ABSTRACT
Idiopathic pulmonary fibrosis (IPF) is a chronic, progressive, irreversible and the most common fatal interstitial lung disease, which is characterized by damaged alveolar structure, the massive proliferation of fibroblasts and deposition of extracellular matrix (ECM). While the pathogenesis of IPF remains unclear, it has been clearly established that the excessive proliferation of lung fibroblasts is the most direct cause of fibrogenesis. Numerous proliferating fibroblasts form fibrous foci and secrete a large amount of ECM to aggravate the process of pulmonary fibrosis. Tissue plasminogen activator (tPA) is a kind of serine protease, its main function is to activate zymogens into active enzymes involved in fibrinolysis. Our study found tPA functioned as a cytokine to promote the proliferation of lung fibroblasts through intracellular signaling events involving Erk1/2, p90RSK, GSK-3β phosphorylation, and cyclinD1 induction. We also uncovered that tPA indirectly activated the Wnt/β-catenin signaling pathway by regulating the GSK-3β phosphorylation level. It’s well-known that Wnt/β-catenin signaling pathway plays an important role in the pathogenesis of pulmonary fibrosis, in which the accumulation of β-catenin in the cytoplasm is an important signal of the activation of Wnt/β-catenin signaling pathway. Our study unveiled that tPA can serve as a cytokine involved in Wnt/β-catenin signaling pathway and be implicated in pulmonary fibrosis.
Induction
Idiopathic pulmonary fibrosis (IPF) is a devastating, age-related lung disease with unknown etiology and consequent few treatment options [Citation1,Citation2]. It is characterized by excessive fibroblasts proliferation, large amounts of ECM deposition and destruction of alveolar structures [Citation3]. The clinical manifestation is dyspnea and accompanied with irritating cough [Citation4]. As conventional treatments have little impact on the process of fibrosis, the condition of IPF patients continues to deteriorate and eventually the patients die of respiratory failure [Citation2,Citation5]. Although the FDA has approved two new drugs Pirfenidone and Nidanib in 2014, these two drugs can only slowdown the pulmonary dysfunction but neither of them can be a cure for IPF [Citation6]. As a kind of typical senile disease, the incidence of IPF increases with age [Citation7]. Attributed to population aging, the incidence of IPF has been on the rise in recent years, along with only 20% of the five-year survival rate [Citation8]. Therefore, it is urgent and essential to clarify the molecular mechanism of IPF pathogenesis to find effective intervention targets for developing relevant treatment strategies.
IPF features the excessive proliferation of lung fibroblasts and deposition of extracellular matrix (ECM) such as collagen and fibrin, which makes the alveolar structure disorder and aggravates the process of pulmonary fibrosis. Fibroblasts are the main cells secreting ECM, and its excessive proliferation is considered as the most direct cause of pulmonary fibrosis [Citation9]. Fibroblasts can migrate to the injured area and proliferate rapidly to form fibroblast foci that secretes a large amount of ECM and fibroblast foci is considered as a critical prognostic factor associated with survival of IPF patients [Citation10,Citation11].
Tissue-type plasminogen activator (tPA) is a member of the serine protease family, which plays a pivotal role in the homeostasis of blood coagulation/fibrinolysis and matrix regulation [Citation12]. Its main biological function is to hydrolyze plasminogen into fibrinolytic enzyme, thereby activating the fibrinolytic system and dissolving thrombus. Because of its high catalytic activity and good specificity, FDA has recommended tPA as the preferred therapeutic agent for thrombolysis. Recent reports have indicated that tPA is actually a dual functional molecule. In addition to playing an important role in the thrombolytic system as a protease, tPA can also participate in the signaling pathway as a cytokine [Citation13,Citation14]. As a cytokine, tPA can promote epithelial–mesenchymal transition (EMT), contribute to the activation and accumulation of interstitial fibroblasts, and improve the anti-apoptosis ability of interstitial fibroblasts [Citation15]. In our study, we found that tPA promotes lung fibroblast proliferation by triggering sequential proliferative signaling events involving Erk1/2, p90RSK, GSK-3β phosphorylation, and cyclinD1 induction.
Wnt/β-catenin signaling is an evolutionarily conserved signaling pathway that has been suggested to be crucial in regulating embryonic development and tissue homeostasis [Citation16–Citation18]. At the cellular level, this signaling controls the generation of cell polarity, self-renewal of stem cells, and cell proliferation and motility. At present, multiple studies have demonstrated that Wnt/β-catenin signaling pathway plays a pivotal role in the pathogenesis of IPF, which is activated in lung interstitial stem cells, alveolar epithelial cells and fibroblasts, inducing lung interstitial stem cells and alveolar epithelial cells into fibroblasts and promoting fibroblasts proliferation [Citation19–Citation21]. Wnt/β-catenin signaling was mainly activated by Wnt proteins, resulting in phosphorylation of GSK-3β, which inhibits its’ activity. Inhibition of GSK-3β activity unable to make downstream β-catenin ubiquitinated and degraded, leading to accumulation of β-catenin in the cytoplasm. Consequently, β-catenin translocates into the nucleus and binds to T-cell factor (TCF)/lymphocyte enhance factor (LEF) to regulate the transcription of target genes and activate the Wnt/β-catenin signaling pathway [Citation22,Citation23]. The hallmark of the activation of Wnt/β-catenin signaling is β-catenin accumulation in the cytoplasm and its translocation into the nucleus [Citation24]. We noticed that whether the GSK-3β is active or not is a key point for the level of β-catenin. Intriguingly, we found that tPA phosphorylates GSK-3β in the proliferative signaling events and asked if it is possible that tPA is involved in Wnt/β-catenin signaling pathways and affects the pulmonary fibrosis. The present study sought to investigate the role of tPA in lung fibroblasts and Wnt/β-catenin signaling pathways.
Materials and methods
Ethics statement
The animal experiments were performed according to the Guide for the Care and Use of Laboratory Animals (The Ministry of Science and Technology of China, 2006) and all experimental protocols were approved under the animal protocol number SYXK (Su) 2009–0017 by the Animal Care and Use Committee of Nanjing University.
Bleomycin-induced mouse pulmonary fibrosis model
C57BL/6 mice weighing approximately 20 to 22 g were maintained under standard conditions with free access to water and laboratory rodent food. After anesthesia with pentobarbital sodium (3 mg/kg), mice received a single, slow intratracheal injection of 5 mg/kg bleomycin (Nippon Kayaku Co, Tokyo, Japan) dissolved in 40 μl of saline with MicroSprayer (Penn-Century, Wyndmoor, PA). Sham control mice received 40 μl of saline instead. Mice treated with bleomycin were sacrificed on day 7, day 14 and day 21 ()). And mice of the control group were sacrificed on day 7, day 14 and day 21. In the preventive study ()), PBS or PD98059 was administered into mice by daily intraperitoneal injections at the dosage of 20 μg/kg body weight. A week later, mice received bleomycin or saline intratracheally. Mice were sacrificed 7 days and 14 days after bleomycin instillation.
Figure 1. The expression of tPA is upregulated in lung tissues from bleomycin-induced mice. (a) The lung sections from the mice after different periods of bleomycin treatment were stained by hematoxylin-eosin (H&E) and Masson. (b) The expression of α-smooth muscle actin (α-SMA), collagen and β-catenin after different periods of bleomycin treatment was determined by western blot and represented graphically viz-a-viz the control. (c) The protein level of tPA after different periods of bleomycin treatment was determined by western blot. (d) The expression of tPA in lung tissues was examined by immunofluorescence assay. Nuclei were stained with DAPI (blue). (e) The expression of tPA in lung tissues was examined by immunohistochemical staining
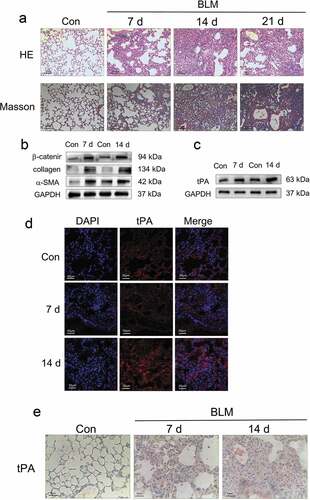
Histopathology
The lungs of mice was fixed in 4% neutral phosphate-buffered paraformaldehyde overnight, dehydrated, transparentized and embedded in paraffin before sectioning into 5 μm-thick slices. The sections were stained with hematoxylin-eosin (H&E) for structured observation, or with Masson trichrome stain for detection of collagen deposits, or were subjected to immunohistochemistry/immunofluorescence analyses.
Immunohistochemistry
Five micrometer-thick slides were deparaffinized with xylene before rehydration using an ethanol gradient. Next, quenching of endogenous peroxidase activity was achieved by incubation with 3% H2O2. After blocking with 3% BSA, the sections were incubated with rabbit anti-tPA overnight at 4°C. The secondary antibodies incubated were horseradish peroxidase-conjugated goat anti-rabbit IgG (Boster). The DAB Substrate System (DAKO) was used to reveal the immunohistochemical staining.
Immunofluorescence staining
The immunofluorescence analysis of lung tissues were performed as described previously [Citation25]. The primary antibody was rabbit anti-tPA (Proteintech). Alexa Fluor 488-conjugated goat anti-rabbit antibody was used as the secondary antibody. Nuclei were stained with DAPI (Sigma). The images were captured using a confocal fluorescence microscope (Olympus, Tokyo, Japan).
Isolation of mouse lung fibroblasts
C57BL/6 mice aged 4–6 weeks were purchased from the Medical School of Yangzhou University (Yangzhou, China). Mouse lung fibroblasts were isolated and cultured as previously reported by us and others [Citation26]. In brief, mouse lung parenchyma tissues were digested by collagenase and dispersing enzyme after cutting with scissors. After digestion, 1000r/min centrifugation was performed for 5 min, supernatant was discarded, tissue blocks were cultured with DMEM containing 10% fetal bovine serum, 4% L-glutamine, 1% nonessential amino acids, and 1% penicillin and streptomycin, and maintained in a humidified atmosphere of 95% air, 5% CO2 at 37°C. The mouse lung fibroblasts were passaged 1:2 using 0.25% trypsin when they reached 70–90% confluence.
CCK-8 assay
Cell Counting Kit-8 (CCK-8, Dojindo Laboratories, Kumamoto, Japan), which measures cell viability, is based on the conversion of an orange-colored product from water-soluble tetrazolium salt (WST-8) by dehydrogenases in live cells. Mouse lung fibroblasts were treated with various concentrations of tPA (0, 5 nM, 10 nM, 20 nM, and 50 nM) in 96-well plates for 48 h followed by the CCK-8 assay according to the instructions from the manufacturer.
Western blot
Proteins were purified from either cells or lung tissues. Western blot analysis was performed as previously described. Proteins were separated using 10% SDS-PAGE and electrophoretically transferred to polyvinylidene fluoride (PVDF) membranes. The primary antibodies employed were: rabbit anti-glycogen synthase kinase-3β (GSK-3β), rabbit anti-p-GSK-3β (Ser9), rabbit anti-β-catenin, rabbit anti-tPA, rabbit anti-Erk1/2, rabbit anti-p-Erk1/2, rabbit anti-p90RSK, rabbit anti-p-p90RSK (Ser380), rabbit anti-Cyclin D1, mouse anti-GAPDH, rabbit anti-collagen I, and mouse anti-α-SMA. Species-matched horseradish peroxidase-conjugated IgG (Boster, Wuhan, China) was used as the secondary antibody. The chromogenic signal intensity was detected using an Odyssey Scanning System (LI-COR, Lincoln, NE) and quantified using image J software (NIH, Bethesda, MD).
Statistical analysis
The data are presented as mean values ± SD. Differences were analyzed for significance (P ≤ 0.05) by one-way analysis of variance using SPASS for windows version 11.0 (SPASS, Chicago, IL).
Results
The Expression of tPA is Upregulated in Lung Tissues from Bleomycin-induced Mice.
Following intratracheal spray of bleomycin, the alveolar structure of mice was destroyed and collagen deposition gradually increased in lung tissues from day 7 to day 21 as assessed by H&E and Masson’s trichrome staining ()). On day 7, it was characterized by intrapulmonary structural disturbance and a slight thickening of the alveolar septa. Collagen began to build up around the trachea and the thickened alveolar walls. On day 14, fibrosis was at its most severe. Collagen increased greatly and fibroblast foci diffused throughout the lung tissues. On day 21, lung structure disorder seemed to alleviate, possibly due to self-healing. In addition to changes in lung architecture, we also examined the expression of proteins associated with fibrosis. The western blot results showed that the expression of α-SMA and collagen was increased after different periods of bleomycin treatment ()). And the expression level of β-catenin was also increased, which suggests that Wnt/β-catenin signaling was activated in the pathogenesis of pulmonary fibrosis ()). To investigate the expression of tPA in lung tissues from bleomycin-induced mice, we used western blot, immunofluorescence, and immunohistochemistry to determine. The results showed that compared to the control group, the expression of tPA was increased after bleomycin treatment, especially on day 14 ().
(2) tPA Promotes Lung Interstitial Fibroblast Proliferation
While the etiology of IPF is still unclear and the development of IPF is affected by many factors, it is convinced that the excessive proliferation of lung fibroblasts is the direct cause to accelerate the development of IPF. To study the effect of tPA on interstitial fibroblast, mouse primary lung fibroblasts were incubated with different concentrations of tPA for 48 h and then cell viability was measured by CCK8. The results showed that tPA concentration at 20nM significantly promoted the proliferation of lung interstitial fibroblasts ()). We next investigated cell proliferation-related signaling events in interstitial fibroblasts after tPA treatment. Western blot results showed that tPA rapidly induced Erk1/2 phosphorylation as early as 10–15 min ()) followed by p90RSK phosphorylation about 30 min ()). Intriguingly, tPA also triggered phosphorylation of GSK-3β later ()). The time course of GSK-3β phosphorylation after the activation of p90RSK suggests that GSK-3β may be a downstream effector of p90RSK kinase in the tPA signaling pathway. Subsequently, tPA induced the sustained expression of Cyclin D1 between 24 h to 48 h ()). Thus, it is possible that tPA activates proliferative signaling involving phosphorylation of Erk1/2, p90RSK, GSK3β, and induction of cyclin D1 in a temporally coordinated manner.
(3) The Effect of tPA on Wnt/β-catenin Signaling Pathway.
Figure 2. tPA promotes lung interstitial fibroblast proliferation via sequential phosphorylation of Erk1/2, p90RSK, GSK-3β, and induction of Cyclin D1. (a) The change of cell proliferation was observed with the cell counting kit (CCK-8) assay. *P ≤ 0.05 versus control. (b-c) Cells were incubated with tPA (20 nM) for various periods of time as indicated and then subjected to western blot for phospho-Erk1/2 and phospho-p90RSK (Ser9). (d-e) Cells were treated with tPA (20 nM) for different periods of time and the protein levels of phospho-GSK-3β (Ser380) and cyclin D1were assessed via western blot
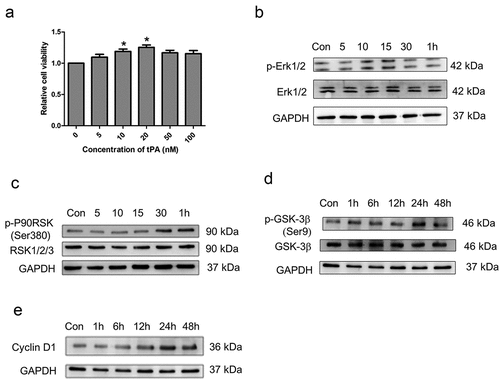
As is well-known that Wnt/β-catenin signaling plays an important role in the development of pulmonary fibrosis. And GSK-3β is a key signal molecule whose phosphorylation level directly affects the downstream β-catenin. Accumulated β-catenin will translocate into the nucleus, where it binds to T-cell factor (TCF)/lymphocyte enhance factor (LEF) and converts them from transcriptional repressors to activators. In our study, we found that the expression of p-GSK-3β and β-catenin were increased after tPA treatment ()). It suggested that tPA may indirectly activate the Wnt/β-catenin signaling by phosphorylating GSK-3β. To further investigated whether tPA can activate Wnt/β-catenin signaling pathway through regulating the phosphorylation level of GSK-3β, we used tPA inhibitor (PAI) and tPA signaling inhibitor PD98059 (inhibition of the Erk1/2 site) respectively, to explore the effect of tPA on Wnt/β-catenin signaling pathway. The results showed that the levels of p-GSK-3β and β-catenin were decreased after inhibitor treatment ()), which indicates that PAI and PD98059 could both reduce the activation effect of tPA on the Wnt/β-catenin signaling pathway.
(4) Effects of PD98059 on Wnt/β-catenin Signaling Pathway in Animals.
Figure 3. The effect of tPA on Wnt/β-catenin signaling pathway. (a) Primary lung fibroblasts were treated with 20 nM tPA for different periods of time and the expression levels of phospho-GSK-3β (Ser9) and β-catenin were assessed by western blot. (b) Primary lung fibroblasts were pretreated with tPA inhibitor PAI (5 nM) for 1 h and subsequently exposed to tPA (20 nM) for 48 h, then subjected to western blot for p-GSK-3β (Ser9) and β-catenin. (c) Primary lung fibroblasts were pretreated with inhibitor PD98059 at 20 μg/ml for 1 h and subsequently exposed to tPA (20 nM) for 48 h; expressions of p-GSK-3β (Ser9) and β-catenin were assessed by western blot
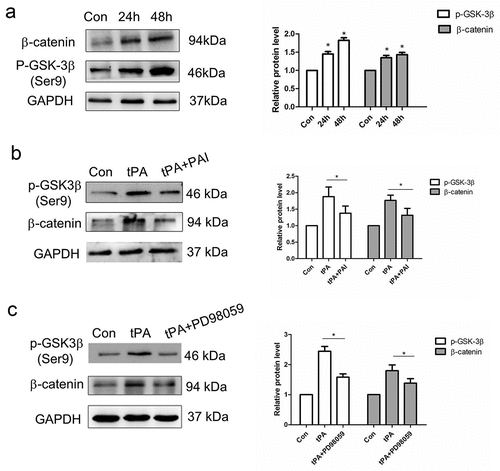
In the previous experiments, we have demonstrated tPA promotes lung fibroblasts proliferation in vitro. Next, we detected the expression of tPA signaling pathway-related proteins in lung tissues from IPF model mouse by western blot. The results showed that compared to the control group, the expression of p-Erk1/2, p-p90RSK, p-GSK-3β, and Cyclin D1 were increased ()), which indicated that the tPA signaling pathway was activated in lung tissues. In addition, we have demonstrated that tPA indirectly activated the Wnt/β-catenin signaling pathway through tPA proliferative signaling, while inhibitor PD98059 dampened the activation effect in vitro. In order to confirm whether PD98059 can regulate the Wnt/β-catenin signaling pathway in animals, we established IPF mouse model with bleomycin as the control group and the repair group were injected with inhibitor PD98059. After 14 days of intraperitoneal injection, the results showed that the expression levels of p-GSK-3β and β-catenin in lung tissues from the repair group were decreased compared to the control group ()), which suggests that PD98059 can inhibit the Wnt/β-catenin signaling pathway in animals. Subsequently, we want to explore whether PD98059 could intervene in the development of pulmonary fibrosis in animals. After 14 days of intraperitoneal injection of PD98059, H&E staining results showed that compared to the control group, PD98059 could reduce the area of fibrous proliferation in lung tissue and the alveolar structure tended to be normal ()). Besides, the results of Masson staining showed that PD98059 could significantly reduce collagen deposition and slowdown the progression of pulmonary fibrosis ()). Together, it was convincing that PD98059 inhibited the development of pulmonary fibrosis.
Figure 4. Effects of PD98059 on Wnt/β-catenin signaling pathway in animals. (a) Protein levels of p-Erk、p-p90RSK (Ser380)、p-GSK-3β (Ser9) and CyclinD1 in lung tissues from bleomycin-induced mice were assessed via western blot. (b) Bleomycin-induced mice were treated intraperitoneally PD98059 (20 μg/kg) for 14 days then subjected to western blot for the expression levels of p-GSK-3β (Ser9) and β-catenin. (c) Representative pictures of lung sections from the control group and PD98059-treated group with H&E and Masson’s trichrome staining
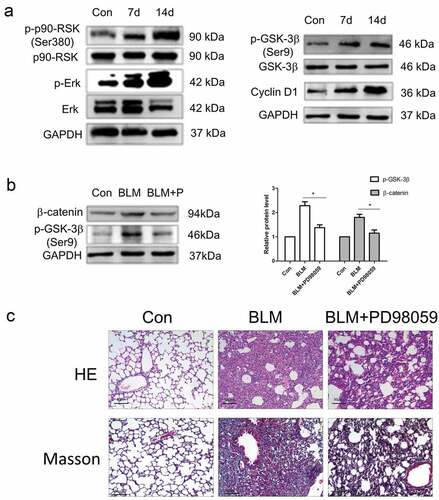
Discussion
IPF is a fatal lung disease characterized by the progressive and irreversible destruction of lung architecture [Citation27]. Though the etiology of IPF is still unclear, the outcome is the excessive deposition of ECM such as collagen and fibrin and the destruction of alveolar structure. As the major matrix-producing cells, the size of the interstitial fibroblast population is a critical factor in the development of pulmonary fibrosis. In addition, fibroblasts can migrate to the injured area and be functionally activated [Citation28,Citation29]. It has been reported that injured alveolar epithelial cells can produce PDGF, IL-1, FGFs and TGF-β, which contribute to the proliferation and activation of lung fibroblasts and aggravate pulmonary fibrosis [Citation30,Citation31]. In this study, we demonstrated that tPA activates a cascade of proliferative signaling events and promotes interstitial fibroblast expansion. This finding establishes a previously unrecognized function of tPA in controlling the fate of interstitial fibroblasts.
tPA was originally discovered as a member of the serine protease family, whose main function is to activate zymogens into active enzymes involved in fibrinolysis [Citation12,Citation32]. Recently, studies have found that tPA also elicits many biological activities through protease-independent mechanism [Citation14,Citation33–Citation36]. It is reported that tPA promotes fibroblast activation through TGF-β1–mediated fibronectin and collagen I expression in the progress of kidney fibrosis [Citation37]. tPA elicits its fibrogenic action by a novel signal transduction pathway in which it binds to the LDL receptor-related protein 1 (LRP-1) and triggers the receptor tyrosine phosphorylation that in turn recruits β1 integrin and subsequently activates ILK signaling. In addition, tPA also protected TGF-β1–activated myofibroblasts from apoptosis [Citation33]. This antiapoptotic effect of tPA was independent of its protease activity but as a cytokine.
We established the pulmonary fibrosis mouse model by tracheal administration of bleomycin and we found that the expression of tPA in the lung tissues was increased ()). It is reported that tPA is a hybrid molecule which has the function of protease and cytokine. However, the specific role of tPA in pulmonary fibrosis is unclear. Intriguingly, in our study, we found that tPA promoted the primary lung fibroblasts proliferation ()). tPA activates a cascade of proliferative signaling events involving the phosphorylation of Erk1/2, p90RSK, GSK-3β, and induction of Cyclin D1. It seems that tPA plays an important role in controlling the fate of interstitial lung fibroblasts, indicating that tPA acts as a cytokine in modulating the phenotypic change of interstitial fibroblasts from quiescence to higher mitogenic potential.
It’s well known that Wnt/β-catenin signaling pathway plays an important role in the development of IPF [Citation38]. Multiple studies have a focus on Wnt/β-catenin signaling pathway in pulmonary fibrosis and researchers blocked different sites of Wnt/β-catenin signaling pathway. When inhibiting the binding of β-catenin with TCF/LEF, it leads to a large number of cell death. After blocking Dsh and Axin site, the expression of β-catenin was still higher than normal in tissues, which indicated that the Wnt/β-catenin signaling pathway was still partially activated [Citation39,Citation40]. Therefore, we speculated that maybe there are other factors can activate the Wnt/β-catenin signaling pathway. In the present study, we demonstrated that Wnt/β-catenin signaling pathway was activated in fibrotic lung tissues, the key proteins p-GSK-3β and β-catenin are both upregulated ()). We noticed that tPA can also trigger GSK-3β phosphorylation in proliferative signaling ()). Thus, we wondered if it’s possible that tPA involved in the Wnt/β-catenin signaling pathway by regulating the phosphorylation of GSK-3β. After adding tPA to the primary lung fibroblasts, the phosphorylation level of GSK-3β is elevated. And we found that the expression of β-catenin was increased ()), which suggests that tPA can activate Wnt/β-catenin signaling pathway. We used tPA inhibitor PAI and tPA signaling inhibitor PD98059 (inhibition of the Erk1/2 site) to further confirm that tPA has an impact on Wnt/β-catenin signaling through regulating the phosphorylation level of GSK-3β. The results showed that both inhibitors reduced GSK-3β phosphorylation and the expression of β-catenin in cells ()).
We have demonstrated that tPA can activate Wnt/β-catenin signaling pathway and PD98059 can inhibit this activation in vitro. What’s more, we need to know whether the tPA proliferative signaling is activated in animals. The results of western blot showed that it is activated in fibrotic lung tissues ()) and inhibitor PD98059 reduced the expression of β-catenin in animals ()). And the H&E staining and Masson staining results showed that PD98059 effectively repressed the development of pulmonary fibrosis on the 14th day ()). These findings provide some experimental basis for the study on how to effectively control the development of IPF and provide some new insight into clinical treatment.
Conclusion
In summary, our present study showed that the expression of tPA was increased significantly in lung tissues from bleomycin-induced IPF model mouse, suggesting that tPA may play a critical role in the development of pulmonary fibrosis. And we found that tPA promotes the proliferation of lung fibroblasts by activating a cascade of proliferative signaling events involving the phosphorylation of Erk1/2, p90RSK, GSK-3β, and induction of Cyclin D1. In addition, tPA activates the Wnt/β-catenin signaling pathway by regulating the activity of GSK-3β. What’s more, tPA signaling pathway is activated in fibrotic lung tissues. tPA signaling pathway inhibitor PD98059 can reduce the phosphorylation level of GSK-3β and inhibit the Wnt/β-catenin signaling pathway in animals, which slows down the development of pulmonary fibrosis ().
Disclosure statement
No potential conflict of interest was reported by the authors.
Additional information
Funding
References
- Selman M, King TE, Pardo A. Idiopathic pulmonary fibrosis: prevailing and evolving hypotheses about its pathogenesis and implications for therapy. Ann Intern Med. 2001;134:136–151.
- Meyer KC. Pulmonary fibrosis, part I: epidemiology, pathogenesis, and diagnosis. J Expert Rev Respir Med. 2017;11:343–359.
- Swigris JJ, Kuschner WG, Kelsey JL, et al. Idiopathic pulmonary fibrosis: challenges and opportunities for the clinician and investigator. Chest. 2005;127:275–283.
- Ley B, Collard HR, King TE Jr. Clinical course and prediction of survival in idiopathic pulmonary fibrosis. Am J Respir Crit Care Med. 2011;183:431–440.
- Sgalla G, Biffi A, Richeldi L. Idiopathic pulmonary fibrosis: diagnosis, epidemiology and natural history. Respirology. 2016;21:427–437.
- Richeldi L, Du Bois RM, Raghu G, et al. Efficacy and safety of nintedanib in idiopathic pulmonary fibrosis. N Engl J Med. 2014;370:2071–2082.
- Kishaba T, Nagano H, Nei Y, et al. Clinical characteristics of idiopathic pulmonary fibrosis patients according to their smoking status. J Thorac Dis. 2016;8:1112.
- Thabut G, Christie JD, Ravaud P, et al. Survival after bilateral versus single-lung transplantation for idiopathic pulmonary fibrosis. Ann Intern Med. 2009;151:767–774.
- Phan SH. Biology of fibroblasts and myofibroblasts. Proc Am Thorac Soc. 2008;5:334–337.
- KING JR TE, Schwarz MI, Brown K, et al. Idiopathic pulmonary fibrosis: relationship between histopathologic features and mortality. Am J Respir Crit Care Med. 2001;164:1025–1032.
- Nicholson AG, Fulford LG, Colby TV, et al. The relationship between individual histologic features and disease progression in idiopathic pulmonary fibrosis. Am J Respir Crit Care Med. 2002;166:173–177.
- Castellino FJ, Ploplis VA. Structure and function of the plasminogen/plasmin system. J Thromb Haemost. 2005;93:647–654.
- Yang J, Shultz RW, Mars WM, et al. Disruption of tissue-type plasminogen activator gene in mice reduces renal interstitial fibrosis in obstructive nephropathy. J Clin Invest. 2002;110:1525–1538.
- Hu K, Yang J, Tanaka S, et al. Tissue-type plasminogen activator acts as a cytokine that triggers intracellular signal transduction and induces matrix metalloproteinase-9 gene expression. J Biol Chem. 2006;281:2120–2127.
- Liu Y. Renal fibrosis: new insights into the pathogenesis and therapeutics. J Kidney Int. 2006;69:213–217.
- Clevers H. Wnt/β-catenin signaling in development and disease. J Cell. 2006;127:469–480.
- Clevers H, Nusse R. Wnt/β-catenin signaling and disease. J Cell. 2012;149:1192–1205.
- MacDonald BT, Tamai K, He X. Wnt/β-catenin signaling: components, mechanisms, and diseases. J Dev Cell. 2009;17:9–26.
- Pongracz JE, Stockley RA. Wnt signalling in lung development and diseases. Respir Res. 2006;7:15.
- Guo Y, Xiao L, Sun L, et al. Wnt/β-catenin signaling: a promising new target for fibrosis diseases. J Physiol Res. 2012;61:337–346.
- Van Scoyk M, Randall J, Sergew A, et al. Wnt signaling pathway and lung disease. Transl Res. 2008;151:175–180.
- Henderson WR, Chi EY, Ye X, et al. Inhibition of Wnt/β-catenin/CREB binding protein (CBP) signaling reverses pulmonary fibrosis. J Proc National Acad Sci. 2010;107:14309–14314.
- Zhou B, Liu Y, Kahn M, et al. Interactions between β-catenin and transforming growth factor-β signaling pathways mediate epithelial-mesenchymal transition and are dependent on the transcriptional co-activator cAMP-response element-binding protein (CREB)-binding protein (CBP). J Biol Chem. 2012;287:7026–7038.
- Moon RT, Bowerman B, Boutros M, et al. The promise and perils of Wnt signaling through β-catenin. Science. 2002;296:1644–1646.
- Moheimani F, Roth HM, Cross J, et al. Disruption of β-catenin/CBP signaling inhibits human airway epithelial–mesenchymal transition and repair. Int J Biochem Cell Biol. 2015;68:59–69.
- Hegab AE, Kubo H, Fujino N, et al. Isolation and characterization of murine multipotent lung stem cells. J Stem Cells Dev. 2010;19:523–536.
- Gross TJ, Hunninghake GW. Idiopathic pulmonary fibrosis. J N Engl J Med. 2001;345:517–525.
- Willis BC, Liebler JM, Luby-Phelps K, et al. Induction of epithelial-mesenchymal transition in alveolar epithelial cells by transforming growth factor-β1: potential role in idiopathic pulmonary fibrosis. Am J Pathol. 2005;166:1321–1332.
- Gabbiani G. The biology of the myofibroblast. J Kidney Int. 1992;41:530–532.
- Agostini C, Gurrieri C. Chemokine/cytokine cocktail in idiopathic pulmonary fibrosis. J Proc Am Thoracic Soc. 2006;3:357–363.
- Sime PJ, Xing Z, Graham FL, et al. Adenovector-mediated gene transfer of active transforming growth factor-beta1 induces prolonged severe fibrosis in rat lung. J Clin Invest. 1997;100:768–776.
- Andreasen PA, Egelund R, Petersen HH. The plasminogen activation system in tumor growth, invasion, and metastasis. J Cell Mol Life Sci Cmls. 2000;57:25–40.
- Hu K, Lin L, Tan X, et al. tPA protects renal interstitial fibroblasts and myofibroblasts from apoptosis. J Am Soc Nephrol. 2008;19:503.
- Hu K, Mars WM, Liu Y, et al. Novel actions of tissue-type plasminogen activator in chronic kidney disease. Front Biosci. 2008;13:5174–5186.
- Hu K, Mars WM, Liu Y. Novel actions of tissue-type plasminogen activator in chronic kidney disease: a paradigm shift. J Front Biosci. 2008;13:5174–5186.
- Shi Y, Mantuano E, Inoue G, et al. Ligand binding to LRP1 transactivates Trk receptors by a Src family kinase–dependent pathway. Sci Signal. 2009;2:ra18–ra18.
- Hu K, Wu C, Mars WM, et al. Tissue-type plasminogen activator promotes murine myofibroblast activation through LDL receptor–related protein 1–mediated integrin signaling. J Clin Invest. 2007;117:3821–3832.
- Chilosi M, Poletti V, Zamò A, et al. Aberrant Wnt/β-catenin pathway activation in idiopathic pulmonary fibrosis. J Am J Pathol. 2003;162:1495–1502.
- Chen X, Shi C, Meng X, et al. Inhibition of Wnt/β-catenin signaling suppresses bleomycin-induced pulmonary fibrosis by attenuating the expression of TGF-β1 and FGF-2. J Exp Mol Pathol. 2016;101:22–30.
- Wang C, Dai J, Sun Z, et al. Targeted inhibition of disheveled PDZ domain via NSC668036 depresses fibrotic process. Exp Cell Res. 2015;331:115–122.