ABSTRACT
The orientation of the mitotic spindle is a crucial process during development and adult tissue homeostasis and multiple mechanisms have been shown to intrinsically regulate this process. However, much less is known about the extrinsic cues involved in modulating spindle orientation. We have recently uncovered a novel function of Eph intercellular signaling in regulating spindle alignment by ultimately ensuring the correct cortical distribution of central components within the intrinsic spindle orientation machinery. Here, we comment on these results, novel questions that they open and potential additional research to address in the future.
Eph signaling
Erythropoietin-producing hepatocellular carcinoma (Eph) receptors, the largest family of Receptor Tyrosine Kinases (RTKs), are key regulators of both developmental processes and adult tissue homeostasis, whose misregulation has been associated with different pathologies, including cancer [Citation1–Citation10]. Eph receptors and the Eph family receptor-interacting (ephrin) ligands are highly conserved throughout the animal kingdom. In vertebrates, a large and redundant family of about 16 Eph receptors and a family of 9 ephrin ligands has been characterized, both divided into two subclasses, A and B. Most EphAs bind to glycosylphosphatidylinositol (GPI)-membrane anchored ephrinAs and most EphBs interact to transmembrane ephrinBs, although some class-crossing interactions between both subtypes exist. Drosophila, by contrast, has a single Eph receptor and a single Ephrin ligand, the latter being more similar to vertebrate ephrinBs (). All Eph receptors, like other RTKs, are transmembrane proteins with an N-terminal extracellular domain containing the ligand-binding motif and an intracellular carboxyl-terminal domain with diverse motifs including the kinase domain [Citation11] (). Thus, ephrin-Eph signaling relies mainly on cell-cell direct contact. However, additional mechanisms that are cell contact-independent have been recently reported [Citation12]. Another unique feature of ephrin-Eph signaling is the bidirectional nature of the cell-cell communication: it can be a forward signaling (ephrin-Eph) or a reverse signaling (Eph-ephrin), transduced in the Eph or ephrin expressing cell, respectively [Citation3,Citation13]. As mentioned above, even though Eph receptors were first identified in human tumors [Citation2] and then studied profusely in the context of axon guidance promoting cell-cell attraction/repulsion [Citation3,Citation14], a wide spectrum of developmental processes has been shown to depend on Eph signaling over the past decades. These processes include cell proliferation, cell migration, tissue boundary formation, cell-cell junction dynamics and apoptosis [Citation3,Citation9,Citation15]. We have now uncovered a novel function of the Eph signaling in regulating a fundamental cell process, the orientation of the mitotic spindle, in the Drosophila optic lobe neuroepithelial cells [Citation16].
Figure 1. Ephrin and Eph receptor family members in vertebrates and in Drosophila. The structure of Eph receptors is similar in vertebrates and in Drosophila, while Drosophila Ephrin is more similar to vertebrate ephrinBs; PDZ BD: PSD95/Dlg1/ZO-1 Binding Domain; RBD: Receptor Binding Domain; LBD: Ligand Binding Domain; CRD: Cysteine-rich domain; FNIII: Fibronectin type III; JM: JuxtaMembrane; TK; Tyrosine Kinase; SAM: Sterile Alpha Motif. Most vertebrate EphA and B receptors bind promiscuously to any ephrin A or B ligands, respectively (black arrows), with some exceptions, in which the Eph receptor only binds particular ephrin ligand (red arrows). Some class-crossing interactions, in which Eph receptors bind ephrin ligands from a different subtype, also exist (orange arrows). In Drosophila, there is a single Eph receptor and a single Ephrin ligand.
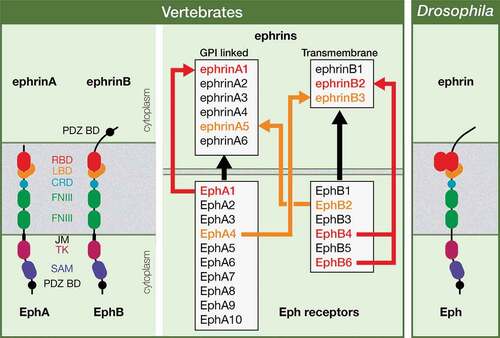
Relevance of mitotic spindle orientation in development and tumorigenesis
Proper orientation of the mitotic spindle is critical as it determines tissue architecture or cell fate specification in the context of a symmetric or an asymmetric cell division. Epithelial cells frequently divide symmetrically, parallel to the anterior-posterior axis of cell polarity, while stem cells/progenitors (for example, Drosophila neural stem cells, aka neuroblasts), can divide asymmetrically, along the apical-basal axis of cell polarity, to generate another stem cell and a daughter cell committed to differentiate () [Citation17–Citation21]. Thus, failures in spindle alignment could cause loss of tissue organization or an increased number of self-renewal stem cells in detriment of differentiated cells, both features being potential tumor-promoting events [Citation22–Citation24]. However, the defects in tissue architecture and cell proliferation that might imply a misregulated mitotic spindle positioning are normally counteracted by different mechanisms that have evolved to avoid such lethal phenotypes. For example, in the Drosophila wing disc epithelium, basal cell extrusion and apoptosis are used to eliminate those cells with misorientated spindles [Citation23,Citation25]. In other epithelia, such as the Drosophila follicular epithelium, misplaced cells due to defects in the planar orientation of the division, are reintegrated into the epithelium, a process promoted by the adhesion molecules Fasciclin 2 and Neuroglian [Citation26]. Some human tumors have been associated with particular mutations in genes such as APC or E-Cadherin, which are known spindle orientation regulators [Citation27–Citation34]. This is very suggestive but it does not strictly prove a cause-and-effect relationship between the spindle phenotype and the tumor development [Citation35]. Moreover, in Drosophila neuroblasts, single mutations in core components of the spindle orientation machinery are not enough to induce tumor-like overgrowth in the larval brain, at least in part because of the high redundancy in the regulation of the whole process [Citation36]. The spindle alignment regulator Pins/LGN is an intriguing case. On one hand, pins mutant neuroblast lineages in the larval central brain do not show overgrowth (rather they are smaller and sometimes without any neuroblast) [Citation37], similar phenotype shown by neuroblast lineages mutant in other core components of the spindle orientation machinery, such as Mud/NuMA, Canoe/Afadin or Dlg1, even though in these latter cases it is frequent the presence of ectopic neuroblasts within the mutant clone [Citation36,Citation38,Citation39]. On the other hand, pieces of pins mutant larval brain implanted into the abdomen of adult hosts originate big tumoral masses after some weeks and can even metastasize into other organs [Citation22]. No such GFP-labeled allograft transplants have been performed with mutant tissue from other spindle regulators. It would be interesting to determine whether Pins is an exceptional case or whether the microenvironment in which the mutant cells grow makes all the difference[Citation36]. Considering all this, we can conclude that mitotic spindle misalignment alone cannot induce cancer development. However, a proper orientation of the mitotic spindle is a relevant process to have into account as it might constitute a sensitized condition that, in combination with other eventual tumor suppressor mutations or oncogene activation, could trigger tumorigenesis [Citation35,Citation40,Citation41].
Mechanisms that regulate spindle orientation: intrinsic and extrinsic cues
Given the relevance of correctly aligning the mitotic spindle during development and tissue homeostasis, it is not unexpected the existence of multiple mechanisms that ensure a robust regulation of this process. Particularly, autonomous or intrinsic cues underlying spindle orientation have been extensively covered in different systems, and recent publications have reviewed these increasingly growing number of autonomous factors in detail [Citation17,Citation40–Citation50]. Among the intrinsic factors, the Pins/LGN-Gαi-Mud-NuMA complex stands at the core of the regulatory network of spindle positioning. However, even some of these molecules, such as Pins/LGN-Gαi, have been shown to be dispensable in some systems, while Mud/NuMA stays as an essential component in all of them [Citation51–Citation53]. Thus, a widespread notion in the field is that the regulation of spindle orientation is highly context-dependent, with variations in the spindle alignment machinery depending on the cell type and organism.
In addition to the extensive analyses of the autonomous cues involved in modulating spindle orientation, over the past few years the role of different extrinsic cues and, importantly, their link with the intrinsic spindle orientation machinery is being uncovered. For example, E-cadherin-mediated intercellular signaling, which affects the orientation of the spindle in multiple systems including mammalian epithelia and Drosophila germline [Citation27,Citation29–Citation32,Citation34], has been recently linked to LGN/Pins both in vitro and in vivo [Citation54,Citation55]. In one of these studies, authors show that E-Cadherin performs an instructive role in spindle orientation by recruiting LGN, which binds to E-Cadherin cytosolic tail through the TPR domain at interphase. At metaphase, NuMA is released from the nucleus and competes with E-Cadherin for binding the TPR repeat domain of LGN, which remains attached to the membrane through the Gαi subunit at the adherens junction region. In this way, astral microtubules can contact this region and the spindle is correctly orientated[Citation54]. In the other study, performed in mouse prostate luminal cells, authors also show a direct interaction between E-Cadherin and LGN. In addition, they found that the polarity protein Scribble (SCRIB) forms a ternary complex with them and it is essential for their interaction. At the same time, this complex allows a precise location of the mitotic spindle by linking polarity proteins with spindle orientation cues[Citation55]. Planar cell polarity activated by Wnt extracellular signals has been shown to modulate spindle alignment in different contexts [Citation56–Citation58]. However, like in the case of E-Cadherin, the mechanism by which this pathway directly affects the orientation of the spindle was revealed only some years ago[Citation53]. In this work, a conserved direct interaction between the Wnt effector Dishevelled (Dsh) and the intrinsic cue Mud/NuMA was established both in Drosophila and zebrafish. Finally, intercellular Semaphorin-Plexin signaling was recently shown to regulate spindle orientation by controlling the activity of Cdc42, a known regulator of mitotic spindle, in mouse spinal cord and during mouse kidney development and repair [Citation59,Citation60].
Eph intercellular signaling as a novel extrinsic cue regulating spindle orientation
In a recent work, we have unveiled a role of Eph signaling as a novel extrinsic mechanism required to regulate the mitotic spindle positioning in the neuroepithelial cells of the Drosophila optic lobe[Citation16]. We have shown that Eph signaling activates aPKC and that this is necessary to fully activate P-myosin II/P-Sqh. This, in turn, impinges on spindle alignment by contributing to the proper cortical localization of intrinsic cues located at the core of the spindle orientation machinery, such as Canoe/Afadin, Mud/NuMA and Dlg1 (). Based on our results and other published data in vertebrates, we propose that a reverse Eph-Ephrin signaling is responsible for aPKC activation at the subapical region. In the epithelia of Xenopus embryos, it has been demonstrated a competition between ephrinB1 and active Cdc42 for binding to Par-6. Par-6, in a complex with Par-3, must bind active Cdc42 for this in turn to activate aPKC at the tight junctions, which is a domain equivalent to the subapical region in Drosophila [Citation61–Citation64]. Thus, a Par-3/Par-6/active Cdc42/aPKC complex is necessary for the formation or/and maintenance of the tight junctions, and a Par3/Par-6/ephrin complex hampers aPKC activation and leads to the disruption of the tight junctions. In these epithelial cells, the phosphorylation of ephrin induced by an Eph reverse signaling prevents the interaction of ephrin with Par-6, allowing the binding of Cdc42, the activation of aPKC and the consequent stabilization of the tight junctions[Citation63]. In Drosophila Eph mutant neuroepithelial cells, we found defects in aPKC activation, as a constitutively activated form of aPKC was able to rescue the Eph mutant phenotype, including defects in the mitotic spindle orientation as well as failures in the localization of phosphomimetic myosin II/SqhEE in neuroepithelial cells. Moreover, in Eph mutants, the subapical region of the neuroepithelial cells was completely collapsed. This suggests that under normal conditions, and similarly to that observed at the tight junctions of Xenopus epithelia, an Eph reverse signaling leads to the phosphorylation of Ephrin at the subapical region. In fact, constitutively activated aPKC rescued the formation of the subapical region in Drosophila Eph mutant neuroepithelial cells[Citation16]. It would be interesting to analyze in these mutant cells the localization of Cdc42, which would be expected to be altered if the hypothesis of the Eph reverse signal at the level of the subapical region is correct in our system.
Figure 3. Eph signaling regulates mitotic spindle orientation: working model. Both a reverse and a forward Eph signaling would be operating between neuroepithelial cells in the Drosophila optic lobe. A forward signaling activates Rok/ROCK, which (1) inhibits PI3K-Akt1 signaling pathway and hence proliferation and (2) phosphorylates and activates myosin II Sqh/RLC. A reverse signaling at the level of SA activates aPKC, which fully activate P-Sqh/P-RLC and this, in turn, impacts on spindle orientation by contributing to the correct cortical localization of intrinsic cue regulators, such as Cno/Afadin, Dlg1, and Mud/NuMA. SA: SubApical region; AJs: Adherens Junctions; BL: Basolateral region. (Adapted from Franco and Carmena, 2019).
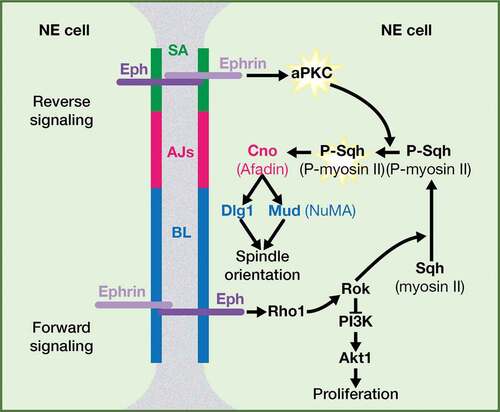
Other questions that arise from our work/model are: 1) what is exactly the meaning of “fully activated” P-myosin II/P-Sqh and 2) how aPKC is mediating this state? “Fully activated” would imply that apart of being phosphorylated by the kinase Rok/ROCK, myosin II/Sqh would require aPKC for regulating its apical localization. And an obvious candidate downstream of aPKC involved in the activation state of myosin II/Sqh is the myosin II/Sqh repressor Lethal (2) giant larvae (L(2)gl). aPKC phosphorylates L(2)gl blocking its binding to myosin II/Sqh in different systems; as a consequence, L(2)gl cannot inhibit myosin II/Sqh [Citation65–Citation71]. Hence, we might expect an ectopic apical activation of L(2)gl in Eph mutant neuroepithelial cells, in which aPKC is inactive, and the consequent mislocalization of myosin II/Sqh.
In addition to the aPKC activation through a reverse Eph-Ephrin signaling, we propose that an Ephrin-Eph forward signaling also functions between neuroepithelial cells to trigger Rho1/RhoA-Rok/ROCK activation (). A good candidate for mediating the link between the Eph receptor and Rho is the Rho-guanine nucleotide exchange factor (GEF) Ephexin, which physically interacts with Eph and activates Rho1/RhoA in response to Ephrin-stimulated Eph in neuronal growth cones, both in Drosophila and in vertebrates [Citation72–Citation74]. Another potential connection between Eph and Rho activation is Dsh/DVL, the main effector of Wingless/Wnt-Frizzled signaling pathway [Citation75,Citation76]. In Xenopus, Xdsh forms a complex with EphB1/B2 and ephrin-B1, regulating RhoA activity in both a forward and a reverse Eph signaling[Citation77]. In our working model, the activation of Rho1/RhoA-Rok/ROCK signaling by Eph has two main consequences: 1) the phosphorylation and activation of myosin II/Sqh and 2) the inhibition of the PI3K-Akt signaling pathway, a major regulator of cell proliferation. In fact, Eph mutant optic lobe neuroepithelia show overgrowth[Citation16]. It is important to remark that this overproliferation phenotype is the result of the upregulation of Akt signaling in Eph mutants and not a consequence of spindle misorientation. Actually, the overproliferation phenotype of Eph mutants is suppressed by specifically downregulating in the neuroepithelia either Akt or PI3K[Citation16]. As mentioned before, different mechanisms have evolved to avert the fatal consequences that failures in the orientation of the spindle could imply. In the Drosophila optic lobe neuroepithelium, it operates the same adhesion molecule-mediated protective mechanism as in the follicular epithelium to reintegrate misplaced cells into the neuroepithelium[Citation26]. As a result, we do not observe an increase in the multilayering/width of the neuroepithelium, as it would be expected. On the contrary, the reintegration process plus the defects in myosin II/Sqh distribution present in Eph mutants lead to narrower, simple organization neuroepithelia by late third instar larvae. It would be interesting to analyze the consequences of mutating those adhesion molecules on an Eph mutant background. We expect a very strong tissue disorganization, given the misorientation of the spindle along with the increase in proliferation observed in Eph mutants.
Finally, one relevant question that emerges from our work is how conserved is the function of Eph signaling as a spindle orientation regulator in other Drosophila and vertebrate epithelia. As mentioned above, the mechanisms that control spindle alignment are very diverse and highly context-dependent. For example, aPKC is not essential for spindle planar orientation in the chicken neuroepithelium, neither in the Drosophila wing disc and follicular epithelium [Citation51,Citation78,Citation79]. Thus, we could speculate that these epithelia would be independent of Eph signaling for regulating spindle orientation, given that aPKC activation stands central downstream of Eph signaling in the optic lobe neuroepithelium. However, we cannot completely discard a function of Eph in those epithelia, as Eph might operate through alternative mechanisms in those tissues and, in addition, it still might be required for regulating Rho1/RhoA-Rok/ROCK signaling. Further work will be necessary in the future to solve all these questions.
Acknowledgments
We thank Stuart Ingham for helping with the figures. This work was supported by the Spanish Ministry of Economy and Competitiveness (MINECO) under Grants BFU2012-33020 and BFU2015-64251, and by FEDER (European Regional Development Fund). The “Instituto de Neurociencias” in Alicante is a Severo Ochoa Center of Excellence.
Disclosure statement
No potential conflict of interest was reported by the authors.
Additional information
Funding
References
- Batlle E, Wilkinson DG. Molecular mechanisms of cell segregation and boundary formation in development and tumorigenesis. Cold Spring Harb Perspect Biol. 2012;4:a008227.
- Hirai H, Maru Y, Hagiwara K, et al. A novel putative tyrosine kinase receptor encoded by the eph gene. Science. 1987;238:1717–1720.
- Kania A, Klein R. Mechanisms of ephrin-Eph signalling in development, physiology and disease. Nat Rev Mol Cell Biol. 2016;17:240–256.
- Lackmann M, Boyd AW. Eph, a protein family coming of age: more confusion, insight, or complexity?. Sci Signal. 2008;1:re2.
- Lisabeth EM, Falivelli G, Pasquale EB. Eph receptor signaling and ephrins. Cold Spring Harb Perspect Biol. 2013;5:a009159.-
- Merlos-Suarez A, Batlle E. Eph-ephrin signalling in adult tissues and cancer. Curr Opin Cell Biol. 2008;20:194–200.
- Pasquale EB. Eph-ephrin bidirectional signaling in physiology and disease. Cell. 2008;133:38–52.
- Pasquale EB. Eph receptors and ephrins in cancer: bidirectional signalling and beyond. Nat Rev Cancer. 2010;10:165–180.
- Taylor H, Campbell J, Nobes CD. Ephs and ephrins. Curr Biol. 2017;27:R90–R5.
- Wilkinson DG. Regulation of cell differentiation by Eph receptor and ephrin signaling. Cell Adh Migr. 2014;8:339–348.
- Himanen JP. Ectodomain structures of Eph receptors. Semin Cell Dev Biol. 2012;23:35–42.
- Gong J, Korner R, Gaitanos L, et al. Exosomes mediate cell contact-independent ephrin-Eph signaling during axon guidance. J Cell Biol. 2016;214:35–44.
- Holland SJ, Gale NW, Mbamalu G, et al. Bidirectional signalling through the EPH-family receptor Nuk and its transmembrane ligands. Nature. 1996;383:722–725.
- Egea J, Klein R. Bidirectional Eph-ephrin signaling during axon guidance. Trends Cell Biol. 2007;17:230–238.
- Niethamer TK, Bush JO. Getting direction(s): the Eph/ephrin signaling system in cell positioning. Dev Biol. 2019;447:42–57.
- Franco M, Carmena A. Eph signaling controls mitotic spindle orientation and cell proliferation in neuroepithelial cells. J Cell Biol. 2019;218:1200–1217.
- Bergstralh DT, Dawney NS, St Johnston D. Spindle orientation: a question of complex positioning. Development. 2017;144:1137–1145.
- Gallaud E, Pham T, Cabernard C. Drosophila melanogaster neuroblasts: a model for asymmetric stem cell divisions. Results Probl Cell Differ. 2017;61:183–210.
- Morin X, Bellaiche Y. Mitotic spindle orientation in asymmetric and symmetric cell divisions during animal development. Dev Cell. 2011;21:102–119.
- Morrison SJ, Kimble J. Asymmetric and symmetric stem-cell divisions in development and cancer. Nature. 2006;441:1068–1074.
- Williams SE, Fuchs E. Oriented divisions, fate decisions. Curr Opin Cell Biol. 2013;25:749–758.
- Caussinus E, Gonzalez C. Induction of tumor growth by altered stem-cell asymmetric division in Drosophila melanogaster. Nat Genet. 2005;37:1125–1129.
- Nakajima Y, Meyer EJ, Kroesen A, et al. Epithelial junctions maintain tissue architecture by directing planar spindle orientation. Nature. 2013;500:359–362.
- Royer C, Lu X. Epithelial cell polarity: a major gatekeeper against cancer?. Cell Death Differ. 2011;18:1470–1477.
- Guilgur LG, Prudencio P, Ferreira T, et al. Drosophila aPKC is required for mitotic spindle orientation during symmetric division of epithelial cells. Development. 2012;139:503–513.
- Bergstralh DT, Lovegrove HE, St Johnston D. Lateral adhesion drives reintegration of misplaced cells into epithelial monolayers. Nat Cell Biol. 2015;17:1497–1503.
- Den Elzen N, Buttery CV, Maddugoda MP, et al. Cadherin adhesion receptors orient the mitotic spindle during symmetric cell division in mammalian epithelia. Mol Biol Cell. 2009;20:3740–3750.
- Fleming ES, Temchin M, Wu Q, et al. Spindle misorientation in tumors from APC(min/+) mice. Mol Carcinog. 2009;48:592–598.
- Inaba M, Yuan H, Salzmann V, et al. E-cadherin is required for centrosome and spindle orientation in Drosophila male germline stem cells. PLoS One. 2010;5:e12473.
- Le Borgne R, Bellaiche Y, Schweisguth F. Drosophila E-cadherin regulates the orientation of asymmetric cell division in the sensory organ lineage. Curr Biol. 2002;12:95–104.
- Lechler T, Fuchs E. Asymmetric cell divisions promote stratification and differentiation of mammalian skin. Nature. 2005;437:275–280.
- Lu B, Roegiers F, Jan LY, et al. Adherens junctions inhibit asymmetric division in the Drosophila epithelium. Nature. 2001;409:522–525.
- Quyn AJ, Appleton PL, Carey FA, et al. Spindle orientation bias in gut epithelial stem cell compartments is lost in precancerous tissue. Cell Stem Cell. 2010;6:175–181.
- Yamashita YM, Jones DL, Fuller MT. Orientation of asymmetric stem cell division by the APC tumor suppressor and centrosome. Science. 2003;301:1547–1550.
- Pease JC, Tirnauer JS. Mitotic spindle misorientation in cancer – out of alignment and into the fire. J Cell Sci. 2011;124:1007–1016.
- Carmena A. Compromising asymmetric stem cell division in Drosophila central brain: revisiting the connections with tumorigenesis. Fly (Austin). 2018;12:71–80.
- Lee CY, Robinson KJ, Doe CQ. Lgl, Pins and aPKC regulate neuroblast self-renewal versus differentiation. Nature. 2006;439:594–598.
- Bowman SK, Neumuller RA, Novatchkova M, et al. The Drosophila NuMA homolog mud regulates spindle orientation in asymmetric cell division. Dev Cell. 2006;10:731–742.
- Rives-Quinto N, Franco M, de Torres-Jurado A, et al. Synergism between canoe and scribble mutations causes tumor-like overgrowth via Ras activation in neural stem cells and epithelia. Development. 2017;144:2570–2583.
- Nakajima YI. Mitotic spindle orientation in epithelial homeostasis and plasticity. J Biochem. 2018;164:277–284.
- Seldin L, Macara I. Epithelial spindle orientation diversities and uncertainties: recent developments and lingering questions. F1000Res. 2017;6:984.
- Bhattarai SR, Begum S, Popow R, et al. The ciliary GTPase Arl3 maintains tissue architecture by directing planar spindle orientation during epidermal morphogenesis. Development. 2019;146.
- Di Pietro F, Echard A, Morin X. Regulation of mitotic spindle orientation: an integrated view. EMBO Rep. 2016;17:1106–1130.
- Kotak S. Mechanisms of spindle positioning: lessons from worms and mammalian cells. Biomolecules. 2019;9:80.
- Kschonsak YT, Hoffmann I. Activated ezrin controls MISP levels to ensure correct NuMA polarization and spindle orientation. J Cell Sci. 2018;131:jcs214544.
- Lecland N, Hsu CY, Chemin C, et al. Epidermal development requires ninein for spindle orientation and cortical microtubule organization. Life Sci Alliance. 2019;2:e201900373.
- Lee BH, Schwager F, Meraldi P, et al. p37/UBXN2B regulates spindle orientation by limiting cortical NuMA recruitment via PP1/Repo-Man. J Cell Biol. 2018;217:483–493.
- McHugh T, Gluszek AA, Welburn JPI. Microtubule end tethering of a processive kinesin-8 motor Kif18b is required for spindle positioning. J Cell Biol. 2018;217:2403–2416.
- Nakajima YI, Lee ZT, McKinney SA, et al. Junctional tumor suppressors interact with 14-3-3 proteins to control planar spindle alignment. J Cell Biol. 2019;218:1824–1838.
- Vodicska B, Cerikan B, Schiebel E, et al. MISP regulates the IQGAP1/Cdc42 complex to collectively orchestrate spindle orientation and mitotic progression. Sci Rep. 2018;8:6330.
- Bergstralh DT, Lovegrove HE, Kujawiak I, et al. Pins is not required for spindle orientation in the Drosophila wing disc. Development. 2016;143:2573–2581.
- Johnston CA, Manning L, Lu MS, et al. Formin-mediated actin polymerization cooperates with mushroom body defect (Mud)-Dynein during frizzled-dishevelled spindle orientation. J Cell Sci. 2013;126:4436–4444.
- Segalen M, Johnston CA, Martin CA, et al. The Fz-Dsh planar cell polarity pathway induces oriented cell division via Mud/NuMA in Drosophila and zebrafish. Dev Cell. 2010;19:740–752.
- Gloerich M, Bianchini JM, Siemers KA, et al. Cell division orientation is coupled to cell-cell adhesion by the E-cadherin/LGN complex. Nat Commun. 2017;8:13996.
- Wang X, Dong B, Zhang K, et al. E-cadherin bridges cell polarity and spindle orientation to ensure prostate epithelial integrity and prevent carcinogenesis in vivo. PLoS Genet. 2018;14:e1007609.
- Gong Y, Mo C, Fraser SE. Planar cell polarity signalling controls cell division orientation during zebrafish gastrulation. Nature. 2004;430:689–693.
- Habib SJ, Chen BC, Tsai FC, et al. A localized Wnt signal orients asymmetric stem cell division in vitro. Science. 2013;339:1445–1448.
- Saburi S, Hester I, Fischer E, et al. Loss of Fat4 disrupts PCP signaling and oriented cell division and leads to cystic kidney disease. Nat Genet. 2008;40:1010–1015.
- Arbeille E, Reynaud F, Sanyas I, et al. Cerebrospinal fluid-derived Semaphorin3B orients neuroepithelial cell divisions in the apicobasal axis. Nat Commun. 2015;6:6366.
- Xia J, Swiercz JM, Banon-Rodriguez I, et al. Semaphorin-plexin signaling controls mitotic spindle orientation during epithelial morphogenesis and repair. Dev Cell. 2015;33:299–313.
- Joberty G, Petersen C, Gao L, et al. The cell-polarity protein Par6 links Par3 and atypical protein kinase C to Cdc42. Nat Cell Biol. 2000;2:531–539.
- Knust E, Bossinger O. Composition and formation of intercellular junctions in epithelial cells. Science. 2002;298:1955–1959.
- Lee HS, Nishanian TG, Mood K, et al. EphrinB1 controls cell-cell junctions through the Par polarity complex. Nat Cell Biol. 2008;10:979–986.
- Shin K, Fogg VC, Margolis B. Tight junctions and cell polarity. Annu Rev Cell Dev Biol. 2006;22:207–235.
- Barros CS, Phelps CB, Brand AH. Drosophila nonmuscle myosin II promotes the asymmetric segregation of cell fate determinants by cortical exclusion rather than active transport. Dev Cell. 2003;5:829–840.
- Kalmes A, Merdes G, Neumann B, et al. A serine-kinase associated with the p127-l(2)gl tumour suppressor of Drosophila may regulate the binding of p127 to nonmuscle myosin II heavy chain and the attachment of p127 to the plasma membrane. J Cell Sci. 1996;109(Pt 6):1359–1368.
- Plant PJ, Fawcett JP, Lin DC, et al. A polarity complex of mPar-6 and atypical PKC binds, phosphorylates and regulates mammalian Lgl. Nat Cell Biol. 2003;5:301–308.
- Raman R, Damle I, Rote R, et al. aPKC regulates apical localization of Lgl to restrict elongation of microridges in developing zebrafish epidermis. Nat Commun. 2016;7:11643.
- Tian AG, Deng WM. Lgl and its phosphorylation by aPKC regulate oocyte polarity formation in Drosophila. Development. 2008;135:463–471.
- Betschinger J, Mechtler K, Knoblich JA. The Par complex directs asymmetric cell division by phosphorylating the cytoskeletal protein Lgl. Nature. 2003;422:326–330.
- Betschinger J, Eisenhaber F, Knoblich JA. Phosphorylation-induced autoinhibition regulates the cytoskeletal protein Lethal (2) giant larvae. Curr Biol. 2005;15:276–282.
- Frank CA, Pielage J, Davis GW. A presynaptic homeostatic signaling system composed of the Eph receptor, ephexin, Cdc42, and CaV2.1 calcium channels. Neuron. 2009;61:556–569.
- Sahin M, Greer PL, Lin MZ, et al. Eph-dependent tyrosine phosphorylation of ephexin1 modulates growth cone collapse. Neuron. 2005;46:191–204.
- Shamah SM, Lin MZ, Goldberg JL, et al. EphA receptors regulate growth cone dynamics through the novel guanine nucleotide exchange factor ephexin. Cell. 2001;105:233–244.
- Mlodzik M. The dishevelled protein family: still rather a mystery after Over 20 years of molecular studies. Curr Top Dev Biol. 2016;117:75–91.
- Sharma M, Castro-Piedras I, Simmons GE Jr, et al. Dishevelled: a masterful conductor of complex Wnt signals. Cell Signal. 2018;47:52–64.
- Tanaka M, Kamo T, Ota S, et al. Association of dishevelled with Eph tyrosine kinase receptor and ephrin mediates cell repulsion. Embo J. 2003;22:847–858.
- Bergstralh DT, Lovegrove HE, St Johnston D. Discs large links spindle orientation to apical-basal polarity in Drosophila epithelia. Curr Biol. 2013;23:1707–1712.
- Peyre E, Jaouen F, Saadaoui M, et al. A lateral belt of cortical LGN and NuMA guides mitotic spindle movements and planar division in neuroepithelial cells. J Cell Biol. 2011;193:141–154.