ABSTRACT
Corneal neovascularization (CRNV) is a prevalence eye disorder that affects the transparency and refraction properties of eyes. To explore the correlation between the level of Angiotensin II (Ang II) and corneal angiogenesis, the rat model of CRNV was established using alkali-burn, while the human umbilical vein endothelial cells (HUVECs) were stimulated using VEGF to induce the CRNV cells in vitro. RNA immunoprecipitation (RIP) and RNA pull-down were performed to validate the relationship between MIAT and miR-1246. The expression of MIAT and Ang II was increased, while miR-1246 was decreased in CRNV rat model. VEGF stimulation significantly promoted cell proliferation and migration of HUVECs, knockdown of MIAT dramatically reversed the effects of VEGF, while cells co-transfected with miR-1246 inhibitor obviously abolished the effect of VEGF+si-MIAT, however, enalaprilat abolished the effects of VEGF+si-MIAT+miR-1246 inhibitor. MIAT directly regulated the expression of miR-1246. In conclusion, VEGF stimulation promoted cell proliferation and migration of HUVECs mainly through regulating MIAT/miR-1246/ACE.
Introduction
Corneal neovascularization (CRNV), the pathological process of the cornea that affects the transparency and refraction properties, is often occurring by trauma, hypoxia, inflammation, infection toxicity, corneal transplant and nutritional insults [Citation1–Citation3]. The pathogenesis has been demonstrated to associate with the balance between angiogenic and antiangiogenic factors. Even though great progress has been achieved in CRNV treatment, it nonetheless did not satisfy the demands of patients. Thus, to explore the possible mechanism of CRNV is essential for its further treatment.
The rennin-angiotensin system (RAS) is a powerful regulator of blood in the human body and plays an important role in brain, heart, kidney, vessels, pancreas and gonads. The RAS is constituted by kidney rennin and Angiotensin-converting enzyme (ACE). ACE is a single carboxypeptidase, which has been considered to promote the conversion of angiotensin I (Ang I) to angiotensin II (Ang II). The previous study has reported that ACE could promote CRNV, and Enalapril, an inhibitor of ACE, could significantly decrease corneal angiogenesis in VEGF-induced rabbit model of CRNV [Citation4]. The Ang II receptor could inhibit the corneal angiogenesis [Citation5]. The angiotensin II stimulates Ang II expression through AT1 receptor-mediated PKC and MAPK pathways in cultured bovine retinal endothelial cells, indicating that Ang II may work a new role in CRNV of cow [Citation6]. Nevertheless, whether Ang II plays an important role in CRNV was still required further exploration.
Long non-coding RNA (lncRNA) is aset of non-coding RNA, with the length of more than 200 nucleotides (nt) and regulates gene expression in post-transcription. Large evidence has revealed that lncRNA is involved in various biological processes, and mediated cellular growths, oxidative stress and metastasis. LncRNA is abnormally expressed in many diseases, and functions as a vital regulator in gene expression. For example, the abnormally upregulated lncRNA H19 has existed in multiple myeloma, and further regulates the activation of the NF-kB pathway [Citation7]. LncRNA LINC00978 is adiagnostic biomarker of gastric cancer [Citation8]. LncRNA TUG1 knockdown could significantly ameliorate atherosclerosis [Citation9]. In addition, lncRNA H19 negatively regulates corneal epithelial proliferation [Citation10]. LncRNA MIAT is a regulator of endothelial cell dysfunction and regulates the neurovascular remodeling in the psyche and optic [Citation11]. Moreover, MIAT was overexpressed in diabetes and diabetic retinopathy, and up-regulated in hypoxia-induced Muller cell and human umbilical vein endothelial cell (HUVEC), while the role of MIAT in CRNV was still unreadable.
MicroRNA (miRNA) is a category of non-coding RNA with the length of ~22 nt. MiRNA regulates the gene expression at the post-transcriptional level by binding at the 3ʹUTR of mRNA and thus inhibits the protein expression. MiR-1246 plays an important role in breast cancer [Citation12], and inhibits cell invasion and epithelial-mesenchymal transition in lung cancer [Citation13]. The previous study revealed that miR-1246 was down-regulated in CRNV, and overexpressed miR-1246 could significantly inhibit the proliferation of HUVEC [Citation14]. Furthermore, ACE is the target gene of miR-1245 [Citation15]. Recently, the competing endogenous RNA (ceRNA) has been crosstalk between lncRNA and miRNA. In other words, lncRNA acts as a sponge of miRNA and further release miRNA’s inhibitory function to target genes. The online prediction revealed that MIAT could bind with miR-1246; thus, we infer that MIAT could sponge miR-1246 to regulate the corneal angiogenesis.
In the present study, the expression of MIAT, miR-1246 and AngII was detected. The interaction between MIAT and miR-1246 was determined. Our purpose is to explore the possible mechanism of CRNV.
Materials and methods
Animals and treatments
A total of 20 healthy Wister rats (4–6 weeks old, 200 ± 20 g) were purchased from Shanghai National Laboratory Animal Center. The animals were fed in a humidified atmosphere with 12 h day/night cycle, the water and food were available. The experiments were approved by the Experimental Animal Committee of the First Affiliated Hospital of Zhengzhou University.
The animals were randomly divided into two groups, including control and CRNV groups. The CRNV rats were anesthetized with pentobarbital and then received a drop of tetracaine. The NaOH was used to induce alkali burn in the corneal surface. One week later, the corneal tissues of the rats were isolated forfurther experiments.
Cell culture and treatment
Human umbilical vein endothelial cells (HUVEC) were purchased from American Type Culture Collection (ATCC) and cultured in a 96-well plate and maintained using endothelial cell medium (ECM), which supplemented with 10% fetal bovine serum at 37°C in 5% CO2. VEGF was obtained from Sigma. Cells (5 × 104) were stimulated with 100 ng/ml of VEGF and then incubated at 37°C for 24 h.
Real-time PCR
Total RNA was isolated from cells or tissues using TRIzol Reagent (Invitrogen). The quality was determined using a 0.8% agarose gel. cDNA was synthesized using 1 μg of RNA and PrimeScriptTM RT reagent Kit (Takara). The cDNA was used for real-time PCR according to the instructions of the qRT-PCR kit. GAPDH was used as an internal control. The relative expression was determined using the 2−ΔΔCt method.
Western blot
The protein was isolated from tissues or cells using RIPA buffer. The protein quality was determined using the Enhanced BCA Protein Assay Kit (Beyotime, Shanghai, China). An equal amount of protein was separated using SDS-PAGE, and the protein was transferred onto polyvinyl difluoride (PVDF) membrane and incubated with the primary antibodies at 4°C for 24 h. After washing with TBS, the membrane was incubated with the second antibody at room temperature for 1 h. The protein bands were visualized using enhanced chemiluminescence reagents.
Cell proliferation
Cell proliferation was performed using MTT assay. Briefly, cells were cultured in a 96-well plate until a final density of 1 × 105 per well. The MTT (Sigma, Japan) reagent was used to evaluate the cell proliferation according to its manufacturer’s protocols.
Cell migration
Cell migration was determined using Transwell chambers as previously described [Citation16]. Briefly, cells were starved overnight, and suspended with the concentration of 1 × 105 per well. Cells were planted in the upper chamber of the transwell chambers, while the VEGF was loaded in the lower chamber. The chambers were incubated at 37°C for 24 h. The migrated cells in the lower chamber were fixed, stained and finally counted using a microscope in five randomly selected fields.
RNA immunoprecipitation (RIP)
Online TagetScan predicted that MIAT could bind with miR-1246. To confirm the relationship between MIAT and miR-1246, the RIP assay was performed using a RIP RNA-Binding Protein Immunoprecipitation Kit (Beyotime, Shanghai, China). Briefly, HUVECs were lysed using lysis buffer and digested using RNase. The cell extracts were incubated with magnetic beads conjugated with Ago2 antibody. Then, the proteinase K was used for the protein digestion. Following, the real-time PCR was performed using the immunoprecipitated RNA. Total RNA and mouse IgG were detected to determine signals of RNAs that specifically bound to Ago2.
RNA pull-down
RNA pull-down assay was carried out to identify the relationship between MIAT and miR-1246. In brief, biotin-labeled RNA was incubated with cellular protein extracts (1 mg), mixed with prewashed streptavidin-agarose beads for 1 h. The proteins were resolved by SDS-PAGE, and the selected bands were identified using mass spectrometry and Western blotting.
Cell transfection
Cells were maintained in a 24-well plate for 24 h. Cell transfection was performed using Lipofectamine 2000 Reagent (Invitrogen, USA). Si-control and si-MIAT were purchased from Invitrogen (Shanghai, China). miR-1246 inhibitor and the negative control were synthesized from Invitrogen (Shanghai, China).
CRNV model establishment
The healthy Wister rats were housed in temperature, humidity, and light controlled room. Food and water were available ad libitum. All rats were confirmed to be free of diseases before experimentation. The rats were anesthetized with an intraperitoneal injection of 40 mg/kg pentobarbital and received topical administration with a drop of tetracaine. A round filter paper (3.5 mm in diameter) soaked with 1 mM NaOH was placed on the center of the corneal surface for 30 sec to induce alkali burn. The ocular surface was then rinsed with 10 mL PBS. After the alkali burn injury was successfully established, the rats were immediately divided into two groups (n = 6 in each group) and the one group received conjunction injection of 20 μl si-control, and the other received 20 μl of si-MIAT. The lengths and areas of rats’ CNVs were detected on day 3, 5 and 7 using the slit lamp microscopy. The rats were sacrificed on day 7th after the operation.
Statistical analysis
Data were presented as means±SD, the ANOVA was done to examine the statistical deviation. P < 0.05 is considered statistically significant.
Results
The expression of MIAT was increased in the rat tissues of CRNV
We first investigate the expression of MIAT, miR-1246 and AngII in the rat tissue of CNV. The real-time PCR revealed that MIAT was increased, while miR-126 was deceased in the CRNV tissues (n = 10 rats) compared with control (n = 10 rats) (). Real-time PCR and western blot presented that both mRNA and protein expression of AngII and ACE were dramatically increased while AngI was decreased in comparing with control (). *p < 0.05 vs control.
Figure 1. The expression pattern of MIAT in corneal neovascularization tissues of rat model. (a) The expression of MIAT was determined using real-time PCR; (b) The expression of miR-1246 was detected using real-time PCR; (c) The expression of AngII, AngI and ACE was measured using real-time PCR and western blot. *P < 0.05 vs control.
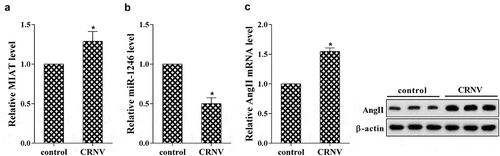
MIAT knockdown significantly suppressed cell proliferation and migration
In order to confirm the role of MIAT on cell proliferation and migration, HUVEC cells were transfected with si-MIAT and stimulated using 100 ng/mL VEGF. The results demonstrated that VEGF stimulation promoted the expression of MIAT, while si-MIAT significantly decreased its expression (). Furthermore, the expression of miR-1246 was significantly decreased in the stimulation of VEGF, while si-MIAT transfection reversed the effect of VEGF (). VEGF stimulation also promoted the expression of AngII and ACE but decreased AngI, however, si-MIAT transfection abolished the effect of VEGF (). Additionally, VEGF stimulation promoted cell migration and proliferation, while si-MIAT transfection abolished the effects of VEGF (). *p < 0.05 vs control; #p < 0.05 vs VEGF+si-control.
Figure 2. Effects of MIAT on cell proliferation and migration of HUVECs. (a) Effects of VEGF and si-MIAT on the expression of MIAT was determined using real-time PCR; (b) Effects of VEGF and si-MIAT on the expression of miR-1246 was measured using real-time PCR; (c) Effects of VEGF and si-MIAT on the expression of AngII, AngI and ACE was determined using real-time PCR and western blot; (d) Effects of VEGF and si-MIAT on the expression of cell migration and proliferation were determined using transwell assay and MTT assay, respectively. (e) The cell migration pictures under the treatments of VEGF and si-MIAT. *P < 0.05 vs control, #P < 0.05 vs si-control + VEGF.
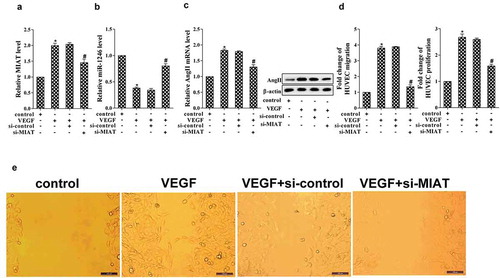
MIAT directly regulated the expression of miR-1246
Online prediction revealed that MIAT could bind with miR-1246 (). Then, RIP and RNA pull-down were performed to verify the relationship between MIAT and miR-1246. RIP-western assay revealed that MIAT and miR-1246 were enriched in AGO2, and real-time PCR revealed that MIAT and miR-1246 were increased in AGO2 compared with IgG (). RNA pull-down showed that MIAT pulled down AGO2 in compound (). MiR-1246 was enriched in the compounds of MIAT (). *p < 0.05 vs IgG; #p < 0.05 vs NC.
Figure 3. MIAT regulates the expression of miR-1246. (a) Online prediction found that MIAT could bind with miR-1246. (b) RIP assay revealed that MIAT and miR-1246 were accumulated in AGO2. (c) RNA pull-down revealed that MIAT pulled down the AGO2 in the compound. (d) miR-1246 was accumulated in the pull-down compound of MIAT. *P < 0.05 vs IgG, #P < 0.05 vs NC.
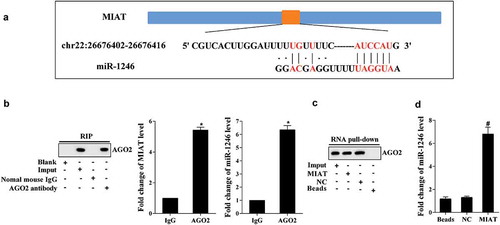
VEGF stimulation regulated the expression of ACE via MIAT/miR-1246
To determine the role of VEGF on the expression of ACE, the HUVECs were divided into six groups. As is presented in , VEGF stimulation significantly promoted the expression of ACE, MIAT knockdown decreased the expression of ACE, while miR-1246 knockdown reversed the effect of MIAT knockdown (). Additionally, the expression of AngII was increased under the stimulation of VEGF, MIAT knockdown abolished the effects of VEGF, while miR-1246 knockdown reversed the effects of MIAT knockdown (). *p < 0.05 vs control; #p < 0.05 vs VEGF+si-control; &p vs si-MIAT+NC.
VEGF stimulation promoted cell migration and proliferation via MIAT/miR-1246/ACE
To determine the role of VEGF in cell migration and proliferation, HUVEC cells were randomly divided into seven groups and received different treatments. As is presented in , VEGF stimulation promoted cell proliferation and migration, while si-MIAT transfection abolished it. Cells co-transfected with miR-1246 inhibitor reversed the effects of si-MIAL, while enalaprilat abolished the effect of miR-1246 inhibitor. *p < 0.05 vs control; #p < 0.05 vs VEGF+si-control; &p vs si-MIAT+NC; $p vs miR-1246 inhibitor.
Figure 5. Effects of VEGF, MIAT, miR-1246 and enalaprilat on cell proliferation and migration. *P < 0.05 vs control, #P < 0.05 vs si-control, &P < 0.05 vs NC, $P < 0.05 vs miR-1246 inhibitor.
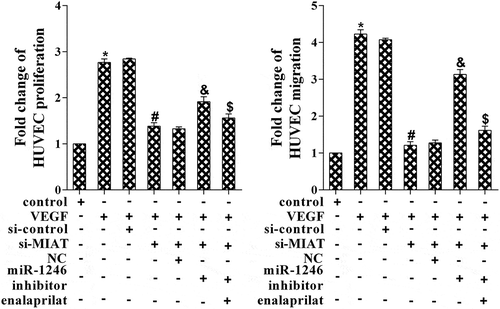
In addition, cell apoptosis of HUVECs were determined using Annenix V-FITC/7-AAD double staining. The results revealed that VEGF stimulation significantly suppressed cell apoptosis, while MIAT knockdown reversed the effect of VEGF, then miR-1246 inhibitor transfection significantly reversed the effects of si-MIAT, however, enalaprilat abolished the effect of si-MIAT (Supplementary figure 1). *p < 0.05 vs control; #p < 0.05 vs VEGF+si-control; &p vs si-MIAT+NC; $p vs miR-1246 inhibitor.
In vivo to examine the effects of MIAT on the generation of CRNV
The CRNV rat model was received the conjunctival injection of si-control or si-MIAT (20 μL). As is presented in , si-MIAT treatment significantly decreased the length and area of CRNV (). Real-time PCR revealed that si-MIAT significantly suppressed the expression of MIAT but decreased miR-1246, western blot showed that the expression of AngII was significantly decreased in si-MIAT group (). *p < 0.05 vs si-control.
Figure 6. In vivo to validate the role of si-MIAT on corneal neovascularization. (a) The lengths and areas of corneal neovascularization on day 3, 5 and 7 after operation. (b) The expression of MIAT and miR-1246 was determined using real-time PCR, the expression of AngII was determined using western blot. *P < 0.05 vs si-control.
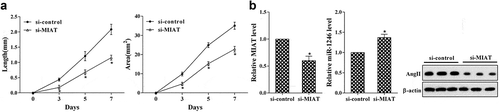
Discussion
Corneal neovascularization is a popular eye problem in the recent clinical data. Mounting of studies has focused on the mechanism of corneal neovascularization, and models of alkali-burn injury have been widely utilized. For example, Sun et al. explored cell cycle and apoptosis of corneal neovascularization based on the alkali-burn injured rat model [Citation17]. The alkali-burn injured rat model of corneal neovascularization was used to identify the inflammatory and oxidative mechanism [Citation18]. Additionally, the alkali-burn injured rat model of corneal injury was also taken to explore the retinal damage [Citation19], the therapeutic effects of antagomir-21 [Citation20] and the inflammatory fibrosis [Citation21]. In the present study, the corneal neovascularization rat model was established using the alkali-burn, our results obtained that the in vivo model was highly reproducible, which provided meaningful instruction for further corneal neovascularization study. HUVECs are characterized by the potency of stem cells, and widely used in the in vitro experiments of vascular endothelium. Vascular endothelial growth factor (VEGF) is a direct angiogenesis stimulator and widely used to evaluate the anti-VEGF factors [Citation22]. In our present study, VEGF was used to stimulate the HUVECs and mimicked the corneal neovascularization in vitro, which should accessible and reproducible.
AngII is consisted of AT1R and AT2R receptors, AT1R mainly conducted cell proliferation and smooth muscle contraction, while AT2R mainly modulates cell apoptosis and smooth muscle relaxation [Citation23]. It has been reported that AngII is mediated in various diseases, such as renal disease [Citation24], energy metabolism [Citation25], and myocardial disease [Citation26]. The previous study identified that AngII is involved in angiogenesis [Citation27]. In our present study, the expression of AngII is abnormally increased in the tissues of CRNV rat model, indicating the possible role of AngII in CNV. In addition, the ACE is the important promoter of AngI transited to AngII, thus higher level of AngII might also result in higher expression of ACE.
The miR-1246 expression profile has been reported in the preliminary study, which showed that curcumin significantly promoted the expression of miR-1246 in HUVECs, and further inhibited cell apoptosis [Citation14]. Another study validated that miR-1246 targets ACE to regulate its work. Therefore, we conducted that the expression of AngII was indirectly regulated by miR-1246.
Further, to identify the regulator of miR-1246, we obtained that MIAT acted as the ceRNA to regulate its expression. And the expression of MIAT related to the levels of miR-1246 and AngII in HUVECs. Additionally, knockdown of MIAT significantly decreased cell proliferation and migration in VEGF-induced HUVECs. The results indicated that MIAT served as an important biomarker in corneal neovascularization.
The currently used CRNV animal models mainly include (i) physically induced CRNV animal model with thermal cauterization or corneal suture. For the thermal cauterization method, perforation of the cornea caused by too much burning easily leads to modeling failure [Citation28]; for the corneal suture method, the success of suture is greatly affected by the skill of the surgeon; too deep or too shallow leads to corneal perforation or suture loss [Citation29]; (ii) corneal pocket implantation induced CRNV model. This model is too difficult to establish, and the operation itself can cause CRNV [Citation30–Citation32]; (iii) corneal xenografts, which may lead to immune rejection of receptors [Citation33]; (iv) alkali burn-induced CRNV model. This model is a good simulation of the pathological process of human corneal alkali burn. Moreover, this model neither cause corneal perforation, nor affects the measurement of new blood vessels, which costs low and is easy to operate [Citation34,Citation35], indicating that alkali burn-induced CRNV model is the ideal animal model, and is widely used in CRNV studies [Citation36–Citation38]. Alkali burn-induced CRNV model was used during our 2-year’s study, which is an ideal CRNV model in studying corneal chemical burns, thermal burns, surgical trauma, and cornea-related autoimmune diseases.
In summary, the present study demonstrated that MIAT knockdown significantly suppressed the corneal neovascularization, among which, miR-1246/ACE served as the critical elements. The present study might provide promising therapeutic targets for further clinical therapy of corneal neovascularization.
Authors’ contributions
YB put forward the concept of the study, designed the study, prepared the manuscript and contributed to the statistical analysis. WW contributed to the data acquisition. YZ contributed to the quality control of data and algorithms. FZ analyzed the data and interpretation. HZ edited the manuscript.
Ethics approval and consent to participate
The study was approved by the ethics committee of The First Affiliated Hospital of Zhengzhou University.
Consent for publication
The study was undertaken with the patient’s consent.
Supplemental Material
Download Zip (3.7 MB)Disclosure statement
No potential conflict of interest was reported by the authors.
Supplementary material
Supplemental data for this article can be accessed here.
Additional information
Funding
References
- Chen P, Yin H, Wang Y, et al. Inhibition of VEGF expression and corneal neovascularization by shRNA targeting HIF-1α in a mouse model of closed eye contact lens wear. Mol Vis. 2012;18:864–873.
- Menzel-Severing J. Emerging techniques to treat corneal neovascularisation. Eye (Lond). 2012;26:2–12.
- Qazi Y, Wong G, Monson B, et al. Corneal transparency: genesis, maintenance and dysfunction. Brain Res Bull. 2010;81:198–210.
- Sharma A, Bettis DI, Cowden JW, et al. Localization of angiotensin converting enzyme in rabbit cornea and its role in controlling corneal angiogenesis in vivo. Mol Vis. 2010;16:720–728.
- Usui T, Sugisaki K, Iriyama A, et al. Inhibition of corneal neovascularization by blocking the angiotensin II type 1 receptor. Invest Ophthalmol Vis Sci. 2008;49:4370–4376.
- Otani A, Takagi H, Oh H, et al. Angiotensin II induces expression of the Tie2 receptor ligand, angiopoietin-2, in bovine retinal endothelial cells. Diabetes. 2001;50:867–875.
- Sun Y, Pan J, Zhang N, et al. Knockdown of long non-coding RNA H19 inhibits multiple myeloma cell growth via NF-κB pathway. Sci Rep. 2017;7:18079.
- Fu M, Huang Z, Zang X, et al. Long noncoding RNA LINC00978 promotes cancer growth and acts as a diagnostic biomarker in gastric cancer. Cell Prolif. 2017;51:e12425.
- Zhang L, Cheng H, Yue Y, et al. TUG1 knockdown ameliorates atherosclerosis via up-regulating the expression of miR-133a target gene FGF1. Cardiovasc Pathol. 2018;33:6–15.
- Klein RH, Stephens DN, Ho H, et al. Cofactors of LIM domains associate with estrogen receptor α to regulate the expression of noncoding RNA H19 and corneal epithelial progenitor cell function. J Biol Chem. 2016;291:13271–13285.
- Jiang Q, Shan K, Qun-Wang X, et al. Long non-coding RNA-MIAT promotes neurovascular remodeling in the eye and brain. Oncotarget. 2016;7:49688–49698.
- Li XJ, Ren ZJ, Tang JH, et al. Exosomal MicroRNA MiR-1246 promotes cell proliferation, invasion and drug resistance by targeting CCNG2 in breast cancer. Cell Physiol Biochem. 2017;44:1741–1748.
- Xu X, Cao L, Zhang Y, et al. MicroRNA-1246 inhibits cell invasion and epithelial mesenchymal transition process by targeting CXCR4 in lung cancer cells. Cancer Biomark. 2017;21:251–260.
- Bai Y, Wang W, Sun G, et al. Curcumin inhibits angiogenesis by up-regulation of microRNA-1275 and microRNA-1246: a promising therapy for treatment of corneal neovascularization. Cell Prolif. 2016;49:751–762.
- Fang Y, Gao F, Hao J, et al. microRNA-1246 mediates lipopolysaccharide-induced pulmonary endothelial cell apoptosis and acute lung injury by targeting angiotensin-converting enzyme 2. Am J Transl Res. 2017;9:1287–1296.
- Jin PY, Lu HJ, Tang Y, et al. The effect of DNA-PKcs gene silencing on proliferation, migration, invasion and apoptosis, and in vivo tumorigenicity of human osteosarcoma MG-63 cells. Biomed Pharmacother. 2017;96:1324–1334.
- Sun Y, Su L, Wang Z, et al. H-RN, a peptide derived from hepatocyte growth factor, inhibits corneal neovascularization by inducing endothelial apoptosis and arresting the cell cycle. BMC Cell Biol. 2013;14:8.
- Oguido A, Hohmann MSN, Pinho-Ribeiro FA, et al. Naringenin eye drops inhibit corneal neovascularization by anti-inflammatory and antioxidant mechanisms. Invest Ophthalmol Vis Sci. 2017;58:5764–5776.
- Paschalis EI, Zhou C, Lei F, et al. mechanisms of retinal damage after ocular alkali burns. Am J Pathol. 2017;187:1327–1342.
- Zhang Y, Zhang T, Ma X, et al. Subconjunctival injection of antagomir-21 alleviates corneal neovascularization in a mouse model of alkali-burned cornea. Oncotarget. 2017;8:11797–11808.
- Okada Y, Shirai K, Miyajima M, et al. Loss of TRPV4 function suppresses inflammatory fibrosis induced by alkali-burning mouse corneas. PLoS One. 2016;11:e0167200.
- Nauta TD, Duyndam MC, Weijers EM, et al. HIF-2α expression regulates sprout formation into 3D fibrin matrices in prolonged hypoxia in human microvascular endothelial cells. PLoS One. 2016;11:e0160700.
- Williams PJ, Mistry HD, Innes BA, et al. Expression of AT1R, AT2R and AT4R and their roles in extravillous trophoblast invasion in the human. Placenta. 2010;31:448–455.
- Tassi E, Lai EY, Li L, et al. Blood pressure control by a secreted FGFBP1 (fibroblast growth factor-binding protein). Hypertension. 2018;71:160–167.
- Than A, Xu S, Li R, et al. Angiotensin type 2 receptor activation promotes browning of white adipose tissue and brown adipogenesis. Signal Transduct Target Ther. 2017;2:17022.
- Verjans R, Peters T, Beaumont FJ, et al. MicroRNA-221/222 family counteracts myocardial fibrosis in pressure overload-induced heart failure. Hypertension. 2017;71:280–288.
- Arrieta O, Villarreal-Garza C, Vizcaino G, et al. Association between AT1 and AT2 angiotensin II receptor expression with cell proliferation and angiogenesis in operable breast cancer. Tumour Biol. 2015;36:5627–5634.
- Boneham GC, Collin HB. Steroid inhibition of limbal blood lymphatic vascular cell growth. Curr Eye Res. 1995;14(1):1–10.
- Dana MR, Zhu SN, Yamada J. Topical modulation of interleukin-1 activity in corneal neovascularization. Cornea. 1998;17(4):403–409.
- Li WW, Grayson G, Folkman J, et al. Sustained-release endotoxin. A model for inducing corneal neovascularization. Invest Ophthalmol Vis Sci. 1991;32(11):2906–2911.
- Murata T, Ishibashi T, Yoshikawa H, et al. Tecogalan sodium inhibits corneal neovascularization induced by basic fibroblast growth factor. Ophthalmic Res. 1995;27(6):330–334.
- Becker MD, Kruse FE, Azzam L, et al. In vivo significance of ICAM-1 dependent leukocyte adhesion in early corneal angiogenesis. Invest Ophthalmol Vis Sci. 1999;40(3):612–618.
- Benelli U, Ross JR, Nardi M, et al. Corneal neovascularization induced by xenografts or chemical cautery. Inhibition by cyclosporine A. Invest Ophthalmol Vis Sci. 1997;38(2):274–282.
- Conners MS, Urbano F, Vafeas C, et al. Alkli burn-induced synthesis of inflammatory eicosanoids in rabbit corneal epithelium. Invest Ophthalmol Vis Sci. 1997;38(10):1963–1971.
- Giacomini C, Ferrari G, Bignami F. Alkali burn versus suture-induced corneal neovascularization in C57BL/6 mice: an overview of two common animal models of corneal. Exp Eye Res. 2014;121:1–4.
- Chen L, Zhong J, Li S, et al. The long-term effect of tacrolimus on alkali burn-induced corneal neovascularization and inflammation surpasses that of anti-vascular endothelial growth factor. Drug Des Devel Ther. 2018 Sep 12;12:2959–2969.
- Tang M, Yang Y, Yu J, et al. Tetramethylpyrazine in a murine alkali-burn model blocks NFκB/NRF-1/CXCR4-signaling-induced corneal neovascularization. Invest Ophthalmol Vis Sci. 2018 Apr 1;59(5):2133–2141.
- Han Y, Shao Y, Liu T, et al. Therapeutic effects of topical netrin-4 inhibits corneal neovascularization in alkali-burn rats. PLoS One. 2015 Apr 8;10(4):e0122951.