ABSTRACT
Aneuploidy caused by abnormal chromosome segregation during early embryo development leads to embryonic death or congenital malformation. Centromere protein F (CENPF) is a member of centromere protein family that regulates chromosome segregation during mitosis. However, its necessity in early embryo development has not been fully investigated. In this study, expression and function of CENPF was investigated in mouse early embryogenesis. Detection of CENPF expression and localization revealed a cytoplasm, spindle and nuclear membrane related dynamic pattern throughout mitotic progression. Farnesyltransferase inhibitor (FTI) was employed to inhibit CENPF farnesylation in zygotes. The results showed that CENPF degradation was inhibited and its specific localization on nuclear membranes in morula and blastocyst vanished after FTI treatment. Also, CAAX motif mutation leads to failure of CENPF-C630 localization in morula and blastocyst. These results indicate that farnesylation plays a key role during CENPF degradation and localization in early embryos. To further assess CENPF function in parthenogenetic or fertilized embryos development, morpholino (MO) and Trim-Away were used to disturb CENPF function. CENPF knockdown in Metaphase II (MII) oocytes, zygotes or embryos with MO approach resulted in failure to develop into morulae and blastocysts, revealing its indispensable role in both parthenogenetic and fertilized embryos. Disturbing of CENPF with Trim-Away approach in zygotes resulted in impaired development of 2-cell and 4-cell, but did not affect the morula and blastocyst formation because of the recovered expression of CENPF. Taken together, our data suggest CENPF plays an important role during early embryonic development in mice.
Abbreviation: CENPF: centromere protein F; MO: morpholino; FTI: Farnesyltransferase inhibitor; CENPE: centromere protein E; IVF: in vitro fertilization; MII: metaphase II; SAC: spindle assembly checkpoint; Mad1: mitotic arrest deficient 1; BUB1: budding uninhibited by benzimidazole 1; BUBR1: BUB1 mitotic checkpoint serine/threonine kinase B; Cdc20: cell division cycle 20
Introduction
Cell proliferation is essential for mammal embryogenesis prior to uterine implantation. Appropriate chromosomes separation during cell proliferation guarantees successful offspring birth. The centromere is a specialized chromosome region of a chromosome [Citation1,Citation2] that the spindle fibers attach to via the kinetochore. Centromere protein F (CENPF) is a member of the centromere protein family, an outer kinetochore component [Citation3], which are the principal sites of interaction between chromosomes with spindle microtubules [Citation4,Citation5]. CENPF is a 3210 amino acid protein, comprising mainly coiled-coil domains, two internal repeats, and several leucine heptad repeats [Citation6–Citation9]. During the cell cycle, CENPF localizes to multiple cellular structures, including the nuclear envelope in late G2/early prophase and kinetochores throughout mitosis [Citation10,Citation11]. Localization of CENPF is regulated by a nuclear localization signal [Citation12], and its degradation results from farnesylation [Citation11,Citation13]. In addition, CENPF is expressed in a cell cycle-dependent way. CENPF gradually accumulates during the cell cycle until it reaches peak levels in the G2/M phase and is rapidly degraded upon the completion of mitosis [Citation6].
In somatic cells, silencing of CENPF induces mitotic defects, including incorrect spindle assembly, misaligned chromosomes, abnormal chromosome segregation, and even cell death caused by aneuploidy [Citation14]. Absence of CENPF does not compromise the spindle assembly checkpoint (SAC), and the chromosome alignment defect induced by CENPF RNA interference is accompanied by a prolonged mitosis, indicating checkpoint activation [Citation7]. It has been shown that defective spindle formation and chromosomes in CENPF deleted cells are caused by reduced centromere protein E (CENPE) at the kinetochore in a microtubule-dependent manner [Citation15]. Additionally, the amount of CENPF protein available is key for its function. Bomont et al. found that CENPF knockdown resulted in two different phenotypes that were dependent on the protein expression level of CENPF [Citation16]. The phenotypes were as follows: (1) Complete CENPF depletion resulted in the failure of kinetochore assembly and proper chromosome segregation, which was consistent with the findings of Laoukili et al. [Citation14]. (2) Partial CENPF knockdown caused instability of the kinetochore-microtubule structure, resulting in mitotic delay.
In previous studies, loss of CENPF leads to G2/M arrest [Citation17], chromosome segregation defects [Citation16], mitotic division delay [Citation18], and cell death [Citation15]. The mechanism of CENPF-dependent cell cycle regulation may depend on interaction with Rab5 [Citation18,Citation19], budding uninhibited by benzimidazole 1 (BUB1) [Citation20], cell division cycle 20 (Cdc20) [Citation21], and CENPE [Citation22]. Loss of CENPF may cause reductions in a series of mitotic checkpoint proteins, such as mitotic arrest deficient 1 (Mad1), BUB1, Mad2 and BUB1 mitotic checkpoint serine/threonine kinase B (BUBR1). The fact that after overnight nocodazole treatment cells depleted of CENPF accumulated fewer mitotic cells than those seen for control cells indicate CENPF is required for cell to sustain robust mitotic checkpoint [Citation23]. Interestingly, CENPF is overexpressed in most cancers, including breast cancer [Citation24–Citation26], hepatocellular carcinomas [Citation27], and esophageal squamous cell carcinomas [Citation28].
Currently, there remains only one study reporting that embryonic development was inhibited at the eight-cell stage with CENPF knockdown in bovine [Citation29]. Systematic study on the necessary of CENPF during early embryo development has not been conducted yet. Here, we reveal by loss-of-function that CENPF is essential for embryogenesis in mice. These results may provide support the clinical research in human infertility.
Materials and methods
Ethics statement and animal feeding regimens
All study procedures were in adherence with the National Research Council Guide for the Care and Use of Laboratory Animals and were approved by the Institutional Animal Care and Use Committee at the Inner Mongolia University (Approval number: SYXK 2014–0002). Mice were maintained under the care of the SPF laboratory animal facility in Inner Mongolia University. Mice were kept at a constant temperature of 22 ± 2°C on a 12 h light/dark cycle and had unrestricted access to food and water.
Parthenogenetic activation, in vitro fertilization (IVF) and embryo culture
MII stage oocytes after MO injection were used for parthenogenetic activation. Parthenogenetic embryos were generated by incubating the MII oocytes for 6 h in calcium-free CZB-Glutamine medium supplemented with 10 mM SrCl2 and 5 mg/ml cytochalasin B. Adult male ICR mice (8–10 weeks of age) were used for sperm collection. The sperm suspension was capacitated for 2 h in T6 medium supplemented with 10 mg/ml BSA. MII oocytes were incubated with spermatozoa for 6 h in T6 medium supplemented with 20 mg/ml BSA. The zygotes were collected and cultured in CZB medium without glucose under a humidified atmosphere of 5% CO2 at 37°C for the first 48 h and then transferred to CZB medium supplemented with 5.5 mM glucose when embryos reached the 4-cell stage. The percent of the 2-cell stage was used for fertilization evaluation. Embryos were checked at 48, 72, and 96 h after fertilization to calculate the percentage of 4-cell stage embryos, morulae, and blastocysts, respectively.
Immunoblot analysis
A total of 100 embryos per sample were lysed in Laemmli sample buffer (Bio-Rad Hercules, CA, USA). All the other western blot procedures were conducted as previously reported [Citation30]. Rabbit anti-CENPF (1:500, Abcam, Cambridge, UK), mouse anti-TUBB (1:500, Abcam) and mouse anti-Myc tag (1:500, Thermo Fisher Scientific, MA, USA) were used as primary antibodies. Bands on nitrocellulose membranes were detected using an Enhanced Chemiluminescence Detection Kit (Thermo Fisher Scientific) and captured by Chemiluminescence Imaging System (Tanon, 5200, Shanghai, China).
Immunofluorescence
Embryos were fixed in 4% paraformaldehyde (Electron Microscopy Sciences, Hatfield, PA, USA) for 50 min at room temperature, followed by permeabilization with 0.5%Triton X-100 for 2 h. Samples were then blocked with 1% BSA for 1 h and incubated with primary antibodies including sheep anti-CENPF (1:700, a gift from Prof. Stephen S. Taylor), mouse anti-β tubulin (1:1000, Abcam) and mouse anti-Myc tag (1:100, Thermo Fisher Scientific) overnight at 4°C. Embryos were labeled with secondary antibodies for 1 h. DNA was stained with 10 μg/ml Hoechst 33342 (Sigma-Aldrich, St. Louis, MO, USA).
Drug treatment
Farnesyltransferase inhibitor (FTI) SCH66336 (Selleck Chemicals, Houston, Texas, USA) was used to inhibit farnesylation of early embryos. SCH66336 was dissolved in DMSO to make a stock solution at 1 mM and diluted in CZB medium to final concentrations of 1 µM, 3 µM, and 10 µM before use.
Microinjection of CENPF morpholino (MO)
CENPF MOs designed for translational block (named as CENPF-MO-1) and the one designed for splice inhibit (named as CENPF-MO-2) were synthesized by GeneTool (Philomath, OR, USA). CENPF-MO-1 (5ʹ-GGC CCA GCT CAT CTT GTT TTA TTT T-3ʹ) and CENPF-MO-2 (5ʹ-GCC TGC CAC ACT GAT ACC TTC TG-3ʹ) were injected into oocytes or embryos, respectively, to knockdown CENPF protein levels. The sequence Ctrl-MO-1 (5ʹ-CCT CTT ACC TCA GTT ACA ATT TAT A-3ʹ) and Ctrl-MO-2 (5ʹ-GCC TCC GAC ACT CAT AGC TTG TG-3ʹ) were used as the control of CENPF-MO-1 and CENPF-MO-2, respectively. Total amount of 5 pl MO in 1 mM was microinjected into each MII oocyte or embryo. Parthenogenetic activation was used to evaluate embryonic development after MII stage oocytes were injected with CENPF MO or Ctrl MO. Zygotes were injected with CENPF MO and then cultured in CZB medium for further development. For 2-cell injection, CENPF MO labeled with carboxyfluorescein was injected into one blastomere, Ctrl MO labeled with lissamine was injected into another blastomere.
Cenpf-c630 plasmid construction and in vitro transcription
Oocyte total RNA were used to conduct reverse transcription and generate cDNA. Total of 1893 bp (C630-WT, from 7102 bp to 8994 bp) of Mouse Cenpf was cloned. Two mutants (C630-V:D, valine mutated to aspartic acid; C630-Δ4, loss of CAAX) with mutations of CAAX motif were cloned with the specific primers. Forward primer was common: 5ʹ-TCA GAA ATT CAA AGA ATG GCC CAA A-3ʹ; C630-WT reverse primer was 5ʹ-TCA CTG GAC CCG GCA GTT TTC G-3ʹ; C630-V:D reverse primer was 5ʹ-TCA CTG GTC CCG GCA GTT TTC G-3ʹ; C630-Δ4 reverse primer was 5ʹ-TCA GTT TTC GTT GTT CGT GGG ATC A-3ʹ. The sequences coding C630 were cloned in pCS2-myc plasmid. mMESSAGE mMACHINE® SP6 Transcription Kit (Thermo Fisher Scientific) was used to produce capped mRNA and the products were purified by MEGAclear™ Kit (Thermo Fisher Scientific). mRNA was dissolved in nuclease-free water to a concentration of 400 μg/ml before injection into zygotes.
Trim-away for CENPF degradation
pRK5-Trim21 plasmid is a gift from Prof. Heng-Yu Fan of Zhejiang University. mMESSAGE mMACHINE® T7 Transcription Kit (Thermo Fisher Scientific) was used for pRK5-Trim21 plasmid transcription. Mixtures of TRIM21 mRNA and CENPF antibody were directly injected into zygotes or 2-cell embryos. The final concentration of TRIM21 mRNA and CENPF antibody for injection is 1 mg/ml and 1.25 mg/ml, respectively. Zygotes or 2-cell embryos were cultured in CZB medium for further development.
Confocal microscopy and time-lapse live imaging
The stained samples were mounted on glass slides using Vectashield mounting media and examined with a laser-scanning confocal microscope (Nikon, A1R, Japan). Optical sections were captured at 2-μm intervals. Images were analyzed with NIS-Element AR3.0 software (Nikon).
For time-lapse live imaging, a Zeiss LSM880 confocal microscope was controlled by an autofocus macro. Images were gathered every 1 h for 70 h. Data analysis was performed using Zeiss Blue software.
Statistical analysis
The data are presented as the mean ± S.D. from three replicate experiments. Cell number per embryo was analyzed using two-tailed Student’s t-tests for absolute values and were calculated in Excel software (Microsoft). The percentages for early embryo development (2-cell, 4-cell, morula, and blastocyst stages) were analyzed using nonparametric Kruskal–Wallis tests in SPSS 19.0 software (IBM, Armonk, NY). P < 0.05 was determined as statistically significant.
Results
Expression and localization of CENPF during mouse embryo preimplantation
Western blot analyses revealed that CENPF can be detected at all stages during early embryo development. Expression of CENPF gradually decreased from the 2-cell embryo stage to the lowest amount at the blastocyst stage (), indicating CENPF protein degradation with embryogenesis progression.
Figure 1. Expression and subcellular localization of CENPF during early embryo development. a. CENPF expression was detected by western blot. TUBB was used as a loading control. Experiments were performed as triplicates. b. and c. Early embryos at different stages were stained with antibody to detect CENPF. DNA was stained with Hoechst 33342. The slides were examined under a confocal microscope. Scale bar, 20 μm.
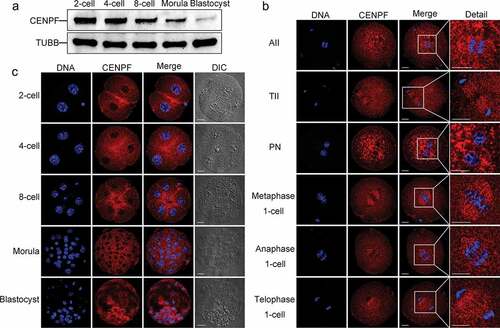
Immunofluorescence confocal staining showed that CENPF can be observed in cytoplasm throughout the embryo development, and this may be due to its function of mitochondria transport. Specifically, CENPF localized to spindle region during anaphase II after oocyte fertilization and disaggregated from centromeres during telophase II. Upon pronucleus formation, CENPF translocated to nuclear membranes. During the first mitosis of zygotes, CENPF accumulated to chromosome centromeres and spindles during metaphase and anaphase and disaggregated from centromeres during telophase (). During zygote mitotic division, CENPF localized to nuclear membranes at the 2-cell, 4-cell, 8-cell, morula, and blastocyst stages. Besides, some localization was observed at the cortical area between daughter cells at the 2-cell, 4-cell and 8-cell embryo stages ().
CENPF degradation and localization were dependent on farnesylation during early embryo development
It is interesting that CENPF expression showed a continuously decreasing pattern from 2-cell to blastocyst. We want to know if farnesylation is responsible for this decreasing pattern, like its role in CENPF degradation and localization in somatic cells. In our study, zygotes were cultured in CZB medium with different concentrations of FTI SCH66336 to inhibit CENPF farnesylation and therefore its degradation. Samples were collected at 12, 24, 36, 48, 72 and 96 h for immunoblotting or immunofluorescence (). For dose optimization, 1 μM, 3 μM, and 10 μM SCH66336 were used. The data showed that morula and blastocyst rates were reduced when 3 μM and 10 μM SCH66336 concentrations were used (Figure S1). After FTI treatment, we collected embryos for western blots. Zygotes treated with FTI for 12 h (), 24 h (), 36 h (), 48 h (), and 72 h () did not exhibit significant changes in CENPF expression levels. However, a treatment time of 96 h resulted in a significant elevation in CENPF expression (), suggesting delayed CENPF degradation due to the required modification of farnesylation. Further detection of CENPF localization showed that after 12 h and 24 h of FTI treatment, CENPF localized to the nuclear membranes of 2-cell stage embryos (. After 36 h and 48 h of FTI treatment, CENPF remained localized to the nuclear membranes at 4-cell stage embryos (. However, when zygotes were treated with 3 μM and 10 μM FTI for 72 h or 96 h, CENPF signals on the nuclear membranes disappeared (.
Figure 2. CENPF localization during early embryo development was farnesylation dependent. a. Illustration of FTI SCH66336 treatment on zygotes. Zygotes were collected and cultured in medium with different concentrations of FTI. Samples were collected at 12 h, 24 h, 36 h, 48 h, 72 h, and 96 h for immunoblotting and immunofluorescence analyses. CENPF expression after FTI treatment for b. 12 h, c. 24 h, d. 36 h, e. 48 h, f. 72 h, and g. 96 h; TUBB was used as a loading control. Localization of CENPF during different early embryo stages after FTI treatment for h. 12 h, i. 24 h, j. 36 h, k. 48 h, l. 72 h, and m. 96 h. DNA was stained with Hoechst 33342. White arrows indicate CENPF localization to nuclear membranes. White frame indicates the region shown in detail. Scale bar, 20 μm.
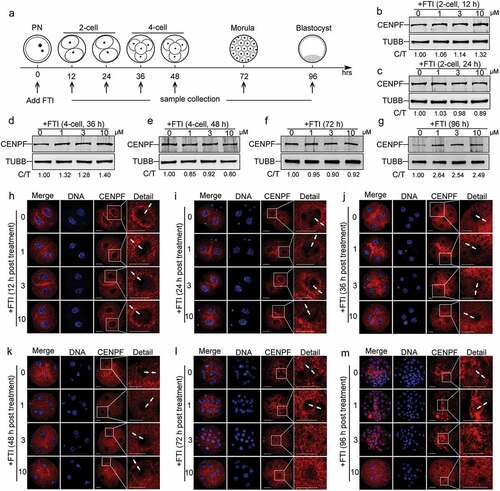
To confirm farnesylation roles in CENPF degradation and localization, we expressed the C-terminal 630 amino acids of CENPF, referred to here as C630. Specific mutants of CENPF-C630 with CAAX motif mutation were used. We replaced valine with aspartic acid, defined as C630-V:D; deleted the CAAX motif, defined as C630-Δ4 (). C630 mRNA was injected into zygotes and treated with FTI SCH66336 for 96 h. Western blot results showed that when CAAX was mutated or deleted, C630 degradation was inhibited. In addition, FTI treatment can lead to failure of C630-WT degradation, which indicated that farnesylation is essential for CENPF degradation (). Differed from the endogenous CENPF localization to nuclear membrane, exogenous C630 localized to nuclear at 2-cell. CAAX motif mutation and FTI treatment did not affect C630 localization in 4-cell (), but lead to failure of its localization in morula () and blastocyst (). Combined with the results of FTI treatment on endogenous CENPF, our results indicated that farnesylation is essential for CENPF localization during embryo development after morula formation.
Figure 3. CENPF-C630 localization during early embryo development was farnesylation dependent. a. Schematic of C630 and C630 mutants. b. Immunoblot showing the relative expression levels of C630 and the CAAX mutants with or without FTI treatment. Samples were collected at 96 h after 10 μM SCH66336 treatment. C630 expression was detected using anti-cMyc antibody. TUBB was used as a loading control. Experiments were performed as triplicates. Localization of C630 and the CAAX mutants at c. 4-cell (48 h), d. morula (72 h), and e. blastocyst (96 h) with or without FTI treatment. Embryos were stained with anti-cMyc antibody. DNA was stained with Hoechst 33342. Scale bar, 20 μm.
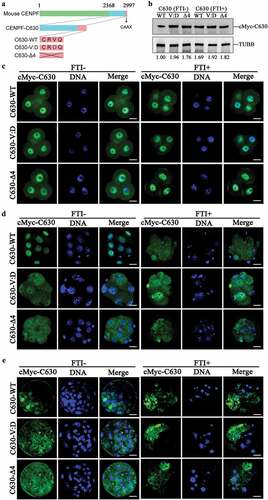
Depletion of CENPF in MII stage oocytes impaired parthenogenetic embryo development
To further demonstrate that depletion of CENPF affects parthenogenetic embryo development, MII oocytes were injected with CENPF-MO for gene knockdown and parthenogenetic activation was performed. Parthenogenetic embryos were collected for western blot analysis 48 h after CENPF-MO injection. Data confirmed that CENPF expression was significantly reduced and CENPF localization was disappeared after CENPF-MO injection (. There was no statistically significant difference between the control and CENPF-MO-injected groups in the percentage of 2-cell stage embryos. However, CENPF depletion significantly reduced the formation of embryos from the 4-cell (P < 0.01) to blastocyst stages (P < 0.01) (). Moreover, CENPF knockdown reduced blastomere numbers per embryo 78 h post-MO injection compared with the control group; most embryos were arrested at the 8-cell stage (P < 0.001) (. Furthermore, population-based images of blastocysts demonstrated significantly lower percentages after CENPF depletion (). Collectively, these results indicate that depletion of CENPF in MII stage oocytes results in abnormalities and impairment of embryo development.
Figure 4. Depletion of CENPF in MII oocytes by CENPF-MO-1 injection caused early embryo development failure. a. Western blot of CENPF expression after control or CENPF-MO injection. TUBB was used as a loading control. One representative experiment is shown. b. Localization of CENPF at 4-cell after Ctrl-MO-1 or CENPF-MO-1 injected into MII stage oocytes. Embryos were stained with anti-CENPF antibody. DNA was stained with Hoechst 33342. Scale bar, 20 μm. c. Percentages for embryonic developmental stages of cultured MO-injected MII oocytes. d. Morphology of embryos. White-dashed lines indicate embryos. DNA was stained with Hoechst 33342. Scale bar, 30 μm. e. Cell numbers per embryo 78 h after MO injection of MII oocytes. f. Image of blastocysts 102 h after MO injection. Black arrows indicate the embryos shown in detail in the lower corners. Scale bar, 50 μm. Quantified data are shown as mean ± S.D. **P < 0.01; ***P < 0.001.
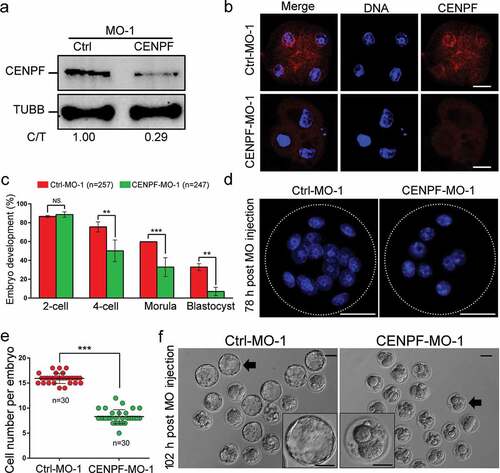
Depletion of CENPF in zygotes impaired IVF embryo development
Necessity of CENPF in fertilized embryo development was assessed with IVF embryo. We knocked down CENPF in the zygotes using CENPF-MOs. To confirm the phenotype caused by the CENPF-MO-1 is specific, we also designed CENPF-MO-2, which can block CENPF mRNA splice. We confirmed CENPF knockdown by western blot and found that CENPF expression was significantly reduced ( & Figure S2A) and CENPF localization was lost (Figure S2B) 48 h after CENPF-MO-2 injection. Similar to the results of knockdown in MII stage oocyte, no statistically significant difference was observed between the control and CENPF-MO groups in the percentage of 2-cell stage embryos (P> 0.05). However, CENPF depletion significantly reduced the formation of 4-cell, morula (P < 0.05), and blastocyst (P < 0.001) ( & Figure S2C). Moreover, CENPF depletion results in 8-cell stage arrest 72 h after CENPF-MO injection (P < 0.001) (, Figure S2D, & E). CENPF depletion also damaged final blastocyst formation ( & Figure S2F). Collectively, these results indicate that depletion of CENPF in zygotes results in abnormalities and impairment of embryo development in specifically.
Figure 5. Depletion of CENPF in zygotes by CENPF-MO-1 injection caused early embryo development failure. a. CENPF expression analysis after MO injection. Zygotes injected with Ctrl-MO or CENPF-MO were cultured to the 4-cell stage for western blot analysis. TUBB was used as a loading control. The results of one representative experiment of three independent replicates are presented. b. CENPF-MO injection caused early embryo development failure. c. Morphology of embryos. White-dashed lines indicate embryos. DNA was stained with Hoechst 33342. Scale bar, 30 μm. d. Cell numbers per embryo 72 h after MO injection of zygotes. e. CENPF-MO injection caused blastocyst formation failure. Images were acquired 96 h after MO injection. Black arrows indicate the embryos shown in detail in the lower corners. Scale bar, 50 μm. Quantified data are shown as mean ± S.D. * P < 0.05; ***P < 0.001.
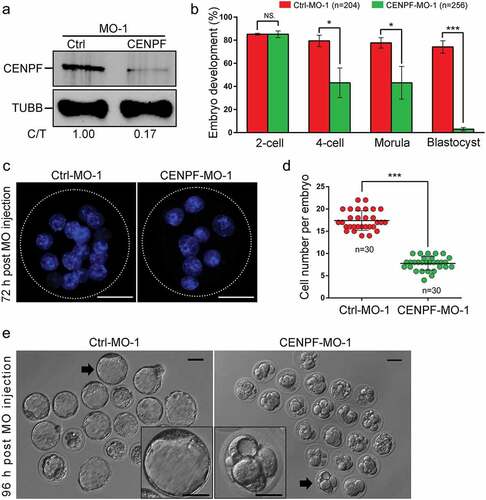
Figure 6. Depletion of CENPF in 2-cell stage embryos by MO injection resulted in early embryo development failure. a. Scheme of injection of 2-cell stage embryos for the three MO experimental groups. Both blastomeres of a 2-cell stage embryo were injected with Ctrl-MO, defined as “Ctrl-Ctrl”. One blastomere of a 2-cell stage embryo was injected with Ctrl-MO and the other blastomere was injected with CENPF-MO, defined as “Ctrl-CENPF”; both two blastomeres of 2-cell were injected with CENPF-MO, defined as “CENPF-CENPF”. b. Western blot analysis after CENPF-MO injection. 2-cell stage oocytes injected with MO were cultured to the morula stage prior to sample collection. The results of one representative experiment of three independent replicates are presented. TUBB was used as a loading control. c. CENPF-MO injection in 2-cell stage embryos caused early embryo development failure. Different superscripts (a, b, and c) on the bars indicate statistical difference (P < 0.01). d. Morphology of embryos. White-dashed lines indicate embryos. DNA was stained with Hoechst 33342. Scale bar, 30 μm. e. Cell numbers per embryo 48 h after MO injection of 2-cell stage embryos. Quantified data are shown as mean ± S.D. ***P < 0.001. f. Image of embryos 48 h and 72 h after MO injection. Black arrows indicate the embryos with blastocoels. Scale bar, 50 μm. g. Time-lapse imaging of 2-cell stage embryo after Ctrl-MO (red) or CENPF-MO (green) injection.
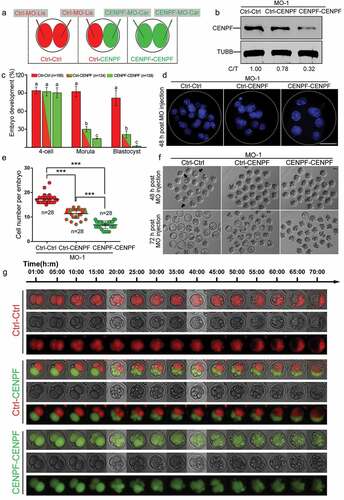
Depletion of CENPF in 2-cell stage embryos impaired early embryo development
To determine CENPF elimination has negative effects on embryo development after zygotic genome activation, 2-cell stage embryos were injected with two different MOs labeled with fluorophores. Ctrl-MO was labeled with lissamine and CENPF-MO was labeled with carboxyfluorescein. Three different experimental groups were designated as follows: (1) Ctrl-Ctrl group, the blastomeres of a 2-cell stage embryo were both injected with Ctrl-MO; (2) Ctrl-CENPF group, one blastomere of a 2-cell embryo was injected with Ctrl-MO, the other blastomere was injected with CENPF-MO; (3) CENPF-CENPF group, both blastomeres of a 2-cell stage embryo were injected with CENPF-MO (). CENPF expression was significantly decreased in the Ctrl-CENPF group, and further decreased in the CENPF-CENPF group (). No significant differences were observed at the 4-cell stage between the Ctrl-Ctrl, Ctrl-CENPF and CENPF-CENPF groups. High percentages were observed in Ctrl-Ctrl group for morula and blastocyst formation. In contrast, relatively low percentages of morulae and blastocysts were observed in the Ctrl-CENPF group (P < 0.01); the lowest percentages of morulae and blastocysts were observed in the CENPF-CENPF group compared to the Ctrl-Ctrl group (P < 0.01) (). Cell numbers per embryo were calculated at 48 h after MO injection. The Ctrl-CENPF group had a lower cell number per embryo compared to the Ctrl-Ctrl group (P < 0.001); however, the CENPF-CENPF group had the lowest cell number per embryo compared to the Ctrl-Ctrl group (P < 0.001) and Ctrl-CENPF group (P < 0.001), respectively (. Moreover, blastocoels formed at 48 h after MO injection in the Ctrl-Ctrl group, but none were observed in the Ctrl-CENPF and CENPF-CENPF groups. At 72 h after MO injection, most of the embryos in the Ctrl-Ctrl group developed into blastocysts; fewer were observed in the Ctrl-CENPF group and almost none were observed in the CENPF-CENPF group ().
We recorded embryo development after MO injection using time-lapse imaging. The two blastomeres injected with Ctrl-MO completed division and reached the 4-cell stage after 10 h and blastocoels appeared after 55 h (; Supplementary Movie 1). For the Ctrl-CENPF group, the blastomere injected with Ctrl-MO completed division after 15 h, while the development of the blastomere injected with CENPF-MO was delayed. At last, the blastomere injected with Ctrl-MO developed into a blastocyst (red part), but the blastomere injected with CENPF-MO became fragmentations (green part) that were pushed aside by the normal embryos (red) (; Supplementary Movie 2). For the CENPF-CENPF group, the embryo became fragmented and failed to develop further (; Supplementary Movie 3).
Depletion of CENPF in zygotes and 2-cell stage embryos with trim-away impaired early embryo development
Trim-Away is a technique which was invented recently to degrade endogenous proteins acutely in mammalian cells [Citation31,Citation32]. We used Trim-Away to knockdown CENPF in zygotes and 2-cells. Western blot results showed that 4 h after mixture of TRIM21 and anti-CENPF injected into zygotes, CENPF level was reduced acutely. This reduction was maintained at 48 h, and recovered after 72 h of injection (). We injected TRIM21+ IgG into one blastomere and TRIM21+ anti-CENPF into another blastomere of 2-cell stage embryos. The results showed that at 2 h, 3 h and 4 h after injection, CENPF localization can be detected by immunostaining in the blastomere which injected with TRIM21+ IgG, while the other blastomere injected with TRIM21+ anti-CENPF has no specific staining. Interesting, blastomeres division were delayed at 12 h and 18 h when CENPF was depleted ( & Figure S3). However, in morula and blastocyst, CENPF localization was recovered even TRIM21+ anti-CENPF was injected into zygotes or 2-cells (), indicating the possible exhausting of TRIM21 or CENPF antibody enable the re-expression of CENPF. CENPF depletion in zygotes significantly reduced the percent of 2-cell and 4-cell formation (P < 0.05). However, due to the re-expression of CENPF after 72 h of TRIM21 injection, the percent of morula and blastocyst formation was not affected (P> 0.05) (.
Figure 7. Depletion of CENPF using Trim-Away approach resulted in early embryo development failure. a. CENPF expression analysis after Trim-Away in zygotes. Zygotes injected with TRIM21+ IgG or TRIM21+ anti-CENPF were cultured to 4 h, 34 h and 72 h for western blot analysis. TUBB was used as a loading control. The results of one representative experiment of three independent replicates are presented. b. CENPF localization at 2 h, 3 h, 4 h, 12 h, 18 h, and 20 h after TRIM21+ IgG and TRIM21+ anti-CENPF injected into different blastomeres of 2-cells. White arrows showed the blastomere injected with TRIM21+ IgG, and black arrows showed the blastomere injected with TRIM21+ anti-CENPF. DNA was stained with Hoechst 33342. Scale bar, 20 μm. c. CENPF localization in morula (72 h or 48 h) and blastocyst (96 h or 72 h) after TRIM21 + IgG and TRIM21 + anti-CENPF injected into zygotes or 2-cells. Embryos were stained with anti-CENPF antibody. DNA was stained with Hoechst 33342. Scale bar, 20 μm. d. TRIM21+ anti-CENPF injection into zygotes caused early embryo development failure. Quantified data are shown as mean ± S.D. *P < 0.05. e. Image of blastocyst at 96 h after TRIM21 + IgG or TRIM21 + anti-CENPF injected into zygotes. Scale bar, 50 μm.
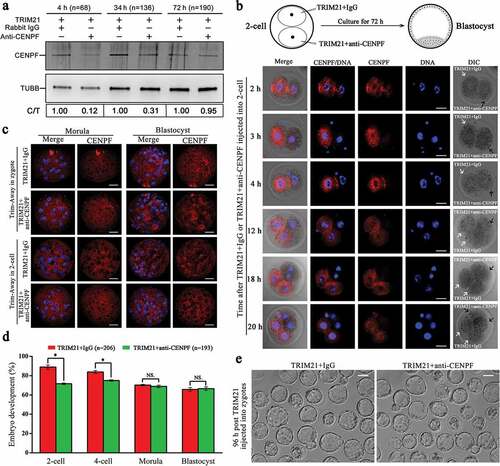
Discussion
CENPF plays a key role in mitosis [Citation33] in various cell types such as myocytes [Citation34], breast cancer cells [Citation25], human salivary gland tumor cells [Citation35], and bovine embryos [Citation29]. This is the first study reporting CENPF necessity during embryo preimplantation in mice. CENPF expression was gradually reduced from the 8-cell to blastocyst stages and could be due to maternal CENPF degradation. Localization of CENPF in cytoplasm throughout the embryo development is probably due to its function of mitochondria transportation [Citation36]. At the onset of anaphase, CENPF translocate from kinetochores to central spindles and later accumulates at intracellular bridges between daughter cells during telophase [Citation6,Citation11]. Different from somatic cells, CENPF localized on spindle area during metaphase, and no localization was observed at intracellular bridge area between daughter cells during telophase of the first mitotic division of zygotes. However, CENPF localization in the interphase after zygote was similar to that in somatic cells.
The experiments of FTI treatments were based on the interesting found that CENPF expression showed a continue decreasing pattern from 2-cell to blastocyst. It has been reported that farnesylation of CENPF is required for its localization and degradation in somatic cells [Citation13,Citation21,Citation37]. Does the same mechanism regulate CENPF localization and degradation in early stage embryos? Although hundreds of proteins farnesylation can be modified by FTI [Citation38,Citation39], use of this inhibitor can still illustrate the effect of farnesylation modification on CENPF’s localization and degradation in embryos. In proliferating cancer cells, CENPF localization to metaphase kinetochores, but not prometaphase kinetochores, was lost after FTI treatment [Citation37]. In contrary, some studies reported that FTI treatment did not affect CENPF localization to kinetochores in human lung cancer cells [Citation40], skin fibroblasts [Citation41], and A549 cells [Citation42]. Compared with the previous studies, our results showed that FTI did not affect CENPF localization or expression during early embryo stages. However, CENPF degradation was inhibited 96 h after FTI treatment. Furthermore, CENPF localization on nuclear membranes disappeared when the embryos were cultured in high FTI concentrations (3 μM and 10 μM) for 72 and 96 h. The experiments of overexpression of exogenous C630 showed the similar results. Interestingly, CENPF inside embryos need different concentrations of FTI treatment time to inhibit its farnesylation. In some reports, 1 μM SCH66336 were used in HeLa cells for 18 h [Citation13], in hTCLs, A549, and NCI-H460 cells for 72 h to inhibit CENPF farnesylation [Citation41]. The FTI SCH66336 concentrations were determined according to the type of cells. It has been reported that 5 μM FTI was used to treat human HNSCC cell lines (UMSCC38 and SqCC/Y1) [Citation43]. The IC50 of SCH66336 in CR1R12 cell line was 9 μM [Citation44]. In another study, higher FTI concentration was used. B16, Colo 853 or NIH 3T3 cells were treated with increasing concentrations of SCH66336 (3–30 μM) for 72 h [Citation45]. We believe the significant differences of cell types between early embryos (or oocytes) and somatic cells determine the IC50 of FTI.
CENPF is an indispensable regulator of chromosome segregation during cellular division via microtubule dynamics [Citation33]. CENPF potentially acts as a transporter of cellular cargoes by attaching them to dynamic microtubule ends during polym II and in one-cell stage metaphase and anaphase erization and depolymerization of tubulin [Citation46]. Furthermore, CENPF promotes anterograde mitochondrial movement and proper mitochondrial distribution in daughter cells by interacting with Miro [Citation36]. Compared with the IVF embryos, parthenogenetic embryos lack the paternal gene expression [Citation47]. Several epigenetic modifications, such as DNA methylation and histone modification, have been observed to be lacked during the development of parthenogenetic embryos because of the deficiency of HDAC1 and DNMT1 expression [Citation48]. In addition, down-regulated expression of TET1, TET2, and TET3 has been confirmed in parthenogenetic embryos [Citation49]. To detect CENPF function in both IVF and parthenogenetic embryos, MII oocytes and zygotes were used for CENPF knockdown. In our study, loss of CENPF in MII stage oocytes and zygotes leads to 8-cell stage arrest, These results are consistent with the study in bovine, showing that depletion of CENPF mRNA in bovine zygotes leads to a dramatic reduction of developmental competence after embryonic genome activation [Citation29].
In theory, both blastomeres of 2-cell are totipotent [Citation50]. Even one blastomere failed to development after CENPF-MO injection, the normal blastomere can still form blastocyst. However, in our study, knockdown of CENPF in one blastomere of 2-cell lead to failure of blastocyst formation in most embryos. Recent studies reported that cell fate in mammalian 2-cell embryos is biased [Citation51,Citation52]. We speculate that the function of blastomere without CENPF cannot be compensated by another normal blastomere, finally leading to the impaired blastocyst formation in Ctrl-CENPF group.
Compared with the approach of MO, Trim-Away is an ideal technique to degrade proteins more rapidly [Citation31,Citation32,Citation53]. CENPF level was reduced significantly with Trim-Away in our study in a short time. The phenotypes caused by CENPF Trim-Away were similar to those caused by the MOs in early embryo stages. A noteworthy phenomenon is that CENPF Trim-Away did not affect morula or blastocyst formation, which dues to the re-expression of CENPF after TRIM21 exhausting in latter stage. A recent study on zebrafish embryos reported that TRIM21 can be exhausted at 25 h after injection. Injection of TRIM21 and an anti-Ddx19B antibody into the embryo yolks caused small eyes, but these phenotypes gradually recovered with time and were completely restored at 5 days postfertilization [Citation54].
In sum, our study illustrated that CENPF is necessary for mouse early embryo development, and functional disturbing of CENPF with MO or Trim-Away approach will impair the developmental potential of early stage embryos.
Author’s roles
C.G.L., C.J.Z.: conceived and designed experiments; C.J.Z., X.Y.W., Z.H., D.H.W.: performed experiments; C.J.Z., C.G.L.: analyzed data. C.J.Z., Y.Z.M., C.G.L.: contributed to writing the manuscript.
Correction Statement
This article has been republished with minor changes. These changes do not impact the academic content of the article.
Supplemental Material
Download QuickTime Video (4.3 MB)Supplemental Material
Download QuickTime Video (3.8 MB)Supplemental Material
Download QuickTime Video (3.9 MB)Supplemental Material
Download QuickTime Video (3.9 MB)Supplemental Material
Download Zip (16.7 MB)Acknowledgments
We thank Prof. Stephen S. Taylor at the University of Manchester for providing CENPF antibody. We also thank Prof. Heng-Yu Fan at Zhejiang University for providing pRK5-Trim21 plasmid.
Disclosure statement
No potential conflict of interest was reported by the authors.
Supplementary Material
Supplemental data for this article can be accessed here.
Additional information
Funding
References
- Cleveland DW, Mao Y, Sullivan KF. Centromeres and kinetochores: from epigenetics to mitotic checkpoint signaling. Cell. 2003;112:407–421.
- Giunta S, Funabiki H Integrity of the human centromere DNA repeats is protected by CENP-A, CENP-C, and CENP-T. Proc Natl Acad Sci USA 2017; 114:1928–1933.
- Wan X, O’Quinn RP, Pierce HL, et al. Protein architecture of the human kinetochore microtubule attachment site. Cell. 2009;137:672–684.
- Karpen GH, Allshire RC. The case for epigenetic effects on centromere identity and function. Trends Genet. 1997;13:489–496.
- Walczak CE, Cai S, Khodjakov A. Mechanisms of chromosome behaviour during mitosis. Nat Rev Mol Cell Biol. 2010;11:91–102.
- Liao H, Winkfein RJ, Mack G, et al. CENP-F is a protein of the nuclear matrix that assembles onto kinetochores at late G2 and is rapidly degraded after mitosis. J Cell Biol. 1995;130:507–518.
- Holt SV, Vergnolle MA, Hussein D, et al. Silencing Cenp-F weakens centromeric cohesion, prevents chromosome alignment and activates the spindle checkpoint. J Cell Sci. 2005;118:4889–4900.
- Welner S, Trier NH, Frisch M, et al. Correlation between centromere protein-F autoantibodies and cancer analyzed by enzyme-linked immunosorbent assay. Mol Cancer. 2013;12:95.
- Zhu X. Structural requirements and dynamics of mitosin-kinetochore interaction in M phase. Mol Cell Biol. 1999;19:1016–1024.
- Berto A, Doye V. Regulation of Cenp-F localization to nuclear pores and kinetochores. Cell Cycle. 2018;17:2122–2133.
- Rattner JB, Rao A, Fritzler MJ, et al. CENP-F is a .ca 400 kDa kinetochore protein that exhibits a cell-cycle dependent localization. Cell Motility Cytoskeleton. 1993;26:214–226.
- Loftus KM, Cui H, Coutavas E, King DS, Ceravolo A, Pereiras D, Solmaz SR. Mechanism for G2 phase-specific nuclear export of the kinetochore protein CENP-F. Cell Cycle. 2017;16:1414–1429.
- Hussein D, Taylor SS. Farnesylation of Cenp-F is required for G2/M progression and degradation after mitosis. J Cell Sci. 2002;115:3403–3414.
- Laoukili J, Kooistra MR, Bras A, et al. FoxM1 is required for execution of the mitotic programme and chromosome stability. Nat Cell Biol. 2005;7:126–136.
- Yang Z, Guo J, Chen Q, et al. Silencing mitosin induces misaligned chromosomes, premature chromosome decondensation before anaphase onset, and mitotic cell death. Mol Cell Biol. 2005;25:4062–4074.
- Bomont P, Maddox P, Shah JV, et al. Unstable microtubule capture at kinetochores depleted of the centromere-associated protein CENP-F. Embo J. 2005;24:3927–3939.
- Dai Y, Liu L, Zeng T, et al. Characterization of the oncogenic function of centromere protein F in hepatocellular carcinoma. Biochem Biophys Res Commun. 2013;436:711–718.
- Serio G, Margaria V, Jensen S, et al. Small GTPase Rab5 participates in chromosome congression and regulates localization of the centromere-associated protein CENP-F to kinetochores. Proc Natl Acad Sci USA 2011; 108:17337–17342.
- Ma R, Hou X, Zhang L, et al. Rab5a is required for spindle length control and kinetochore-microtubule attachment during meiosis in oocytes. Faseb J. 2014;28:4026–4035.
- Xiao B, Verma SC, Cai Q, et al. Bub1 and CENP-F can contribute to Kaposi’s sarcoma-associated herpesvirus genome persistence by targeting LANA to kinetochores. J Virol. 2010;84:9718–9732.
- Gurden MD, Holland AJ, van Zon W, Tighe A, Vergnolle MA, Andres DA, Spielmann HP, Malumbres M, Wolthuis RM, Cleveland DW, et al. Cdc20 is required for the post-anaphase, KEN-dependent degradation of centromere protein F. J Cell Sci. 2010;123:321–330.
- Ciossani G, Overlack K, Petrovic A, et al. The kinetochore proteins CENP-E and CENP-F directly and specifically interact with distinct BUB mitotic checkpoint Ser/Thr kinases. J Biol Chem. 2018;293:10084–10101.
- Feng J, Huang H, Yen TJ. CENP-F is a novel microtubule-binding protein that is essential for kinetochore attachments and affects the duration of the mitotic checkpoint delay. Chromosoma. 2006;115:320–329.
- Ueda S, Kondoh N, Tsuda H, et al. Expression of centromere protein F (CENP-F) associated with higher FDG uptake on PET/CT, detected by cDNA microarray, predicts high-risk patients with primary breast cancer. BMC Cancer. 2008;8:384.
- Brown HK, Ottewell PD, Coleman RE, et al. The kinetochore protein Cenp-F is a potential novel target for zoledronic acid in breast cancer cells. J Cell Mol Med. 2011;15:501–513.
- Clark GM, Allred DC, Hilsenbeck SG, et al. Mitosin (a new proliferation marker) correlates with clinical outcome in node-negative breast cancer. Cancer Res. 1997;57:5505–5508.
- Kim HE, Kim DG, Lee KJ, et al. Frequent amplification of CENPF, GMNN and CDK13 genes in hepatocellular carcinomas. PloS One. 2012;7:e43223.
- Mi YJ, Gao J, Xie JD, et al. Prognostic relevance and therapeutic implications of centromere protein F expression in patients with esophageal squamous cell carcinoma. Dis Esophagus. 2013;26:636–643.
- Toralova T, Susor A, Nemcova L, et al. Silencing CENPF in bovine preimplantation embryo induces arrest at 8-cell stage. Reproduction. 2009;138:783–791.
- Lu A, Zhou CJ, Wang DH, et al. Cytoskeleton-associated protein 5 and clathrin heavy chain binding regulates spindle assembly in mouse oocytes. Oncotarget. 2017;8:17491–17503.
- Clift D, McEwan WA, Labzin LI, et al. A Method for the Acute and Rapid Degradation of Endogenous Proteins. Cell. 2017;171:1692–706 e18.
- Clift D, So C, McEwan WA, et al. Acute and rapid degradation of endogenous proteins by Trim-Away. Nat Protoc. 2018;13:2149–2175.
- Pfaltzgraff ER, Roth GM, Miller PM, et al. Loss of CENP-F results in distinct microtubule-related defects without chromosomal abnormalities. Mol Biol Cell. 2016;27:1990–1999.
- Manalo A, Schroer AK, Fenix AM, et al. Loss of CENP-F Results in Dilated Cardiomyopathy with Severe Disruption of Cardiac Myocyte Architecture. Sci Rep. 2018;8:7546.
- Shigeishi H, Mizuta K, Higashikawa K, et al. Correlation of CENP-F gene expression with tumor-proliferating activity in human salivary gland tumors. Oral Oncol. 2005;41:716–722.
- Kanfer G, Courtheoux T, Peterka M, et al. Mitotic redistribution of the mitochondrial network by Miro and Cenp-F. Nat Commun. 2015;6:8015.
- Schafer-Hales K, Iaconelli J, Snyder JP, et al. Farnesyl transferase inhibitors impair chromosomal maintenance in cell lines and human tumors by compromising CENP-E and CENP-F function. Mol Cancer Ther. 2007;6:1317–1328.
- Moudgil DK, Westcott N, Famulski JK, et al. A novel role of farnesylation in targeting a mitotic checkpoint protein, human Spindly, to kinetochores. J Cell Biol. 2015;208:881–896.
- Wright LP, Philips MR. Thematic review series: lipid posttranslational modifications. CAAX modification and membrane targeting of Ras. J Lipid Res. 2006;47:883–891.
- Crespo NC, Ohkanda J, Yen TJ, et al. The farnesyltransferase inhibitor, FTI-2153, blocks bipolar spindle formation and chromosome alignment and causes prometaphase accumulation during mitosis of human lung cancer cells. J Biol Chem. 2001;276:16161–16167.
- Ashar HR, James L, Gray K, et al. Farnesyl transferase inhibitors block the farnesylation of CENP-E and CENP-F and alter the association of CENP-E with the microtubules. J Biol Chem. 2000;275:30451–30457.
- Verstraeten VL, Peckham LA, Olive M, et al. Protein farnesylation inhibitors cause donut-shaped cell nuclei attributable to a centrosome separation defect. Proc Natl Acad Sci U S A. 2011;108:4997–5002.
- Oh SH, Kang JH, Kyu Woo J, et al. A multiplicity of anti-invasive effects of farnesyl transferase inhibitor SCH66336 in human head and neck cancer. Int J Cancer. 2012;131:537–547.
- Wang E, Casciano CN, Clement RP, Johnson WW. The farnesyl protein transferase inhibitor SCH66336 is a potent inhibitor of MDR1 product P-glycoprotein. Cancer Res. 2001;61:7525–7529.
- Smalley KS, Eisen TG. Farnesyl transferase inhibitor SCH66336 is cytostatic, pro-apoptotic and enhances chemosensitivity to cisplatin in melanoma cells. Int J Cancer. 2003;105:165–175.
- Kanfer G, Peterka M, Arzhanik VK, et al. CENP-F couples cargo to growing and shortening microtubule ends. Mol Biol Cell. 2017;28:2400–2409.
- Liu JH, Zhu JQ, Liang XW, et al. Diploid parthenogenetic embryos adopt a maternal-type methylation pattern on both sets of maternal chromosomes. Genomics. 2008;91:121–128.
- Peng M, Li Y, Huang H, et al. The expression of GCN5, HDAC1 and DNMT1 in parthenogenetically activated mouse embryos. J Obstet Gynaecol. 2015;35:131–135.
- Gao W, Yu X, Hao J, et al. Ascorbic acid improves parthenogenetic embryo development through TET proteins in mice. In: Bioscience reports. London: Portland Press; 2019. p. 39.
- Rodriguez-Terrones D, Gaume X, Ishiuchi T, Weiss A, Kopp A, Kruse K, Penning A, Vaquerizas JM, Brino L, Torres-Padilla ME. A molecular roadmap for the emergence of early-embryonic-like cells in culture. Nat Genet. 2018;50:106–119.
- Wang J, Wang L, Feng G, et al. Asymmetric Expression of LincGET Biases Cell Fate in Two-Cell Mouse Embryos. Cell. 2018;175:1887–901 e18.
- Hupalowska A, Jedrusik A, Zhu M, et al. CARM1 and Paraspeckles Regulate Pre-implantation Mouse Embryo Development. Cell. 2018;175:1902–16 e13.
- Mehlmann LM, Uliasz TF, Lowther KM. SNAP23 is required for constitutive and regulated exocytosis in mouse oocytes. Biol Reprod. 2019;101:338–346.
- Chen X, Liu M, Lou H, et al. Degradation of endogenous proteins and generation of a null-like phenotype in zebrafish using Trim-Away technology. Genome Biol. 2019;20:19.