ABSTRACT
Mammalian oocyte meiosis is a special form of cell division that provides haploid gametes for fertilization. Unlike in mitosis, post-translational modifications (PTMs) are more crucial during meiosis because of the absence of de novo mRNA transcription. As a classic PTM, protein neddylation is a biological process that mediates protein degradation by modifying cullin proteins and activating the Cullin-Ring E3 ligases. This process plays important roles in various biological processes such as autophagy and tumorigenesis. However, the function of neddylation in germ cells is unknown. In this study, we observed that the inhibition of neddylation by its specific inhibitor MLN4924 significantly arrests mouse oocyte at the stage of metaphase during meiosis. The arrested oocytes display impaired spindles with over-activation of spindle assembly checkpoint (SAC). Accordingly, we identified early mitosis inhibitor 1 (Emi1), a key inhibitor of anaphase-promoting complex/cyclosome (APC/CFzr1), as a substrate of neddylation-mediated protein degradation. Thus, our study uncovered an unknown role of neddylation in female germ cells and suggests that proper neddylation is essential for oocyte maturation.
Introduction
Infertility and sterility are currently a global health issue affecting 12–28% of couples [Citation1]. Errors in oocyte meiosis comprise one of the major causes of infertility. During meiosis, oocytes arrest at the germinal vesicle (GV) phase for decades and enter germinal vesicle breakdown (GVBD) in response to a surge of luteinizing hormone [Citation2,Citation3]. Subsequently, the oocyte enters the prophase of metaphase I (Pro-MI), during which spindles assemble, centrosomes move to the poles and chromosomes are condensed. Next, during the metaphase of meiosis I (MI) homologous chromosomes align on the metaphase plate. Spindle microtubules attach to the kinetochores, preparing for chromosome segregation in the following anaphase I. Accurate spindle assembly and chromosome segregation are indispensable for successful meiosis [Citation4]. During the metaphase of meiosis II (MII), the oocyte excludes the first polar body and awaits fertilization. Any abnormality in oocyte maturation can lead to female infertility, birth defects, and failures in assisted reproductive technology [Citation5,Citation6].
As de novo mRNA transcription does not occur during oocyte maturation [Citation7], protein post-translational modifications (PTMs) become obviously critical for oocyte maturation. Ubiquitination is a classical PTM that facilitates the degradation of target proteins [Citation8]. Analogous to ubiquitination, neddylation is also catalyzed through a three-step enzymatic cascade. Specifically, it is a process wherein neural precursor cell expressed developmentally down-regulated 8 (Nedd8) is covalently conjugated to its substrates [Citation9], relying on its own Nedd8-activating enzyme (E1), Nedd8-conjugating enzyme (E2) and specific Nedd8 ligases (E3s) [Citation10–Citation13]. As a ubiquitin-like protein, Nedd8 shares 59% amino acid identity and 76% similarity with ubiquitin [Citation14,Citation15] and is ubiquitously expressed in adult tissues. Nedd8 contains a carboxy-terminal tail and covalently binds target proteins via the Gly-Gly sequence of the tail [Citation16]. It is first synthesized as a precursor with no function, then the precursor is hydrolyzed at the G76 residue and becomes mature Nedd8 [Citation17,Citation18]. Mature Nedd8 is activated by Nedd8 E1 activating enzyme (NAE1), then transferred from E2 (including UBE2M and UBE2F) to a lysine residue, and finally covalently conjugated to its substrates via isopeptide bonds with the help of E3 during neddylation process [Citation17,Citation19]. Unlike ubiquitination, the Nedd8 pathway regulates the activity rather than the abundance of its substrates [Citation20]. Although neddylation plays important roles in various biophysical and pathological processes of somatic cells [Citation11,Citation13], whether it functions during meiosis is still unknown.
It is known that the cullin proteins are the most important substrates of neddylation [Citation21]. They are core structures of the Cullin-RING E3 complex and are involved in the ubiquitination of many important proteins. Cullin proteins belong to a superfamily including eight proteins (Cullin1, Cullin2, Cullin3, Cullin4A, Cullin4B, Cullin5, Cullin7, and Cullin9) in mammals [Citation22]. Among these, Cullin1 exerts multiple biological effects on processes such as cell cycle progression [Citation23]. Inhibition of neddylation leads to diminished activity of cullin proteins and an increase of their substrates because of impaired ubiquitination [Citation24]. However, whether neddylation functions during oocyte maturation is unclear.
In this study, we found that both Nedd8 and Cullin1 were expressed in mouse oocytes and the oocytes were arrested at the MI phase after the inhibition of neddylation with MLN4924 (a selective inhibitor of NAE1) treating and by knocking down the Nedd8 of mouse oocytes. The arrested oocytes displayed impaired spindles with over-activation of spindle assembly checkpoint (SAC) together with failed chromosome segregation. Based on proteomics analysis, we identified Emi1, a key inhibitor of anaphase-promoting complex/cyclosome (APC/CFzr1), as a substrate of Nedd8-mediated protein degradation. Thus, our study uncovers an unknown role of neddylation in oocytes and suggests that proper neddylation is essential for meiotic maturation.
Materials and methods
Antibodies
Rabbit monoclonal anti-Nedd8 antibody, rabbit monoclonal anti-Bub3 antibody, goat polyclonal anti-BubR1 antibody, and rabbit polyclonal anti-Mad2 were purchased from Abcam (USA; Cat#: ab81264, ab133699, ab28193, ab70383, respectively). Mouse monoclonal anti-Emi1 antibody was purchased from Santa Cruz Biotechnology (USA; Cat#: sc-365,212). Rabbit polyclonal anti-Fzr1 antibody was purchased from Novus (USA; Cat#: NBP2-15,840). Rabbit polyclonal anti-Cullin1 antibody was purchased from Proteintech (USA; Cat#: 12,895–1-AP). Mouse monoclonal anti-α-tubulin-FITC antibody was purchased from Sigma (USA; Cat#: F2168). Alexa Fluor 555 goat anti-rabbit IgG (H + L) (USA; Cat#: A21429) and Alexa Fluor 555 donkey anti-mouse IgG (H + L) were purchased from Invitrogen (USA; Cat#: A31570).
Oocyte collection and culture
Animal care and handling were conducted in accordance with the Institutional Animal Welfare and Ethics Committee Policies of Peking University Health Science Center. Female ICR mice (4–6 weeks) were hormonally stimulated with 5 IU pregnant mare serum gonadotropin for 44–46 hours and sacrificed by cervical dislocation. GV-stage oocytes were collected from ovaries and transferred into warmed M2 medium (USA, Sigma). To determine the optimal dose of MLN4924 for oocyte cultivation, gradient concentrations of MLN4924 (0.1 μM, 0.5 μM, 1 μM and 5 μM) were utilized. These oocytes were cultured at 37°C in a humid atmosphere of 5% CO2. For subsequent analysis, oocytes were harvested at GV, GVBD, Pro-MI, MI, and MII stages, respectively at 0, 2, 6, 8, and 12 h.
Dot blotting
Mouse oocytes of GV, GVBD, Pro-MI, MI, and MII stages were collected, and 30 oocytes were used for each sample. After lysis in RIPA buffer (China, Beyotime), the lysate was spotted on a nitrocellulose membrane. The membrane was dried in air and blocked with 5% skim milk (USA, Becton Dickinson) in TBST (China, Applygen) for 1 h at room temperature, and then washed three times with TBST. Then, the membrane was incubated in anti-Nedd8 antibody (1:1000) at 4°C overnight. After three washes in TBST, the membrane was incubated with horseradish peroxidase (HRP)-conjugated secondary antibody for 1 h at room temperature (RT). The dot blot was detected using an enhanced chemiluminescence (ECL) detection system (China, Tanon).
Immunoblotting
All samples were lysed in RIPA buffer containing a proteinase inhibitor on ice for 15 min. Proteins were extracted after centrifuging (12,000 × g, 10 min, 4°C). After the proteins were boiled for 5 min, proteins were loaded and separated using sodium dodecyl sulfate-polyacrylamide gel electrophoresis (SDS-PAGE). Proteins were electrically transferred to polyvinylidene fluoride membranes (USA, Millipore). These membranes were blocked in TBST containing 5% skim milk for 1 h at RT, and then incubated with specific primary antibodies (diluted by 1% skim milk in TBST) overnight at 4°C. After three washes with TBST, membranes were further incubated with corresponding HRP-conjugated secondary antibodies for 1 h at RT. The membranes were incubated with ECL buffer and visualized using an automatic ECL system.
Silver staining and mass spectrum preparation
Total proteins of mouse oocytes were extracted as previously described. Protein samples were separated by SDS-PAGE. Silver staining of all protein bands was performed according to the standard protocol of the Pierce TM Silver Stain for Mass Spectrometry kit (USA, Thermo Scientific). Bands of predicted location in the gel was cut and performed in-gel trypsin digestion for the following mass spectrum assay.
RNA extraction and quantitive real-time PCR (qRT-PCR)
Total RNA was extracted from mouse oocytes (200 oocytes/sample) using the RNeasy Micro Kit (Germany, QIAGEN) according to the manufacturer’s instructions. Then, 1 μg of total RNA was subjected to reverse transcription and converted to cDNA using a RevertAid First Strand cDNA Synthesis Kit (USA, Thermo Fisher Scientific) following the manufacturer’s instructions. qRT-PCR was performed using SYBR Green master mix with a QuantStudio 3 Real-Time PCR System (USA, Applied Biosystems). Each experiment was performed three times with triplicate samples. The primers sequences were as follows: Cul1 (5′-AGTGGGAAGATTACCGATTCTCC; 3′-CACGGCGAACCCAATGTCTA); Cul2 (5′-ATGTTGGAATATGTCGAGCGAG; 3′- AGTGGTTCAGGATAGGCCACA); Cul3 (5′-GAGATCAAGTTGTACGGTATGGG; 3′- GCTCTCTGGCAATCATATCCAAT); Cul4A (5′-TCTCACAAAGTCTCCCCAACG; 3′-AGGACGTAGGTTCGATCCAGA); Cul4B (5′-CGACTCAGAAGATAACAGTTCGG; 3′-GGCAGACACTTTTCATAGCTCC); Cul5 (5′-CGCCAGGAATCTGTAACAAAACA; 3′-CAGTACACGAGCCTGTGCTT); Cul7 (5′-GACCCAGATCCTTCTGTCATTG; 3′-GTGTTCCGTGAGAGTAGCCTG); Cul9 (5′-AGCGAAGAGGGCAAAGTGAG; 3′-CCTTTAGGTATTGGCCGCTC).
Immunofluorescence staining and image analysis
For immunofluorescence analysis, the zona pellucida was removed using hyaluronidase (1 mg/mL, 5 min). To detect protein expression, eggs were fixed in 4% paraformaldehyde in PBS (pH 7.4) for 30 min at RT and permeabilized using 0.5% triton X-100 in PBS for 30 min at RT. The oocytes were then incubated in blocking buffer (1% BSA in PBS) for 1 h at RT followed by incubating with primary antibodies (diluted in blocking buffer) at 4°C overnight, after washing three times (10 min each) in washing buffer (0.1% tween-20, 0.01% triton X-100 in PBS). Appropriate fluorescent secondary antibodies were used to label the oocytes for 1 h at RT. To label the DNA, oocytes were incubated in 10 μg/mL Hoechst 33342 for 15 min at RT, or were labeled with a direct-fluorescence α-tubulin antibody (1:200) for 1 h at RT. The immunostaining was visualized using a confocal microscope (Germany, Carl Zeiss 710). To ensure comparable results, all settings among different groups and replications were kept constant.
Chromosome spreading
According to previous reports [Citation25], the zona pellucida was removed and oocytes were transferred to M2 medium for a 5-min recovery. Oocytes were lysed and the chromosomes were fixed in a drop of hypotonic fix buffer (1% paraformaldehyde in H2O, 0.15% triton X-100, 3 mM dithiothreitol, pH 9.2) on a glass slide for 30 min in a drying oven at 37°C. The immunofluorescence process and image analysis were continued as described previously herein.
Microinjection and RNAi experiment
Fully grown oocytes of GV-stage were microinjected with non-targeting (control) or targeting small interfering RNA (siRNA) by a Diaphot ECLIPSE Ti-S inverted microscope (Japan, Nikon) equipped with MM-93B hydraulic three-dimensional (3-D) micromanipulators (USA, Narishige). Microinjected oocytes were cultured in M2 medium with 2.5 μM milrinone for 16 h to facilitate the targeting mRNA depletion. Next, the GV-stage oocytes were washed and cultured with fresh M2 medium. The siRNA sequences were as follows: Nedd8 (5ʹ-GAUUGAGAUAGACAUCGAATT-3ʹ, 5ʹ-UUCGAUGUCUAUCUCAAUCTT-3ʹ; China, GenePharma), Cullin1 (5ʹ-GCCGCAUGUAUAAUCUUGUTT-3ʹ, 5ʹ- ACAAGAUUAUACAUGCGGCTT-3ʹ; China, GenePharma).
3-D modeling
Oocytes were imaged using the Zeiss program as a z-series of 0.5-μm intervals to capture the entire spindle and chromosome structures based on three-dimensional confocal imaging. The Imaris program (USA, Bitplane) was used to further investigate oocyte meiosis status. Surface Creation Wizard was used to reconstruct Emi1 localization and chromosome status.
Statistical analysis
All experiments were repeated at least three times to ensure consistency. Means and standard deviations were plotted. Two-tailed Student’s t-test and ANOVA was performed for statistical analysis. The level of significance was accepted as p < 0.05.
Results
Expression and subcellular localization of nedd8 during mouse oocyte meiosis
To investigate the function of Nedd8 during oocyte meiosis, we first examined its expression level at each stage of oocyte maturation by dot blotting. Oocytes were collected after culture for 0, 2, 6, 8, and 12 h, corresponding to GV, GVBD, Pro-MI, MI, and MII stages, respectively. As shown in , Nedd8 was constantly expressed from GV to MII stages (p > 0.05; ). Furthermore, we examined the subcellular localization of Nedd8 by immunofluorescence staining. Results showed that in GV stage, Nedd8 mainly accumulated in the nucleus (), whereas during GVBD and Pro-MI stages, it was localized to the cytoplasm (). During the following MI and MII stages when spindles had been already assembled, Nedd8 was found to be distributed on the spindle (). These results suggested that proteins involved in MI and MII stages might exhibit Nedd8 modifications and that the neddylation pathway might play a potential role in oocyte maturation.
Figure 1. Expression and subcellular localization of Nedd8 during mouse oocyte meiosis.
(a) Mouse oocytes were collected after culture for 0, 2, 6, 8, and 12 h, corresponding to germinal vesicle (GV), GV breakdown (GVBD), prophase of metaphase I (pro-MI), metaphase I (MI) and metaphase II (MII) stages, respectively. Whole lysates from 30 oocytes were loaded in each lane for dot blotting. Nedd8 levels were determined using an anti-Nedd8 antibody. The relative staining intensity of Nedd8 was assessed by densitometry in the histogram. Error bars represent the standard deviation. (b-f) Oocytes of different stages were collected for immunofluorescence staining. Blue: DNA; Green: α-tubulin; Red: Nedd8. Scale bar, 10 μm.
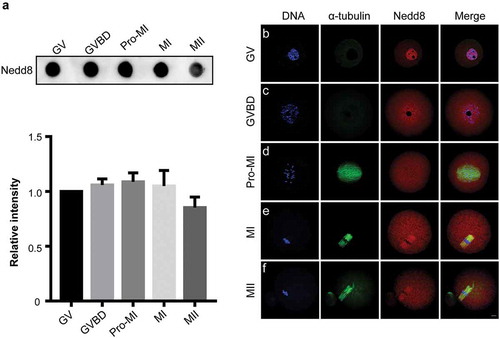
Inhibition of neddylation causes MI arrest and spindle disorder during oocyte maturation
To further investigate the role of neddylation during oocyte meiosis, we used MLN4924, a first-in-class NAE1 inhibitor [Citation24], to disrupt the Nedd8 pathway. Mouse oocytes were cultured with gradient concentrations (0, 0.1 μM, 0.5 μM, 1 μM and 5 μM) of MLN4924 for 12 h. Results showed that oocytes exhibited MI arrest and the polar body exclusion (PBE) rate dose-dependently decreased when the MLN4924 concentration was higher than 0.5 μM (47.75 ± 5.17%, 0.5 μM MLN4924 vs 80.49 ± 2.58%, control, p < 0.01; 8.52 ± 1.11%, 1 μM MLN4924 vs 80.49 ± 2.58%, control, p < 0.001; ). The inhibitory effect reached the maximum at 1 μM MLN4924, which is similar to that of 5 μM MLN4924 (8.52 ± 1.11%, 1 μM MLN4924 vs 7.11 ± 2.05%, 5 μM MLN4924, p > 0.05; ). Accordingly, the expression level of Nedd8 significantly decreased at the doses of 0.5 μM (0.56 ± 0.02 vs 1.03 ± 0.05, p < 0.001), 1 μM (0.29 ± 0.01 vs 1.03 ± 0.05, p < 0.001; ). Therefore, 1 μM MLN4924 was selected for subsequent study. To explore the subcellular oocyte phenotype after inhibition of neddylation, immunofluorescent staining was performed. Results revealed that in the MLN4924-treated group, the oocytes were arrested at the MI stage. Meanwhile, the spindle could not form bipolar structures and chromosomes could not segregate in the MI stage ().
Figure 2. Inhibition of neddylation causes oocyte MI arrest.
(a) Images of oocytes in the control group and MLN4924-treated groups. Oocytes were cultured in medium with different concentrations of MLN4924 (0.1 μM, 0.5 μM, 1 μM and 5 μM) or without MLN4924. Scale bar, 100 μm. (b) Germinal vesicle breakdown (GVBD) rate and polar body exclusion (PBE) rate of control and MLN4924-treated oocytes. Error bars represent the standard deviation. ns: no statistical significance, *p < 0.05, **p < 0.01, ***p < 0.001. (c-d) Expression of Nedd8 after treated with different concentrations of MLN4924. Oocytes were cultured at MI stage and total lysate of 80 oocytes per group was loaded for dot blotting and western blotting. Nedd8 levels were determined using an anti-Nedd8 antibody. β-actin was used as a loading control. Relative intensities of bands are shown in the histograms. Error bars represent the standard deviation. ns: No statistical significance, *p < 0.05, **p < 0.01, ***p < 0.001. (e) Spindle morphology in control and MLN4924-treated oocytes. Blue: DNA; Green: α-tubulin; Red: Nedd8. Scale bar, 10 μm.
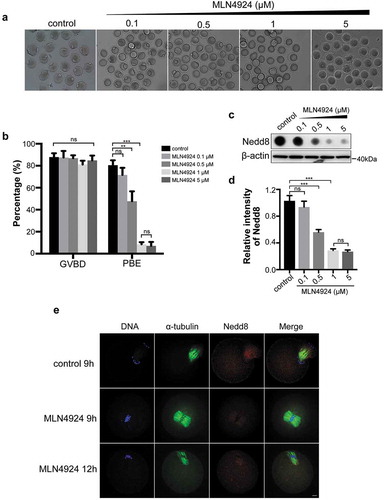
As Nedd8 is the most critical factor during neddylation process, siRNA mediated knockdown of Nedd8 was performed to verify the importance of neddylation during mouse oocyte maturation. Oocytes of Pro-MI stage were collected for dot blotting. The results confirmed an effective depletion of Nedd8 (). Control and Nedd8 RNAi mouse oocytes were cultured in vitro for 12 h. The results revealed that depletion of Nedd8 had no effect on the GVBD stage (87.53 ± 1.42% vs 82.55 ± 2.047%, p > 0.05; ), but induced a significant decrease of PBE rate (81.00 ± 2.34% vs 4.69 ± 1.74%, p < 0.001; ). Moreover, the immunofluorescent staining results showed that homologous chromosomes could not segregate and spindle failed to form bipolar structure in the Nedd8 RNAi oocytes (). All these results demonstrated that neddylation was critical for meiosis and that inhibiting the neddylation pathway caused MI arrest during oocyte maturation.
Figure 3. Depletion of Nedd8 blocks mouse oocytes maturation process.
(a-b) Control and Nedd8 RNAi oocytes were cultured to Pro-MI stage, and were collected for dot blotting assay and western blotting. β-actin was used as a loading control. Relative intensities of bands are shown in the histograms. Error bars represent the standard deviation. ***p < 0.001. (c) Images of control and Nedd8 RNAi oocytes. Mouse oocytes were cultured to MII stage. Scale bar, 100 μm. (d) GVBD rate and PBE rate of control and Nedd8 RNAi oocytes. Error bars represent the standard deviation. ns: no statistical significance, ns: no statistical significance. ***p < 0.001. (e) Spindle morphology of control and Nedd8 RNAi oocytes. Blue: DNA; Green: α-tubulin; Red: Nedd8. Scale bar, 10 μm.
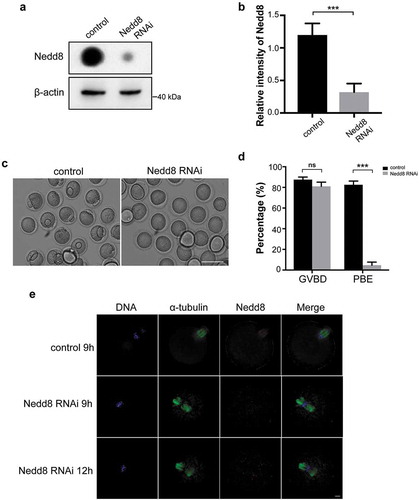
The SAC is over-activated upon neddylation inhibition
SAC proteins (such as Bub3, Mad2, and BubR1) function during the MI phase to ensure the accuracy of homologous chromosome segregation [Citation26,Citation27]. To analyze SAC status, we performed a chromosome spreading assay by chromosome immunofluorescence staining using anti-Bub3, anti-Mad2, and anti-BubR1 antibodies. In the control group, SAC was activated when the oocytes were cultured for 7 h, while the signal of SAC disappeared after 9 h culture (). However, when oocytes were cultured for 9 h with MLN4924, Bub3, Mad2, and BubR1 were still detected at the kinetochores of chromosomes (). Besides, the protein level of SAC proteins were similar between control and MLN4924-treated groups (). This indicates that impaired neddylation might affect chromosome segregation as manifested by the over-activation of SAC.
Figure 4. Inhibition of neddylation induces over-activation of the spindle assembly checkpoint (SAC).
Mouse oocytes were cultured in M2 medium for 7 h and 9 h, or in MLN4924 for 9 h, and then collected for chromosome spreading. (a-c) Immunofluorescence staining was performed after chromosome spreading. Core SAC proteins (Bub3, Mad2, and BubR1) were stained using specific primary and secondary antibodies. In control oocytes, the SAC was activated at 7 h, but the positive signal at kinetochores disappeared after MI at 9 h. However, they retained at kinetochores after MI in MLN4924-treated oocytes. Blue: DNA; Red: SAC core proteins. Scale bar, 10 μm. (d) Two hundred oocytes in each sample were lysed, and followed by western blotting for Bub3, Mad2, and BubR1 detection. β-actin was used as a loading control.
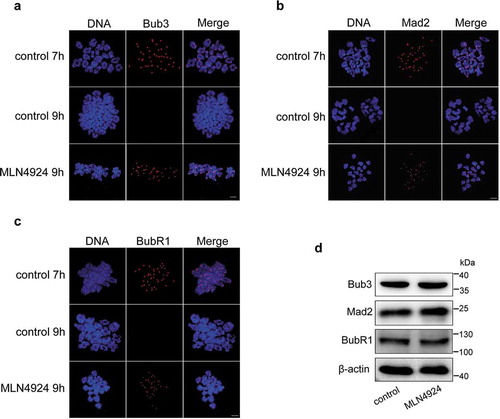
Cullin1 is the main substrate of Nedd8 in mouse oocytes
Since neddylation regulates the cellular biochemical functions through modulating the activity of its substrates, we investigated the substrates of Nedd8 in mouse oocytes [Citation22]. Cullins are the most important substrates of Nedd8 to date. To identify their expression in mouse oocytes, we measured the levels of eight cullin transcripts by qRT-PCR. Results showed that Cul1 was the most abundant cullin in mouse oocytes (1.63 ± 0.08; ). To demonstrate if Cullin1 has Nedd8 modification in mouse oocytes, we used specific Cullin1 antibody to examine its expression. The results confirmed that Cullin1 was modified by Nedd8 as evidenced by the higher molecular weight bands (). Moreover, a detection of Cullin1-Nedd8 complex by mass spectrum further confirmed this speculation (). In addition, the expression level of Cullin1-Nedd8 complex in each stage of oocyte meiosis was examined by western blotting. The results revealed that Nedd8 modified Cullin1 was continuously expressed during mouse oocyte (). As for the control and MLN4924 treated mouse oocytes, the expression of unmodified Cullin1 in both groups was similar (0.891 ± 0.02 vs 0.87 ± 0.02, p > 0.05; ), whereas the Cullin1-Nedd8 level decreased in the MLN4924-treated group (0.59 ± 0.02 vs 0.26 ± 0.02, p < 0.001; ). This indicated that Cullin1 might be the main substrate of Nedd8 in mouse oocytes. MLN4924 treatment suppressed the Nedd8 modification of Cullin1 and downregulated Cullin1 activity in oocytes.
Figure 5. Cullin1 neddylation decreased after neddylation inhibition.
(a) qRT-PCR was performed to examine the expressions of eight cullin transcripts in mouse oocytes (200 oocytes/sample). The relative expression levels are shown in the histograms. Error bars represent the standard deviation. (b-c) The expression of Cullin1 and Cullin1-Nedd8 during mouse oocyte maturation. β-actin was used as a loading control. Relative intensities of bands are shown in the histograms. Error bars represent the standard deviation. (d-e) Anti-Cullin1 antibody was used to examine Cullin1 and Cullin1-Nedd8 in the control and MLN4924-treated mouse oocytes. β-actin was used as a loading control. Relative intensities of bands are shown in the histograms. Error bars represent the standard deviation. ns: no statistical significance, ***p < 0.001. (f-g) Expression of Cullin1 in control and Cullin1 RNAi oocytes. β-actin was used as a loading control. Relative intensities of bands are shown in the histograms. Error bars represent the standard deviation. ***p < 0.001. (h-i) Images of control and Cullin1 RNAi oocytes after cultured for 12 h. GVBD and PBE rates are shown in the histograms. Error bars represent the standard deviation. ns: no statistical significance, ***p < 0.001. Scale bar, 100 μm.
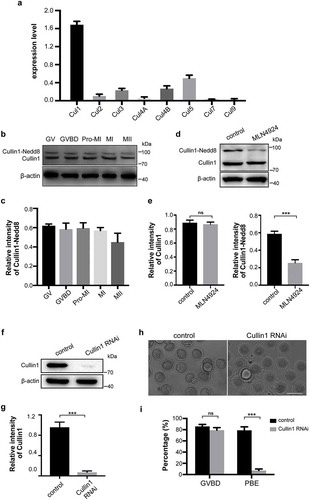
To gain insight into the importance of Cullin1 during mouse oocyte meiosis, gene-targeting siRNA microinjection was performed to knockdown Cullin1 in oocytes. Western blotting showed a successful depletion of Cullin1 (0.96 ± 0.06 vs 0.07 ± 0.02, p < 0.001; ). Furthermore, we cultured the control and Cullin1 RNAi oocytes in vitro for 12 h to investigate the maturation status in both groups. Results confirmed that the PBE rate of Cullin1 RNAi oocytes was much lower than that of control groups (80.24 ± 2.37 vs 7.17 ± 1.52, p < 0.001; ). In conclusion, as one of the most critical substrates of Nedd8, Cullin1 involves in the oocyte maturation process. When Cullin1 is depleted, oocyte meiosis is arrested at MI stage. This phenotype is consistent with that of neddylation inhibition.
Inhibition of neddylation increases the expression of Emi1
Emi1 is a known substrate of Cullin1 [Citation28] and plays a critical role in the cell cycle progression of somatic cells [Citation29]. To investigate the role of Emi1 in oocyte meiosis, we performed immunofluorescence assays and western blotting to explore its subcellular localization and expression changes when the Nedd8 pathway was inhibited. The immunofluorescence results showed that Emi1 mainly localized to the mouse oocyte spindle (). This result implied that Emil may play similar function in oocytes as in somatic cells. Upon treatment with MLN4924, the Emil expression appeared to be stronger than that in the control group (). Western blotting results further confirmed that the expression level of Emi1 increased after treated with MLN4924 (0.81 ± 0.05, n = 3 vs 0.54 ± 0.04, n = 3, p < 0.01; ). Similarly, the expression of Emi1 increased in both Nedd8 RNAi oocytes (0.81 ± 0.03, n = 3 vs 0.54 ± 0.04, n = 3, p < 0.01) and Cullin1 RNAi oocytes (0.85 ± 0.03 vs 0.54 ± 0.04, n = 3, p < 0.01; ). These results implied that Emi1 might be regulated by neddylation.
Figure 6. Emi1 is a potential substrate of neddylation-mediated ubiquitination.
(a) Control and MLN4924-treated mouse oocytes were cultured and collected at MI stage for immunofluorescence staining. Blue: DNA; Green: α-tubulin; Red: Emi1. Scale bar, 10 μm. (b) 3-D modeling of control and MLN4924-treated oocytes. 3-D models were constructed using the Imaris program. Isosurface renderings of the oocytes were created and rotated in 3-D space to assess morphology. (c-f) Western blotting detection of Emi1 and Fzr1 protein levels in control, Nedd8 RNAi and Cullin1 RNAi oocytes. β-actin was used as loading control. Relative intensities of bands are shown as bar graph in the histograms. Error bars represent the standard deviation. *p < 0.05, ***p < 0.001.
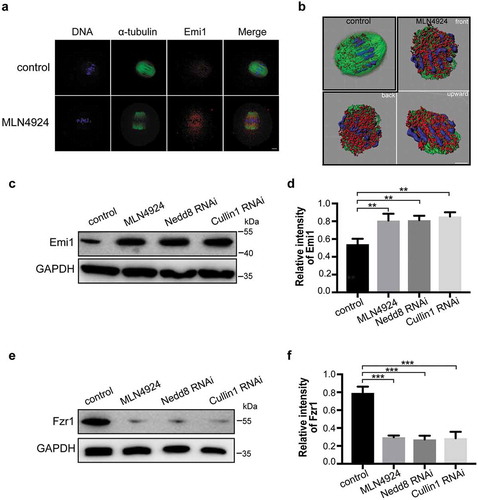
Since Emi1 is an inhibitor of APC/CFzr1 [Citation30] in which Fzr1 is a crucial activator of APC/C and forms a complex with it [Citation31], we tested the expression of Fzr1. To examine if the expression level of Fzr1 could be affected by Nedd8 pathway inhibition, we performed western blotting to detect its protein level. Results showed that compared to the control group, the expression of Fzr1 was decreased in MLN4924-treated group (0.30 ± 0.01, n = 3 vs 0.79 ± 0.04, n = 3, p < 0.001; ), Nedd8 RNAi (0.27 ± 0.02, n = 3 vs 0.79 ± 0.04, n = 3, p < 0.001) and Cullin1 RNAi groups (0.29 ± 0.04, n = 3 vs 0.79 ± 0.04, n = 3, p < 0.001; ). These results suggested that neddylation is essential for APC/C activation. Thus, we concluded that when neddylation was inhibited, the expression of Emi1 was increased and that APC/CFzr1 was inhibited, resulting in MI arrest in oocytes.
Discussion
Stringent regulation is indispensable for correct mouse oocyte meiosis otherwise it is highly error-prone [Citation6,Citation32,Citation33]. Since transcription does not occur during oocyte meiosis, PTMs appear to be crucial. As a ubiquitin-like PTM, neddylation is implicated in many biophysical regulatory processes such as autophagy, cell proliferation and signal transduction [Citation34–Citation36]. It is a process in which Nedd8 covalently binds to its substrates. Neddylation regulates the activity of its substrates rather than their abundance [Citation20]. Cullins are the most important substrates of Nedd8 and are essential for G1 to S cell cycle transition [Citation21,Citation37]. Moreover, they comprise a family of proteins containing a conserved Cullin domain that can be modified by Nedd8 [Citation19]. In this manner, neddylation regulates the activity of cullin proteins and activates the subsequent ubiquitin transfer [Citation38]. Thus, Cullin1 neddylation regulates the degradation of a set of proteins through the Cullin1-RING type E3 ligases [Citation19]. However, whether this process functions during oocyte meiosis has not been well studied. In this study, we first identified that Nedd8 was constitutively expressed from GV to MII stages. In addition, Nedd8 localized to nucleus during the GV stage and translocated to the cytoplasm during GVBD and Pro-MI stages, after which it finally localized to the spindle. This suggested that proteins involved in spindle assembly and meiosis might be modified by Nedd8.
To investigate how neddylation affects meiosis, we treated oocytes with MLN4924, which is an adenosine sulfamate analogue that can form a covalent adduct with Nedd8, thereby suppressing the Nedd8-AMP step and selectively locking NAE1 into an inactive state [Citation24,Citation39]. Moreover, it has anti-tumor activity and is widely used for the treatment of many types of cancers [Citation39,Citation40]. Using MLN4924 or Nedd8 siRNA to inhibit neddylation, we revealed that oocytes arrested at the MI stage, exhibiting disorders in spindle assembly and chromosome segregation. Thus, we showed for the first time that neddylation was essential for oocyte maturation. As previously mentioned, cullins are core substrates of Nedd8 [Citation21]. Given that eight members of the cullin family exist in cells, we performed qRT-PCR and identified Cullin1 as the major cullin member expressed in mouse oocytes. Since Cullin1 neddylation is required for the activation of CRL E3 ubiquitination ligase, we examined the Cullin1 neddylation status using the specific anti-Cullin1 antibody. In the Cullin1 western blotting, we confirmed that Cullin1 was modified by Nedd8 as evidenced by the two bands representing Cullin1 and Cullin1-Nedd8 respectively. Moreover, there was no significant difference in the Cullin1 expression in either groups. However, compared to the MLN4924-treated group, the level of Cullin1-Nedd8 decreased. These results verified that Cullin1 was the major neddylation substrate in mouse oocytes and when neddylation was inhibited, Nedd8 modified Cullin1 diminished. In this manner, neddylation could further regulate the following ubiquitination mediated by Cullin1 in mouse oocytes.
Based on our hypothesis, the inhibition of neddylation might suppress the degradation of Cullin1 substrates. Emi1, also known as Fbxo5, is a substrate of Cullin1 [Citation28] and an inhibitor of APC/C [Citation41–Citation43]. In contrast, Fzr1 acts as an activator of APC/C and forms a complex with APC/C. During the cell cycle, APC/C can be activated to ensure that cells can exit metaphase [Citation44,Citation45]. Previous studies have shown that Emi1 inactivates APC/C by competing with APC/C for Fzr1 binding, thereby stabilizing APC/C substrates [Citation41,Citation46]. When APC/CFzr1 is completely inhibited, cells irreversibly revert to a quiescent state [Citation47]. However, it is still unclear whether and how Emi1 regulates meiosis through neddylation. First, we identified that Emi1 was mainly localized to the spindle of mouse oocytes. It implied that Emi1 might function in spindle assembly. In addition, we examined the expression of Emi1 in the control group and the MLN4924-treated group. In well accordance with previous data, we found that the inhibition of neddylation suppressed the activity of Cullin1, following the increased expression level of Emi1, leading to APC/CFzr1 inactivation and subsequent MI arrest. Moreover, the spindle could not form bipolar structures and chromosomes were not segregated. Consistent with our hypothesis, a pathway underlying the regulation of oocyte maturation by neddylation is presented.
Taken together, this study identified for the first time that neddylation plays a key role in mouse oocyte maturation. We propose a model wherein Cullin1 neddylation regulates oocyte meiosis. As a substrate of Cullin1 mediated ubiquitin E3 ligase, Emi1 is possibly ubiquitinated and downregulated to allow oocyte meiosis by activating APC/CFzr1. When neddylation is selectively inhibited, Emi1 upregulation blocks the oocyte meiosis. In this manner, oocyte meiosis is disturbed, manifesting as MI arrest, a deficiency of chromosome segregation, and spindle disorders. Errors in meiosis are a major cause of female infertility and in vitro fertilization failure. Accordingly, this study might provide a potential marker for the diagnosis of infertility and offer new clues for the development of novel therapeutic strategies.
Supplemental Material
Download Zip (558.7 KB)Acknowledgments
We are grateful to Dr. Bingteng Xie for the insightful comments and suggestions on the manuscript.
Disclosure statement
The authors declare that they have no competing interests.
Supplementary material
Supplemental data for this article can be accessed here.
Additional information
Funding
References
- Himmel W, Ittner E, Kochen MM, et al. Management of involuntary childlessness. Br J Gen Pract. 1997 Feb;47(415):111–118. PMID: 9101672.
- Tripathi A, Kumar KV, Chaube SK. Meiotic cell cycle arrest in mammalian oocytes. J Cell Physiol. 2010 Jun;223(3):592–600. PMID: 20232297.
- Jaffe LA, Egbert JR. Regulation of mammalian oocyte meiosis by intercellular communication within the ovarian follicle. Annu Rev Physiol. 2017 Feb 10;79:237–260. PMID: 27860834.
- Chen L, Yin T, Nie ZW, et al. Survivin regulates chromosome segregation by modulating the phosphorylation of Aurora B during porcine oocyte meiosis. Cell Cycle (Georgetown, Tex). 2018;17(21–22):2436–2446. PMID: 30382773.
- Engmann L, Romak J, Nulsen J, et al. In vitro viability and secretory capacity of human luteinized granulosa cells after gonadotropin-releasing hormone agonist trigger of oocyte maturation. Fertil Steril. 2011 Jul;96(1):198–202. PMID: 21601197.
- Baumann C, Wang X, Yang L, et al. Error-prone meiotic division and subfertility in mice with oocyte-conditional knockdown of pericentrin. J Cell Sci. 2017 Apr 1;130(7):1251–1262. PMID: 28193732.
- Chen J, Melton C, Suh N, et al. Genome-wide analysis of translation reveals a critical role for deleted in azoospermia-like (Dazl) at the oocyte-to-zygote transition. Genes Dev. 2011 Apr 1;25(7):755–766. PMID: 21460039.
- Goldstein G, Scheid M, Hammerling U, et al. Isolation of a polypeptide that has lymphocyte-differentiating properties and is probably represented universally in living cells. Proc Natl Acad Sci U S A. 1975 Jan;72(1):11–15. PMID: 1078892.
- Enchev RI, Schulman BA, Peter M. Protein neddylation: beyond cullin-RING ligases. Nat Rev Mol Cell Biol. 2015 Jan;16(1):30–44. PMID: 25531226.
- Herrmann J, Lerman LO, Lerman A. Ubiquitin and ubiquitin-like proteins in protein regulation. Circ Res. 2007 May 11;100(9):1276–1291. PMID: 17495234.
- Soucy TA, Dick LR, Smith PG, et al. The NEDD8 conjugation pathway and its relevance in cancer biology and therapy. Genes Cancer. 2010 Jul;1(7):708–716. PMID: 21779466.
- Brown JS, Jackson SP. Ubiquitylation, neddylation and the DNA damage response. Open Biol. 2015 Apr;5(4):150018. PMID: 25833379.
- Zhao Y, Morgan MA, Sun Y. Targeting neddylation pathways to inactivate cullin-RING ligases for anticancer therapy. Antioxid Redox Signal. 2014 Dec 10;21(17):2383–2400. PMID: 24410571.
- Whitby FG, Xia G, Pickart CM, et al. Crystal structure of the human ubiquitin-like protein NEDD8 and interactions with ubiquitin pathway enzymes. J Biol Chem. 1998 Dec 25;273(52):34983–34991. PMID: 9857030.
- Kumar S, Yoshida Y, Noda M. Cloning of a cDNA which encodes a novel ubiquitin-like protein. Biochem Biophys Res Commun. 1993 Aug 31;195(1):393–399. PMID: 8395831.
- Choi YS, Jeon YH, Ryu KS, et al. 60th residues of ubiquitin and Nedd8 are located out of E2-binding surfaces, but are important for K48 ubiquitin-linkage. FEBS Lett. 2009 Oct 20;583(20):3323–3328. PMID: 19782077.
- Kamitani T, Kito K, Nguyen HP, et al. Characterization of NEDD8, a developmentally down-regulated ubiquitin-like protein. J Biol Chem. 1997 Nov 7;272(45):28557–28562. PMID: 9353319.
- Hemelaar J, Borodovsky A, Kessler BM, et al. Specific and covalent targeting of conjugating and deconjugating enzymes of ubiquitin-like proteins. Mol Cell Biol. 2004 Jan;24(1):84–95. PMID: 14673145.
- Duda DM, Borg LA, Scott DC, et al. Structural insights into NEDD8 activation of cullin-RING ligases: conformational control of conjugation. Cell. 2008 Sep 19;134(6):995–1006. PMID: 18805092.
- Gao Q, Yu GY, Shi JY, et al. Neddylation pathway is up-regulated in human intrahepatic cholangiocarcinoma and serves as a potential therapeutic target. Oncotarget. 2014 Sep 15;5(17):7820–7832. PMID: 25229838.
- Schwechheimer C. NEDD8-its role in the regulation of Cullin-RING ligases. Curr Opin Plant Biol. 2018 Oct;45(Pt A):112–119. PMID: 29909289.
- Jones J, Wu K, Yang Y, et al. A targeted proteomic analysis of the ubiquitin-like modifier nedd8 and associated proteins. J Proteome Res. 2008 Mar;7(3):1274–1287. PMID: 18247557.
- Jiang H, He D, Xu H, et al. Cullin-1 promotes cell proliferation via cell cycle regulation and is a novel in prostate cancer. Int J Clin Exp Pathol. 2015;8(2):1575–1583. PMID: 25973042.
- Soucy TA, Smith PG, Milhollen MA, et al. An inhibitor of NEDD8-activating enzyme as a new approach to treat cancer. Nature. 2009 Apr 9;458(7239):732–736. PMID: 19360080.
- Hodges CA, Hunt PA. Simultaneous analysis of chromosomes and chromosome-associated proteins in mammalian oocytes and embryos. Chromosoma. 2002 Sep;111(3):165–169. PMID: 12355205.
- Tadros W, Lipshitz HD. The maternal-to-zygotic transition: a play in two acts. Development. 2009 Sep;136(18):3033–3042. PMID: 19700615.
- Li M, Li S, Yuan J, et al. Bub3 is a spindle assembly checkpoint protein regulating chromosome segregation during mouse oocyte meiosis. PloS One. 2009 Nov 2;4(11):e7701. PMID: 19888327.
- Emanuele MJ, Elia AE, Xu Q, et al. Global identification of modular cullin-RING ligase substrates. Cell. 2011 Oct 14;147(2):459–474. PMID: 21963094.
- Havens CG, Ho A, Yoshioka N, et al. Regulation of late G1/S phase transition and APC Cdh1 by reactive oxygen species. Mol Cell Biol. 2006 Jun;26(12):4701–4711. PMID: 16738333.
- Cappell SD, Mark KG, Garbett D, et al. EMI1 switches from being a substrate to an inhibitor of APC/C(CDH1) to start the cell cycle. Nature. 2018 Jun;558(7709):313–317. PMID: 29875408.
- Skaar JR, Pagano M. Cdh1: a master G0/G1 regulator. Nat Cell Biol. 2008 Jul;10(7):755–757. PMID: 18591966.
- Nagaoka SI, Hassold TJ, Hunt PA. Human aneuploidy: mechanisms and new insights into an age-old problem. Nat Rev Genet. 2012 Jun 18;13(7):493–504. PMID: 22705668.
- Jones KT, Lane SI. Molecular causes of aneuploidy in mammalian eggs. Development. 2013 Sep;140(18):3719–3730. PMID: 23981655.
- Lammer D, Mathias N, Laplaza JM, et al. Modification of yeast Cdc53p by the ubiquitin-related protein rub1p affects function of the SCFCdc4 complex. Genes Dev. 1998 Apr 1;12(7):914–926. PMID: 9531531.
- Lv Y, Li B, Han K, et al. The Nedd8-activating enzyme inhibitor MLN4924 suppresses colon cancer cell growth via triggering autophagy. Korean J Physiol Pharmacol. 2018 Nov;22(6):617–625. PMID: 30402022.
- Park SY, Park JW, Lee GW, et al. Inhibition of neddylation facilitates cell migration through enhanced phosphorylation of caveolin-1 in PC3 and U373MG cells. BMC Cancer. 2018 Jan 5;18(1):30. PMID: 29301501.
- Mathias N, Johnson SL, Winey M, et al. Cdc53p acts in concert with Cdc4p and Cdc34p to control the G1-to-S-phase transition and identifies a conserved family of proteins. Mol Cell Biol. 1996 Dec;16(12):6634–6643. PMID: 8943317.
- Boh BK, Smith PG, Hagen T. Neddylation-induced conformational control regulates cullin RING ligase activity in vivo. J Mol Biol. 2011 Jun 3;409(2):136–145. PMID: 21463634.
- Oladghaffari M, Shabestani Monfared A, Farajollahi A, et al. MLN4924 and 2DG combined treatment enhances the efficiency of radiotherapy in breast cancer cells. Int J Radiat Biol. 2017 Jun;93(6):590–599. PMID: 28291374.
- Yang D, Tan M, Wang G, et al. The p21-dependent radiosensitization of human breast cancer cells by MLN4924, an investigational inhibitor of NEDD8 activating enzyme. PloS One. 2012;7(3):e34079. PMID: 22457814.
- Vaidyanathan S, Cato K, Tang L, et al. In vivo overexpression of Emi1 promotes chromosome instability and tumorigenesis. Oncogene. 2016 Oct 13;35(41):5446–5455. PMID: 27065322.
- Reimann JD, Freed E, Hsu JY, et al. Emi1 is a mitotic regulator that interacts with Cdc20 and inhibits the anaphase promoting complex. Cell. 2001 Jun 1;105(5):645–655. PMID: 11389834.
- Di Fiore B, Pines J. Emi1 is needed to couple DNA replication with mitosis but does not regulate activation of the mitotic APC/C. J Cell Biol. 2007 May 7;177(3):425–437. PMID: 17485488.
- Peters JM. The anaphase promoting complex/cyclosome: a machine designed to destroy. Nat Rev Mol Cell Biol. 2006 Sep;7(9):644–656. PMID: 16896351.
- Wasch R, Engelbert D. Anaphase-promoting complex-dependent proteolysis of cell cycle regulators and genomic instability of cancer cells. Oncogene. 2005 Jan 6;24(1):1–10. PMID: 15637585.
- Teixeira LK, Reed SI. Ubiquitin ligases and cell cycle control. Annu Rev Biochem. 2013;82:387–414. PMID: 23495935.
- Cappell SD, Chung M, Jaimovich A, et al. Irreversible APC(Cdh1) inactivation underlies the point of no return for cell-cycle entry. Cell. 2016 Jun 30;166(1):167–180. PMID: 27368103.