ABSTRACT
Several studies have demonstrated the core circadian rhythm gene Bmal1 could regulate the clock control genes (CCGs) expression and maintain the integrity in cartilage tissue. In addition, its abnormal expression is connected with the occurrence and development of several diseases including osteoarthritis (OA). However, the relationship between Bmal1 and cartilage development still needs to be fully elucidated. Here, we bred tamoxifen-induced cartilage-specific knockout mice to learn the effects of Bmal1 on the cartilage development and its underlying mechanisms at specific time points. We observed that Bmal1 ablated mice showed growth retardation during puberty, and the length of whole growth plate and the proliferation zone were both shorter than those in the control group. Deletion of Bmal1 significantly inhibited the chondrocytes proliferation and activated cells apoptosis in the growth plate. Meanwhile, knockout of Bmal1 attenuated the expression of VEGF and HIF1α and enhanced the level of MMP13 and Runx2 in the growth plate chondrocytes. Consistent with these findings in vivo, ablation of Bmal1 could also lead to decrease chondrocytes proliferation, the expression of HIF1α and VEGF and elevate apoptosis in cultured chondrocytes. These findings suggest that Bmal1 plays a pivotal role in cartilage development by regulating the HIF1α-VEGF signaling pathway.
1. Introduction
The formation of osseous tissue occurs via two distinct ways of endochondral ossification and intramembranous ossification during embryogenesis [Citation1]. Endochondral ossification is the main growth formation for limb bone, ribs and vertebrae through forms the growth plate cartilage. Under the well-ordered regulation of several factors, the chondrocytes gradually turn into hypertrophic chondrocytes from the resting and proliferating chondrocytes in the growth plate cartilage [Citation2]; meanwhile, the extracellular matrix secreted by chondrocytes also changes from Col2a to Col10a [Citation3]. Eventually, at the end of the growing period, with the invasion of the blood vessels, the tissues are transformed from the hypoxic and avascular cartilage tissue to the rich oxygen and vascular osseous tissue.
The circadian clock system is an evolved physiological function by organisms in order to adapt and synchronize the 24-h light and darkness changes in the environment [Citation4]. The exact clock system impacts on different physiological processes through rhythmically oscillatory expression of several core clock genes and furthermore to induce and change a large number of downstream CCGs expression level. In human and mammalian body, the circadian clock system is built on an intricate and hierarchical manner, and the phase information was transmitted from ocular photoreception to the suprachiasmatic nucleus (SCN) located at the hypothalamus and the signal next relayed to the rest of brain and peripheral tissues. In addition to the “master synchronizer”, there are also tissue-specific synchronizers of circadian rhythms [Citation5]. The clock system maintains and controls the circadian rhythm by a feedback loop: the BMAL1 interacts with CLOCK to form heterodimers and initiate the transcription of target genes, for example, Cry and Per and the resulting negative proteins (CRY and PER) further inhibit BMAL1 and CLOCK transcriptional activity [Citation6]. Under the regulation of circadian clock, many important physiological activities in body show obvious circadian rhythm; on the other side of the coin, accumulating studies have recently given evidence of the link of abnormal circadian clock system and severe diseases [Citation7].
As a conserved mediation process, mammals could synchronize the intricate circadian activity due to the similar cellular mechanism of circadian rhythm within an individual cell. Although the cartilage is comprised of unitary chondrocyte around extracellular matrix is an avascular and hypoxic tissue, it also displays the circadian rhythm through measurement the chondrocyte mitotic index [Citation8] and the serum levels of Col2a and cartilage oligomeric matrix protein (COMP) [Citation9,Citation10]. Numbers of work have indicated that the pivotal circadian clock gene indeed existed in cartilage tissue; furthermore, the time-series microarray analyses test revealed that more than 600 genes expressed in cartilage had periodic rhythmicity which were also controlled and influenced by clock genes [Citation11]. Through using the mouse model of osteoarthritis, a test revealed that in OA mice articular cartilage the expression level of many circadian clock proteins were observably influenced when compared with control mice and the mice with deletion of Bmal1 gene showed the progressive noninflammatory arthritis [Citation12]. Another study demonstrated that conditional knockout of Bmal1 in cartilage affected the integrity of the cartilage and induced the joint more prone to osteoarthritis [Citation13]. All the above studies indicate the close link of biological clock and cartilage tissue.
As a unique avascular and hypoxic tissue in body, the cartilage tissue requires various factors to regulate and maintain the normal process of development and metabolism in the harsh environment, for example, the hypoxia-inducible factors α (HIFα) family which consists HIF1α, HIF2α and HIF3α [Citation14,Citation15]. Many studies have proposed Bmal1 could not only regulate the expression of many CCGs but also interact with the HIF1α or HIF2α to mediate several key physiological functions [Citation16]. Some investigators have found that in mouse nucleus pulposus cells, Bmal1 gene controlled the transcriptional activity of HIF1α and once ablation it would lead to inhibit the growth of nucleus pulposus [Citation17]. Under the hypoxic condition, the expression of HIF1α in zebrafish also exhibited a periodic rhythm similarly as a clock control gene [Citation18]. In addition to the role of independent regulation of cell response to hypoxia, HIF1α and HIF2α also combine with hypoxia-responsive elements (HRE) to start downstream factors, for example, vascular endothelial growth factor (VEGF) transcriptional activity to impact a wide range of important physiological phenomena [Citation19–Citation21]. A number of studies have also shown that desynchronization by inhibiting the circadian clock genes could decrease the genes transcription level as to response to the hypoxia [Citation22].
Although there are many studies on the relationship between Bmal1 gene and osteoarthritis [Citation23], there is still little research on the effect of Bmal1 gene on the cartilage development and more detailed work is needed. By constructing a tamoxifen-induced, cartilage-specific Bmal1 knockout mice model, we hope to observe and elucidate whether and how Bmal1 affects the cartilage development at the specific time window. And in this study, we propose knockout Bmal1 inhibits growth plate chondrogenesis and this effect is associated with VEGF and HIF.
2. Materials and methods
2.1 Transgenic mice generation and tamoxifen injection
To generate the TamCartBmal1flox/flox mice, we firstly used the Bmal1flox/flox mouse which had two LoxP sites flanking of Bmal1 (a gift from professor Tong weimin of Institute of Basic Medical Sciences, Chinese Academy of Medical Sciences) bred with Col2α1-CreER transgenic mouse which encoded a fusion protein of the Cre recombinase induced by tamoxifen and regulated by the α1 (II) promoter for cartilage-specific expression (purchased from Jackson Laboratory, Bar Harbor, ME, USA; stock number 006774) to produce Col2α1;Bmal1flox/- mice. Then, Col2α1;Bmal1flox/- mice were mated with each other or Bmal1flox/flox mouse to generate TamCartBmal1flox/flox mice. To knock out the Bmal1 gene specific in TamCartBmal1flox/flox mice cartilage afterbirth, the mice were intraperitoneally injected with tamoxifen for four doses respectively at postnatal 5, 7, 9 and 11 days. In order to delete the Bmal1 during embryonic period, the pregnant female mice were given one dose of tamoxifen (1.5mg/mouse) at 13.5 days post-coitus. In this study, we refer to the TamCartBmal1−/- mice as BMAL1CKO mice and the Bmal1flox/flox control littermates as control mice.
2.2 Genotyping and determination of tissue-specific deletion of the Bmal1 gene
The specific genotyping for Cre and floxed-Bmal1 alleles was analyzed through amplified the total DNA extracting from tail (by the mouse direct PCR Kit from Biotool company) by the polymerase chain reaction (PCR) analyses with standard condition. The sequences for genotyping Bmal1flox/flox mice were ACT GGA AGT AAC TTT ATC AAA CTG and CTG ACC AAC TTG CTA ACA ATT A; a 327-bp PCR product and a 431-bp PCR product signified the wild-type (WT) and the homozygous Bmal1flox/flox mice, respectively, the heterozygous mice showed the above all products. The PCR primers CAC TGC GGG CTC TAC TTC AT and ACC AGC AGC ACT TTT GGA AG were used to detect a 358-bp product for the Cre transgene. Besides the mice tail, we also extracted and tested other tissues DNA in the same mouse using DNeasy Blood and Tissue Kit (Qiagen, Valencia, CA, USA; Cat.No. 69504) to verify the conditional knockout Bmal1 gene in the growth plate cartilage.
2.3 Animal care and administration
All animals used in this study were generated in a controlled environment in which the temperature maintained 22°C and humidity maintained 40–50% and to feed with food and water ad libitum. During the study, light was also administrated to keep light from 7 am to 7 pm and darkness for the other 12 h. The weight was measured every week from birth to 6 weeks. The length of body, tibia and femur were measured at indicated time points. This experiment was run in triplicate. All the animal experiments involved in our study were approved by the Animal Experiment Administration Committee of the Medicine of Xi’an Jiaotong University in Shaanxi, People’s Republic of China.
2.4 Immunohistochemistry and immunofluorescence staining
In order to observe and analyze the growth plate cartilage, the obtained tibias at indicated time points firstly fixed in 4% formalin followed decalcified in 0.5 M EDTA, pH 7.4. After embedded in paraffin, tibias were cut and then stained with hematoxylin and eosin. To detect the protein level in the growth plate, the paraffin sections were next immunostained. In brief, the sections were pretreated with trypsin (0.25%) for 30 min before treatment with 3% H2O2 for 15 min and blocked with 10% goat serum for 1 h at room temperature (RT). Afterward, the sections were stained with antibodies against BMAL1 (Santa Cruz, sc-365645), HIF-1α (Gene Tex, GTX127309), VEGF (Santa Cruz, sc-7269), MMP13 (Abcam, ab39012), Col10a (Abcam, ab49945) and Runx2 (Santa Cruz, sc-101145) overnight at 4°C. After incubated through secondary antibodies, the sections were colored by EnVision+System-HRP (DAB) kit and counterstained with hematoxylin. For immunofluorescence, species-matched Alexa Fluor 488-labelled secondary antibody was used (1:400 in 1% BSA, 1 h) at 37°C and sections were mounted with DAPI before imaging.
2.5 Chondrocyte primary culture and plasmid infection
The cartilage of the knee and rib was isolated from BMAL1CKO and control mouse embryos used a stereo light microscope. After removed skin, fat and muscle around cartilage, put cartilage tissue into 0.2% collagenase (Sigma) for 40 min followed in 0.05% collagenase for 1.5 h to digest thoroughly. To remove the impurity, the cell suspension was filtered by a 70-μm filtering membrane and rinsed several times in PBS and serum-free DMEM. Primary chondrocytes were cultured as described previously (Xiaomin Kang and Wei Yang, 2017). The primary chondrocytes were transfected with the overexpression plasmid of Bmal1 (Genechem technology Inc) by Lipofectamine2000 (Invitrogen, USA) according to the manufacturer’s instructions. For in vitro hypoxia experiments, we placed primary chondrocytes in Triple gas incubator at 37°C for indicated time under condition of 1% oxygen tension supplemented with 5% CO2.
2.6 siRNA transfections
Chondrocytes were transfected with validated siRNA targeting Bmal1 by Lipofectamine 2000 (Invitrogen) according to the procedure recommended by the manufacturer. The cell transfection happened in serum-free DMEM (Invitrogen) and after 4 h the medium was removed and replaced with DMEM containing 10% FBS. And the transfection result was tested by Western blot or qPCR 72 h after transfection.
2.7 Western blotting
The extracts from chondrocyte or cartilage growth plate disrupted by RIPA buffer were through electrophoresis on a polyacrylamide gel containing SDS. The separated protein then was transferred to polyvinylidene difluoride membranes (Millipore, Billerica, MA, USA). The blots were subjected to block within skimmed milk and probed with designated primary antibodies overnight at 4°C. The membranes were then incubated with horseradish peroxidase-conjugated secondary antibody for 1 h and rinsed using enhanced chemiluminescence (ECL) reagents. The gray value of each band was tested by Image J software.
2.8 RNA extraction and quantitative real-time PCR analysis
The cartilage and chondrocytes RNA were extracted by Trizol reagent (Invitrogen; Cat.No. 15596-026) according to the manufacturer’s instruction and followed reverse transcription using RevertAid First Strand cDNA Synthesis kit (Thermo Fisher Scientific, Waltham, MA, USA; Cat.No. K1621). The cDNA products then subjected qPCR by SYBR Premix Ex Taq II (Takara Bio, Otsu, Japan; Cat.No. RR047A) used an MJ Mini Personal Thermal Cycler. mRNA expression was quantitated using the 2−△△ct method and normalized to transcripts for the indicated housekeeper.
2.9 BrdU incorporation assay
As an intuitionistic assay for chondrocyte proliferation, BrdU test was administrated in this study. Mice were injected with BrdU (Invitrogen; Cat. No. 00-0103) 1 ml per 100 g body weight intraperitoneally, followed euthanasia after 2 or 48 h. Through fixed, decalcified and embedded, the tibias were finally made and cut 4-6-μm-thick longitudinal paraffin sections. The BrdU-positive cells in the tissue sections were observed by immunohistochemistry using a BrdU staining kit (Invitrogen; Cat. No. 933943). In order to test and analyze the cell proliferation in vitro, the BrdU labeling medium was added in the cell culture medium and followed incubated for 1 h; the cell was then administrated by a BrdU labeling and detection kit II (Roche; Cat. No. 11299964001). The measure was completed by a researcher blinded to the treatment category.
2.10 TUNEL assay
For cartilage tissue sections and primary chondrocytes, we detected in situ apoptosis using In Situ Cell Death Detection Kit (Roche Diagnostics, Mannheim, Germany; Cat.No. 12156792910) in accordance with the manufacturer’s instructions. In brief, the tissue sections and chondrocytes were fixed, permeated, TUNEL reaction mixture labeled and finally observed by fluorescence microscopy. The nucleus was stained by DAPI and the number of positive cells and total cells in a certain area were calculated for quantitative analysis. The final result was obtained from the average of three independent experiments. The number of cells was counted by an experimenter who was not clear the treatment category.
2.11 Statistical analysis
The differences between groups were checked using the two-tailed Student’s t tests and ANOVA method by SPSS17.0 software. P < 0.05 was considered statistically significant. Data were collected from three independent experiments.
3. Results
3.1 The Bmal1 gene was specifically deleted in cartilage tissue
To validate the specificity of Bmal1 gene excision in the mice chondrocytes, PCR analyses, immunohistochemical staining and Western blot were performed at the indicated time points. As shown in , the gene excision (△-bmal1) was only observed in the growth plate cartilage from BMAL1CKO mice but did not occur in other tissues in the same BMAL1CKO mice or the control mice. Bmal1 mRNA and protein levels were significantly reduced in BMAL1CKO mice (4 weeks old) by PCR ()) and Western blot ()) test, respectively. In addition, immunohistochemical staining showed that BMAL1 expression was decreased in growth plate chondrocytes of BMAL1CKO mice at 6 weeks old ()).
Figure 1. Deletion of Bmal1 in the postnatal stage. (a) The genomic DNA extracted from different tissues of BMAL1CKO mice and control littermates was analyzed by PCR as described in “Materials and Methods”. (b) The total RNA was extracted from the growth plate and liver of BMAL1flox/flox and BMAL1CKO mice at 4 weeks and the relative expression of Bmal1 mRNA was detected by PCR (n= 5/group). Results are presented as gene expression levels in all groups normalized to controls. (c) The total protein was extracted from the BMAL1flox/flox and BMAL1CKO mice growth plate at 4 weeks and tested by Western blot (n = 3/group). A representative blot from three independent experiments was presented and quantification of protein expression of BMAL1 was shown on the right. BMAL1 protein level was normalized to GAPDH. (d) Immunohistochemistry was used to analyze the expression of BMAL1 in tibia growth plate of BMAL1flox/flox and BMAL1CKO mice at 6 weeks. Scale bars = 50μm. Data are expressed as means ± SE in each bar graph. *p < 0.05, **p < 0.01.
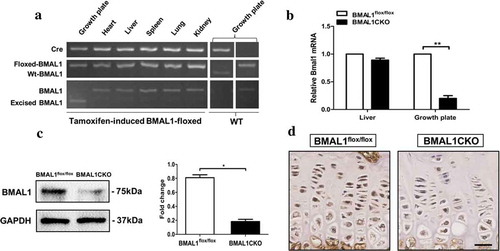
3.2 Rhythmic gene expression in the growth plate cartilage
Since Bmal1 is a core molecular circadian oscillator to initiate the clock feedback circuit in mammals, we next investigated whether conditional disruption of Bmal1 on growth plate cartilage intervened the physiological circadian clockwork. As shown in ), qPCR results revealed that the expression of Bmal1 and the negative transcriptional and post-translational feedback loop (TTL) component Cry1 and Per1 varied circadian rhythmically over zeitgebers (ZT) time in control mice, while ablation of Bmal1 led to increased expression of Cry1 mRNA in growth plate; in addition, the normal circadian expression of Cry1 and Per1 was also inhibited in BMAL1CKO mice. Besides the expression of rhythmic clock genes, we also determined whether loss of Bmal1 induced an altered expression of several marker genes of cartilage by qPCR and Western blot. The results presented in (a) showed that in the control mice the expression of HIF1α, similar to VEGF and Runx2, was demonstrated a fluctuation during the day; however, in BMAL1CKO mice, the expression level of HIF1α and VEGF was dampened and the expression of Runx2 was increased. And the expression fluctuation of all above factors was not obvious, suggesting that deletion of Bmal1 in cartilage could influence not only the other core clock genes but also several key genes associated with cartilage development. As expected, the protein level of BMAL1 was significantly decreased in cartilage of BMAL1CKO mice and its expression was not rhythmic in control mice ((b)). Consistent with the results shown by qPCR test, the protein expression level of HIF1α and VEGF in mutant mice was obviously decreased ((b)). And MMP13 expression exhibited a similar rhythmicity in the growth plate of control and BMAL1CKO mice although its expression level was up-regulated in mutant mice ((b)).
Figure 2. Cartilage-specific ablation of bmal1 disrupted molecular rhythms and several genes associated with normal cartilage development. (a) The total RNA was extracted from the growth plate of BMAL1flox/flox and BMAL1CKO mice at different time points and the relative expression of mRNA was detected by qPCR (n= 3 for each time point and group). Relative expressions were normalized to GAPDH. (b) Western blot was performed to analyze the clock genes and cartilage-related factors expression in growth plate from the BMAL1CKO mice and the BMAL1flox/flox littermates at 4-h intervals for 24 h and quantification of protein expression were also shown. The clock and cartilage-related protein level was normalized to GAPDH. Data are expressed as means ± SE in each bar graph. *p < 0.05.
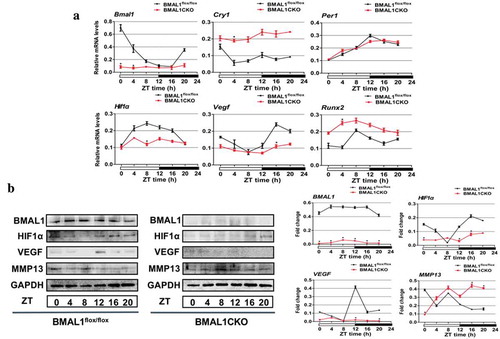
3.3 Ablation of Bmal1 during embryogenesis did not affect long bone growth
To study the possible role of Bmal1 gene on cartilage development during the embryonic period, we firstly intraperitoneal injected 1.5 mg tamoxifen for pregnant mouse at embryonic 13.5 day (E13.5) and harvested the embryos at E18.5 (supplemental Fig. 1A).
There was no significant difference observed between BMAL1CKO and control littermates with respect to the length of body, femur, tibia, humerus and vertebra (L1 to L5) (supplemental Fig. 1B,C). In addition, no obvious morphological abnormalities were found in BMAL1CKO embryo as assessed by HE staining as well as by immunohistochemistry for type X collagen (Col10a1) (supplemental Fig. 1D-F).
3.4 Effects of Bmal1 ablation on endochondral bone development afterbirth
We then determined whether loss of Bmal1 influenced postnatal bone and cartilage development. To knock out the Bmal1 gene specific in TamCartBmal1flox/flox mice growth plate cartilage postnatally, four doses tamoxifen (0.2 mg/mouse) were administrated intraperitoneally at 5, 7, 9, 11 days afterbirth and the mice were harvested at 0, 14, 28 and 42 days (). BMAL1CKO mice were born at the Mendelian ratio and no differences were observed between BMAL1CKO and control mice in the body length, femur and tibia length at 0 day and 2 week of age (data not shown). No significant difference was observed in body weight between BMAL1CKO and control mice (supplemental Fig. 1G) throughout the study period. However, from 4 weeks of age, the body length, femur and tibia length of the BMAL1CKO mice were shorter than those of control littermate which suggested the Bmal1 gene was required for normal endochondral bone growth (). Morphological analysis revealed a shorter growth plate in BMAL1CKO mice than that of control littermate concomitant with a narrower proliferative zone as well as an expanded hypertrophic zone at 4 and 6 weeks of age which implied that ablation of Bmal1 affected the normal chondrogenesis ().
Figure 3. Deletion of Bmal1 in the postnatal stage affected the endochondral bone formation in BMAL1CKO mice. The TamCartBmal1flox/flox mice were generated and in which Bmal1 gene was conditional ablated in growth plate chondrocytes by injection of tamoxifen intraperitoneally. (a) A schematic model depicting tamoxifen administration schedule postnatally. The blue arrows indicated the time points of tamoxifen administration at P5, P7, P9 and P11. The red arrows indicated the harvest time at P0, P14, P28 and P42. (b) Femur and tibia from BMAL1flox/flox and BMAL1CKO mice at 4 or 6 weeks of age. (c–e) The body length, femur length and tibia length were analyzed at indicated time points and the effect of Bmal1 ablation in the postnatal stage was observed at 4-week-old and 6-week-old BMAL1CKO mice (n= 10/group). Histological examination of tibia growth plate of BMAL1flox/flox and BMAL1CKO mice at 4weeks and 6 weeks of age (f) and the growth plate RZ, PZ and HZ heights were quantitatively analyzed, respectively (g and h) (n= 10/group). Scale bars = 100μm. (i) Percentage of the length of RZ (red), PZ (green) and HZ (blue) over the total length of tibia growth plate of the BMAL1flox/flox and BMAL1CKO mice at 4weeks and 6 weeks of age. Data are expressed as means ± SE in each bar graph. *p < 0.05. RZ = resting zone; PZ = proliferative zone; HZ = hypertrophic zone.
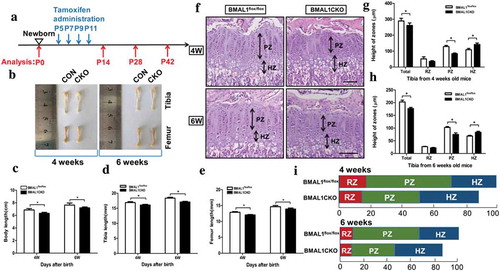
3.5. Effect of Bmal1 ablation on chondrocyte proliferation, differentiation and apoptosis in the growth plate
To gain insights into how Bmal1 ablation influenced endochondral bone growth, we analyzed the chondrocyte proliferation, differentiation and apoptosis in BMAL1CKO and control mice. The number of Bromo-2-deoxyUridine (BrdU)-positive cells was significantly reduced in the proliferative zone of growth plate in BMAL1CKO mice ()). In addition, we administrated mice with a single intraperitoneal injection of BrdU and harvested their tibias 48 h after injection. It was shown that in control mice the BrdU-labelled cells were not only located in the proliferative zone of growth plate but also remained in the hypertrophic zone, while in BMAL1CKO mice it could just be seen significantly less BrdU-labelled cells in proliferative zone and none was found in the hypertrophic zone (). To evaluate whether differentiation of chondrocytes was altered within the growth plate, we next examined the protein and mRNA expression of Col10a which was specifically secreted by hypertrophic chondrocytes by immunohistochemical staining and qPCR. As expected, the Col10a staining in growth plate was observed exclusively in the hypertrophic zone and such expression was clearly decreased in BMAL1CKO mice compared with the control mice at 4 weeks and 6 weeks of age (). Consistent with the immunohistochemistry finding, a similar result shown by the qPCR test confirmed the inhibitory effect in growth plate (). As a continuous process, chondrocytes undergo proliferation, differentiation and apoptosis and finally finish the physiological change from cartilage tissue to bone tissue, so we next evaluated whether deletion of Bmal1 affected the chondrocytes apoptosis. As shown in and g), conditional knockout of Bmal1 induced more TUNEL-positive cells in BMAL1CKO mice growth plate than that in the control group at 4 weeks and 6 weeks of age, respectively. Upregulation of caspase-3 and downregulation of Bcl-2 further implied the induced chondrocytes apoptosis in BMAL1CKO mice ()).
Figure 4. Bmal1 ablation affected chondrocytes proliferation, differentiation and apoptosis. Representative images of BrdU immunohistochemical analysis of tibia growth plate from BMAL1flox/flox and BMAL1CKO mice at 4-week-old after 2 h (a) and 48 h (b) of BrdU assay. BrdU-positive cells were stained by brown color (indicated by the arrow). Scale bars = 100μm. Boxed areas on the right represent higher magnification. Scale bars = 50μm. (c) The number of BrdU positive cells quantitatively (n= 5/group). (d) Immunohistochemical analysis of Col10a expression in BMAL1flox/flox and BMAL1CKO mice tibia tissue at 4-week and 6-week (n= 5/group). Scale bars = 100μm. (e) Col10a mRNA in growth plate cartilage of BMAL1flox/flox and BMAL1CKO mice was tested by qPCR (n= 5/group). (f) Representative images of the TUNEL-positive cells in tibial growth plate at 4-week-old and 6-week-old BMAL1flox/flox and BMAL1CKO mice. TUNEL positive cells were labeled red fluorescence. Scale bars = 100μm. (g) Quantitatively analyzed the number of TUNEL-positive cells, respectively (n= 5/group). (h) Western blot analysis showing the expression of Bcl-2 and Caspase-3 in tibia growth plate of BMAL1flox/flox and BMAL1CKO mice (n= 3/group). Data are expressed as means ± SE in each bar graph. *p < 0.05.
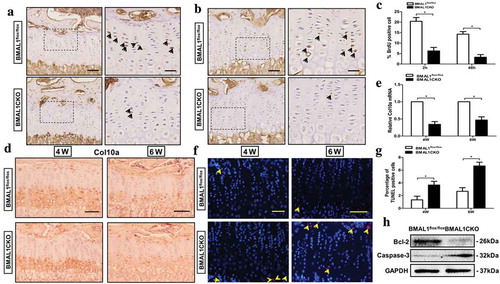
3.6. Deletion of Bmal1 led to the VEGF, HIF1α and other key factors expression change in growth plate chondrocytes
Given that lacking VEGF led to an enlargement of hypertrophic zone of the growth plate and finally affected the normal endochondral bone development [Citation24,Citation25], we then determined whether Bmal1 ablation induced the alteration of VEGF expression in the growth plate. As shown in and b), immunofluorescence staining analysis revealed that in BMAL1CKO growth plate chondrocytes, the VEGF expression was clearly decreased compared to the control group at 4 and 6 weeks age. Next, we tested the VEGF expression in cartilage by IHC, Western blot and qPCR and all of the assays determined an inhibitory effect on VEGF in BMAL1CKO mice growth plate (–f)). These findings were reminiscent of the previous study and observation that Bmal1 could interact with hypoxia-inducible transcription factor (HIF) [Citation16] and the HIF1α played a core controller for the chondrocytes to adapt the changing oxygen circumstance by producing several factors, for example, VEGF in the growth plate [Citation26]. Thus, we evaluated the expression of HIF1α in the growth plate, and the results showed that compared with the control group the HIF1α expression in BMAL1CKO mice decreased markedly (–f)). In addition, we observed that MMP13 and Runx2 mRNA and protein level were significantly activated in the growth plate in BMAL1CKO mice compared with the control mice (–f)).
Figure 5. Deletion of Bmal1 Led to the alteration of VEGF, HIF1α and Several Factors Expression in the Growth Plate Cartilage. (a) VEGF immunofluorescence in longitudinal sections of BMAL1flox/flox and BMAL1CKO mice tibias at 4-week-old and 6-week-old. VEGF, green; DNA, blue. Scale bars = 100μm. (b) Quantitatively analyzed the number of VEGF-positive cells, respectively (n= 4/group). (c) Immunohistochemical analysis of MMP13, Runx2, VEGF and HIF1α expression in BMAL1flox/flox and BMAL1CKO mice tibia tissue at 4-week age mice (n= 5/group). Scale bars = 100μm. (d) Quantitatively analyzed the number of IHC-positive cells, respectively (n= 4/group). (e) Western blot analyzed the protein expression of Runx2, MMP13, VEGF and HIF1α in cartilage tissues harvested from 4-week-old and 6-week-old BMAL1flox/flox and BMAL1CKO mice. (f) Relative expression of Runx2, MMP13, VEGF and HIF1α mRNA in the growth plate of BMAL1flox/flox and BMAL1CKO mice at 4-week-old and 6-week-old was detected by qPCR (n= 5/group). Results were presented as gene expression levels in all groups normalized to controls. Data are expressed as means ± SE in each bar graph. *p < 0.05.
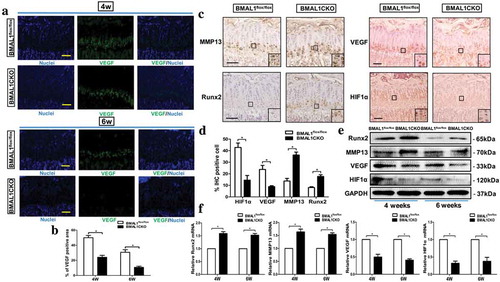
3.7. Rhythmic gene expression in the cultured primary chondrocytes
To evaluate whether and how ablation of Bmal1 affected the circadian rhythmicity of other proteins in vitro, we analyzed rhythmic expression in primary cultured chondrocytes isolated from control or BMAL1CKO mice exposed to 100μM dexamethasone (Dex) for 2 h to synchronize different cellular stages during culture. As shown in the supplemental Fig. 2A and B), the expression of BMAL1 was no significant rhythmic either in the control or in BMAL1CKO chondrocytes. The blot showed that the expression profiles of HIF1α exhibited the significant rhythmicity with maximum expression at ZT0 and the minimum expression at ZT4 in control chondrocyte. Likewise, the expression of HIF1α was reduced and a loss the rhythmicity was observed in the chondrocytes extracted from BMAL1CKO mice (similarly found in the growth plate as shown the )). Runx2 and MMP13 showed the rhythmic expression both in control and BMAL1CKO chondrocytes with the MMP13 expression being elevated (supplemental Fig. 2A and B). The above results demonstrated that ablation of Bmal1 also affected the circadian rhythmicity of several proteins, for example, HIF1α, Runx2 and MMP13 in vitro.
3.8 Effect of Bmal1 ablation on chondrocyte function and key factors expression in vitro
We used several different assays (BrdU, qPCR and TUNEL) to test whether deletion of Bmal1 influenced the chondrocytes proliferation, differentiation and apoptosis in the primary cultured chondrocytes. Similar to what was found in vivo, ablation of Bmal1 led to an obvious inhibition of chondrocytic proliferation ( and b)) and differentiation () and induced a significant increase of TUNEL-positive cells ( and e)) in vitro.
Figure 6. Effect of Bmal1 ablation on chondrocyte function and HIF1α, HIF2α and VEGF Expression in vitro (a and b) BrdU labeling of primary chondrocytes derived from BMAL1flox/flox and BMAL1CKO mice. The arrows showed the representative BrdU-positive cells. The labeled cells were also quantitatively analyzed (n= 4/group). (c) Col10a mRNA expression in cultured chondrocytes from control and BMAL1CKO mice was tested by qPCR (n= 5/group). (d and e) Deletion of Bmal1 increased the number of TUNEL-positive cells in cultured chondrocytes. The representative TUNEL positive cells were labeled by red fluorescence. The result was also quantitatively analyzed (n= 5/group). (f and g) Western blot and qPCR analysis were used to show the expression of Bmal1, HIF1α, HIF2α and VEGF in primary chondrocytes derived from BMAL1CKO and control mice under normoxia and hypoxia (n= 5/group). (h and i) Western blot and qPCR analysis showing the expression of Bmal1 and other factors in chondrocytes transfected with Bmal1 siRNA under normal oxygen and low oxygen (n= 3/group). Data are expressed as means ± SE in each bar graph. *p< 0.05. **p < 0.01.
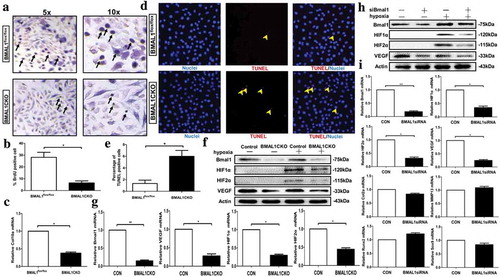
To further investigate whether Bmal1 ablation affected the expression of VEGF, HIF1α and HIF2α in chondrocytes, we cultured primary chondrocytes derived from BMAL1CKO and control mice under normoxia and hypoxia. As expected, we observed a significant decrease of Bmal1 protein (under normoxia and hypoxia) and mRNA (under hypoxia) levels in chondrocytes from BMAL1CKO mice ( and g)). In addition, we found that the protein (under normoxia and hypoxia) and mRNA (under hypoxia) expression of VEGF were also decreased in chondrocytes from BMAL1CKO mice as detected by Western blot and qPCR test ( and g)). Both of HIF1α and HIF2α were not detectable under normoxia while under low oxygen tensions their expressions were significantly upregulated. HIF1α and HIF2α were decreased in chondrocytes derived from BMAL1CKO mice compared with control chondrocytes ((f)). The similar result was seen in the mRNA level (). Furthermore, to confirm the interaction of Bmal1 and HIF1α, we knocked down Bmal1 by transfecting chondrocyte with Bmal1 siRNA. As shown in , Bmal1 siRNA decreased protein and mRNA expression level of HIF1α, HIF2α and VEGF under hypoxia with the mRNA level of other factors (Col2a, MMP13, Runx2 and Sox9) being unaffected.
3.9 Increased Bmal1 expression could improve the chondrocytes function normalization
Since deletion of Bmal1 influenced the chondrocytes proliferation, differentiation, apoptosis and several key factors expression in vivo and vitro, we then restored Bmal1 expression in chondrocytes from BMAL1CKO mice by overexpression of Bmal1 and then tested the cell function. As shown in , we observed that restoration of Bmal1 increased the expression level of HIF1α, HIF2α and VEGF in BMAL1CKO chondrocytes cultured in hypoxia by Western blot and qPCR assays. Meanwhile, overexpression of Bmal1 increased the PCNA, Cyclin B1, Col10a, Col2a and Aggrecan level in BMAL1CKO chondrocytes which implied the cell proliferation and differentiation function were recovered partly (). Finally, restoration of Bmal1 decreased the number of TUNEL-positive cell in BMAL1CKO chondrocytes ()).
Figure 7. Recovery of Bmal1 expression improved the chondrocytes function. (a) For the rescue experiment, chondrocytes from BMAL1CKO mice were transfected with overexpressed Bmal1 plasmid, the expression of Bmal1, HIF1α, HIF2α and VEGF were detected by Western blot. (b) Proliferation and differentiation of the BMAL1CKO mice chondrocytes were partly recovered by transfection of overexpression plasmid for Bmal1. (c) Relative mRNA expression level of several factors for proliferation, differentiation and apoptosis in BMAL1CKO chondrocytes transfected with Bmal1 overexpression plasmid was detected by qPCR test (n= 3/group). Results were presented as gene expression levels in all groups normalized to controls. (d) Overexpression of Bmal1 decreased the number of TUNEL-positive cells in cultured BMAL1CKO mice chondrocytes. The representative TUNEL positive cells were labeled by red fluorescence (n= 3/group). Data are expressed as means ± SE in each bar graph. *p < 0.05.
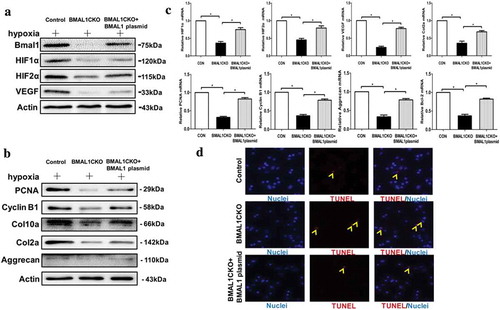
4. Discussion
In this report, we have demonstrated that postnatal deletion of Bmal1 specific in cartilage causes decrease of chondrocyte proliferation and promotion of chondrocyte hypertrophy, leading to impairment of growth plate chondrogenesis and in turn, a retardation of longitudinal bone growth. Together with several previous studies which have established that normal circadian rhythm genes expression is essential for maintaining cartilage and bone homeostasis [Citation11,Citation27,Citation28], our research elucidated that Bmal1 played an important role for physiological growth plate chondrogenesis afterbirth.
The circadian clock system conserves and activates hierarchically from a biological clock in the suprachiasmatic nuclei of the hypothalamus to the peripheral circadian oscillators in different tissues and even the individual cell also possesses the basic unit of circadian timekeeping [Citation29]. Furthermore, more and more studies have established a link between the disruption of the clock system and several pathological states, for example, metabolic diseases, cancers and osteoarthritis [Citation30–Citation32]. The autonomous clock exists in cartilage tissue and the circadian rhythm disruption induced by the environmental change could lead to OA-like pathologic alteration in articular cartilage [Citation27], all of which indicate that maintaining the normal clock system is vital for cartilage tissue homeostasis.
Besides the induction of environmental change, a study showed that the circadian gene mutant also induced the osteoarthritis-like changes in knee joint [Citation13]. Indeed, another study has demonstrated that the expression level of clock gene gradually decreased which was associated with age and the fluctuation effected the aging contributes to OA, implied the complicated function of clock gene in cartilage homeostasis [Citation11]. Moreover, a recent study showed that numbers of core clock genes (Bmal1, Per1 and Cry2) and cartilage-specific transcriptional factors (Runxs and FGFs) maintained the circadian expression and played a key role in normal endochondral ossification [Citation33]. Another research indicated that conditional deletion of the Bmal1 in chondrocytes led to growth retardation and shorter length of tibia and femur than that of control mice which was involved in decrease the expression of Indian hedgehog (IHH) [Citation34]. However, the underlying mechanism of circadian gene in controlling the chondrocytes homeostasis and development still requires further investigation.
To shed light on the effect of Bmal1 deletion on endochondral ossification in a special temporal window, we generate conditional Bmal1 knockout mice which Bmal1 gene in growth plate chondrocytes could be ablated by injecting tamoxifen intraperitoneally. Our data demonstrate that the Bmal1 knockout leads to reduced body and longitudinal bone length postnatally mainly due to a shorter proliferative zone of the growth plate. Of note, quantification of the ratio of the hypertrophic zone to total length of growth plate displays an obviously increase in BMAL1CKO mice compared with the control group. Furthermore, the chondrocytes proliferation, differentiation and apoptosis are all influenced by deletion of Bmal1 postnatally. Consistent with our findings, it was evidenced that the ablation of Bmal1 in cartilage caused the significant decrease in the length of body and longitudinal bone (femur and tibia) compared with the control mice [Citation34]. The conditional deletion of Bmal1 in cartilage also influenced the expression of several key factors, for example, phosphorylated SMAD2/3 (p-SMAD2/3), Acan and Sox9 [Citation13]. Together with our finding, the above studies defined that the core circadian gene Bmal1 was essential for maintenance of normal endochondral ossification.
As a principal engine of body and bone growth postnatal, the growth plate regulates the rate of new cartilage development and formation mainly by controlling the chondrocyte proliferation, differentiation and hypertrophy under many systemic and local signaling molecules. Our studies indicated that impaired circadian rhythm postnatal by deletion of Bmal1 in growth plate inhibited the chondrocyte proliferation and differentiation and caused a decrease and discrepant proliferative zone and hypertrophic zone, finally leading to growth retardation.
It is known that the growth plate which directs the growth of endochondral bones and consists of the chondrocytes and cartilaginous matrix is an avascular and hypoxic tissue. How to adapt to hypoxia is a critically evolutional event not only for chondrocytes subsist but also to the cell proliferation and differentiation which in part relies on the transcriptional regulatory protein HIF1α and HIF2α.
Although HIF1α and HIF2α are induced hydroxylation and polyubiquitination by oxygen-dependent prolyl hydroxylase domain (PHD) enzymes and von Hippel–Lindau (Vhl) protein complex under normoxic conditions [Citation35–Citation39], they are stable and heterodimerized with the constitutive HIF protein family β-subunit members under hypoxic conditions and tissues, for example, cartilage [Citation19]. The crucial role of HIF protein in modulating and maintaining the cartilaginous development and homeostasis is appreciated. As a main controller to regulate the oxygen metabolism in cell, HIF1α specific deletion in cartilage could induce the significant cell death in the central zone on account of the mistake switch between aerobic metabolism and anaerobic metabolism [Citation21,Citation40,Citation41]. In addition to its role in decreasing the overconsumption of scarce oxygen in this growth plate, HIF1α also influenced the hydroxylation of the collagen proline residues which was a critical step in the formation of the collagen triple helix [Citation42,Citation43]. Furthermore, deletion of HIF1α inhibited the normally terminal differentiation of chondrocytes involved in regulating the expression of SRY-type high-mobility group box-9 (SOX9) [Citation44,Citation45]. Besides HIF1α, several studies also shown HIF2α effected the transcriptional regulation of endochondral ossification [Citation46,Citation47].
Bmal1 exists as a player of β-subunit members of the HIF protein family interacting with α-subunit to regulate tissues to adapt the different environment in the transcriptional level and in the protein level, and it shares the same major protein domains with HIF1α [Citation48]. The relation between Bmal1 and HIFs (HIF1α and HIF2α) has been studied in several different tissues and cells for a few years. It has been seen that the interaction between the above genes was not in the same action mode in a different cell. It has been reported that Bmal1 could form a heterodimer with HIF1α and HIF2α in the TACGTGA DNA binding sites and drive transcription in order to respond to cellular hypoxia in COS-1 cell [Citation16]. In yeast, by using the two-hybrid system, HIF1α was demonstrated as partner PAS proteins of Bmal1 and when co-expression of Bmal1 and HIF-1α in Arnt-deficient c4 cells enhanced the transcription level of a reporter gene driven include by the HRE sequences [Citation49]. A study used the Neuro-2A cell showed that HIF1α induced the vasopressin promoter expression by cooperating with circadian gene Clock which finally influenced the Bmal1 binding [Citation50]. Besides Bmal1, several studies have observed that HIF1α also affected another circadian genes rhythm, for example, Per2 [Citation51]. But, the interaction between Bmal1 and HIF was not observed in all cells, a study using ES cell suggested that Bmal1 could not form complexes with HIF1α under a variety of criteria [Citation52].
To gain insight into the further mechanism which caused the growth retardation, we next performed several studies in vitro. We found that the levels of the HIF1α and HIF2α were significantly increased in hypoxia as compared to normoxia in primary chondrocytes. Down-regulation of Bmal1 expression induced by tamoxifen or administration of Bmal1 siRNA all decreased the level of VEGF, HIF1α and HIF2α in primary chondrocytes. Meanwhile, the results of Western blot demonstrated that transfection of Bmal1 significantly enhanced the expression level of VEGF, HIF1α and HIF2α in primary chondrocytes.
VEGF, a classic target of HIFs, plays a core role in cartilage development and particularly in the conversion of the avascular growth plate cartilage into highly vascularized bone which terms angiogenic–osteogenic coupling [Citation53,Citation54]. During the normal growth plate cartilage development, different chondrocytes showed individual feature for vascular invasion which mainly induced by VEGF; proliferative chondrocytes were resistant to vascular invasion due to the presence of angiogenic inhibitors [Citation55], by contrast when cells transformed from the proliferative chondrocytes to hypertrophic chondrocytes, production of angiogenic stimuli (such as VEGF) coincided with the appearance of hypertrophic chondrocytes [Citation56]. Postnatal, the vessels invade the epiphyseal cartilage which mainly induced by VEGF modulates further bone growth and extension.
Similar to the phenotype that observed in VEGF inactivated mice [Citation25], our finding that BMAL1CKO mice exhibited delayed long bone growth prompted us to ask whether the effect of Bmal1 ablation on growth plate chondrogenesis is mediated by VEGF. Indeed, in our study, we demonstrated that expression of VEGF was decreased in the BMAL1CKO mice growth plate as well as the HIF1α and HIF2α. Consistently, inhibition the VEGF could relay the vessel invasion and increase the length of hypertrophic zone [Citation25].
Numerous studies have shown that the VEGF which was a downstream target of HIF1α also mediated the survival-promoting functions of HIF1α mainly in the central zone of growth plate [Citation21]. HIF1α controlled the VEGF level in hypoxic tissue; furthermore, conditional knockout of VEGF in chondrocytes caused the cell death phenotype mainly happened in the proliferative and upper hypertrophic zones, similar to what was observed in HIF1α conditional knockout mice [Citation57]. Besides, several lines of evidence have demonstrated that HIF1α acts as a core regulator of pro- and anti-apoptotic genes [Citation58,Citation59]. So, the increased number of apoptotic cells observed in the BMAL1CKO growth plate and primary chondrocytes partly resulted from the inhibition of the HIF1α and VEGF expression. In addition to playing a survival factor for chondrocytes, HIF1α also influenced the balance between anabolism and catabolism in cartilage. It was reported that HIF1α depletion in chondrocyte led to the significantly higher MMP13 level than that in the control group [Citation60]. Consistent with that finding, in our study, the increased expression of MMP13 in BMAL1CKO growth plate assessed by IHC was possibly induced by the down-regulated HIF1α.
Hence, our findings indicate the circadian gene Bmal1 not only controls the relative genes circadian rhythm in cartilage but also regulates the physiological chondrogenesis in coupling with HIF1α and VEGF (). Thus, it might provide a novel insight into the regulation of circadian rhythm on cartilage.
Figure 8. Schematic diagram of proposed model to explain the molecular mechanism of Bmal1 deficiency influenced the HIF1α-VEGF axis in the chondrocytes. The BMAL1/CLOCK complex could not only trigger the circadian rhythms by binding to DNA elements E-box in the promoters of target genes, for example, Cry1 and Per1 but also regulate the HIF1α activity in chondrocytes. Then, the affected HIF1α by ablation of BMAL1 in chondrocytes inhibited the downstream factor, for example, VEGF and finally induced a disordered endochondral ossification afterbirth.
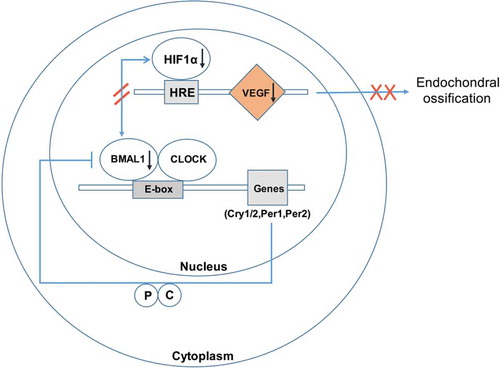
In summary, in this study, we demonstrate a key role of normal circadian rhythm for growth plate chondrogenesis and inhibition of the rhythm influences the chondrocyte proliferation, differentiation and apoptosis. In addition to the multiplicity of circadian clock and their connection to the VEGF and HIF in the growth plate cartilage, future studies will aim at the circadian clock and its role in regulating of metabolic pathways in the growth plate.
Author contributions
Study design: HZ Sun, SF Wu, ZM Ma and XX Jin. Acquisition of data: SF Wu, ZM Ma, XX Jin, HX Li, Z Qian, M Xu and Y Zhang. Data analysis: ZM Ma, XM Kang, Y Zhang, F Li, X Gao, LT Zhao and ZM Zhang. Drafting manuscript: ZM Ma and XX Jin. Critical revision of the manuscript: HZ Sun and SF Wu. Approving final version of manuscript: All authors.
Supplemental Material
Download MS Word (1.3 MB)Disclosure statement
No potential conflict of interest was reported by the authors.
Supplementary Material
Supplemental data for this article can be accessed here.
Additional information
Funding
References
- Long F, Ornitz DM. Development of the endochondral skeleton. Cold Spring Harb Perspect Biol. 2013 Jan; 5(1):a008334 [pii]. PubMed PMID: 23284041.
- Kronenberg HM. Developmental regulation of the growth plate. Nature. 2003 May 15; 423(6937):332–336. nature01657 [pii]. PubMed PMID: 12748651.
- Ortega N, Behonick DJ, Werb Z. Matrix remodeling during endochondral ossification. Trends Cell Biol. 2004 Feb;14(2):86–93; S0962892403003027 [pii]. PubMed PMID: 15102440.
- Schibler U, Sassone-Corsi P. A web of circadian pacemakers. Cell. 2002 Dec 27;111(7):919–922. S0092867402012254 [pii]. PubMed PMID: 12507418.
- Yoo SH, Yamazaki S, Lowrey PL, et al. PERIOD2::lUCIFERASEreal-time reporting of circadian dynamics reveals persistent circadian oscillations in mouse peripheral tissues. Proc Natl Acad Sci U S A. 2004 Apr 13;101(15):5339–5346. 0308709101 [pii]. PubMed PMID: 14963227.
- Fu L, Lee CC. The circadian clock: pacemaker and tumour suppressor. Nat Rev Cancer. 2003 May;3(5):350–361; nrc1072 [pii]. PubMed PMID: 12724733.
- Hastings MH, Reddy AB, Maywood ES. A clockwork web: circadian timing in brain and periphery, in health and disease. Nat Rev Neurosci. 2003 Aug;4(8):649–661; nrn1177 [pii]. PubMed PMID: 12894240.
- Stevenson S, Hunziker EB, Herrmann W, et al. Is longitudinal bone growth influenced by diurnal variation in the mitotic activity of chondrocytes of the growth plate? J Orthop Res. 1990 Jan;8(1):132–135. PubMed PMID: 2293628.
- Andersson ML, Petersson IF, Karlsson KE, et al. Diurnal variation in serum levels of cartilage oligomeric matrix protein in patients with knee osteoarthritis or rheumatoid arthritis. Ann Rheum Dis. 2006 Nov;6511:1490–1494. ard.2005.051292 [pii]. PubMed PMID: 16707535.
- Kong SY, Stabler TV, Criscione LG, et al. Diurnal variation of serum and urine biomarkers in patients with radiographic knee osteoarthritis. Arthritis Rheum. 2006 Aug;54(8):2496–2504. PubMed PMID: 16868970.
- Gossan N, Zeef L, Hensman J, et al. The circadian clock in murine chondrocytes regulates genes controlling key aspects of cartilage homeostasis. Arthritis Rheum. 2013 Sep;65(9):2334–2345. PubMed PMID: 23896777.
- Bunger MK, Walisser JA, Sullivan R, et al. Progressive arthropathy in mice with a targeted disruption of the Mop3/Bmal-1 locus. Genesis. 2005 Mar;41(3):122–132. PubMed PMID: WOS:000228176700004.
- Dudek M, Gossan N, Yang N, et al. The chondrocyte clock gene Bmal1 controls cartilage homeostasis and integrity. J Clin Invest. 2016 Jan;1261:365–376. 82755 [pii]. PubMed PMID: 26657859.
- Provot S, Schipani E. Fetal growth plate: a developmental model of cellular adaptation to hypoxia. Ann N Y Acad Sci. 2007 Nov;1117:26–39. PubMed PMID: 18056035. 1117/1/26[pii].
- Schipani E. Hypoxia and HIF-1alpha in chondrogenesis. Ann N Y Acad Sci. 2006 Apr;1068:66–73. PubMed PMID: 16831906. 1068/1/66[pii].
- Hogenesch JB, Gu YZ, Jain S, et al. The basic-helix-loop-helix-PAS orphan MOP3 forms transcriptionally active complexes with circadian and hypoxia factors. Proc Natl Acad Sci U S A. 1998 May 12;95(10):5474–5479. PubMed PMID: 9576906.
- Suyama K, Silagi ES, Choi H, et al. Circadian factors BMAL1 and RORalpha control HIF-1alpha transcriptional activity in nucleus pulposus cells: implications in maintenance of intervertebral disc health. Oncotarget. 2016 Apr 26;7(17):23056–23071. 8521 [pii]. PubMed PMID: 27049729.
- Egg M, Koblitz L, Hirayama J, et al. Linking oxygen to time: the bidirectional interaction between the hypoxic signaling pathway and the circadian clock. Chronobiol Int. 2013 May;30(4):510–529. PubMed PMID: 23421720.
- Keith B, Johnson RS, Simon MC. HIF1alpha and HIF2alpha: sibling rivalry in hypoxic tumour growth and progression. Nat Rev Cancer. 2011 Dec 15; 12(1):9–22. nrc3183 [pii]. PubMed PMID: 22169972.
- Rankin EB, Giaccia AJ, Schipani E. A central role for hypoxic signaling in cartilage, bone, and hematopoiesis. Curr Osteoporos Rep. 2011 Jun;9(2):46–52. PubMed PMID: 21360287
- Schipani E, Ryan HE, Didrickson S, et al. Hypoxia in cartilage: HIF-1alpha is essential for chondrocyte growth arrest and survival. Genes Dev. 2001 Nov 1;15(21):2865–2876. PubMed PMID: 11691837.
- Egg M, Paulitsch M, Ennemoser Y, et al. Chronodisruption increases cardiovascular risk in zebrafish via reduced clearance of senescent erythrocytes. Chronobiol Int. 2014 Jun;31(5):680–689. PubMed PMID: 24568569.
- Snelling SJ, Forster A, Mukherjee S, et al. The chondrocyte-intrinsic circadian clock is disrupted in human osteoarthritis. Chronobiol Int. 2016;33(5):574–579. PubMed PMID: 27019373.
- Liu Y, Olsen BR. Distinct VEGF functions during bone development and homeostasis. Arch Immunol Ther Exp (Warsz). 2014 Oct;62(5):363–368. PubMed PMID: 24699630
- Gerber HP, Vu TH, Ryan AM, et al. VEGF couples hypertrophic cartilage remodeling, ossification and angiogenesis during endochondral bone formation. Nat Med. 1999 Jun;5(6):623–628. PubMed PMID: 10371499.
- Cramer T, Schipani E, Johnson RS, et al. Expression of VEGF isoforms by epiphyseal chondrocytes during low-oxygen tension is HIF-1 alpha dependent. Osteoarthritis Cartilage. 2004 Jun;126:433–439. S1063458404000275 [pii]. PubMed PMID: 15135139.
- Kc R, Li X, Voigt RM, et al. Environmental disruption of circadian rhythm predisposes mice to osteoarthritis-like changes in knee joint. J Cell Physiol. 2015 Sep;230(9):2174–2183. PubMed PMID: 25655021.
- Maronde E, Schilling AF, Seitz S, et al. The clock genes Period 2 and Cryptochrome 2 differentially balance bone formation. PLoS One. 2010 Jul 12;5(7):e11527. PubMed PMID: 20634945.
- Tahara Y, Shibata S. Chronobiology and nutrition. Neuroscience. 2013 Dec 3;253:78–88. S0306-4522(13)00737-9 [pii]. PubMed PMID: 24007937.
- Akagi R, Akatsu Y, Fisch KM, et al. Dysregulated circadian rhythm pathway in human osteoarthritis: NR1D1 and BMAL1 suppression alters TGF-beta signaling in chondrocytes. Osteoarthritis Cartilage. 2017 Jun;256:943–951. S1063-4584(16)30406-X [pii]. PubMed PMID: 27884645.
- Lamia KA, Storch KF, Weitz CJ. Physiological significance of a peripheral tissue circadian clock. Proc Natl Acad Sci U S A. 2008 Sep 30; 105(39):15172–15177. 0806717105 [pii]. PubMed PMID: 18779586.
- Ha NH, Long J, Cai Q, et al. The circadian rhythm gene Arntl2 is a metastasis susceptibility gene for estrogen receptor-negative breast cancer. PLoS Genet. 2016 Sep;129:e1006267. PGENETICS-D-16-01059 [pii]. PubMed PMID: 27656887.
- Zvonic S, Ptitsyn AA, Kilroy G, et al. Circadian oscillation of gene expression in murine calvarial bone. J Bone Miner Res. 2007 Mar;22(3):357–365. PubMed PMID: 17144790.
- Takarada T, Kodama A, Hotta S, et al. Clock genes influence gene expression in growth plate and endochondral ossification in mice. J Biol Chem. 2012 Oct 19;287(43):36081–36095. PubMed PMID: 22936800.
- Bunn HF, Poyton RO. Oxygen sensing and molecular adaptation to hypoxia. Physiol Rev. 1996 Jul;76(3):839–885. PubMed PMID: 8757790
- Kaelin WG Jr. How oxygen makes its presence felt. Genes Dev. 2002 Jun 15;16(12):1441–1445. PubMed PMID: 12080083.
- Liu L, Simon MC. Regulation of transcription and translation by hypoxia. Cancer Biol Ther. 2004 Jun;3(6):492–497. 1010 [pii]. PubMed PMID: 15254394.
- Wang GL, Jiang BH, Rue EA, et al. Hypoxia-inducible factor 1 is a basic-helix-loop-helix-PAS heterodimer regulated by cellular O2 tension. Proc Natl Acad Sci U S A. 1995 Jun 6;92(12):5510–5514. PubMed PMID: 7539918.
- Min JH, Yang H, Ivan M, et al. Structure of an HIF-1alpha -pVHL complex: hydroxyproline recognition in signaling. Science. 2002 Jun 7;296(5574):1886–1889. 1073440 [pii]. PubMed PMID: 12004076.
- Giaccia AJ, Simon MC, Johnson R. The biology of hypoxia: the role of oxygen sensing in development, normal function, and disease. Genes Dev. 2004 Sep 15; 18(18):2183–2194. 18/18/2183 [pii]. PubMed PMID: 15371333.
- Maes C, Araldi E, Haigh K, et al. VEGF-independent cell-autonomous functions of HIF-1alpha regulating oxygen consumption in fetal cartilage are critical for chondrocyte survival. J Bone Miner Res. 2012 Mar;27(3):596–609. PubMed PMID: 22162090.
- Myllyharju J, Schipani E. Extracellular matrix genes as hypoxia-inducible targets. Cell Tissue Res. 2010 Jan;339(1):19–29. PubMed PMID: 19662436
- Pfander D, Cramer T, Schipani E, et al. HIF-1alpha controls extracellular matrix synthesis by epiphyseal chondrocytes. J Cell Sci. 2003 May 1;116(Pt 9):1819–1826. PubMed PMID: 12665562.
- Amarilio R, Viukov SV, Sharir A, et al. HIF1alpha regulation of Sox9 is necessary to maintain differentiation of hypoxic prechondrogenic cells during early skeletogenesis. Development. 2007 Nov;13421:3917–3928. dev.008441 [pii]. PubMed PMID: 17913788.
- Robins JC, Akeno N, Mukherjee A, et al. Hypoxia induces chondrocyte-specific gene expression in mesenchymal cells in association with transcriptional activation of Sox9. Bone. 2005 Sep;37(3):313–322. S8756-3282(05)00208-5 [pii]. PubMed PMID: 16023419.
- Saito T, Fukai A, Mabuchi A, et al. Transcriptional regulation of endochondral ossification by HIF-2alpha during skeletal growth and osteoarthritis development. Nat Med. 2010 Jun;166:678–686. nm.2146 [pii]. PubMed PMID: 20495570.
- Araldi E, Khatri R, Giaccia AJ, et al. Lack of HIF-2alpha in limb bud mesenchyme causes a modest and transient delay of endochondral bone development. Nat Med. 2011 Jan;171:25–6; author reply 27–9. nm0111-25 [pii]. PubMed PMID: 21217667.
- Sabatini PV, Lynn FC. All-encomPASsing regulation of beta-cells: PAS domain proteins in beta-cell dysfunction and diabetes. Trends Endocrinol Metab. 2015 Jan;26(1):49–57; S1043-2760(14)00190-8 [pii]. PubMed PMID: 25500169.
- Takahata S, Sogawa K, Kobayashi A, et al. Transcriptionally active heterodimer formation of an Arnt-like PAS protein, Arnt3, with HIF-1a, HLF, and clock. Biochem Biophys Res Commun. 1998 Jul 30;248(3):789–794. S0006-291X(98)99012-X [pii]. PubMed PMID: 9704006.
- Ghorbel MT, Coulson JM, Murphy D. Cross-talk between hypoxic and circadian pathways: cooperative roles for hypoxia-inducible factor 1alpha and CLOCK in transcriptional activation of the vasopressin gene. Mol Cell Neurosci. 2003 Mar;22(3):396–404. S1044743102000192 [pii]. PubMed PMID: 12691740.
- Okabe T, Kumagai M, Nakajima Y, et al. The impact of HIF1alpha on the Per2 circadian rhythm in renal cancer cell lines. PLoS One. 2014;9(10):e109693. PONE-D-14-01264 [pii]. PubMed PMID: 25333958.
- Cowden KD, Simon MC. The bHLH/PAS factor MOP3 does not participate in hypoxia responses. Biochem Biophys Res Commun. 2002 Feb 1; 290(4):1228–1236. S0006291X01963090 [pii]. PubMed PMID: 11811994.
- Schipani E, Maes C, Carmeliet G, et al. Regulation of osteogenesis-angiogenesis coupling by HIFs and VEGF. J Bone Miner Res. 2009 Aug;24(8):1347–1353. PubMed PMID: 19558314.
- Riddle RC, Khatri R, Schipani E, et al. Role of hypoxia-inducible factor-1alpha in angiogenic-osteogenic coupling. J Mol Med (Berl). 2009 Jun;87(6):583–590. PubMed PMID: 19415227.
- Hiraki Y, Shukunami C. Angiogenesis inhibitors localized in hypovascular mesenchymal tissues: chondromodulin-I and tenomodulin. Connect Tissue Res. 2005;46(1):3–11. Q8NKV4H1Q261Q211 [pii]. PubMed PMID: 16019413.
- Zelzer E, Olsen BR. Multiple roles of vascular endothelial growth factor (VEGF) in skeletal development, growth, and repair. Curr Top Dev Biol. 2005;65:169–187. S007021530465006X [pii]. PubMed PMID: 15642383.
- Zelzer E, Mamluk R, Ferrara N, et al. VEGFA is necessary for chondrocyte survival during bone development. Development. 2004 May;1319:2161–2171. dev.01053 [pii]. PubMed PMID: 15073147.
- Leo C, Giaccia AJ, Denko NC. The hypoxic tumor microenvironment and gene expression. Semin Radiat Oncol. 2004 Jul;14(3):207–214; S1053-4296(04)00058-X [pii]. PubMed PMID: 15254863.
- Zhang FJ, Luo W, Lei GH. Role of HIF-1alpha and HIF-2alpha in osteoarthritis. Joint Bone Spine. 2015 May;82(3):144–147; S1297-319X(14)00229-2 [pii]. PubMed PMID: 25553838.
- Thoms BL, Dudek KA, Lafont JE, et al. Hypoxia promotes the production and inhibits the destruction of human articular cartilage. Arthritis Rheum. 2013 May;65(5):1302–1312. PubMed PMID: 23334958.