ABSTRACT
Noc3p (Nucleolar Complex-associated protein) is an essential protein in budding yeast DNA replication licensing. Noc3p mediates the loading of Cdc6p and MCM proteins onto replication origins during the M-to-G1 transition by interacting with ORC (Origin Recognition Complex) and MCM (Minichromosome Maintenance) proteins. FAD24 (Factor for Adipocyte Differentiation, clone number 24), the human homolog of Noc3p (hNOC3), was previously reported to play roles in the regulation of DNA replication and proliferation in human cells. However, the role of hNOC3 in replication licensing was unclear. Here we report that hNOC3 physically interacts with multiple human pre-replicative complex (pre-RC) proteins and associates with known replication origins throughout the cell cycle. Moreover, knockdown of hNOC3 in HeLa cells abrogates the chromatin association of other pre-RC proteins including hCDC6 and hMCM, leading to DNA replication defects and eventual apoptosis in an abortive S-phase. In comparison, specific inhibition of the ribosome biogenesis pathway by preventing pre-rRNA synthesis, does not lead to any cell cycle or DNA replication defect or apoptosis in the same timeframe as the hNOC3 knockdown experiments. Our findings strongly suggest that hNOC3 plays an essential role in pre-RC formation and the initiation of DNA replication independent of its potential role in ribosome biogenesis in human cells.
Introduction
DNA replication is a strictly regulated process for duplicating the genomic DNA faithfully once per cell cycle. Pre-RC (pre-replicative complexes) assembly is one of the mechanisms that regulate DNA replication. To initiate DNA replication, pre-RCs have to be assembled at replication origins in a stepwise manner during the M-to-G1 transition [Citation1–Citation2]. In budding yeast, the six subunits of ORC and Noc3p bind to replication origins throughout the cell cycle, where they function as the origin recognition scaffold [Citation3–Citation5]. Pre-RC assembly begins at the M-to-G1 transition with the loading of Cdc6p by Orc1-6p, Noc3p and Ipi3 [Citation3–Citation6], followed by the association of the Cdt1p-MCM2-7p heptamer [Citation7–Citation16]. After pre-RC assembly, replication origins are “licensed” and ready for firing (origin activation) [Citation17–Citation19]. From late G1 to S-phase, pre-RCs are disassembled, while pre-RC reassembly and re-replication within the same cell cycle are inhibited through multiple mechanisms, primarily by the phosphorylation of some pre-RC components [Citation20–Citation25]. In late M-phase, Cdc14p dephosphorylates pre-RC components to allow a new round of licensing for DNA replication in the next cell cycle [Citation26]. The importance of pre-RC assembly, regulation and genetic integrity is emphasized by the existence of functionally conserved homologs of these proteins in many eukaryotes, including Saccharomyces cerevisiae, Schizosaccharomyces pombe, Drosophila melanogaster, Xenopus laevis, Mus musculus and Homo sapiens, etc [Citation27–Citation30].
Noc3p was initially identified as a part of the heterodimeric Noc1p-Noc2p and Noc2p-Noc3p complexes that play roles in ribosome biogenesis in the budding yeast Saccharomyces cerevisiae [Citation31–Citation33]. It was later shown to be essential for the chromatin association of Cdc6p and MCM proteins during pre-RC formation and S-phase entry [Citation5]. It associates with chromatin throughout the cell cycle in vivo at replication origins, commonly known as ARS (autonomously replicating sequence) elements. FAD24 (Factor for Adipocyte Differentiation, clone number 24) was identified as the human homolog of the budding yeast Noc3p, with 30% identity and 47% similarity between the two protein sequences. Previous publications indicate that the homologs of Noc3p in humans and other eukaryotes (Mus musculus and Sus scrofa domesticus) are involved in various biological processes including adipogenesis, cell cycle re-entry, DNA replication, cell proliferation and hyperplasia [Citation27,Citation34–Citation37]. However, the functions of hNOC3 in replication licensing was unclear.
In this report, we show that hNOC3 is essential for pre-RC assembly and DNA replication in human cells. hNOC3 binds chromatin throughout the cell cycle, and preferentially associates with known human replication origins. hNOC3 is required for the recruitment of hCDC6 and hMCM to the chromatin during pre-RC assembly at the M-to-G1 transition. Knockdown of hNOC3 led to defects in S-phase entry, reduced EdU incorporation, and eventual apoptosis. Moreover, hNOC3 physically interacts with multiple pre-RC proteins including hORC and hMCM proteins. We also demonstrate that defects in ribosome biogenesis, by specific inhibition of the ribosome biogenesis pathway through knockdown of RPA194 (the largest subunit of RNA polymerase I) or treatment with the RNA polymerase I inhibitor CX-5461, did not result in any observable defect in pre-RC assembly, EdU incorporation or cell cycle progression within a comparable timeframe. Therefore, hNOC3, like hIPI3 and hCDC6 [Citation6,Citation38], plays an essential role in replication licensing and may also serve to coordinate DNA replication and ribosome biogenesis.
Materials and methods
Cell culture and harvest
HeLa cells and HEK-293T cells [Citation39] were cultured in Dulbecco’s Modified Eagle Medium (Life Technologies) with 10% Fetal Bovine Serum and maintained at 37 °C in a humidified incubator with 5% CO2. Cells were harvested as described before [Citation6].
Transient transfection, siRNA and plasmids
Cells were seeded in dishes or multi-well plates of different surface areas (according to experiment requirements) and transfected at 30% – 40% (for siRNA) or 50%-70% (for plasmid) confluency. Lipofectamine RNAiMax (Invitrogen) and Lipofectamine 2000 Reagent (Invitrogen) were used according to the manufacturer’s instructions for siRNA and plasmid transfections, respectively. sihNOC3#1 (sense 5ʹ-3ʹ: GGAGAAGAAGAUGUAUAUUdTdT; antisense 5ʹ-3ʹ: AAUAUGCAUCUUCUUCUCCdTdT) sihNOC3#2 (sense 5ʹ-3ʹ: AGAGACAAAGGAUACUUAAdTdT; antisense 5ʹ-3ʹ: UUGAGUAUCCUUUGUCUCUdTdT) were used at 40 nM to knock down hNOC3.
The HA affinity tag was inserted between the start and the second codon of the hNOC3 coding sequence and cloned into the mammalian expression vector pcDNATM3.1/Zeo (+) (Life Technologies). Plasmids were purified using Endo-free Plasmid Midi Kit (Omega) according to the manufacturer’s instructions.
Cell cycle synchronization and release
L-mimosine (Sigma) was applied to cells at 0.4 mM for 20 to 24 hrs to synchronize cells at the G1/S-phase boundary. Nocodazole (Sigma) was applied at 40 ng/ml to synchronize cells in M-phase for 12 hrs followed by mitotic shake-off to collect loosely attached M-phase cells. Release from the cell cycle blocks was achieved by washing the cells three times with pre-warmed growth medium. Cells at different time points were harvested as desired and cell synchrony was confirmed by flow cytometry.
Chromatin-binding assay
For the chromatin association profile of pre-RC proteins, HeLa cells were synchronized at M-phase by nocodazole, released and seeded in 6-well plates. Cells at different time points were harvested for flow cytometry and chromatin-binding assay as previously described [Citation6,Citation39].
For knockdown assay, HeLa cells were seeded in 6-well plates and transfected with siRNA. Mimosine was applied 4 hrs post-transfection. Cells were then released for 8 hrs and mimosine was re-applied. Cells were harvested after the second mimosine block. Chromatin-binding assays were performed as previously described [Citation6,Citation39].
Chromatin immunoprecipitation (ChIP) assay
HEK 293T cells were plated on 10-cm dishes and transfected with HA-hNOC3-expressing plasmid. Forty-eight hrs later, ChIP-qPCR assay was performed using the same method and primer sets as previously described [Citation6].
Edu incorporation assay
HeLa cells were seeded in 24-well plates and transfected with siRNAs. Mimosine was applied 12 hrs later. Cells were cultured for 30 min in medium containing 50 mM EdU (5-ethynyl-2′-deoxyuridine) 1 hr after release. Cells were then fixed in 4% paraformaldehyde for 30 min. EdU was detected with Cell-Light™ EdU DNA Cell Proliferation Kit (Ribobio) according to the manufacturer’s instructions. DNA was stained by Hoechst 33,342 for 30 min at room temperature. Signals were observed under a fluorescence microscope (Nikon TE2000S) (at least 200 cells per sample).
Apoptosis assay
HeLa cells were seeded on 24-well plates and transfected with siRNAs. Mimosine was applied 6 hrs post-transfection. Cells were then released and harvested at 4-hr intervals for 24 hrs. The Annexin V-Cy3™ Apoptosis Detection Kit (Sigma) was used to examine apoptosis in accordance with the manufacturer’s instructions. Signals were observed under a fluorescence microscope (Nikon TE2000S) (at least 200 cells per sample).
Co-immunoprecipitation assay (co-IP)
HEK 293T cells were seeded in 10-cm dishes and transfected with HA-hNOC3-expressing plasmid or empty vector. Cells were harvested for co-IP assay at 48 hrs post-transfection. Co-IP assay was performed as previously described [Citation39].
Yeast two-hybrid analysis
Yeast two-hybrid analysis was performed as previously described [Citation40–Citation43] with modifications. Transformed cells were first streaked on SCM-Trp-Leu (Synthetic complete medium) and SCM-Trp-Leu-His plates and allowed to grow for 3 days. Single colonies were selected and patched on SCM-Trp-Leu and SCM-Trp-Leu-His plates and allowed to grow for 3 days.
Flow cytometry
The FACSort machine (Beckon Dickinson) was used for flow cytometry analysis as previously described [Citation6,Citation39]. Data analysis was performed using Flowing Software 2.
Immunoblotting
Protein samples were mixed with an equal volume of 2x Laemmli’s buffer, boiled for 5 min, spun down and loaded onto 12.5% SDS-PAGE gels. Resolved proteins were transferred to nitrocellulose membranes and blocked with 5% non-fat milk in TBST (0.1% Tween-20) for 30 min at room temperature, followed by sequential incubation with primary and secondary antibodies. Signals were visualized using SuperSignal West Pico Chemiluminescent Substrate (Thermo Scientific) according to the manufacturer’s instructions. Antibodies and the dilutions used in the analysis are as follows: polyclonal rabbit anti-hNOC3, self-production, 1:300; monoclonal mouse anti-HA, Roche, 12CA5, 1:10,000; monoclonal mouse anti-hCDC6, Santa Cruz, sc-9964, 1:250; polyclonal goat anti-ORC4: Abcam, ab9641, 1:1000; polyclonal rabbit anti-hORC5, LSBio, LS-C178970, 1:500; monoclonal mouse anti-BM28 (hMCM2), BD Transduction Laboratory, 610,701, 1:1000; polyclonal goat anti-hMCM3: Santa Cruz, sc-9850, 1:500; monoclonal mouse anti-hMCM5: Santa Cruz, sc-165,995, 1:500; monoclonal mouse anti-hMCM7: Santa Cruz, sc-9966, 1:500; monoclonal mouse anti-phospho-Histone H3 (serine 10), Millipore, #05–806, 1:2000; monoclonal mouse anti-β actin, Sigma, A2228, 1:2000; and polyclonal rabbit anti-Histone H3, Santa Cruz, sc-11,278, 1:2000.
RNA extraction and reverse transcription real-time PCR (RT-qPCR)
Total RNA was extracted from HeLa cells with TRIzol reagent (Invitrogen), followed by reverse transcription using First Strand cDNA Synthesis Kit (Thermo Scientific), according to the manufacturers’ instructions. Real-time PCR was performed using FastStart Universal SYBR Green Master (Rox) (Roche). β-Actin was used as internal control for normalization. The following primers were used: 18S-F: GCAATTATTCCCCATGAACG; 18S-R: GGGACTTAATCAACGCAAGC; 28S-F: TTACCCTACTGATGATGTGTTGTTG; 28S-R: CCTGCGGTTCCTCTCGTA; 45S-F: TCAGGCGTTCTCGTCTCC; 45S-R: CACCACATCGATCACGAAGA; β-actin-F: TCCTTCCTGGGCATGGAGTCCT; β-actin-R: TGCCAGGGCAGTGATCTCCT.
Statistical analysis
All statistical analyses were done by paired t-test. Results were displayed as average ± standard deviation from three independent experiments.
Results
hNOC3 physically interacts with multiple Pre-RC proteins
In budding yeast, Noc3p interacts with Orc2p, Orc3p, Orc5p, Orc6p, Mcm6p and Cdt1p by yeast two-hybrid analysis and/or co-immunoprecipitation (co-IP) [3, 5, and our unpublished data], and interacts with Orc1p and Mcm2p in yeast whole cell extracts by co-IP [Citation5]. We performed yeast two-hybrid analysis to examine physical interactions of hNOC3 with hORC1-6 and hMCM2-7. We found that hNOC3 interacted with hORC2, hORC4, hORC5, hMCM2, hMCM3 and hMCM7 in both directions of the yeast two-hybrid system (). To confirm the protein interactions detected by yeast two-hybrid analysis, co-IP was used to examine the interactions of hNOC3 with hMCM proteins in human cell extracts. DNase I-treated (to eliminate potential DNA-mediated interactions) whole-cell extracts from HEK-293T cells expressing HA-NOC3 were immunoprecipitated with an anti-HA antibody, and the immunoprecipitates were analyzed by immunoblotting with anti-MCM antibodies. hMCM2, hMCM3 and hMCM7 were found to be co-immunoprecipitated with HA-hNOC3 by the anti-HA antibody, while hMCM5, which did not interact with hNOC5 in the yeast two-hybrid assay and served as a negative control in co-IP, was not co-immunoprecipitated. Moreover, reciprocal co-IP using anti-hMCM3 antibody also successfully co-precipitated hNOC3 from HEK-293T cell extracts with roughly two to three-fold overexpression of HA-hNOC3 (,). Collectively, these data show that hNOC3 interacts with several hORC and hMCM components, suggesting that it plays a role in the establishment of pre-RC in human cells.
Figure 1. hNOC3 interacts with hORC and hMCM subunits in yeast two-hybrid assays. The AH109 host yeast cells were co-transformed with the indicated combinations of plasmids, and the transformants were patched onto SCM-Trp-Leu (SCM-2) and SCM-Trp-Leu-His (SCM-3) plates and incubated to test cell growth. Cells containing AD-T (SV40 large T-antigen) and BD-p53 were used as the positive interaction control. Cells containing the two empty AD and BD vectors, AD-hNOC3+ BD vector, and AD vector+BD-hNOC3 were patched on the top three spots of the plates (labelled as Negative controls). Other negative controls also included individual AD-ORC/MCM or BD-ORC/MCM plasmids together with the opposite empty vectors.
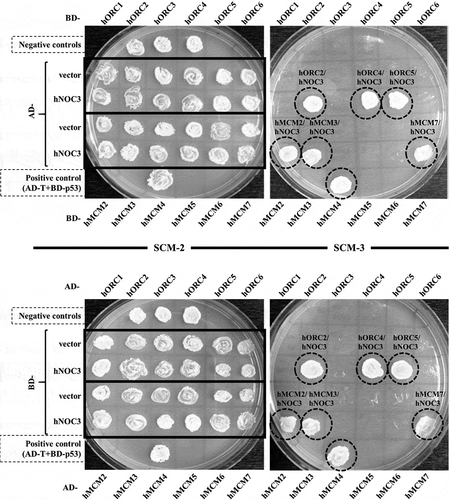
Figure 2. hNOC3 interacts with hMCM subunits in co-IP. HEK-293T cells were transfected with HA-hNOC3 plasmid and harvested for immunoprecipitation after 48 hrs post-transfection. (a) DNase I-treated whole-cell extracts (WCE) were immunoprecipitated with an anti-HA antibody or the control mouse IgG. Co-IP assay results show that hNOC3 interacts with hMCM2, hMCM3 and hMCM7, but not hMCM5. *: Cross-reactive band. (b) Reciprocal co-IP was done using hMCM3 antibody and hNOC3 was co-immunoprecipitated.
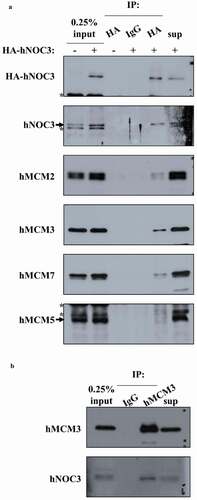
hNOC3 binds chromatin throughout the cell cycle and is associated with known replication origins
Previous studies suggest that hNOC3 localizes in the nucleus, particularly at nuclear speckles, where pre-mRNA splicing and maturation take place [Citation34]. hNOC3 has also been shown to recruit Histone Acetyltransferase HBO1 onto chromatin for the stimulation of adipogenesis [Citation44]. To build upon the existing knowledge regarding hNOC3 and examine its potential role in DNA replication initiation, as has been shown in yeast, we examined the chromatin association pattern of hNOC3 in the cell cycle. HeLa cells were blocked in M-phase by nocodazole followed by mitotic shake-off to obtain mitotic cells, which were then released into the cell cycle. Cells were then harvested at different time points after release to obtain cells at different cell cycle phases. Cell cycle progression was examined by flow cytometry, and chromatin-binding assay was used to determine the chromatin association pattern of hNOC3 and other pre-RC proteins (). The results show that hNOC3 remained bound to the chromatin throughout the cell cycle, although with some observable variations in the protein level. Moreover, ChIP assay results show that HA-hNOC3 preferentially associated with several well-known replication origins including those at the c-myc, lamin B2 and β-globin loci, but not associated with the non-origin sequences that are several kb away from these origins (). These data suggest that hNOC3 binds replication origins and hence it likely plays a role in DNA replication initiation.
Figure 3. hNOC3 binds to chromatin, preferentially at replication origins, throughout the cell cycle. (a) HeLa cells were synchronized in M-phase by nocodazole followed by mitotic shake-off to obtain mitotic cells, which were then released onto the cell cycle. Cells were then collected at different time points as indicated and analyzed by flow cytometry and chromatin-binding assay to examine the cell cycle progression and chromatin association of hNOC3 and other pre-RC proteins. (b) HEK-293T cells were transfected with HA-hNOC3 plasmid and harvested for ChIP assay after 48 hrs post-transfection. DNA from the immunoprecipitate was analyzed by qPCR using primer sets to quantify the replication origin and non-origin control sequences. α-HA, IP by α-HA antibody; IgG, mock IP by non-specific mouse IgG; UT, IP from untagged hNOC3 control extracts. (**: p < 0.01; ***: p < 0.001.
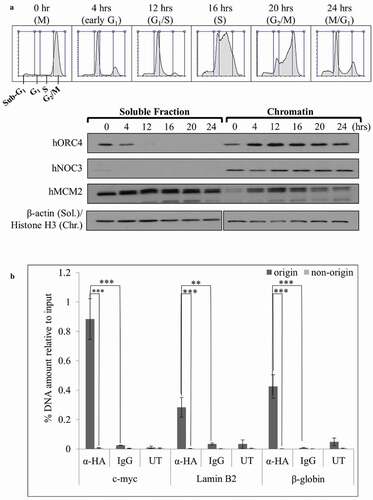
hNOC3 is essential for Pre-RC assembly
To examine the role and mechanisms of hNOC3 in DNA replication initiation, chromatin-binding assay was carried out using G1 cell extracts from hNOC3 knockdown cells. The G1 synchrony was confirmed by flow cytometry (). Our data suggest that depletion of hNOC3 with a siRNA targeted to hNOC3 (sihNOC3#1) did not affect the chromatin association of hORC (represented by hORC5; ). However, the chromatin association of hCDC6 and hMCM (represented by hMCM2) was abrogated ()). Moreover, sihNOC3#2, which has a lower knockdown efficiency than sihNOC3#1, also disrupted the chromatin association of hMCM5, albeit to a lesser extent (). These data suggest that the chromatin association of hORC is independent of hNOC3, while hNOC3 is essential for loading hCDC6 and the hMCM complex onto chromatin and hence pre-RC assembly. To exclude the possibility that the abrogation of pre-RC assembly was due to hNOC3’s potential function in ribosome biogenesis and hence protein synthesis, we determined if specific inhibition of ribosome biogenesis by using the RNA Pol I inhibitor CX-5461 or knocking down the RNA Pol I subunit RPA194 would lead to pre-RC defect phenotypes. The results show that these treatments did not affect the chromatin association of hMCM proteins (). Therefore, our data support the notion that hNOC3’s role in DNA replication initiation is independent of its potential role in ribosome biogenesis.
Figure 4. Pre-RC assembly is abrogated by hNOC3 silencing but not by specific inhibition of the ribosome biogenesis pathway. (a) Pre-RC assembly was abrogated after depletion of hNOC3. HeLa cells were transfected with hNOC3 siRNAs, synchronized in G1-phase by mimosine, and then harvested for chromatin-binding assay. The result for flow cytometry analysis was included showing most of the cells were synchronized in G1-phase. LF, the transfection agent Lipofectamine RNAiMax; siNC, negative control siRNA. (b) Pre-RC assembly was abrogated after depletion of hNOC3 but not by specific inhibition of the ribosome biogenesis pathway. HeLa cells were treated with siRNA targeting hNOC3 or the RNA Pol I subunit RPA194, or treated with the RNA Pol I inhibitor CX-5461. The cells were then synchronized in G1-phase by mimosine and harvested for chromatin-binding assay.
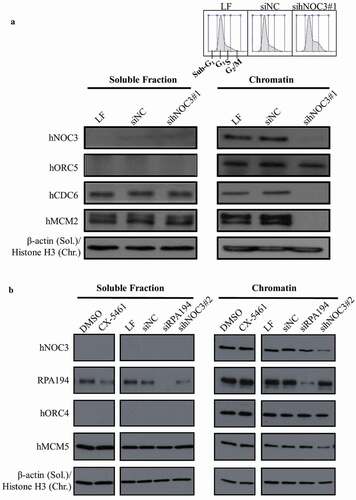
hNOC3 is required for DNA replication initiation
After determining that hNOC3 is required for pre-RC assembly, we further investigated the effects of silencing hNOC3 on DNA replication by EdU incorporation assay. Cells were released from a G1-phase block and then pulse labeled with EdU in early S-phase. Cells were then fixed and stained for EdU and DNA. The results indicate that the percentage of EdU positive cells was significantly reduced after hNOC3 silencing, as approximately 50% of the control cells (LF and siNC) were EdU positive, while only 23% and 27%, respectively, of the cells treated with the two hNOC3 siRNAs were EdU positive (). Furthermore, flow cytometry results indicate that most of the control cells had entered S-phase at the time (1 hr after release) when the cells were harvested for the EdU assay, while the knockdown cells mostly stayed in G1-phase (). These data suggest that cells were defective in the initiation of DNA replication after hNOC3 silencing. For comparison, EdU incorporation assay was performed following specific inhibition of ribosome biogenesis as described above in . The results show that these treatments did not significantly affect EdU incorporation ().
Figure 5. DNA replication is defective after hNOC3 knockdown but not after ribosome biogenesis inhibition. HeLa cells were transfected or treated as indicated and synchronized in G1-phase by mimosine. Cells were released into early S-phase for EdU-incorporation assay. (a) Percentage of EdU-positive cells was reduced after hNOC3 knockdown by either of the two hNOC siRNAs. Flow cytometry result shows that control cells entered S-phase after release while knockdown cells were defective in S-phase entry. LF, Lipofectamine RNAiMax; siNC, negative control siRNA. (b) DNA replication was not affected after specific inhibition of the ribosome biogenesis pathway by siRNA targeting the RNA Pol I subunit RPA194, or by the RNA Pol I inhibitor CX-5461. At least 200 cells/sample were analyzed. (*: p < 0.05).
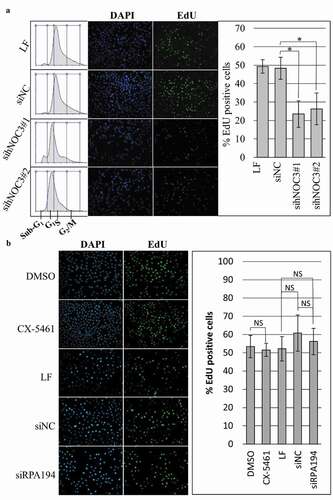
Silencing of hNOC3 induces apoptosis before S-phase completion
Inhibiting DNA replication initiation could lead to other cellular phenotypes such as apoptosis [Citation45]. We examined the effects of hNOC3 knockdown at different time points after the cells were released from G1-phase. Early apoptotic cells were detected using Annexin-V and 6-CFDA staining assay, and immunoblotting and flow cytometry were carried out to monitor cell cycle progress and to detect late apoptotic cells (by the presence of sub-G1 cells in flow cytometry) ( and ). The results of the Annexin-V apoptosis assay show that the hNOC3 knockdown cells underwent apoptosis after release from mimosine (). The percentage of Annexin-Cy3 positive cells increased from ~15% at 4 hrs post-release to 40–50% at 16 hrs post-release, while no more than 8% of the control cells were Annexin-Cy3 positive throughout the experiment (). Flow cytometry results show that most of the knockdown cells were unable to progress through S-phase, and they underwent apoptosis in S-phase, as apparent by the presence of the massive sub-G1 population starting from 4 hrs post-release and the lack of S-phase and G2/M-phase cells at all of the time points, while control cells went through the cell cycle normally without significant apoptosis after release ().
Figure 6. Knockdown of hNOC3 leads to severe apoptosis before S-phase completion HeLa cells were transfected with siRNAs as indicated and synchronized at G1-phase by mimosine. Cells were released and harvested at different time points as indicated. To prevent exit from mitosis, nocodazole was added to a population of the cells at 12 hrs post-release. (a) Results of the Annexin-V apoptosis assay after hNOC3 knockdown. Annexin-Cy3 stains apoptotic cells with disrupted cell membrane, and 6-CFDA stains live cells including early apoptotic cells (early apoptotic cells are positive for both signals). (b) Quantification of the early apoptotic cells in the Annexin-V apoptosis assay in (a). At least 200 cells per sample were examined. (*: p < 0.05; **: p < 0.01; ***: p < 0.001, compared to respective time point of the siNC group) (c) Immunoblotting was done to determine if the cells entered M-phase indicated by the phospho-Histone H3 (serine 10) signal.
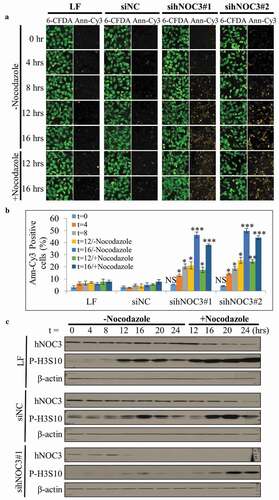
Figure 7. The knockdown of hNOC3, but not specific inhibition of ribosome biogenesis, inhibits S-phase entry and leads to severe apoptosis. HeLa cells were transfected with siRNAs or treated as indicated, and synchronized at G1-phase by mimosine. Cells were released and time points were harvested for flow cytometry analysis. (a) Flow cytometry was performed to examine cell cycle progress and detect apoptotic cells. (b) Flow cytometry result in (a) was confirmed with sihNOC3#2 with a set of simplified time points and compared to that after specific inhibition of the ribosome biogenesis pathway. LF, Lipofectamine RNAiMax; siNC, negative control siRNA.
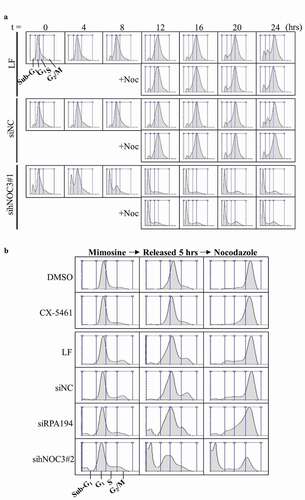
Since apoptosis occurred in hNOC3 knockdown cells mostly during 4 to 8 hrs post-release when the control cells were progressing through S-phase (), we reasoned that the hNOC3 knockdown cells underwent apoptosis as they went into an abortive S-phase, rather than in M-phase. To confirm this, we performed immunoblotting to detect phospho-Histone H3 (serine 10), which is present only in M-phase cells, to demonstrate that hNOC3 knockdown cells failed to complete S-phase and thus were not able to enter M-phase after mimosine release. The results indicate that the control cells entered M-phase between 12 to 16 hrs post-release, while most hNOC3 knockdown cells did not enter M-phase, as indicated by the very low signal of phospho-Histone H3 (serine 10) (). Similar results were obtained in the presence or absence of nocodazole that was added to a population of the cells at 12 hrs post-release to prevent potential mitosis exit so that we would not miss detecting mitotic cells (). Together, these data strongly suggest that hNOC3 knockdown cells were unable to complete S-phase and subsequently underwent apoptosis during the abortive S-phase.
To exclude the possibility that the replication defects after hNOC3 knockdown resulted from defective ribosome biogenesis, we examined cell cycle progression after specific inhibiting ribosome biogenesis by other means than hNOC3 knockdown (). HeLa cells were treated with the RNA Pol I inhibitor CX5461, or transfected with siRNAs targeting the RNA Pol I subunit RPA194 or hNOC3. Flow cytometry results show that, unlike hNOC3 knockdown, inhibition of ribosome biogenesis by either RPA194 silencing or CX5461 treatment did not lead to defects in S-phase entry or progress, or apoptosis (). We confirmed by RT-qPCR that RPA194 knockdown or the CX-5461 treatment actually resulted in significant reduction (70–90%) in the 45S precursor rRNA level and also a reduction (60–70%) in the mature 18S and 28S rRNA levels in a comparable timeframe of the hNOC3 knockdown experiments (), indicating that ribosome biogenesis was indeed defective after RPA194 knockdown or the CX-5461 treatment. This is consistent with the notion that DNA replication can occur normally within a single S-phase even if new ribosomes are not generated, as ribosomes are relatively stable. Therefore, we can conclude that the replication defects and subsequent apoptosis in hNOC3 knockdown cells resulted specifically from replication initiation defects, rather than from any possible defects in ribosome biogenesis. Interestingly, knockdown of hNOC3 did not lead to a significant change in the rRNA levels compared to the negative control (), suggesting that hNOC3 is not involved in rRNA transcription or processing. However, the potential role of hNOC3 in rRNA intracellular transport (as in budding yeast) has yet to be determined. Together, these data strongly suggest that hNOC3 regulates the initiation of DNA replication independent of its potential role in ribosome biogenesis.
Figure 8. Specific inhibition of Pre-rRNA synthesis results in significant reduction in the 45S precursor rRNA level and the mature 18S and 28S rRNA levels. HeLa cells were treated with siRNAs against hNOC3 or the RNA Pol I subunit RPA194, or treated with the RNA Pol I inhibitor CX5461. Total RNA was isolated and quantified by RT-qPCR using primer sets for 18S, 28S and 45S rRNAs. Cells were harvested at comparable time window as in and . (*: p < 0.05; **: p < 0.01, compared to the DMSO control. #: p < 0.05, compared to the siNC control.).
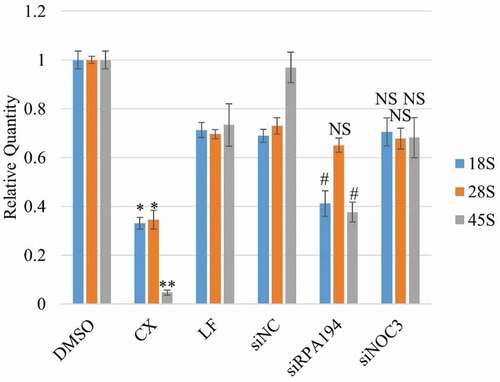
Discussion
Noc3p has previously been reported as an essential protein in pre-RC assembly and the intracellular transport and maturation of ribosomes in budding yeast. FAD24 (hNOC3) was identified as the homolog of Noc3p involved in adipogenesis, cell cycle re-entry, DNA replication, cell proliferation and hyperplasia in human cells [Citation27,Citation34–Citation37]. In this study, we report that hNOC3 is also an essential pre-RC protein, independent of its potential role in ribosome biogenesis, in human cells.
To regulate DNA replication initiation, a protein must directly or indirectly associate with replication origins on the chromosomes and other DNA replication-initiation proteins. Here, we report that hNOC3 binds chromatin throughout the cell cycle and preferentially associates with several known replication origins [Citation46–Citation48]. We have also demonstrated the interactions of hNOC3 with several pre-RC proteins including hORC and hMCM subunits. The interactions of hNOC3 with diverse members of pre-RC proteins as well as replication origins strongly suggest a role for hNOC3 as an essential factor linking ORC with other replication initiation proteins. Indeed, the chromatin association of hCDC6 and hMCM proteins (but not hORC proteins) was abrogated after hNOC3 silencing, suggesting that hNOC3 is required for the recruitment of hCDC6 and hMCM to replication origins, while the origin recognition and binding of hORC is independent of hNOC3. Importantly, DNA replication initiation and cell cycle progression were disrupted, followed by massive apoptosis in S-phase after hNOC3 silencing, while specific inhibition of the ribosome biogenesis pathway by other means than hNOC3 knockdown did not produce any observable effect in DNA replication or apoptosis at comparable time points as the hNOC3 knockdown experiments These results can be easily understood, as protein synthesis can continue for quite a long time using the pre-existing ribosomes after ribosome biogenesis is inhibited, as ribosomes are known to be stable for up to several days in vivo [Citation49,Citation50]. These results indicate that the replication phenotypes of the hNOC3 knockdown cells was unlikely to be secondary to any possible ribosome biogenesis defects.
Moreover, the fact that most of the results were similar with two different siRNAs targeting hNOC3 strongly suggests that the phenotypes after hNOC3 silencing were unlikely to result from off-target effects. Collectively, our data strongly suggest that hNOC3 plays a crucial role in DNA replication initiation in human cells by associating with hORC at replication origins throughout the cell cycle and serves as an essential bridge between hORC and other licensing factors such as hCDC6 and hMCM for pre-RC assembly during the M-to-G1 transition. Consistent with its role in replication initiation, hNOC3 has also been reported to interact with HBO1 histone acetylase [Citation44]. Through interacting with HBO1, hNOC3 stimulates the histone acetylation at replication origins and further facilitates the loading of the HBO1-hCDT1-hMCM complex [Citation51–Citation53].
In addition to the previous reports of Noc3p’s role in DNA replication initiation in budding yeast [Citation3,Citation5] and of hNOC3’s role in human DNA replication [Citation51–Citation53], there were published experimental data indicating that Noc3 in fission yeast also plays a role in DNA replication, as depletion, which was inevitably incomplete in genetic experiments, of Noc3 in fission yeast significantly slowed down S-phase entry and progression, and finally led to a block in G2/M phase, most probably due to incomplete genome duplication, which is reminiscent of the phenotypes in ORC or MCM depleted cells (reviewed in [Citation54]).
Whether or not hNOC3 also has a role in ribosome biogenesis remains elusive. By examining pre-ribosome trafficking and/or the ribosome and polysome profiles following hNOC3 silencing may answer this question. There is a growing possibility that ribosome biogenesis and DNA replication, two very fundamental cellular processes, are linked together. Previously, hIPI3 and hCDC6, as well as Noc3p and Ipi3p in budding yeast, were found to play roles in both DNA replication and ribosome biogenesis [Citation6,Citation38,Citation55]. Similarly, hNOC3 may also be involved in the intracellular transport of the ribosome subunits, like yeast Noc3p. This presents an opportunity for further studying the coordination between DNA replication and ribosome biogenesis.
Acknowledgments
We gratefully acknowledge Kelvin K.L. Sou for providing valuable technical assistance. This work was supported by grants from the Hong Kong Research Grants Council (GRF 661713), Shenzhen Dept. of Science and Information (JCYJ20130329110752138), Guangzhou Science, Technology and Information Commission (P1515), Foshan Science and Technology Bureau, Guangdong, China (2015IT100132), and Center for Nasopharyngeal Carcinoma Research, Hong Kong (AoE/M-06/08).
Disclosure statement
No potential conflict of interest was reported by the authors.
Additional information
Funding
References
- Dutta A, Bell SP. Initiation of DNA replication in eukaryotic cells. Annu Rev Cell Dev Biol. 1997;13:293–332.
- Méndez J, Stillman B. Chromatin association of human origin recognition complex, cdc6, and minichromosome maintenance proteins during the cell cycle: assembly of prereplication complexes in late mitosis. Mol Cell Biol. 2000 Nov;20(22):8602–8612.
- Huo L, Wu R, Yu Z, et al. The Rix1 (Ipi1p-2p-3p) complex is a critical determinant of DNA replication licensing independent of their roles in ribosome biogenesis. Cell Cycle. 2012;11:1325–1339.
- Tanaka T, Knapp D, Nasmyth K. Loading of an Mcm protein onto DNA replication origins is regulated by Cdc6p and CDKs. Cell. 1997 Aug 22;90(4):649–660.
- Zhang Y, Yu Z, Fu X, et al. Noc3p, a bHLH protein, plays an integral role in the initiation of DNA replication in budding yeast. Cell. 2002. 28. 109(7):849–860.
- Huang Y, Amin A, Qin Y, et al. A role of hIPI3 in DNA replication licensing in human cells. PloS One. 2016;11(4):e0151803.
- Bell SP, Kaguni JM. Helicase loading at chromosomal origins of replication. Cold Spring Harb Perspect Biol. 2013 Jan 6;5(6):a010124.
- Cocker JH, Piatti S, Santocanale C, et al. An essential role for the Cdc6 protein in forming the pre-replicative complexes of budding yeast. Nature. 1996 Jan 11;379(6561):180–182.
- Donovan S, Harwood J, Drury LS, et al. Cdc6p-dependent loading of Mcm proteins onto pre-replicative chromatin in budding yeast. Proc Natl Acad Sci U S A. 1997 May 27;94(11):5611–5616.
- Hizume K, Kominami H, Kobayashi K, et al. Flexible DNA path in the MCM double hexamer loaded on DNA. Biochemistry. 2017 May 16;56(19):2435–2445.
- Li N, Zhai Y, Zhang Y, et al. Structure of the eukaryotic MCM complex at 3.8 Å. Nature. 2015 Aug 13;524(7564):186–191.
- Liu C, Wu R, Zhou B, et al. Structural insights into the Cdt1-mediated MCM2-7 chromatin loading. Nucleic Acids Res. 2012 Apr;40(7):3208–3217.
- Quan Y, Xia Y, Liu L, et al. Cell-cycle-regulated interaction between Mcm10 and double hexameric Mcm2-7 is required for helicase splitting and activation during S phase. Cell Rep. 2015 Dec 22;13(11):2576–2586.
- Tanaka S, Diffley JFX. Interdependent nuclear accumulation of budding yeast Cdt1 and Mcm2-7 during G1 phase. Nat Cell Biol. 2002 Mar;4(3):198–207.
- Yuan Z, Bai L, Sun J, et al. Structure of the eukaryotic replicative CMG helicase suggests a pumpjack motion for translocation. Nat Struct Mol Biol. 2016 Mar;23(3):217–224.
- Zhai Y, Cheng E, Wu H, et al. Open-ringed structure of the Cdt1-Mcm2-7 complex as a precursor of the MCM double hexamer. Nat Struct Mol Biol. 2017 Mar;24(3):300–308.
- Miyazawa‐Onami M, Araki H, Tanaka S. Pre‐initiation complex assembly functions as a molecular switch that splits the Mcm2‐7 double hexamer. EMBO Rep. 2017 Aug 17;18:1752–1761.
- Shultz RW, Tatineni VM, Hanley-Bowdoin L, et al. Genome-wide analysis of the core DNA replication machinery in the higher plants arabidopsis and rice. Plant Physiol. 2007 Aug;144(4):1697–1714.
- Tanaka S, Araki H. Helicase activation and establishment of replication forks at chromosomal origins of replication. Cold Spring Harb Perspect Biol. 2013 Jan 12;5(12):a010371.
- Drury LS, Perkins G, Diffley JF. The Cdc4/34/53 pathway targets Cdc6p for proteolysis in budding yeast. Embo J. 1997 Oct 1;16(19):5966–5976.
- Johansson P, Jeffery J, Al-Ejeh F, et al. SCF-FBXO31 E3 ligase targets DNA replication factor Cdt1 for proteolysis in the G2 phase of cell cycle to prevent re-replication. J Biol Chem. 2014 Jun 27;289(26):18514–18525.
- Moritani M, Ishimi Y. Inhibition of DNA binding of MCM2-7 complex by phosphorylation with cyclin-dependent kinases. J Biochem (Tokyo). 2013 Oct;154(4):363–372.
- Nguyen VQ, Co C, Li JJ. Cyclin-dependent kinases prevent DNA re-replication through multiple mechanisms. Nature. 2001. 28. 411(6841):1068–1073.
- Nishitani H, Taraviras S, Lygerou Z, et al. The human licensing factor for DNA replication Cdt1 accumulates in G1 and is destabilized after initiation of S-phase. J Biol Chem. 2001 Nov 30;276(48):44905–44911.
- Wohlschlegel JA, Dwyer BT, Dhar SK, et al. Inhibition of eukaryotic DNA replication by geminin binding to Cdt1. Science. 2000 Dec 22;290(5500):2309–2312.
- Zhai Y, Yung PYK, Huo L, et al. Cdc14p resets the competency of replication licensing by dephosphorylating multiple initiation proteins during mitotic exit in budding yeast. J Cell Sci. 2010 Nov 15;123(Pt 22):3933–3943.
- Johmura Y, Osada S, Nishizuka M, et al. FAD24, a regulator of adipogenesis, is required for the regulation of DNA replication in cell proliferation. Biol Pharm Bull. 2008 Jun;31(6):1092–1095.
- Maiorano D, Moreau J, Méchali M. XCDT1 is required for the assembly of pre-replicative complexes in Xenopus laevis. Nature. 2000 Apr 6;404(6778):622–625.
- Nishitani H, Lygerou Z, Nishimoto T, et al. The Cdt1 protein is required to license DNA for replication in fission yeast. Nature. 2000 Apr 6;404(6778):625–628.
- Whittaker AJ, Royzman I, Orr-Weaver TL. Drosophila double parked: a conserved, essential replication protein that colocalizes with the origin recognition complex and links DNA replication with mitosis and the down-regulation of S phase transcripts. Genes Dev. 2000 Jul 15;14(14):1765–1776.
- Bassler J, Kallas M, Hurt E. The NUG1 GTPase reveals and N-terminal RNA-binding domain that is essential for association with 60 S pre-ribosomal particles. J Biol Chem. 2006 Aug 25;281(34):24737–24744.
- Dlakić M, Tollervey D. The Noc proteins involved in ribosome synthesis and export contain divergent HEAT repeats. Rna N Y N. 2004 Mar;10(3):351–354.
- Milkereit P, Gadal O, Podtelejnikov A, Trumtel S, Gas N, Petfalski E, et al. Maturation and intranuclear transport of pre-ribosomes requires Noc proteins. Cell. 2001 May 18;105(4):499–509.
- Tominaga K, Johmura Y, Nishizuka M, et al. Fad24, a mammalian homolog of Noc3p, is a positive regulator in adipocyte differentiation. J Cell Sci. 2004 Dec 1;117(Pt 25):6217–6226.
- Johmura Y, Watanabe K, Kishimoto K, et al. Fad24 causes hyperplasia in adipose tissue and improves glucose metabolism. Biol Pharm Bull. 2009 Oct;32(10):1656–1664.
- Lei T, Bi Y, Gao MJ, et al. HES1 inhibits adipogenesis of porcine mesenchymal stem cells via transcriptional repression of FAD24. Domest Anim Endocrinol. 2013 Jul;45(1):28–32.
- Ochiai N, Nishizuka M, Osada S, et al. Fad24, a positive regulator of adipogenesis, is required for S phase re-entry of C2C12 myoblasts arrested in G0 PHASE AND INVOLVED in p27(Kip1) expression at the protein level. Biol Pharm Bull. 2016 May 1;39(5):807–814.
- Huang S, Xu X, Wang G, et al. DNA replication initiator Cdc6 also regulates ribosomal DNA transcription initiation. J Cell Sci. [Internet]. 2016. [cited 2016 Jul 18]. Available from: http://jcs.biologists.org/content/129/7/1429.long
- Zhang J, Yu L, Wu X, et al. The interacting domains of hCdt1 and hMcm6 involved in the chromatin loading of the MCM complex in human cells. Cell Cycle Georget Tex. 2010 Dec 15;9(24):4848–4857.
- Kan J, Zou L, Zhang J, et al. Origin recognition complex (ORC) mediates histone 3 lysine 4 methylation through cooperation with Spp1 in Saccharomyces cerevisiae. J Biol Chem. 2008 Dec 5;283(49):33803–33807.
- Lai F, Wu R, Wang J, et al. Far3p domains involved in the interactions of Far proteins and pheromone-induced cell cycle arrest in budding yeast. FEMS Yeast Res. 2011 Feb;11(1):72–79.
- Wang J, Wu R, Lu Y, et al. Ctf4p facilitates Mcm10p to promote DNA replication in budding yeast. Biochem Biophys Res Commun. 2010 May 7;395(3):336–341.
- Wu R, Wang J, Liang C. Cdt1p, through its interaction with Mcm6p, is required for the formation, nuclear accumulation and chromatin loading of the MCM complex. J Cell Sci. 2012 Jan 1;125(Pt 1):209–219.
- Johmura Y, Osada S, Nishizuka M, et al. FAD24 acts in concert with histone acetyltransferase HBO1 to promote adipogenesis by controlling DNA replication. J Biol Chem. 2008 Jan 25;283(4):2265–2274.
- Feng D, Tu Z, Wu W, et al. Inhibiting the expression of DNA replication-initiation proteins induces apoptosis in human cancer cells. Cancer Res. 2003 Nov 1;63(21):7356–7364.
- Abdurashidova G, Deganuto M, Klima R, et al. Start sites of bidirectional DNA synthesis at the human lamin B2 origin. Science. 2000 Mar 17;287(5460):2023–2026.
- Berberich S, Trivedi A, Daniel DC, et al. In vitro replication of plasmids containing human c-myc DNA. J Mol Biol. 1995 Jan 13;245(2):92–109.
- Cimbora DM, Schübeler D, Reik A, et al. Long-distance control of origin choice and replication timing in the human beta-globin locus are independent of the locus control region. Mol Cell Biol. 2000 Aug;20(15):5581–5591.
- Hirsch CA, Hiatt HH. Turnover of liver ribosomes in fed and in fasted rats. J Biol Chem. 1966 Dec 25;241(24):5936–5940.
- Nikolov EN, Dabeva MD, Nikolov TK. Turnover of ribosomes in regenerating rat liver. Int J Biochem. 1983;15(10):1255–1260.
- Feng Y, Vlassis A, Roques C, et al. BRPF3-HBO1 regulates replication origin activation and histone H3K14 acetylation. Embo J. 2016 Jan 18;35(2):176–192.
- Miotto B, Struhl K. HBO1 histone acetylase activity is essential for DNA replication licensing and inhibited by geminin. Mol Cell. 2010 Jan 15;37(1):57.
- Miotto B, Struhl K. HBO1 histone acetylase is a coactivator of the replication licensing factor Cdt1. Genes Dev. 2008 Oct 1;22(19):2633–2638.
- Zhai Y, Yung PYK, Liang C. Cell cycle control of DNA replication by phosphorylation and dephosphorylation of replication-initiation proteins in budding yeast. In: Kušić-Tišma J, editor. Fundamental aspects of DNA replication. Croatia: InTech; 2011. p. 87–106.
- Castle CD, Cassimere EK, Denicourt C. LAS1L interacts with the mammalian Rix1 complex to regulate ribosome biogenesis. Mol Biol Cell. 2012 Feb;23(4):716–728.
- Castle CD, Cassimere EK, Denicourt C. LAS1L interacts with the mammalian Rix1 complex to regulate ribosome biogenesis. Mol Biol Cell. 2012 Feb;23(4):716–728.