-cell lung carcinoma (NSCLC) is a type of epithelial lung cancer that represents 86% to 94% of cases in HIV-infected patients, with adenocarcinoma being the most common (30 to 52%) when compared to age-matched lung cancer control groups [Citation3]. It has been reported that HIV-infected patients are diagnosed with lung cancer a decade earlier than uninfected patients [Citation4]. Even though the mechanisms involved remain to be elucidated, clinical studies revealed that the proportion of deaths attributed to non-AIDS/non-hepatitis-related cancers significantly increased from 11% to 22% between 2000 and 2010 [Citation5].
The relation between low CD4 T-cells count, the duration of immunosuppression and the risk of developing lung cancer remains controversial. Some reported an increased incidence of lung cancer among patients with AIDS-related immunosuppression [Citation1,Citation6], while others showed that lung cancer in HIV-infected individuals is not associated with CD4 counts [Citation7–Citation9]. Further, genomic instability [Citation10] and inflammation [Citation11] have been hypothesized to increase the risk of lung cancer in HIV-infected patients. Hence, it became increasingly accepted that HIV-1 promotes the risk of noninfectious pulmonary diseases. Further, HIV proteins were detected in germinal centers, pulmonary interstitium and bronchoalveolar lavage fluid of patients with lymphocytic interstitial pneumonia [Citation12]. Several of these studies showed a potential involvement of HIV-1 Nef protein in these deregulations [Citation13,Citation14].
The HIV-1 Nef protein is a small myristylated protein that plays a critical role in AIDS progression [Citation15]. Nef affects HIV-infected and uninfected pulmonary vascular cells [Citation16] and is associated with significant pulmonary vascular remodeling and lung lesions in human, macaques and transgenic mice [Citation16–Citation18]. It has been shown to be involved in several oncogenic pathways [Citation19,Citation20]. It synergistically promotes vIL-6-induced angiogenesis and tumorigenesis [Citation19] and supports the neoplastic transformation of immortalized neural cells [Citation21]. Nef blocks apoptosis, via molecules such as PI3K and PAK [Citation22], playing an important role in apoptotic inhibition and stimulation of cell survival during acute HIV-1 infection, probably to avoid premature host cell death and permit virus replication and production [Citation23]. Uptake of Nef protein by podocytes and pulmonary arterial endothelial cells in HIV-infected patients with nephrosis and pulmonary hypertension is a strong indicator of the role of Nef as a regulator of cell survival and proliferation [Citation24].
In here, we showed contribution of HIV-1 Nef protein in changing the microenvironment of stromal and epithelial lung cells.
Results
HIV-1 Nef changes the morphology of lung cells, as well as their proliferation and survival to starvation
We established two cell lines stably transfected with Nef-expressing plasmid. BEAS-2B, a normal human bronchial epithelium, and A549, a human NSCLC cell line. We wanted to assess the effect of Nef in cancerous and non-cancerous cell lines and with different modes of introducing Nef in the cells to ensure the robustness of our results. As shown in ), a phenotypic change emerged in Nef-expressing BEAS-2B and/or A549 cells. We observed multilayered areas with overlapping of the cell nuclei and elongated cells forming crisscross patterns.
Figure 1. HIV-1 Nef changes the morphology of lung cells, as well as their proliferation and survival to starvation. a. Multilayered areas with overlapping of the cell nuclei and elongated cells forming crisscross patterns were observed in Nef-stably transfected BEAS-2B and A549 but not in the parental cell line. b. Measurement of Cell growth using A549 stable cell lines expressing control or HIV-1 Nef, and cell viability using BEAS-2B (c, d) as obtained using Guava ViaCount assay. The absolute number of viable cells compared to nonviable nucleated cells was quantitated by cell size and staining properties using flow cytometry. In C, the cell viability is measured in presence/absence of FBS serum and HIV-1 Nef recombinant protein. BEAS-2B cells are stably transfected with HIV-1 Nef plasmid (panel D), and their viability is measured in the absence of serum. Cell starvation was carried out for 48 hours. Flow cytometry assay (Annexin V/Propidium iodide double staining) used to measure the percentage of necrosis or apoptosis in BEAS-2B cells cultured in the presence and absence of serum and then treated with Nef protein as marked and/or in BEAS-2B cells stably transfected with Nef and treated with 100 mM of cisplatin (e., f.). The percentage of necrosis and apoptosis is presented in the table. Same assay was used to determine cell cycle status in these cells (f.).
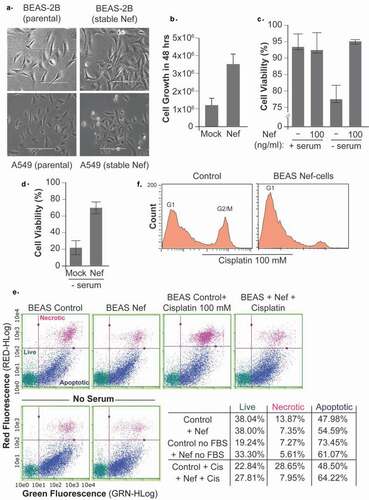
Next, we measured the cell growth and viability by flow cytometry. As shown in ), the expression of the HIV-1 Nef protein in A549 stable cell lines promoted cell proliferation.
It has been shown that serum deprivation induces apoptotic cell death through the elevation of mitochondrial changes and ROS production. Serum starvation is commonly used to mimic a nutrient deprivation state. Further, it is known that tumors have regions with poor blood supply, severe hypoxia, low pH and nutrient starvation. It appears that under stressful conditions the cancer stem cells show the capability to self-renew into progenitor cells. The ability of tumors to resist to this stress is a hallmark of cancer cells. Therefore, we examined the impact of Nef on cells subjected to serum starvation. After 48 hours of serum-starvation, the BEAS-2B cells could be rescued by HIV-1 Nef the addition of Nef recombinant protein ()) or in BEAS cells stably expressing Nef ()).
The serum-starvation is triggering the apoptosis in BEAS-2B cells, but barely any necrosis ()). As shown in the table summarizing the results of ) and obtain by flow cytometry assay, HIV-1 Nef rescued the cells from apoptotic cell death. The treatment of BEAS-2B cells with cisplatin induced apoptosis and necrosis, and Nef also rescued the cells from both modes of cell death.
Furthermore, it was interesting to note that the cisplatin caused cell cycle arrest in G2 phase in addition to cell death in the parental cells, while no G2 arrest was observed in Nef-stably transfected cells ()).
HIV-1 Nef expression disrupts invasion, anchorage-independent growth and attachment
We performed a clonogenic assay using A549 cells (stably transfected with Nef or empty vector as a control) to further assess the effect of Nef expression on cell proliferation. After culturing for 21 days, the colonies are stained with crystal violet, and we observed a substantial increase in the number and size of colonies in Nef-transfected cells compared to the control ()). Similar results were obtained with BEAS-2B stably transfected with Nef (Supp. 1-A).
Figure 2. HIV-1 Nef expression disturbs invasion, anchorage-independent growth and attachment. a. A549 cells (parental or stably transfected with Nef expression plasmid) were cultured for 21 days for the clonogenic assay. Crystal violet stained colonies showed an increase in the number and size of colonies in Nef-transfected cells compared to the control. b. Cell adhesion changes were quantified in the presence of warm 2.5% trypsin in stable cell lines expressing a control plasmid as well as an HIV-1 Nef plasmid. c. BEAS-2B stable cell lines were cultured in a three-dimensional Geltrex system and observed by phase-contrast microscopy after 24 hours. d. BEAS-2B stable cell lines were seeded at 90% confluence, and the wound line was generated using a sterile 10 μl-pipette tip. Images of resulting culture were obtained at 0 and 8 hours using a light microscopy with a digital camera.
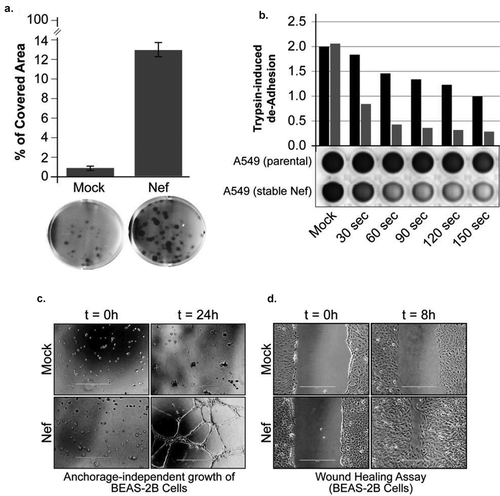
The loss of cell adhesion capability allows malignant tumor cells to detach from the primary tumor mass and facilitate the invasion of the surrounding stroma. Trypsin de-adhesion represents a simple method for examining the biophysical properties of adherent cells. In this assay, upon incubation with warmed trypsin, cells rounding up driven by the rapid severing of cell-matrix adhesions. It appeared that A549 cells expressing Nef present a weaker adhesion during trypsinization ()). Similar results were obtained with BEAS-2B stably transfected with Nef (Supp. 1-B).
The ability to acquire anchorage-independent cell growth, which is the faculty of forming colonies in a semi-solid media, is connected in cancer biology with tumor cell aggressiveness in vivo such as metastatic potential and tumorigenic. We demonstrated that Nef-expressing cells exhibit anchorage-independent growth in a 3-dimensional culture environment when allowed to grow on Geltrex matrix ()). Geltrex LDEV is a reduced growth factor basement matrix that allows anchorage-free growth of cells. Compared with the control BEAS-2B cells, the BEAS-2B Nef cells formed a higher number of invasive colonies, which were enlarged compared to the control and aberrantly shaped. BEAS-2B Nef cells colonized more successfully the surrounding membrane matrix ()).
Using the wound-healing assay, we assessed the effect of HIV-1 Nef expression on cell migration. 8 hours after wound induction, Nef-expressing BEAS-2B cells exhibit almost complete closure of the wound ()). The wound-healing assay mimics to some extents the migration of cells in vivo, but during the course of the assay, the cells will also proliferate. The population doubling time of A549 and BEAS-2B is 22 hours. Our experiments being of 8 hours maximum, we can attribute most of the cells movements to migration (See also Supp. 1-C and D).
Genes associated with lung cancer aggressivity are dysregulated by HIV-1 Nef
VEGF-A is a key facilitator of angiogenesis in cancer. Hence, we determined the expression level of VEGF-A in Nef-treated cells. IMR90 fibroblast and BEAS bronchial epithelial cells were treated with increasing concentration of recombinant Nef protein for 48 hours after which they were subjected to Western blot analysis. As shown in , an increased expression of VEGF-A was observed in all cells confirming the ability of Nef protein to promote cell proliferation and tumor progression.
Figure 3. Genes associated with lung cancer aggressivity are dysregulated by HIV-1 Nef . Expression levels of VEGF-A (panels a and b), p53 (panels b and c), NFAT-1 and MMP-9 (panels d and e) proteins were examined by Western blot assay in IMR90 and BEAS cells treated with an increasing amount of Nef protein as indicated or in A549 cells stably transfected with Nef compared to Control untreated or the parental cells, respectively. f. Western blot assay using parental or stably transfected A549 and BEAS-2B cells were transfected with siRNAs control, MMP-9 and NFAT1 for 72 hours. The expression of MMP-9, and GAPDH proteins are shown. The experiments were repeated 3 times. GAPDH was used as a control of equal protein loading. Similarly, the expression level of VEGF-A mRNA was examined in IMR90 and BEAS (panel A) using qPCR as indicated.
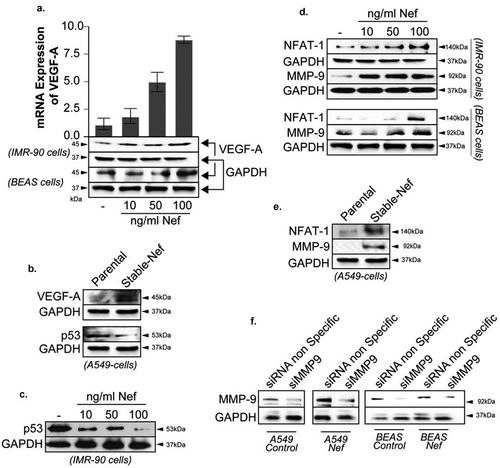
Similarly, the addition of Nef protein led to an increase of VEGF-A mRNA expression as obtained by qPCR (), histogram)
Inactivation of the p53 tumor suppressor is a frequent event in tumorigenesis. Using Western blot analysis, we found that addition of Nef protein to A549 and IMR-90 cells caused a significant decrease of p53 protein expression (, panels B and C).
The expression of HIV-1 Nef has been shown to be associated with higher levels of MMP-9 in PBMC and macrophages, as well as NFAT1 in CD4 T-cells and U937. Further, non-small cell lung cancer (NSCLC) has also been shown to be associated with an increased expression of the nuclear factor of T-cells (NFAT-1) and the matrix metalloproteinases (MMP-9) proteins [Citation25,Citation26]. Note that NFAT1 protein is known to regulate the MMP-9 promoter positively [Citation27]. Therefore, we investigated expression status of both proteins in Nef-treated/transfected A549, BEAS-2B, and IMR-90 cells. Using Western blot analysis, we showed that addition of Nef increased expression levels of NFAT-1 and MMP9 proteins in the three cell lines used (). (Note that GAPDH used in panels A and D and in Figure 4A (BEAS cells panel) are the same, and the ones used in panels B and E are also the same, the western blot membranes have been stripped and reprobed).
Additionally, the cell migration in the wound healing assay was reduced after the cells were treated with MMP-9 and NFAT1 siRNAs. These siRNAs knock-downs decrease the influence of Nef on the cell migration (), and Supp. 1-B and C).
HIV-1 Nef affects mitochondrial dynamics and autophagy
Mitochondrial dynamics and autophagy are two mechanisms tightly regulated during cancer and strongly linked to cell proliferation, survival, and angiogenesis. Therefore, we sought to examine whether Nef affects the expression of the LC3-B protein (microtubule-associated protein 1 Light Chain 3), a marker of the autophagy process. Epithelial (A549, BEAS-2B) and fibroblasts (IMR-90) lung cells were treated with recombinant Nef protein for 24 hours then subjected to Western blot analysis using anti-LC3 antibodies. As shown in ), a conversion of LC3-I to LC3-II was observed confirming autophagic activity and involvement of Nef in lung angiogenesis and development of tumors.
Figure 4. HIV-1 Nef affects mitochondrial dynamics and autophagy. a. The expression level of LC3-BI protein was examined by Western blot assay in IMR-90, A549 and BEAS-2B cells treated with an increasing amount of Nef protein or by flow cytometry using A549 stably transfected with Nef and then with GFP-LC3 expression plasmid as indicated. Anti-GAPDH antibody was used as a control for equal proteins loading. b. The number of fluorescent puncta in these cells was also measured by flow cytometry. c, d. Increased hyperpolarization of mitochondrial membranes potential of IMR90 cells treated with Nef as measured using JC-1 assay staining. The CCCP-treated sample was used as a positive control. Pairwise comparisons between means of different groups were performed using a Student t-test. e. Elongated and mitochondrial donut shapes as obtained in BEAS-2B cells transfected with Mito-dsRed expression plasmid and treated with 100 ng/ml of recombinant Nef protein for 24 hours compared to the Mock untreated.
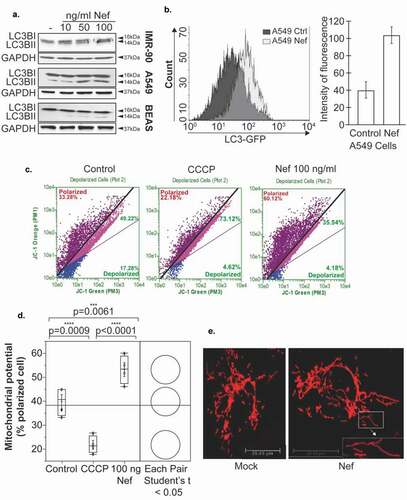
These results were confirmed using flow cytometry assay. Nef-stably transfected A549 cells were transfected with GFP-LC3 expression plasmid and then subjected to flow cytometry quantification. As shown in ), the expression level of LC3 increased in the presence of Nef compared to the parental cells.
IMR90 cells were treated with Nef protein for 24 hours and then subjected to JC-1 assay staining to determine the mitochondrial membrane potential. As shown in , Nef induces the hyperpolarization of mitochondrial membranes when compared to the controls (untreated or CCCP-treated). The CCCP-treated sample was used as a positive control. Similar results were obtained when using BEAS-2B cells stably expressing Nef (Supp. 2).
Furthermore, mitochondrial morphology shapes the cellular response to macroautophagy. Once autophagy is activated, mitochondria elongate in vitro and in vivo. BEAS-2B cells were transfected with Mito-dsRed expression plasmid which encodes a fusion of Discosoma sp. red fluorescent protein (DsRed2) and a mitochondrial targeting sequence of human cytochrome c oxidase subunit VIII, marking in red the mitochondria ()). Interestingly, the mitochondria appear more elongated than the mock; the mitochondria appear hyperfused.
HIV-1 Nef provokes a massive depletion of micro-RNAs in lung epithelial cells
Deregulation of miRNAs occurs widely in carcinogenesis, and altered miRNA expression plays important roles in cancer progression by directly regulating cell proliferation, angiogenesis, and metastasis among other pathways [Citation28]. We chose to determine the expression of 14 miRNAs known for their role in Non-Small-Cell Lung Cancer. Using RNA prepared from BEAS cells (parental and stably transfected with Nef), we found that expression levels of all the miRNAs examined diminished in Nef-transfected cells () and Supp. 3). Our results corroborate with the literature where it has been shown that altered miRNAs expression is a common characteristic of numerous cancers, including NSCLC [Citation29].
Figure 5. HIV-1 Nef provokes a massive depletion of micro-RNAs in lung epithelial cells. a. A major decrease in expression levels of 14 miRNA involved in lung cancer measured in BEAS-2B cells stably transfected with Nef or in the parental cells using qPCR assay. b, c. Expression levels of Dicer and Ago-2 proteins were examined by Western blot assay in BEAS-2B cells transfected with Nef expression plasmid or with pNL4-3 strain of HIV-1 lacking the Env gene as indicated. Experiments were repeated 3 times. GAPDH was used as a control of equal protein loading.
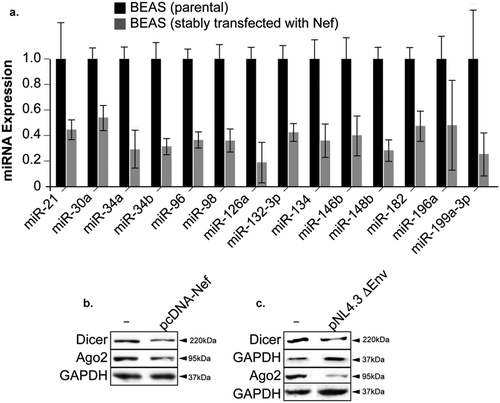
It has been shown that the expression of some components of the miRNA biogenesis machinery is altered by the carcinogenesis process. Downregulation of the Dicer1, a key protein that processes pre-miRNA has been reported in lung cancer, which predicts a poor clinical outcome. Similarly, Ago2 expression, which provides endonuclease activity to RNA-induced silencing complexes (RISC), was lower in human lung adenocarcinomas than in the non-cancerous tissues. These data prompted us to determine their expression level in Nef-transfected cells. BEAS-2B cells transfected with HIV-1 Nef expression plasmid showed lower protein expression of DICER1 and Ago2 ()).
Next, BEAS-2B cells were transfected with 100 ng of pNL4.3Δ Env (kindly obtained from NIH). This plasmid contains the full genome of HIV-1 except the envelope gene replaced by GFP coding sequence. As seen in ) with Nef, expression levels of Dicer and Ago2 decreased in the presence of pNL4-3ΔEnv ()) confirming our results regarding Nef functions.
Discussion
In this study, we described important changes in lung cells in the presence of HIV-1 Nef. The cells acquired several properties that will be alarmingly beneficial for tumorigenic and hyperplastic cells when they are present in the lung tissues. First, we observed the ability of the Nef-expressing cells to proliferate faster with less contact inhibition and less attachment to the matrix ( and ). Also, some proteins important in tissue remodelings like VEGF, NFAT1 or MMP-9 are overexpressed in the presence of HIV-1 Nef (). Further, we demonstrated that Nef can induce autophagy ().
Our results are in agreement with previously published data presenting the ability of Nef to enhance the expression of VEGF in fibroblasts [Citation19].The vascular endothelial growth factor A (VEGF) is the main mediator of angiogenesis. Nef was described to work in synergy with vIL-6 to promote angiogenesis and tumorigenesis on chicken chorioallantoic membranes by activating the AKT pathway. In tumors, stromal fibroblasts, as well as cancer cells, are an important supplier of VEGF promoting angiogenesis [Citation30]. However, the exact molecular mechanism leading HIV-1 Nef to induce VEGF expression was unclear. Note that MMP-9 can induce the release of tumor VEGF and VEGF and MMP-9 are important for the occurrence and development of non-small cell lung cancer (NSCLC). In this regard, we found that Nef is inducing expression of the MMP-9 protein ().
Further, a recent study showed that NFAT1 plays an important role in origination, invasion, and metastasis of Non-Small Lung Cancer cells, which corroborates with our data presented in . The regulation of NFAT is a conserved property of different Nef proteins in different HIV-1 Nef alleles. All tested HIV-1 Nef alleles potently induced the MAPK cascade during NFAT activation. Furthermore, HIV-1 Nef can influence the activation of the Ca2+/calcineurin signaling pathway which is significant for its pathogenic effect in AIDS.
Additionally, previous studies showed that binding of Nef to p53 reduces intracellular p53 concentrations and activity [Citation31]. Interestingly, addition of Nef to lung cells (A549 and IMR-90) led to p53 reduction (). Dysfunction in the tumor microenvironment promotes tumor establishment and progression, and enhanced autophagy in p53-deficient cells ameliorates their resistance to hypoxia and culture in nutrient-free conditions; it is possible that the loss of p53 augments the fitness of cancer cells to adverse metabolic conditions (as we showed in ).
We also presented the effects of HIV-1 Nef on cellular bioenergetics ( and S2). HIV-1-Nef has previously been described to induce autophagy in an IRGM-dependent pathway [Citation32]. During cancers, autophagy has a context-dependent multifaceted role [Citation33]. Autophagy has a function in modulating the microenvironment also and facilitates the establishment of pre-metastatic niches [Citation34]. Briefly, after the translation, pro-LC3 is proteolytically cleaved by an Atg4 protease, ensuing in the formation of LC3-I form. Upon autophagic signal, LC3-I is conjugated to a phosphatidylethanolamine (PE) moiety for the generation of LC3-II form which will be inserted into the membranes of autophagosomes. The conversion of LC3-I to LC3-II via (PE) conjugation is indicative of the autophagic activity (as shown in )). It has been described that the mitochondrial elongation during autophagy (as displayed in )), through a process of fusion, allowing mitochondria to resist autophagic degradation and prevent cell death [Citation35]. Furthermore, sustained mitochondrial elongation is linked to increased ROS levels [Citation36]. HIV-1 Nef can induce the release of superoxide anions in human phagocytic cells [Citation37].
In addition to increased autophagy and decreased p53 levels, the mitochondrial hyperpolarization induced by HIV-1 may affect the survival of the cells. Mitochondrial hyperpolarization is correlated with resistance to apoptosis, partially because the inoperative hyperpolarized mitochondria cannot induce apoptosis. The majority of human cancers are characterized by hyperpolarized mitochondria, and this metabolic conversion is the foundation of the Warburg effect in cancer [Citation38].
Our findings are novel and suggest that Nef can down-regulate massively microRNAs in lung cells ( and S3). A previous report described that Nef directly binds to Ago2, a component of RISC. This interaction could destabilize the miRNA-mRNA-Ago2 complex, inhibit the Dicer protein and the slicing activity of Ago2 [Citation39]. Suppression of microRNA-silencing pathway by HIV-1 during virus replication is not without precedent [Citation40]. These results are not without a precedent, it has been shown that the viral protein HIV-1 Tat has the possibility to interact with the Dicer protein physically and to inhibit its activity [Citation41]. Further, alteration of the Dicer protein is not restricted to HIV-1. For instance, Vaccinia Virus (VACV) infection triggers a significant decrease in Dicer protein expression and a subsequent deficit in pre-miRNA processing [Citation42].
Thus, we concluded that HIV-1 seems not only to modulate cellular miRNA profiles but also to interfere with the overall biogenesis and global expression of miRNAs suggesting potential role of these miRNAs in pathogenesis and disease progression. In cancer, altered miRNA expression profiles suggest an essential role of miRNA and DICER1 in tumor development and prognosis. A reduced expression of DICER and Ago2 in cancers is associated with poor prognosis. MiRNAs can function as tumor suppressors by targeting mRNA transcripts involved in proliferation, differentiation, and apoptosis [Citation43]. Recent work has shown a global decrease of mature miRNA expression in human cancers, and that abrogation of global miRNA processing promotes tumorigenesis [Citation44].
In summary, the mechanisms presented in this study (cell proliferation, autophagy, mitochondrial dynamics) are deeply interconnected, in healthy cells as well as in cancerous cells and can lead to a better understanding of tumor progression in HIV-1 patients. We examined them together to better decipher the pathways disrupted by HIV-1 infection.
Experimental procedures
Cell culture and transfection assay
IMR-90 fibroblasts, BEAS-2B and A549 epithelial cells were maintained at 37°C in Dulbecco’s modified Eagle’s medium (DMEM) (Cellgro, Manassas-VA, USA) (with high glucose for IMR-90) with 10% fetal bovine serum (FBS) IMR-90 and A549 and 2% for BEAS-2B, 100 units/mL of penicillin, 100 μg/mL of streptomycin. A549 cells were transfected with the empty vector pcDNA3.1 or pcDNA3.1-SF2Nef plasmid using transfection reagent (Invitrogen, Carlsbad-CA, USA) as recommended by the manufacturer. IMR-90 cells were kindly provided by Dr. Jean-Pierre Issa (Temple University, Philadelphia).
For 3D culture, plates were coated with Geltrex LDEV-FreeBasement Membrane Matrix, according to the manufactures instructions Gibco® (Thermo Fisher Scientific, Inc.).
Stable cell lines establishment
BEAS-2B and A549 cells were transfected with Lipofectamine 3000 (Invitrogen) with pSF2-Nef (NIH Aids Reagent). Stable cell lines are established by treatment of the cells with G418 1000 µg/ml (Santa Cruz Biotech., Dallas-Tx, USA).
Nef recombinant protein and plasmid
Recombinant full-length HIV-1 Nef protein (clade B), and pcDNA3.1SF2Nef plasmid were obtained from the AIDS Reagent Program, Division of AIDS, NIH (Cat# 11478 and 11431, respectively).
Cell viability measurement
Cell viability was determined utilizing a Guava EasyCyte 5HT® (Merck Millipore). Guava assay distinguishes between viable and non-viable cells based on the differential permeability of DNA-binding dyes in the Guava ViaCount Reagent (Merck Millipore). Viacount™ Software was used for data acquisition and analysis by enabling the EasyFit™ feature. The EasyFit performs analysis in three dimensions and allows better discrimination of live cells, dead cells and debris when they overlap with each other. Cells were diluted 1/20 in Viacount Reagent and incubated for 5 minutes before analyzing the sample. Experiments were performed in triplicate, and the data represented the mean ± standard deviation (s.d.) for each sample.
Wound healing assay
Cells were seeded on 6-cm dishes at 1 x 106 cells/ml, and cultured in DMEM supplemented with 2% FBS until 90% confluence. The wound line was generated by sculpturing cell culture using a sterile 10 μl-pipette tip. Images of resulting culture were obtained at 0 and 8 hours using a light microscopy with a digital camera.
Western blot analysis and antibodies
Cells (2 × 105) were treated with 10 to 100 ng/ml of Nef protein for the time indicated or transfected by the plasmid pcDNA3.1-SF2.Nef and Lipofectamine 3000 (Invitrogen). Cells were then harvested and re-suspended in RIPA lysis buffer as described by the manufacturer (Fermentas-MD, USA). Western blotting (30 μg/sample) was performed using anti- GAPDH, -p53, -VEGF-A, -NFAT1, -and MMP-9 (purchased from Santa Cruz and Cell Signaling).
RNA isolation and quantitative PCR
Total RNA was isolated using an RNeasy kit extraction (Qiagen Inc., Hilden-Germany) following the manufacturer’s instructions. RNA yields were measured using NanoDrop 2000 (Thermo Scientific). For gene expression, the first-strand cDNA was synthesized from total RNA (500 ng) by reverse transcription using Super Script® VILO™ (Invitrogen), according to the manufacturer’s instructions. Real-time PCR was performed using the following primers: p53 forward 5′-gcgagcactgcccaacaaca and (reverse) 5′-ggatctgaagggtgaaatattct; VEGF-A (forward) 5′-actggaccctggctt tactg and (reverse) 5′-tcacttcatgggacttctgc; β-actin (forward) 5′-tgtacgccaacacagtgctg and (reverse) 5′-gctggaaggtggacagcga. β-actin was used as a standard internal control of mRNA expression. miRNA cDNA synthesis was performed using Universal cDNA Synthesis Kit (Exiqon, Denmark). miRNA-specific microRNA LNA™ PCR primer sets were purchased from Exiqon. U6snRNA was used as an internal standard of mRNA expression. Reactions were carried out with Power SYBR Green PCR Master Mix (Applied Biosystems, Warrington, UK) using the StepOne PlusTM Real-Time PCR System (Applied Biosystems, Foster City-CA, USA). The mixture was denatured at 95°C for 10 minutes, followed by 40 cycles at 95°C for 15 seconds and 58°C for 40 seconds. Melting curve analyses were performed to verify the amplification specificity. Relative quantification of gene expression was performed according to the ΔΔ-CT method using StepOne Software 2.3.
Measurement of the mitochondrial membrane potential by JC-1 assay staining
The membrane-permeant JC-1 dye is widely used to monitor mitochondrial health. JC-1 dye exhibits potential-dependent accumulation in mitochondria, indicated by a fluorescence emission shift from green (~529 nm) to red (~590 nm). Consequently, mitochondrial depolarization is indicated by a decrease in the red/green fluorescence intensity ratio. The potential-sensitive color shift is due to the concentration-dependent formation of red fluorescent J-aggregates. Approximately 5 × 105 cells are suspended in 1 ml DMEM medium. For the control tube, 1 μl of 50 mM CCCP, the mitochondrial uncoupler carbonyl cyanide 3-chlorophenylhydrazone (Sigma C2759), is incubated with the cells at 37°C for 5 minutes. All cells were incubated with 2 μM final concentration of JC-1 and incubated at 37°C, 5% CO2, for 30 minutes. Cells were washed once with DMEM and resuspended in 500 μl DMEM. The analysis was performed immediately on a Guava 5HT flow cytometer. The samples are gated on the cells, excluding debris. The CCCP-treated sample is used as a positive control.
Trypsin-induced de-adhesion assay
Cells were first grown to 90% confluence in 12-well plates, then exposed to 2.5% trypsin/0.25 mM EDTA from 30 to 150 seconds. Detached cells were removed with PBS. Remaining adherent cells were fixed with 100% methanol, stained with crystal violet, and quantified at 590 nm absorbance.
Clonogenic assay
100 cells were seeded in 9.5 cm2 dishes in 500 μl of the medium. After 14 days, the resulting colonies were fixed and stained using 0.5% crystal violet in 25% methanol and counted.
Flow cytometry
Cells were cultured in 12-well plates at a density of 75,000 cells/well. For flow cytometric analysis, the culture medium was removed, and cells were incubated in Trypsin-EDTA 0.25% (Gibco) for 5 minutes. Trypsin was inactivated by addition of DMEM with 10%FBS. Flow cytometric analysis was carried out with a Guava, allowing simultaneous excitation and detection of green (488 nm) fluorescent protein. At least 4,000 events were collected for each well. For each sample, cell number and mean fluorescence intensity were reported.
Flow cytometric determination of apoptosis by annexin V/propidium iodide double staining. The FITC-Annexin V Apoptosis Detection Kit (eBioscience ™, ThermoFisher Scientific) was used according to the manufacturer´s protocol. In brief, cells were harvested by scraping, washed twice with cold phosphate buffered saline (PBS) and resuspended in binding buffer at a final density of 10 6 cells/ml. FITC-annexin (5 μl) were added to 200 μL of the cell suspension containing 10 5 cells. The cell suspension was mixed by gentle vortexing and then incubated for 15 min at room temperature in the dark and after another washing, PI was added (10 μl). Cells were analyzed by flow cytometry using Guava Incyte (Millipore).
Mitochondrial shape
BEAS-2B cells stably transfected with Mito-dsRed that encodes a fusion of Discosoma sp. red fluorescent protein and the mitochondrial targeting sequence from subunit VIII of human cytochrome c oxidase. Live cell imaging was performed using fluorescent-based live cell imaging system (Leica DMI4000) with excitation/emission 488/583 nm keeping cells at 37°C with 5% CO2. Cells have been treated for 24 hours with 100 ng/ml of recombinant Nef protein.
siRNA transfection. siRNA duplexes were purchased from Dharmacon (Lafayette, CO). siGENOME non-targeting Control-siRNA were designed by the manufacturer to contain at least four mismatches to any human, rat or mouse genes. For siRNA transfection in 6-well plates, 100 pmoles of siGENOME non-targeting siRNA Pool #1 (D-001206–13), Human Smartpool NFATC2 (4773) or MMP9 (4318) were transfected in the cells with Lipofectamine 3000 (Invitrogen, ThermoFisher Scientific), following the manufacturer’s protocol.
Supplemental Material
Download PDF (790.4 KB)Disclosure statement
No potential conflict of interest was reported by the authors.
Supplementary material
Supplemental data for this article can be accessed here.
Correction Statement
This article has been republished with minor changes. These changes do not impact the academic content of the article.
Additional information
Funding
References
- Marcus JL, Chao C, Leyden WA, et al. Survival among HIV-infected and HIV-uninfected individuals with common non-AIDS-defining cancers. Cancer Epidemiol Biomarkers Prev. 2015;24:1167–1173.
- Fitzpatrick M, Brooks JT, Kaplan JE. Epidemiology of HIV-associated lung disease in the United States. Semin Respir Crit Care Med. 2016;37:181–198.
- D’Jaen GA, Pantanowitz L, Bower M, et al. Human immunodeficiency virus-associated primary lung cancer in the era of highly active antiretroviral therapy: a multi-institutional collaboration. Clin Lung Cancer. 2010;11:396–404.
- Hessol NA, Martinez-Maza O, Levine AM, et al. Lung cancer incidence and survival among HIV-infected and uninfected women and men. Aids. 2015;29:1183–1193.
- Vandenhende MA, Roussillon C, Henard S, et al. Cancer-related causes of death among HIV-infected patients in France in 2010: evolution since 2000. PloS one. 2015;10:e0129550.
- Shiels MS, Pfeiffer RM, Gail MH, et al. Cancer burden in the HIV-infected population in the United States. J Natl Cancer Inst. 2011;103:753–762.
- Chaturvedi AK, Pfeiffer RM, Chang L, et al. Elevated risk of lung cancer among people with AIDS. Aids. 2007;21:207–213.
- Kirk GD, Merlo C, O’driscoll P, et al. HIV infection is associated with an increased risk for lung cancer, independent of smoking. Clin Infect Dis. 2007;45:103–110.
- Shiels MS, Cole SR, Mehta SH, et al. Lung cancer incidence and mortality among HIV-infected and HIV-uninfected injection drug users. J Acquir Immune Defic Syndr. 2010;55:510–515.
- Wistuba II, Behrens C, Milchgrub S, et al. Comparison of molecular changes in lung cancers in HIV-positive and HIV-indeterminate subjects. JAMA. 1998;279:1554–1559.
- Borges AH, Silverberg MJ, Wentworth D, et al. Predicting risk of cancer during HIV infection: the role of inflammatory and coagulation biomarkers. Aids. 2013;27:1433–1441.
- Staitieh B, Guidot DM. Noninfectious pulmonary complications of human immunodeficiency virus infection. Am J Med Sci. 2014;348:502–511.
- Almodovar S, Knight R, Allshouse AA, et al. Human immunodeficiency virus nef signature sequences are associated with pulmonary hypertension. AIDS Res Hum Retroviruses. 2012;28:607–618.
- Witkowski W, Verhasselt B. Contributions of HIV-1 Nef to immune dysregulation in HIV-infected patients: a therapeutic target? Expert Opin Ther Targets. 2013;17:1345–1356.
- Landi A, Iannucci V, Nuffel AV, et al. One protein to rule them all: modulation of cell surface receptors and molecules by HIV Nef. Curr HIV Res. 2011;9:496–504.
- Marecki JC, Cool CD, Parr JE, et al. HIV-1 Nef is associated with complex pulmonary vascular lesions in SHIV-nef-infected macaques. Am J Respir Crit Care Med. 2006;174:437–445.
- Almodovar S, Hsue PY, Morelli J, et al. Pathogenesis of HIV-associated pulmonary hypertension: potential role of HIV-1 Nef. Proc Am Thorac Soc. 2011;8:308–312.
- Hanna Z, Priceputu E, Hu C, et al. HIV-1 Nef mutations abrogating downregulation of CD4 affect other Nef functions and show reduced pathogenicity in transgenic mice. Virology. 2006;346:40–52.
- Zhu X, Guo Y, Yao S, et al. Synergy between Kaposi’s sarcoma-associated herpesvirus (KSHV) vIL-6 and HIV-1 Nef protein in promotion of angiogenesis and oncogenesis: role of the AKT signaling pathway. Oncogene. 2014;33:1986–1996.
- Briggs SD, Scholtz B, Jacque JM, et al. HIV-1 Nef promotes survival of myeloid cells by a Stat3-dependent pathway. J Biol Chem. 2001;276:25605–25611.
- Kramer-Hammerle S, Kohleisen B, Hohenadl C, et al. HIV type 1 Nef promotes neoplastic transformation of immortalized neural cells. AIDS Res Hum Retroviruses. 2001;17:597–602.
- Wolf D, Witte V, Laffert B, et al. HIV-1 Nef associated PAK and PI3-kinases stimulate Akt-independent Bad-phosphorylation to induce anti-apoptotic signals. Nat Med. 2001;7:1217–1224.
- Geleziunas R, Xu W, Takeda K, et al. HIV-1 Nef inhibits ASK1-dependent death signalling providing a potential mechanism for protecting the infected host cell. Nature. 2001;410:834–838.
- He JC, Husain M, Sunamoto M, et al. Nef stimulates proliferation of glomerular podocytes through activation of Src-dependent Stat3 and MAPK1,2 pathways. J Clin Invest. 2004;114:643–651.
- Chen ZL, Zhao SH, Wang Z, et al. Expression and unique functions of four nuclear factor of activated T cells isoforms in non-small cell lung cancer. Chin J Cancer. 2011;30:62–68.
- Simi L, Andreani M, Davini F, et al. Simultaneous measurement of MMP9 and TIMP1 mRNA in human non small cell lung cancers by multiplex real time RT-PCR. Lung Cancer. 2004;45:171–179.
- Sundaram K, Nishimura R, Senn J, et al. RANK ligand signaling modulates the matrix metalloproteinase-9 gene expression during osteoclast differentiation. Exp Cell Res. 2007;313:168–178.
- Melo SA, Esteller M. Dysregulation of microRNAs in cancer: playing with fire. FEBS Lett. 2011;585:2087–2099.
- Croce CM. Causes and consequences of microRNA dysregulation in cancer. Nat Rev Genet. 2009;10:704–714.
- Fukumura D, Xavier R, Sugiura T, et al. Tumor induction of VEGF promoter activity in stromal cells. Cell. 1998;94:715–725.
- Greenway AL, McPhee DA, Allen K, et al. Human immunodeficiency virus type 1 Nef binds to tumor suppressor p53 and protects cells against p53-mediated apoptosis. J Virol. 2002;76:2692–2702.
- Gregoire IP, Richetta C, Meyniel-Schicklin L, et al. IRGM is a common target of RNA viruses that subvert the autophagy network. PLoS Pathog. 2011;7:e1002422.
- Rao S, Tortola L, Perlot T, et al. A dual role for autophagy in a murine model of lung cancer. Nat Commun. 2014;5:3056.
- Mowers EE, Sharifi MN, Macleod KF. Autophagy in cancer metastasis. Oncogene. 2016;36:1619–1630.
- Gomes LC, Di Benedetto G, Scorrano L. During autophagy mitochondria elongate, are spared from degradation and sustain cell viability. Nat Cell Biol. 2011;13:589–598.
- Chou CH, Lin CC, Yang MC, et al. GSK3beta-mediated Drp1 phosphorylation induced elongated mitochondrial morphology against oxidative stress. PloS one. 2012;7:e49112.
- Olivetta E, Pietraforte D, Schiavoni I, et al. HIV-1 Nef regulates the release of superoxide anions from human macrophages. Biochem J. 2005;390:591–602.
- Bonnet S, Archer SL, Allalunis-Turner J, et al. A mitochondria-K+ channel axis is suppressed in cancer and its normalization promotes apoptosis and inhibits cancer growth. Cancer Cell. 2007;11:37–51.
- Aqil M, Naqvi AR, Bano AS, et al. The HIV-1 Nef protein binds argonaute-2 and functions as a viral suppressor of RNA interference. PloS one. 2013;8:e74472.
- Houzet L, Yeung ML, de Lame V, et al. MicroRNA profile changes in human immunodeficiency virus type 1 (HIV-1) seropositive individuals. Retrovirology. 2008;5:118.
- Bennasser Y, Le SY, Benkirane M, et al. Evidence that HIV-1 encodes an siRNA and a suppressor of RNA silencing. Immunity. 2005;22:607–619.
- Grinberg M, Gilad S, Meiri E, et al. Vaccinia virus infection suppresses the cell microRNA machinery. Arch Virol. 2012;157:1719–1727.
- Landi MT, Zhao Y, Rotunno M, et al. MicroRNA expression differentiates histology and predicts survival of lung cancer. Clin Cancer Res off J Am Assoc Cancer Res. 2010;16:430–441.
- Kumar MS, Lu J, Mercer KL, et al. Impaired microRNA processing enhances cellular transformation and tumorigenesis. Nat Genet. 2007;39:673–677.