ABSTRACT
Researches have been focusing on the role of Slit2 in angiogenesis, specifically in cell migration and vessel permeability. Nevertheless, the role of Slit2-N, the bioactive fragment of Slit2, in the proliferation of vascular endothelia in choroidal neovascularization and some related mechanisms have not been studied yet. Thus, our study aimed to explore the role of Slit2-N in proliferation of vascular endothelia and the related mechanisms in choroidal neovascularization. Fluorescein isothiocyanate perfusion and HE staining were performed to evaluate volumes of choroidal neovascularization lesions. The effect of Slit2-N on VEGF165-induced cell proliferation and some related mechanisms were detected by CCK8 assay, flow cytometry, siRNA transfection, and western blotting. We found that Slit2-N reduced volumes of laser-induced choroidal neovascularization networks in vivo. Results of the in vitro study showed Slit2-N reduced VEGF165-induced cell proliferation of both human umbilical vascular endothelial cells and human microvascular endothelial cells possibly via activation of AKT rather than that of ERK1/2. Additionally, Robo4, one of the receptors binding to Slit2-N, was involved in the inhibitory effect of Slit2-N. Generally, our findings revealed the inhibitory role of Slit2-N in proliferation of vascular endothelia and some related mechanisms, and presented some potential targets, molecules along Slit2-N-Robo4-AKT axis, to choroidal neovascularization therapy.
1. Introduction
Age-related macular disease, whose severe pathological outcome is choroidal neovascularization (CNV), is the leading cause of blindness among people over 50 years in the industrial world. Vascular endothelial growth factor (VEGF) plays an essential role in the onset and progression of CNV [Citation1,Citation2]. Thus, therapies of local neutralization of VEGF-A, such as intravitreal injection of Lucentis and Conbercept, have gained success in treating CNV. Even so, the underlying mechanisms of CNV have not been completely understood, and some deficiencies in anti-VEGF treatments remain, for example, some CNV cases fail to respond to the anti-VEGF treatments. In this situation, researches on exploring mechanisms of CNV progression and on finding new treating targets are required.
Slit2, a well-known axon repulsive cue, was first identified in the central nervous system of drosophila melanogaster. In recent years, it has been demonstrated that Slit2 and its receptors, Robo1-4, also play an important role in angiogenesis. However, there are very few studies on the role of Slit2 in proliferation of vessel-associated cells though the impact of Slit2 on cells’ migration, and vascular permeability in a variety of diseases has been well studied. Slit2 inhibited endothelial cells’ migration in the human placental environment [Citation3]. It remarkably reduced PDGF-induced migration of mural cells like pericites and vascular smooth muscle cells by rearranging the cytoskeleton and maintained the stability of blood vessels [Citation4,Citation5]. Besides, in surgical brain injury (SBI), Slit2 inhibited peripheral immune cells’ infiltration and played an anti-inflammation role. Regarded as an ECM gene, Slit2 was upregulated in the specific tissue of Renal Cell Carcinoma (RCC) and was considered to promote RCC metastasis. Hopefully, Slit2 may present a new target of treating metastatic RCC [Citation6]. In breast cancer, Slit2-Robo1 signaling activated Rac and increased focal adhesion kinase activity to enhance cell tension. Thus, Slit2-Robo1 signaling exerted an anti-metastasis function and promoted better survival prognosis of breast cancer [Citation7]. In terms of vascular permeability, Slit2 effectively reduced vessel permeability in a lung cancer condition [Citation8]. In SBI, administration of recombinant Slit2 significantly restored SBI-induced blood-brain barrier (BBB) permeability by stabilizing BBB tight junction through Robo4-paxillin-dependent Rac1 pathway [Citation9].
In general, concerns have been classically centered around the effect of Slit2 on angiogenesis, specifically on the migration of vessel-associated cells and on vascular permeability. However, there are rare studies on the function of Slit2-N, the bioactive fragment of Slit2, which binds to Robo receptors, in proliferation of vascular endothelia in CNV models. Herein, the present research was aimed to explore the role of Slit2-N in proliferation of vascular endothelia in CNV, hoping to find some underlying mechanisms of CNV and present some clues on finding new treating target to CNV.
2. Materials and methods
2.1 Ethics statements
All animal experiments in this study were performed in adherence with the ARVO statements for the Use of Animals in Ophthalmology and Vision Research and Experimental Animal Center of Chongqing Medical University (Chongqing, China).
2.2 Rats
Ninety-six Brown Norway rats (BN rats), specific-pathogen-free male, with a weight of 180 g to 220 g, were purchased from laboratory animal center, Chongqing Medical University, Chongqing, China.
2.3 Laser-induced CNV rat models
CNV rat models were constructed by krypton-argon laser (Novus Varia Dpss, Lumenis, USA) photocoagulation (532 nm, 0.1 s, 100 μm, 150 mW). Photocoagulation ended till a white bubble appeared under the retina of a BN rat. Each lesion was made 2 papillary diameter (PD) away from the optic disc on one eye of each rat. Totally, five lesions were made in each eye.
2.4 Subretinal injection
Rats were anesthetized with 3% pentobarbital via intraperitoneal injection, and their pupils were dilated with the use of 0.5% of tropicamide. The eyelid was opened, and a tunnel, which began at a point that was 2 mm away from corneal limbus, was made by a 30-Gauge beveled-needle (7803–07, Hamilton, Switzerland). A small cover slide was used to press slightly on the cornea of the rat for a better vision of the operator. A small hole was punctured slightly posterior to the limbus. Injection of 50 ng Slit2-N or 5 μl vehicle was done through the hole. Injection ended when a small round heaval appeared under the retina.
2.5 Fluorescein isothiocyanate (FITC) perfusion, preparations of choroid-sclera flat mounts and measurement of CNV areas
Rats were anesthetized and then perfused with 1 ml of 50 mg/ml FITC-labeled dextran (FD2000S, sigma, the USA) dissolved in phosphate buffer solution (PBS, PH 7.3) through their left ventricles. When the conjunctiva, lips and limbs skins appeared slightly yellow, it indicated that the perfusion was successfully performed.
Rats were sacrificed after the perfusion. The eyeballs of the rats were taken out, fixed in 10% formaldehyde solution for 1 h and then were washed slightly by PBS. The corneas, lens, and retina were removed from the eyeball, while the sclera, choroid, and the RPE layer remained for preparation of flat mounts. Five or six incisions were made radically on the flat-mount, and the flat-mount was finally covered by a small slide.
CNV lesions were outlined and the areas of the CNV lesions were measured by Image J.
2.6 HE staining and measurement of the relative thickness of CNV
The rats were sacrificed. Their eyeballs were fixed in 4% of formaldehyde solution for 24 h. Then, they were washed softly by current water for 30 s. After that, they were dehydrated by graded ethanol for 20 min and then were vitrified by dimethylbenzene for 20 min and were embedded in paraffin wax. Five μm-thickness slices were continuously made by being cut through the CNV lesions. The slices were stained with hematoxylin-eosin, and ultimately were observed and photographed under a microscope. The sections with the highest value of (T-C)/C were chosen. The relative thickness of CNV lesions was calculated by the equation, RT = T-C/C (T refers to the thickness from the bottom of the choroid to the top of the vessel networks, and C refers to the thickness of the normal choroid near the lesion).
2.7 Cell culture
Human umbilical vein endothelial cell (HUVEC) and human microvascular endothelial cells (HMVEC) were separately cultured in RMPI1640 medium (SH30809.01B, Hyclone, USA) with 10% of FBS (10099141, Gibco, Australia), 100 units/ml of penicillin, and 100μg/ml of streptomycin (C0222, Beyotime, China). Cells were propagated when cells’ confluence was 70–80%, and they were randomly divided into Control, Slit2-N, VEGF165, and Slit2-N + VEGF165.
2.8 CCK-8 assay for determination of cell proliferation
Cell Counting Kit-8 reagent (C0038, Dojindo, Japan) and cell culture medium were mixed in a ratio of 1: 9 in darkness. Then, the cells were treated with the mixed CCK-8 reagent after the cells were washed twice softly with PBS. Two hours later, the absorbance of the liquid in each well was measured at the wavelength of 450 nm.
2.9 Flow cytometry assessments on cell proliferation and apoptosis
Five, six-carboxyfluorescein diacetate succinimidyl ester (CFSE) (C34554, Life Technologies, USA) was diluted in dimethyl sulfoxide (DMSO) to prepare storage solution in which the concentration of CFSE in the storage solution was 5mM. Cells were suspended in PBS at a final concentration of 106/ml. CFSE in the storage solution was added to the cell suspension, in which the working concentration of CFSE was 5μM. Cells in each group were then incubated with CFSE in darkness at room temperature for 20 min. Cold FBS, in a volume of 40% of the mixed PBS, was added to terminate the CFSE labeling to the cells. The cells were then seeded in 6-well plates in a density of 5 × 105/well. At hour 48 after the CFSE treatment, the cells were harvested. Fluorescence of the cells was measured at once by flow cytometry. Cell proliferation was calculated with the use of the proliferation wizard model. Proliferation index refers to cells’ amount of all the generations divided by the parental cells’ number at the start of the experiment.
Cell apoptosis in VEGF165 and Slit2-N + VEGF165 in HUVEC and HMVEC was measured by flow cytometry at hours 24, 48, and 72 after VEGF165 treatment or Slit2-N + VEGF165 treatment. Cells in each group were harvested and then were washed by cold PBS. Then, the cells were suspended at a concentration of 1 × 106 cells/ml by 1× Binding Buffer. The cell suspensions were then stained with Annexin V and PI at room temperature for 20 min. Cell apoptosis was measured with the use of flow cytometry.
2.10 Western blotting
Total protein of the cells in each group was gained with the use of cell lysis buffer aids (P0013B, Beyotime, China). Fiftyμg protein was separated in SDS-PAGE and then was transferred to PVDF membranes (IPFL20200, Millipore, USA). The PVDF membranes were blocked by 5% of BSA (HZB0148, Sigma, USA) at room temperature for 2 h. Each PVDF membrane was, respectively, incubated with primary antibodies of monoclonal rabbit anti-human GAPDH (bs-2188R, Bioss, China), AKT (#4685, Cell Signaling Technology, USA), p-AKT (#4060, Cell Signaling Technology, USA), ERK1/2 (#4695, Cell Signaling Technology, USA), p-ERK1/2 (#4370, Cell Signaling Technology, USA) and Robo4 (ab103674, Abcam, the UK) at 4℃overnight. After the incubation, the PVDF membranes were washed three times by TBST (Tris-Buffered Saline and Tween 20) and were incubated with HRP-tagged secondary antibody of mouse anti-rabbit IgG (bs-0295M-HRP, Bioss, China) in a dilution of 1: 1500 at room temperature for 1 h. The membranes were then washed three times by TBST, 10 min for each. Finally, the chemiluminescence intensity of HRP tagged to the secondary antibody in each band was detected.
2.11 Robo4 siRNA transfection
Cells were seeded in 6-well plates at a concentration of 105/well. When the cell confluence got around 40%, the transfection was performed according to manufacturers’ instructions. The cells were transfected with Cy3 (siN05815122149-1–1, Ribobio, China), negative control siRNA sequences (siN05815122147-1–5, Ribobio, China) and Robo4 siRNA (stB0011587C-1–5, Ribobio, China) by transfection reagent (C10511-05). At hour 48 after the treatment, total protein in each group was harvested.
2.12 Statistical analysis
The data were presented as mean±SD and were analyzed with one-way ANOVA or multi-way ANOVA followed by Student-Newman-Keuls test for multiple comparisons and Student’s t-test for pairwise comparisons. P<0.05 was considered statistically significant.
3. Results
3.1 CNV rat models were successfully constructed by krypton-argon laser photocoagulation, and subretinal injection was successfully performed
Hardly the laser photocoagulation finished when fundus photography was taken. Through the fundus photography, five white round spots around the optic nerve head were observed (). The white round spots indicate that the Bruch’s membrane is disrupted and five bubbles are successfully formed, which are the signs of successful photocoagulation for CNV model construction. At day 14 after the laser treatment, intraperitoneal injection of 0.1 ml fluorescein sodium was performed. Five leakage areas were observed under a microscope (). The vascular leakages indicate that CNV lesions were induced. As the fundus photography showed, the retina without subretinal injection was flat and smooth (supplemental Figure 1A) and that a smooth reflective projection was formed as the arrow pointed to (supplemental Figure 1A), which indicates subretinal injection was successfully done.
Figure 1. CNV rat models were successfully constructed by krypton-argon laser photocoagulation.
The results showed that, compared with the untreated rats (), there were five round white spots around the optic nerve head, which indicates that the Bruch’s membrane is disrupted and five bubbles are formed successfully (). At day 14 after photocoagulation, fundus fluorescein angiography (FFA) was performed to evaluate CNV formation. The fluorescein leakages of the fundus indicate that angiogenesis networks are formed in the laser-treated rats () compared with the untreated ones ().
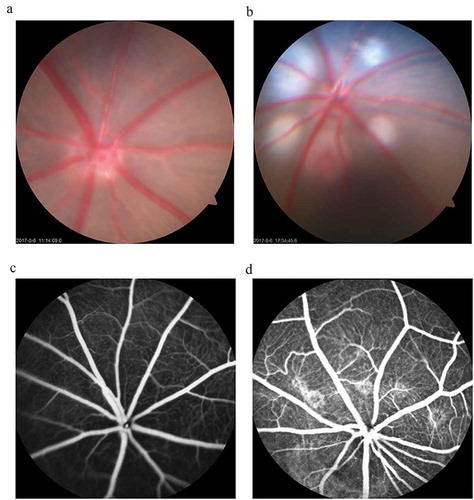
3.2 Slit2-N significantly lessened laser-induced CNV lesions in vivo
For evaluation of areas of the CNV lesions, FITC-dextran perfusion, choroid-sclera flat mounts, paraffin sections, and HE staining were performed at days 7, 14, 21, and 28 after the treatment. The areas of the CNV lesions in Laser + Slit2-N were significantly smaller than that in Laser at days 14, 21, and 28 (P<0.001, P<0.001, P<0.01, respectively) after the treatment, while there was no significant difference in areas of the CNV lesions between Laser and Laser + Vehicle (p>0.05) ().
Figure 2. Slit2-N significantly reduced areas and relative thicknesses of the CNV networks.
Outlines of the CNV lesions in each group at each time point were sketched and measured (). The areas of the CNV networks are significantly smaller at days 14 (p<0.001), 21 (p<0.001) and 28 (p<0.01) in Laser + Slit2-N group than in Laser group while there was no significant difference between Laser group and Laser + Vehicle group at each time point (p>0.05). There was no significant difference among the Laser group, Laser + Vehicle group, Laser + Slit2-N group at day 7 (p>0.05) (). As the white lines show in each picture (), “T” refers to the actual thickness of the vascular networks, from the bottom of the pigmental choroidal layer to the top of the vascular networks. “C” refers to the laser-unaffected normal thickness of the choroid. Relative thickness (ratio) was calculated according to the equation, ratio = T-C/C. There was no significant difference between the Laser group and Laser + Vehicle group at each time point after the treatment (). However, the ratio statistically decreased in Laser + Slit2-N group compared with that in the Laser group at days 14 (p<0.01), 21 (p<0.01) and 28 (p<0.01). There was no significant difference between the two groups at day 7. (*p<0.05,** p<0.01, *** p<0.001)
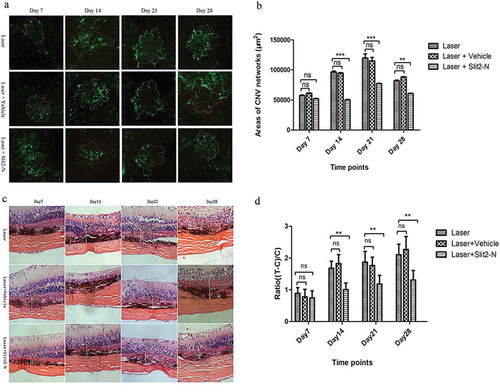
At days 7, 14, 21, and 28 after the treatment, paraffin sections and HE staining were performed to assess relative thicknesses of the CNV lesions. The relative thickness of CNV lesions was calculated according to the ratio (ratio = T/T-C). The ratio in Laser + Slit2-N was significantly smaller than that in Laser at days 14, 21, and 28 (p<0.01, p<0.01, p<0.01, respectively) after the treatment while there was no significant difference in the ratio between Laser and Laser + Vehicle (p>0.05) ().
The data mentioned above showed that the subretinal administration of Slit2-N significantly reduced the volume of CNV lesions at days 14, 21, and 28 after the treatment. The results of the in vivo study indicate that the subretinal administration of Slit2-N lessened the CNV lesions.
3.3 Screens on the minimum effective concentrations of VEGF165 and the maximal safe concentrations of Slit2-N in HUVEC and HMVEC in vitro
At hour 48 after the treatment with protein VEGF165 or Slit2-N to HUVEC and HMVEC, respectively, cell proliferations of each group of HUVEC and HMVEC were measured by CCK-8 assay. The CCK-8 results showed that at hour 48 after the treatment of VEGF165, proliferations of HUVEC at the concentration of 25 ng/ml, 30 ng/ml and 35 ng/ml were significantly higher than that in Control (, P<0.0001, P<0.0001, P<0.0001, respectively), and the proliferations of HMVEC at concentrations of 500 pM and 1000 pM were significantly higher than that in Control group (, P<0.01,P<0.0001, respectively). For the following experiments in HUVEC and HMVEC, the minimum effective concentrations of VEGF165 in both cells were chosen, namely 25 ng/ml and 500 pM, respectively, for HUVEC and HMVEC. The maximal safe concentration of Slit2-N, which did not impact cell viability, namely 16nM in both HUVEC and HMVEC ( and ), was chosen for the experiments followed.
Figure 3. Screens on minimal effective concentrations of VEGF165 and maximal safe concentrations of Slit2-N, respectively, in HUVEC and HMVEC.
CCK-8 assay was used to evaluate proliferation and viability of the cells in each group of HUVEC and HMVEC. At hour 48 after the treatment, 25 ng/ml, 30 ng/ml and 35 ng/ml of VEGF165 significantly prompted the proliferation of HUVEC compared with the untreated group (p<0.0001, p<0.0001, p<0.0001, respectively) (). In HMVEC, 500 pM and 1000 pM of VEGF165 significantly promoted cell proliferation (p<0.01, p<0.0001) ().On the part of Slit2-N, the maximal safe concentration, which did not compact the cell viability, was 16nM in both HUVEC and HMVEC (). (*p<0.05, ** p<0.01, *** p<0.001, **** p<0.0001)
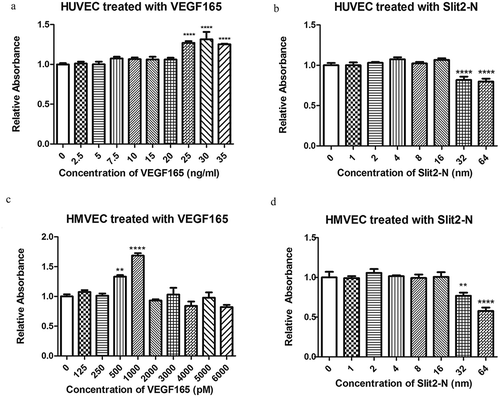
3.4 Slit2-N reduced VEGF165-induced HUVEC and HMVEC proliferation
The CCK-8 results showed that the endothelia proliferation in Slit2-N + VEGF165 was significantly lower than that in VEGF165 in both HUVEC and HMVEC (, p<0.05 and p<0.01, respectively, in HUVEC and HMVEC). There was no significant difference in proliferation of vascular endothelia between Control and Slit2-N in either HUVEC or HMVEC (). In consistence with the CCK-8 results, results of the flow cytometry assessment on CFSE-labeling cells showed that the proliferation index was significantly reduced in Slit2-N + VEGF165 compared with that in VEGF165 in HUVEC and HMVEC (p<0.001 in both HUVEC and HMVEC). No significant difference in proliferation index was found between Control and Slit2-N in either HUVEC or HMVEC (). There was no significant difference in apoptosis between Slit2-N+VEGF165 and VEGF165. (Supplemental Figure 2)
Figure 4. Slit2-N significantly reduced VEGF165-induced endothelia proliferation.
CCK-8 assay showed that, in both HUVEC and HMVEC, the cell proliferation in Slit2-N + VEGF165 group was significantly inhibited compared with that in VEGF165 group (p<0.05 and p<0.01, respectively, in HUVEC and HMVEC) (). Consistent with the CCK-8 results, the flow cytometry results showed that the proliferation index significantly decreased in Slit2-N + VEGF165 group compared with that in VEGF165 group in both HUVEC and HMVEC (p<0.01 in both HUVEC and HMVEC) (). (Proliferation index refers to the sum of the cells in all generations divided by the original parent cells number at the start of the experiment).
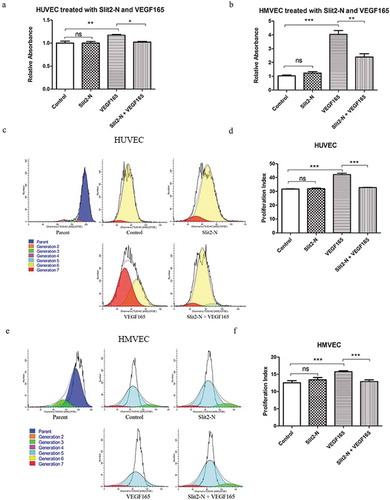
3.5 Slit2-N reduced phosphorylation of AKT activated by VEGF165-VEGFR2 signaling while it failed to impact VEGF165-induced phosphorylation of ERK1/2
Phosphorylations of AKT and ERK1/2 were detected by western blotting (WB). The WB results showed that p-AKT was significantly less in Slit2-N + VEGF165 than in VEGF165 in both HUVEC and HMVEC (p<0.001 in HUVEC, p<0.01 in HMVEC) while there was no significant difference in p-AKT between Control and Slit2-N (P>0.05) (). In contrast with the results of p-AKT, no significant difference in p-ERK1/2 was found between Slit2-N + VEGF165 and VEGF165 (p>0.05) ().
Figure 5. Phosphorylations of AKT and ERK1/2.
Phosphorylation of AKT significantly decreased in Slit2-N + VEGF165 group compared with that in VEGF165 group in both HUVEC and HMVEC (p<0.001 in HUVEC, p<0.01 in HMVEC), while no statistical difference in p-AKT was found between the Control group and Slit2-N group (p>0.05 in both HUVEC and HMVEC) (). No significant difference in phosphorylation of ERK1/2 was found between Slit2-N + VEGF165 group and VEGF165 group in either HUVEC or HMVEC (p>0.05 in both HUVEC and HMVEC) ().
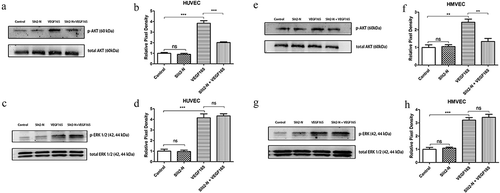
The WB data indicate that the inhibitory effect of Slit2-N on VEGF165-induced proliferation of vascular endothelia may be through VEGFR2-AKT pathway rather than VEGFR2-ERK1/2 pathway.
SC-79, an AKT activator, was used to investigate if phosphorylation of AKT impacts the inhibitory effect of Slit2-N on VEGF165-induced proliferation. The western blot results showed that SC-79 significantly reversed the inhibitory effect of Slit2-N on phosphorylation of AKT in HUVEC (, p<0.001) and HMVEC (, p<0.001). The CCK-8 results showed cell proliferation in SC-79+ Slit2-N+ VEGF165 was significantly higher than in Slit2-N+ VEGF165 in HUVEC (, p<0.001) and HMVEC (, p<0.001). The WB results and CCK-8 results indicate that p-AKT is involved in the inhibitory effect of Slit2-N on VEGF165-induced cell proliferation.
Figure 6. SC-79, an AKT activator, reversed the inhibitory effect of Slit2-N on VEGF165-induced proliferation.
Western blot results showed SC-79 significantly reversed the inhibitory effect of Slit2-N on phosphorylation of AKT in HUVEC () and HMVEC (); CCK-8 results showed that the administration of SC-79 significantly reversed the inhibitory effect of Slit2-N on VEGF165-induced cell proliferation in HUVEC and HMVEC ().
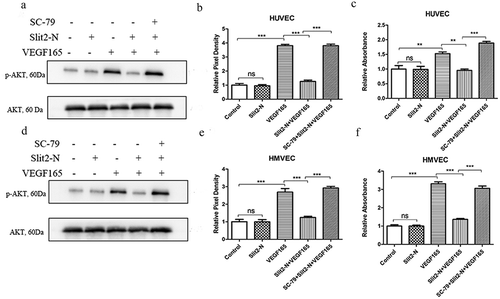
3.6 Knockdown of Robo4 significantly reversed the inhibitory effect of Slit2-N on VEGF165-induced proliferation of vascular endothelia
The CCK-8 results showed that the proliferation of vascular endothelia in Robo4 siRNA + Slit2-N + VEGF165 was significantly higher than that in Slit2-N + VEGF165 in both HUVEC and HMVEC (p<0.01, ). The CCK-8 results indicate that knockdown of Robo4 reduces the inhibitory effect of Slit2-N on VEGF165-induced vascular endothelia proliferation, and the inhibitory effect of Slit2-N on VEGF165-induced proliferation of vascular endothelia is possibly mediated by Robo4.
Figure 7. Robo4 siRNA suppressed Robo4 expression and it significantly reversed the inhibitory effect of Slit2-N on VEGF165-induced cell proliferation.
The Cy3 staining showed that the transfection rate (the number of stained cells divided by the number of the total cells) of the transfection reagent was around 90% in HUVEC () and HMVEC (). The western blotting results () showed that the Robo4 expression was inhibited in Robo4 siRNA group compared with Control group in both HUVEC (p<0.001) and HMVEC (p<0.001) (). The CCK-8 results showed that the proliferation in Robo4 SiRNA+Slit2-N+ VEGF165 group was significantly higher than that of Slit2-N+ VEGF165 group in both HUVEC (p<0.01) and HMVEC (p<0.01) ().
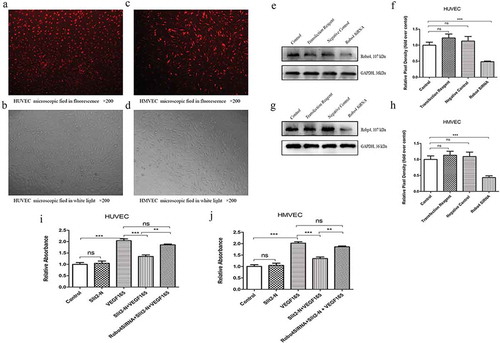
4. Discussion
In the present study, we focused on the role of recombinant protein Slit2-N, which is the bioactive fragment of Slit2 binding to the Robo receptors, in proliferation of vascular endothelia in a CNV model, since the function of gene Slit2 has been studied in it [Citation10,Citation11]. We found that the subretinal administration of Slit2-N significantly lessened areas and relative thicknesses of CNV lesions in vivo, and it inhibited VEGF165-induced proliferation of HUVEC and HMVEC possibly via Slit2-N-Robo4-AKT pathway in vitro.
The in vivo study showed that the areas and relative thicknesses of the CNV lesions in Slit2-N + Laser were significantly less than those in Laser at day 14, day 21, and day 28, while no significant difference between Laser and Laser + vehicle was observed at each time point. The results of the in vivo study indicate that Slit2-N administration has the potent to inhibit angiogenesis events in a CNV rat model. Previous studies showed that intravitreal administration of recombinant Slit2-N reduced the vascular permeability and enhanced the integrity of blood vessels, while Slit2-N failed to induce any mitotic activities of endothelia [Citation12]. In contrast with it, a recent study showed that after laser coagulation, there were more vascular leakages of the CNV lesions in Slit2 transgenic mice than in the wild type mice [Citation10]. These controversial results may be due to the different contexts (e.g. due to the difference between endogenous Slit2 and exogenous one). Anyway, our results in vivo showed subretinal administration of protein Slit2-N lessened CNV lesions in rats, which suggests that Slit2-N may be potentially applied in CNV therapy in the future.
To investigate the effect of Slit2-N on endothelia in vitro and some related mechanisms, we screened the minimum effective concentrations of VEGF165 and Slit2-N’s maximal safe concentrations which did not harm the cell viability and possibly exert its function in the greatest degree in HUVEC and HMVEC. As shown in , the minimum effective concentrations of VEGF165 in the two types of endothelial cells were different, namely 25ng/ml in HUVEC and 500pM in HMVEC. It may be resulted from the differential expressions probe sets involved in angiogenesis-associated cell signaling in different tissue-derived cells [Citation13]. The CCK-8 assay result indicates that it is necessary and important to screen the effective concentration of VEGF165 and safe concentration of Slit2-N in vitro, since different tissue-derived endothelia may have different sensitivities to the same growth factor or exogenous protein.
By analyzing cell proliferation and proliferation index with CCK-8 assay and flow cytometry, we found that cell proliferation and proliferation index were significantly reduced in Slit2-N+ VEGF165 compared with that in VEGF165, which implies that Slit2-N reduced VEGF165-induced cell proliferation of HUVEC and HMVEC. In a previous study, similarly, Slit2-N reduced VEGF-C-induced proliferation of lung-derived lymphatic endothelial cells in a lung cancer condition. However, gene Slit2 alone did not exert any impact on mitogenic activities of endothelia in physiological and pathological vascularization of the retina [Citation11,Citation14]. It was likely that the reduction in cell proliferation in retinal angiogenesis in the Slit2-knockdown mice was due to Slit2 activity on vessel-associated cell types such as mural cells or leukocytes [Citation11]. Nonetheless, some evidences showed that Slit2 promoted HUVEC proliferation. Shanshan Li et al. found that overexpression of Slit2 gene promoted proliferation of HUVEC via Robo1-VEGFR2-ERK1/2 pathway. In diabetic retinopathy, recombinant Slit2 protein promoted proliferation of retinal pigment epithelia and thus initiated the pathogenesis of diabetic retinopathy. These findings from various researches are interesting, conflicting and confusing. Slit2 may exert different roles in cell proliferation in different contexts (e.g. endogenous Slit2 or exogenous Slit2; Growth factors, such as VEGF and PEDF, were involved or not).
As we observed that the endothelia proliferation was reduced in Slit2-N + VEGF165 compared with VEGF165 group, we asked if the reduction of proliferation in Slit2-N+ VEGF165 was due to an increased apoptosis in this group. As shown in supplemental , it suggests that Slit2-N did not induce apoptosis in Slit2-N + VEGF165, which was consistent with the previous finding in vivo [Citation11].
Our results showed that the combined administration of protein Slit2-N and VEGF165 induced proliferation of HUVEC and HUMVE and Slit2-N alone did not impact it. We speculated that the function of Slit2-N on vascular endothelia proliferation is closely associated with the interaction between Slit2-Robo signaling axis and VEGF165-VEGFR2 (vascular endothelia growth factor receptor 2) signaling pathway. In another word, the inhibitory effect of Slit2-N on the vascular endothelia proliferation was based on the activation of VEGFR2 and its downstream molecules. In a cardiovascular disease rabbit model, the researchers transferred gene Slit2 combined with two types of new VEGFs, the snake venom-derived vammin and human VEGF-D, both of which can bind to the VEGFR2, into the limb muscle of New Zealand White rabbits. Slit2 inhibited vammin-induced vascular permeability and capillary size by reducing the activation of the endothelial nitric-oxide synthase (eNOS) [Citation15]. Besides, in the tumor-derived endothelia, elevated Slit2 modulated by deficient Eph-A2, alleviated VEGF-induced vascular events by reducing the activation of Arf6, a downstream molecule of VEGFR [Citation16]. Additionally, recombinant Slit2-N restored VEGF-C-induced lymphangiogenesis in a lung cancer context [Citation17]. Vice versa, Robo1 and Robo2, the binding receptors of Slit2, were indispensable for VEGF-A-induced vessel sprouting in the retina [Citation11]. These evidences hinted us that Slit2-Robo signaling axis had a complex interaction with VEGF-VEGFR signaling.
Based on the findings the recent studies showed to us, we then tested some activated signaling molecules downstream the VEGFR2, p-AKT, and p-ERK1/2, which probably participate in the survival and proliferation of endothelia. No significant difference of the relative expression of p-ERK1/2, a classical mitotic molecule, was observed between Slit2-N+ VEGF165 and VEGF165 in either HUVEC or HMVEC. However, there was a statistical reduction of p-AKT in Slit2-N + VEGF165 compared with VEGF165. Thus, AKT is probably involved in the inhibitory effect of Slit2-N on VEGF165-induced cell proliferation. It is widely believed that Slit2-Robo signaling regulates cell polarity [Citation18], cytoskeleton reorganization [Citation19], lamellipodia formation [Citation20], cell migration [Citation21–Citation23] and vascular permeability [Citation12,Citation14,Citation15,Citation24,Citation25] by modulating the Rho family members such as Cdc42 [Citation26], Rac1 [Citation18], by modulating the adaptor proteins like paxillins [Citation9,Citation14,Citation27] and NCKs [Citation28] and by modulating the Ras superfamily members like ADP-ribosylation factor (ARF) [Citation29]. Among these molecules in the signaling axes, ARF was thought to be associated with the internalization of membrane receptors [Citation30]. Thus, Slit2 may function via modulating the internalization or presentation of membrane receptors, such as VEGFR2. Thereby one potential explanation of our finding is that Slit2-N inhibited the phosphorylation of AKT,and thus modulated the presentation of VEGFR2 and the biological function of endothelia like proliferation.
To investigate if Robo4, one of the receptors of Slit2, was involved in the inhibitory effect of Slit2-N on VEGF165-induced cell proliferation, we selected Robo4 as our study object as it was documented to be an inhibitor in many circumstances [Citation22,Citation23,Citation31]. As is shown in , the inhibitory effect of Slit2-N significantly alleviated in both HUVEC and HMVEC when Robo4 was knocked down. Besides Robo1 and Robo2, Robo4 was the newly identified receptor of Slit2, and it was considered exclusively expressed on the endothelia membrane [Citation32]. Robo4 negatively regulated the Slit2-induced migration by dimerizing with Robo1 on HUVEC in vitro [Citation23]. Besides, some non-receptor tyrosine kinases, Fyn, Yes and Src were modulated by Slit2-Robo4 signaling and alleviated vascular leakages in pathological angiogenesis [Citation12]. Recent studies showed that the ectodomain of Robo4 interacted with UNC5B, a vascular netrin receptor, rather than directly bind with Slit2, to play its role in modulating angiogenesis [Citation31]. The ectodomain of Robo4 interacting with UNC5B was demonstrated participating in the phosphorylation of VEGFR2 and the downstream molecules to modulate angiogenesis [Citation33]. Anyhow, our study demonstrated that Robo4 mediated the inhibitory effect of Slit2-N on VEGF165-induced vascular endothelia proliferation in HUVEC and HMVEC.
In summary, our results showed Slit2-N exerted an inhibitory effect on angiogenesis events in CNV rats, and its inhibitory effect on VEGF165-induced proliferation of endothelial cells was probably via Slit2-N-Robo4-AKT pathway. The present study enriched our understanding of the pathological mechanisms of CNV. Additionally, it presents some potential targets, molecules along Slit2-N-Robo4-AKT signaling axis, to CNV therapy.
Supplemental Material
Download Zip (2.8 MB)Acknowledgments
We are grateful for the facility support from Chongqing Key Laboratory of Ophthalmology, Chongqing, China, and also appreciate the guidance of professor Hang Sun in Institute for Viral Hepatitis, Chongqing Medical University, Chongqing, China.
Disclosure statement
No potential conflict of interest was reported by the authors.
Supplemental material
Supplemental data for this article can be accessed here.
Additional information
Funding
References
- Witmer AN, Vrensen GF, Van Noorden CJ, et al. Vascular endothelial growth factors and angiogenesis in eye disease. Prog Retin Eye Res. 2003;22(1):1–29.
- Penn JS, Madan A, Caldwell RB, et al. Vascular endothelial growth factor in eye disease. Prog Retin Eye Res. 2008;27(4):331–371.
- Chen CY, Tsai CH, Chen CY, et al. Human placental multipotent mesenchymal stromal cells modulate placenta angiogenesis through Slit2-Robo signaling. Cell Adh Migr. 2016;10(1–2):66–76.
- Liu D, Hou J, Hu X, et al. Neuronal chemorepellent Slit2 inhibits vascular smooth muscle cell migration by suppressing small GTPase Rac1 activation. Circ Res. 2006;98(4):480–489.
- Guijarro-Muñoz I, Cuesta AM, Alvarez-Cienfuegos A, et al. The axonal repellent Slit2 inhibits pericyte migration: potential implications in angiogenesis. Exp Cell Res. 2012;318(4):371–378.
- Ho TH, Serie DJ, Parasramka M, et al. Differential gene expression profiling of matched primary renal cell carcinoma and metastases reveals upregulation of extracellular matrix genes. Ann Oncol. 2017;28(3):604–610.
- Le LT, Cazares O, Mouw JK, et al. Loss of miR-203 regulates cell shape and matrix adhesion through ROBO1/Rac/FAK in response to stiffness. J Cell Biol. 2016;212(6):707–719.
- Yang YC, Chen PN, Wang SY, et al. The differential roles of Slit2-exon 15 splicing variants in angiogenesis and HUVEC permeability. Angiogenesis. 2015;18(3):301–312.
- Sherchan P, Huang L, Akyol O, et al. Recombinant Slit2 reduces surgical brain injury induced blood brain barrier disruption via Robo4 dependent Rac1 activation in a rodent model. Sci Rep. 2017;7(1):746.
- Li S, Huang L, Sun Y, et al. Slit2 promotes angiogenesis activity via the robo1-VEGFR2-ERK1/2 pathway in both in vivo and in vitro studies. Invest Ophthalmol Vis Sci. 2015;56(9):5210–5217.
- Rama N, Dubrac A, Mathivet T, et al. Slit2 signaling through Robo1 and Robo2 is required for retinal neovascularization. Nat Med. 2015;21(5):483–491.
- Jones CA, London NR, Chen H, et al. Robo4 stabilizes the vascular network by inhibiting pathologic angiogenesis and endothelial hyperpermeability. Nat Med. 2008;14(4):448–453.
- Browning AC, Halligan EP, Stewart EA, et al. Comparative gene expression profiling of human umbilical vein endothelial cells and ocular vascular endothelial cells. Br J Ophthalmol. 2012;96(1):128–132.
- Jones CA, Nishiya N, London NR, et al. Slit2-Robo4 signalling promotes vascular stability by blocking Arf6 activity. Nat Cell Biol. 2009;11(11):1325–1331.
- Nieminen T, Toivanen PI, Laakkonen JP, et al. Slit2 modifies VEGF-induced angiogenic responses in rabbit skeletal muscle via reduced eNOS activity. Cardiovasc Res. 2015;107(2):267–276.
- Youngblood V, Wang S, Song W, et al. Elevated Slit2 activity impairs VEGF-induced angiogenesis and tumor neovascularization in EphA2-deficient endothelium. Mol Cancer Res. 2015;13(3):524–537.
- Yu J, Zhang X, Kuzontkoski PM, et al. Slit2N and Robo4 regulate lymphangiogenesis through the VEGF-C/VEGFR-3 pathway. Cell Commun Signal. 2014;12:25.
- Fritz RD, Menshykau D, Martin K, et al. SrGAP2-dependent integration of membrane geometry and slit-robo-repulsive cues regulates fibroblast contact inhibition of locomotion. Dev Cell. 2015;35(1):78–92.
- Anand AR, Zhao H, Nagaraja T, et al. N-terminal Slit2 inhibits HIV-1 replication by regulating the actin cytoskeleton. Retrovirology. 2013;10:2.
- Sheldon H, Andre M, Legg JA, et al. Active involvement of Robo1 and Robo4 in filopodia formation and endothelial cell motility mediated via WASP and other actin nucleation-promoting factors. Faseb J. 2009;23(2):513–522.
- Ning Y, Sun Q, Dong Y, et al. Slit2-N inhibits PDGF-induced migration in rat airway smooth muscle cells: WASP and Arp2/3 involved. Toxicology. 2011;283(1):32–40.
- Cai H, Xue Y, Li Z, et al. Roundabout4 suppresses glioma-induced endothelial cell proliferation, migration and tube formation in vitro by inhibiting VEGR2-mediated PI3K/AKT and FAK signaling pathways. Cell Physiol Biochem. 2015;35(5):1689–1705.
- Enomoto S, Mitsui K, Kawamura T, et al. Suppression of Slit2/Robo1 mediated HUVEC migration by Robo4. Biochem Biophys Res Commun. 2016;469(4):797–802.
- Han HX, Geng JG. Over-expression of Slit2 induces vessel formation and changes blood vessel permeability in mouse brain. Acta Pharmacol Sin. 2011;32(11):1327–1336.
- Gorbunova EE, Gavrilovskaya IN, Mackow ER. Slit2-Robo4 receptor responses inhibit ANDV directed permeability of human lung microvascular endothelial cells. Antiviral Res. 2013;99(2):108–112.
- Myers JP, Robles E, Ducharme-Smith A, et al. Focal adhesion kinase modulates Cdc42 activity downstream of positive and negative axon guidance cues. J Cell Sci. 2012;125(Pt 12):2918–2929.
- Tseng RC, Chang JM, Chen JH, et al. Deregulation of SLIT2-mediated Cdc42 activity is associated with esophageal cancer metastasis and poor prognosis. J Thorac Oncol. 2015;10(1):189–198.
- Dubrac A, Genet G, Ola R, et al. Targeting NCK-mediated endothelial cell front-rear polarity inhibits neovascularization. Circulation. 2016;133(4):409–421.
- Zhao H, Ahirwar DK, Oghumu S, et al. Endothelial Robo4 suppresses breast cancer growth and metastasis through regulation of tumor angiogenesis. Mol Oncol. 2016;10(2):272–281.
- D’Souza-Schorey C, Chavrier P. ARF proteins: roles in membrane traffic and beyond. Nat Rev Mol Cell Biol. 2006;7(5):347–358.
- Koch AW, Mathivet T, Larrivée B, et al. Robo4 maintains vessel integrity and inhibits angiogenesis by interacting with UNC5B. Dev Cell. 2011;20(1):33–46.
- Huminiecki L, Gorn M, Suchting S, et al. Magic roundabout is a new member of the roundabout receptor family that is endothelial specific and expressed at sites of active angiogenesis. Genomics. 2002;79(4):547–552.
- Zhang F, Prahst C, Mathivet T, et al. The Robo4 cytoplasmic domain is dispensable for vascular permeability and neovascularization. Nat Commun. 2016;7:13517.