ABSTRACT
Despite recent progress in research on brain tumors, including identification of cancer stem-like cells (CSCs), little is known about the interplay of stemness with the commonly observed infection by the human cytomegalovirus (HCMV) and the widespread features of replication stress in these malignancies. To shed more light on these outstanding issues, here we combine immunohistochemical analysis of archival clinical specimens from a cohort of 25 human pediatric medulloblastomas, complemented by functional experiments and analytical approaches to examine three medulloblastoma cell lines. In the clinical samples, we find consistent, yet individually variable subsets of CSCs expressing the stem-cell markers CD133 and CD15, and a candidate marker VEGFR2, across the spectrum of endogenous DNA damage (γH2AX), expression of HCMV immediate early and late proteins, proliferation rate (Ki67) or molecular class of MB. Contrary to MB cell lines DAOY and D324, the D283 cells showed pronounced phenotypic features of stemness, associated with enhanced endogenous DNA damage, exceptionally high susceptibility to infection with HCMV, unorthodox signaling pathway response to ionizing radiation and hyperactive response to hydroxyurea-induced replication stress. Notably, single-molecule DNA fiber analysis revealed aberrantly slow replication fork progression, pronounced fork asymmetry and inability to timely recover from drug-induced fork stalling in stem-like D283 cells, all hallmarks of pronounced chronic replication stress and propensity to genomic instability. These findings provide insights into human medulloblastoma stemness phenotypes, with various susceptibilities to infection by HCMV and impact on replication fork (mal)function, with implications for better understanding pathogenesis and responses to treatment in pediatric brain malignancies.
Abbreviations: CSC: cancer stem-like cells; FBS: fetal bovine serum; HCMV: human cytomegalovirus; MB: medulloblastoma; MBSC: medulloblastoma stem cells; MOI: multiplicity of infection; PBS: phosphate-buffered saline; RPA: replication protein A; RS: replication stress; SHH: sonic hedgehog; VEGFR2: vascular endothelia growth factor receptor 2.
1. Introduction
Tumor relapse and resistance to standard-of-care treatment modalities are among the most prominent challenges faced by contemporary cancer research and clinical oncology. One aspect of tumor cell biology that is intimately linked with both these challenges is the existence, across a wide spectrum of tumor types, of cancer stem-like cells (CSC), a subset of cells within the heterogeneous and hierarchically organized tumor cell populations that, unlike the bulk of the tumor cells, are: i) commonly more resistant to treatments, ii) able to self-renew, and iii) give rise to the different tumor cell subpopulations that together form the tumor mass, thereby iv) fueling tumorigenicity and formation of recurrent lesions [Citation1].
Another key aspect that contributes to both inter- and intra-tumoral heterogeneity is replication stress (RS), a phenomenon caused endogenously, by oncogene-driven aberrant DNA replication and deregulated cell cycle control, and/or by exogenous insults such as diverse chemotherapeutic drugs that impact DNA replication or certain cancer-promoting microbial pathogens [Citation2]. Among the latter candidates for inducers of RS is the human cytomegalovirus (Bartek, 2017 #124), an emerging tumor-modulatory pathogen present in multiple types of human malignancies including various types of carcinomas and their metastases [Citation3–Citation6], glioblastomas [Citation7,Citation8] and medulloblastomas [Citation9,Citation10]. As a common theme shared by a wide spectrum of tumors and causes of RS, aberrant DNA replication leads to formation of abnormal DNA or RNA/DNA structures, single-stranded and double-stranded DNA breaks, chromosome instability and aneuploidy [Citation11], but also more subtle mutations due to the RS-induced APOBEC3B enzyme [Citation12] or error-prone DNA repair mechanisms [Citation13–Citation15]. As a shared reaction to RS and RS-associated DNA damage, cells activate checkpoint signaling pathways and DNA repair mechanisms to slow down or halt cell cycle progression and repair the potentially hazardous DNA lesions, overall alarming the cellular DNA damage response as an intrinsic anti-cancer barrier [Citation16–Citation20]. At the same time, the chronic RS and activated cell cycle checkpoints or even apoptotic pathways create an environment that provides selective pressure for survival and outgrowth of tumor cells that bypass or inactivate, by mutagenesis, epigenetic silencing or chromosome losses, the key DDR checkpoint responses such as the ATM-Chk2-p53 tumor suppressor axis, thereby allowing aberrant proliferation of the nascent tumor clones at the expense of genomic instability [Citation21], at the same time enhancing tumor diversification and subclonal evolution [Citation2,Citation22,Citation23].
While the above-mentioned concepts appear to be broadly applicable for human tumors of diverse tissue origin, in this study we focus on medulloblastomas, for which some important aspects of pathogenesis and treatment response remain to be elucidated. Medulloblastomas (MB) are the most frequent primary malignant brain tumors in children, with only sporadic cases seen in the adult population. Based on characteristic molecular aberrations and clinical features, medulloblastomas have recently been subdivided into 4 major classes, related to key tumorigenic aberrations in the Sonic Hedgehog (Shh) and Wnt developmental pathways, and group 3 and 4 tumors carrying oncogenes such as Myc [Citation24]. Surgery in combination with chemo- and radiotherapy is considered standard‐of‐care treatment. Although the prognosis of these patients has improved during the last decades, there are still many not responding well to the current therapy, especially among patients with group 3 and 4 MB or SHH tumors with germ-line or somatic TP53 mutations [Citation25–Citation27]. Studies addressing the variable therapeutic responses indicate that MB express a wide variety of genetic and epigenetic alterations, demonstrating a high level of heterogeneity and genetic instability [Citation28,Citation29] which could account, at least in part, for the observed phenotypic plasticity and therapy resistance. In this context, we have previously demonstrated signs of endogenous replication stress and spontaneous activation of DNA damage checkpoints [Citation9] in MB, adding to the observed genetic variability and hence likely contributing to both tumor evolution and therapy resistance. Furthermore, we and others have reported evidence for the presence of HCMV in MB, a virus implicated in oncomodulation, genetic instability as well as therapy resistance [Citation9,Citation10,Citation30,Citation31]. The fact that some laboratories fail to detect HCMV in MB specimens [Citation32–Citation34] most likely reflects technical reasons, such as inappropriate pH conditions in the buffers and insufficient sensitivity of methods for HCMV protein detection by immunohistochemistry, as clarified by our recent study comparing three detection methods used in the literature [Citation9]. Again, broadly analogous to other tumor types, MB contain subsets of CSCs, also called tumor initiating cells, believed to be responsible for medulloblastoma tumor maintenance, initiation, relapse and dissemination. In MB, these CSCs (hereafter referred to as MBSC) have been identified by using the cell surface markers CD133 and/or CD15, with several studies demonstrating therapy resistance of MBSC, mediated by pro-survival/anti-apoptotic signaling, quiescence and/or interaction with the hypoxic tumor microenvironment [Citation35].
Taken together, whereas the three factors, namely i) the presence of MBCS, ii) features of replication stress, and iii) the evidence of HCMV infection, are all documented in the recent literature, there has been no attempt so far to assess and elucidate any potential links among these three factors in MB. To address this urgent unmet need in the field, the aim of our present study was to provide insights into potential presence and significance of endogenous replication stress and the ensuing cellular responses to it, compared to the bulk of MB cells without the stem-cell features, as well as the fate of HCMV infection in MBSC. The results of our analyses, using human clinical specimens from a cohort of human MBs [Citation9] complemented by MB cell culture models, experimental HCMV infections and ensuing immunochemical and functional studies, are presented in the following sections of this report. By elucidating these functional aspects of MB pathobiology in relation to stemness, we hope to advance our understanding of MB pathogenesis including links to HCMV and/or replication stress, with implications for the guidance of MB treatment in the future.
2. Material and methods
2.1. Patient population
In this study, we analyzed clinical specimens from a cohort of pediatric medulloblastoma patients treated at the Neurosurgical and Pediatric Oncology department of the Copenhagen University Hospital (Rigshospitalet). Between 1998 and 2009, 25 consecutive pediatric patients (ages < 16 years) were treated for a newly diagnosed medulloblastoma, 24 of whom were patients undergoing first-time surgical resections, while one (patient no. 16) was treated with secondary surgery due to tumor recurrence, with the first surgery performed 1 year previously. Details of the individual patients and clinicopathological parameters of the tumors were reported in our previous publication on this cohort [Citation9].
2.2. Immunohistochemistry
We employed our established sensitive immunohistochemical staining protocol [Citation18] to examine the expression of three stem-cell-like cell surface markers, CD133, CD15 and VEGFR2. This protocol, optimized in our laboratory in Copenhagen, involves standard deparaffinization of the archival formalin-fixed, paraffin-embedded tissue sections, antigen unmasking in the citrate buffer (pH6) using microwave (15 min), followed by overnight incubation with the primary antibody against the selected protein, and the ensuing processing by the indirect streptavidin-biotin-peroxidase method using the Vectastain Elite kit (Vector Laboratories, Burlingame, CA, USA) and nickel-sulfate-based chromogen enhancement detection as previously described, without nuclear counterstaining [Citation18]. The primary antibodies used for this immunohistochemical analysis included the following reagents: mouse monoclonal antibody against CD133 (AC133 pure, Miltenyi Biotec, diluted 1:50), mouse monoclonal antibody to CD15 (NCL-CD15, Leica, diluted 1:100), rabbit polyclonal antibody against VEGFR2 (Cell Signaling, diluted 1:250), and rabbit polyclonal antibody to phosphorylated RPA32 (Ser 4/8, NBP1-23017, Novus Biologicals, 1:2000). Detection methods and immunohistochemistry results for this patient cohort of the other markers, including HCMV immediate early and late proteins, the proliferative marker Ki67, and γH2AX were described in our previous report [Citation9], as were the protocols and results to subclassify the individual medulloblastomas into the Wnt, Shh and group 3/4 classes, respectively [Citation9], and these results are listed in just to facilitate comparisons with the new results obtained for the 3 stem-cell markers evaluated in this study. As negative controls, sections were incubated with nonimmune mouse or rabbit sera, while sections from human glioblastomas previously shown to express the studied proteins served as positive controls. The stem-cell marker results were evaluated by two experienced researchers including a senior onco-pathologist and the data expressed as percentage of tumor cells positive for the given marker within each lesion, based on examination of at least 500 tumor cells per tumor (see Results and for details, including subcellular localization of the markers).
Table 1. Immunohistochemical data for marker expression in human MB*
2.3. Cell culture and HCMV infection
Human medulloblastoma cell lines D283, DAOY and D324, human strain BJ of normal diploid primary fibroblasts and glioma cell line T98G (all from the ATCC) were grown in the RPMI 1640 medium with 10% fetal bovine serum and antibiotics. In some experiments, the cells were infected with the human cytomegalovirus and the expression of HCMV immediate early protein IE72 was examined in a time-course experiment by immunofluorescence (see a separate method section below) of cells cultured and fixed on coverslips. Infection with the VR1814 or AD169 HCMV strain (MOI 3) was performed at 37°C 5% CO2 in RPMI 1640 with 2% FBS for 1 h, after which cells were washed twice with low-FBS RPMI and grown in low-FBS RPMI for different time periods post-infection, before further analysis. All cell types were regularly tested to exclude mycoplasma infection. Growth of the infected cell lines and HCMV particle production by infected T98G and D283 cells are described in the legend to Supplementary Figure S1.
2.4. Gel electrophoresis and immunoblotting
Preparation of cell lysates from cultured cells, either untreated or exposed to ionizing radiation or hydroxyurea treatment was performed as described in the Results and figure legends, and the ensuing standard polyacrylamide gel electrophoresis under denaturing conditions followed by immunoblotting were performed as described previously [Citation36]. For immunoblotting, we used the following antibodies: mouse monoclonal antibody to human phospho-histone H2A.X (Ser 139) (Millipore, clone JBW 301, diluted 1:2000), rabbit polyclonal antibody to human phosphorylated Chk2 (Thr 68) (Cell Signaling, 1:500), rabbit polyclonal antibody against phosphorylated CHK1 (Ser317, Cell Signaling, 1:500), mouse monoclonal antibody to Chk2 (clone DCS 270, our own antibody, 1:1000, Rwf: [Citation37]) mouse monoclonal antibody to p53 (clone DO1, our own antibody, 1:1000, ref.: [Citation38]) rabbit polyclonal antibody to phosphorylated Kap 1 (Ser 824, ab70369, Abcam, 1:2000), mouse monoclonal antibody to total RPA32 (ab2175, Abcam, 1:500), rabbit polyclonal antibody to phosphorylated RPA32 (Ser33, NB100-544, Novus Biologicals, 1:1000) and rabbit polyclonal antibody to phosphorylated RPA32 (Ser 4/8, NBP1-23017, Novus Biologicals, 1:1000).
2.5. Flow cytometry analysis
Cells were fixed with 70% −20°C ethanol and stored at −20°C for at least 30 min. Cells were then washed with phosphate-buffered saline (PBS), labeled with 10 µg/ml of propidium iodide (PI) (Invitrogen) containing 5 µg/ml of ribonuclease A (Life Technologies) for 5 min at RT and analyzed on FACSVerse (Becton Dickinson). Staining with the mouse monoclonal antibody to HCMV immediate early protein IE72/86 (Millipore-Chemicon, clone MAB 810R, recognizing both isoforms of IE proteins iE72 and IE86, et 1:500, 1 h, RT) and the goat anti-mouse AlexaFluor-488 (Invitrogen) secondary antibody (1 h, RT) was performed before PI labeling. Acquired data were analyzed using FlowJo software.
2.6. Immunofluorescence
Cells grown on coverslips were fixed with 4% cold formaldehyde (VWR Chemicals) (10 min, room temperature (RT)), permeabilized with 0.5% Triton X-100 (Merck) in Phosphate-buffered Saline (PBS) (Gibco) (10 min, RT) and blocked in PBS containing 0.1% Tween® 20 and 1% Bovine Serum Albumin (BSA) (Sigma-Aldrich) (15 min, RT). Cells were then incubated with the IE72/IE86 (MAB810R, Millipore) antibody for 1 h at RT, washed with PBS and stained with the goat anti-mouse AlexaFluor-568 secondary antibody (Invitrogen) for 45 min at RT. Cell nuclei were visualized using Hoechst 33342 (Invitrogen) in PBS (5 min, RT). Finally, coverslips were washed with PBS and ddH2O, air-dried and mounted with the ProLong Gold Antifade Reagent (Life Technologies). Images were acquired using a LSM800 confocal microscope (Carl Zeiss), a 63 × /1.4 oil immersion objective (Carl Zeiss) and LSM ZEN software. Image analysis was performed using ImageJ software.
2.7. DNA fiber analysis
Cells were pulse-labeled with 25 μM of CldU (Sigma-Aldrich) for 20 min at 37°C, washed gently with the fresh pre-warmed RPMI 1640 and incubated in the fresh medium containing 250 μM of IdU (Sigma-Aldrich) for 20 min at 37°C. Cells were harvested and DNA fibers prepared as described previously [Citation39]. Slides were stored at 4°C for at least 24 h before being stained. CldU was detected first with a rat anti-BrdU (OBT0030, Serotec) and a DyLight 550 anti-rat (ThermoFisher Scientific) antibodies and IdU was detected with a mouse anti-BrdU (347580, Becton Dickinson) and the AlexaFluor-488 anti-mouse (Invitrogen) antibodies. Images of well-spread DNA fibers were acquired using the LSM800 confocal microscope, the 63 × /1.4 oil immersion objective and LSM ZEN software. Images were acquired semi-automatically by using software autofocus and tile-arrays. Analysis of double-labeled replication forks was performed manually using LSM ZEN software.
2.8. Ethical approval
The Regional Ethics Committee in Copenhagen, Denmark approved the study (Ref. H-6-2014-010).
3. Results
3.1. Expression of stem-cell markers and HCMV proteins in human medulloblastomas
To assess the fractions of cancer cells expressing the established biomarkers of stemness in human medulloblastomas, we took advantage of our well-characterized cohort of archival paraffin-embedded specimens from 25 pediatric MBs, previously reported by us to feature signs of chronic activation of DNA damage signaling and endogenous replication stress [Citation9]. Here, we examined all 25 tumors for the CD133 and CD15 markers [Citation35], as well as for VEGFR2, the latter inspired by its association with stem-like cells in glioblastomas [Citation40]. Representative examples of immunohistochemical patterns that we observed for the three MBSC markers on tissue sections from MBs, compared with control normal cerebellum, are shown in ), and the overall results presented as a summary for the entire cohort (). These results demonstrated that while each and every one of these 25 cases showed variable fractions of MB cells positive for each of the 3 markers (i.e. there was no case that would be completely negative for any of these 3 proteins), CD133 was the most restricted, with 23 cases showing only 0.5–5% positive cancer cells, and only three cases featuring wider expression, in the range of 7–20% MB cells (). The extent of CD15 expression was comparable with CD133 in 10 cases (the difference between the two markers being within the range of maximally 5% cells), yet in the remaining 15 cases, the CD15 marker was more abundantly expressed (in up to 40% of MB cells) than CD133 ().
Figure 1. Immunohistochemical analysis of human cerebellum and medulloblastomas. (a) Examples of immunoperoxidase staining patterns seen on archival paraffin sections from normal cerebellum and medulloblastomas, stained by antibodies to CD133, CD15 and VEGFR2 markers, respectively, as indicated. Scale bars: 30 μm; (b) Examples of immunoperoxidase staining patterns seen with antibodies against the general DNA damage response marker γH2AX, and the HCMV IE72 and HCMV late antigen, respectively. The bottom-row images show enlarged areas chosen from the lower-magnification images presented in the top row. Note the localization of the HCMV late antigen in endothelium of a blood vessel (with erythrocytes in the lumen). Scale bars: 20 ◽m
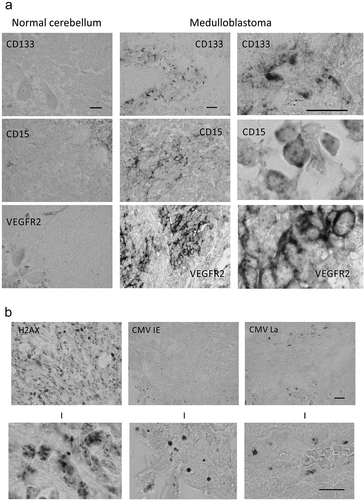
Given that we could detect membrane VEGFR2 in glioblastomas by immunohistochemistry [Citation40], that the other two markers CD133 and CD15 are plasma membrane proteins, and VEGFR2 itself is also a membrane receptor, we decided to score separately its localization on plasma membrane and intra/pan-cellular, respectively. An example of largely membrane staining for VEGFR2 in MB is given in ), and shows both values, in % of MB cells per lesion positive for intracellular VEGFR2/membrane VEGFR2. Notably, the plasma membrane expression pattern was apparent in only a small subset of MB cells per tumor, ranging from 0.5% to 20% of tumor cells (with only 4 of the 25 cases showing 7% or more membrane-positive cells), which is a range closely reminiscent of the CD133 range seen in this cohort (). In contrast, the overall intracellular pattern of VEGFR2 was observed in a much broader tumor cell subpopulation, with 6 cases reaching positivity in as many as 50–90% tumor cells, and all but 2 cases featuring over 10% positive MB cells ().
As the results summarized in demonstrate, the tumors in this cohort generally showed rather high proliferative indexes (Ki67 score), pronounced endogenous DNA damage (γH2AX marker) and a consistent expression, albeit only in a modest fraction of MB cells, of both IE and late HCMV proteins [Citation9]. Examples of immunohistochemical staining patterns for γH2AX and the HCMV proteins are shown in ), and the summary of the results for all markers is presented in . For 15 of the 25 tumors in our cohort, also the MB molecular class was available (). A comparison of the two widely recognized stem-cell markers, CD133 and CD15 with the MB class did not reveal any clear-cut restrictions in terms of high fraction of stemness-positive tumor cells in any specific MB class. On the other hand, it is noteworthy that two of the three cases with the highest fraction of CD133-positive tumor cells were of the Shh class, the only two known Shh-class cases in our cohort (). Furthermore, while 6 of the 12 MBs classified as group 3/4 tumors showed higher proportions of the CD15-positive tumor cells, also the only MB of the Wnt class showed high (30% of cells) CD15 expression (). These considerations are limited given the low numbers of cases in the individual MB classes; however, the overall pattern that is emerging is that a higher fraction of tumor cells featuring the stemness markers can likely occur in any of the currently recognized molecular classes of medulloblastoma. The potential significance and implications of the results presented in are further considered in the Discussion section.
3.2. Stem-cell features and signaling response to ionizing radiation in human MB cell lines
While the above analyses of patients’ specimens are valuable in terms of direct clinical relevance, we then wished to address some of the goals of this study experimentally, and therefore turned to available human cell line models established from MBs. The three human MB-derived cell lines that we examined here include DAOY, D324 and D283 (see Materials and methods). The first striking difference that we noticed when growing these three cell lines in parallel in the same standard medium was the distinct morphology and overall growth pattern. Whereas the DAOY and D324 cell lines showed adherent growth with rather flat morphology in cell culture, the D283 cells were either mostly non-adherent or only very loosely adherent and formed characteristic sphere-like multicellular structures under the same cell culture conditions (), top images). Furthermore, when examined by immunostaining of fixed cells for expression of the most widely recognized stemness marker CD133, both DAOY and D324 were negative, in sharp contrast to the bulk of D283 cells that showed a clear plasma membrane positivity for the CD133 protein ()). Notably, the fraction of CD133-positive cells in exponentially growing D283 cultures was much higher than in any of the 25 clinical cases in our cohort (), suggesting a potential origin (and clonal expansion) of this cell line from MBCS. Among other molecular features that we examined, the extent of endogenous DNA damage deduced from fractions of cells with nuclear staining for the γH2AX marker was higher among the D283 cells, although variable positivity was also seen in the DAOY and D324 cultures, all grown under standard unperturbed conditions ()). Finally, staining for the p53 tumor suppressor protein showed virtually all cell nuclei in both DAOY and D324 models strongly positive, while the p53 staining pattern was more variable, albeit also overall strongly positive among the D283 cells (), bottom images). While the almost homogeneous and strong staining in the DAOY and D324 cell lines is reminiscent of the aberrant overabundance known to reflect p53 mutations [Citation21], the more variable pattern in the D283 cells might be due either p53 mutation, or strong p53 stabilization in response to the prevalent endogenous DNA damage signaling documented by the high abundance of γH2AX in these cells.
Figure 2. Morphology, selected markers and responses to ionizing radiation in the human medulloblastoma cell lines. (a) Top row: phase contrast microscopy images of exponentially growing DAOY, D324 and D283 cell lines; note the characteristic non-adherent, sphere-like growth pattern of the D283 cells, distinct from the flat morphology of the other cell lines. 2nd–4th row: examples of immunoperoxidase staining patterns for the stem-cell marker CD133, overall endogenous DNA damage (γH2AX) and the p53 tumor suppressor, as indicated, in the three MB cell lines grown under unperturbed conditions; Scale bar: 50 μm; (b) Immunoblot analysis of DNA damage markers in un-irradiated versus irradiated (one hour post 3Gy) MB cell lines and control BJ fibroblasts; note the high level of γH2AX in the D283 cells already before irradiation, and the distinct low RPA ser33 phosphorylation; the less apparent Chk1 and RPA response of BJ cells reflects mainly the lower S-phase dependent protein abundance of Chk1 and RPA, rather than aberrant responses. The DCS270 antibody used to detect total Chk2 levels is known to recognize the activated, T68-phosphorylated Chk2 less efficiently than unphosphorylated Chk2, hence the apparently weaker signals after IR, despite Chk2 levels do not fluctuate throughout the cell cycle [Citation37]
![Figure 2. Morphology, selected markers and responses to ionizing radiation in the human medulloblastoma cell lines. (a) Top row: phase contrast microscopy images of exponentially growing DAOY, D324 and D283 cell lines; note the characteristic non-adherent, sphere-like growth pattern of the D283 cells, distinct from the flat morphology of the other cell lines. 2nd–4th row: examples of immunoperoxidase staining patterns for the stem-cell marker CD133, overall endogenous DNA damage (γH2AX) and the p53 tumor suppressor, as indicated, in the three MB cell lines grown under unperturbed conditions; Scale bar: 50 μm; (b) Immunoblot analysis of DNA damage markers in un-irradiated versus irradiated (one hour post 3Gy) MB cell lines and control BJ fibroblasts; note the high level of γH2AX in the D283 cells already before irradiation, and the distinct low RPA ser33 phosphorylation; the less apparent Chk1 and RPA response of BJ cells reflects mainly the lower S-phase dependent protein abundance of Chk1 and RPA, rather than aberrant responses. The DCS270 antibody used to detect total Chk2 levels is known to recognize the activated, T68-phosphorylated Chk2 less efficiently than unphosphorylated Chk2, hence the apparently weaker signals after IR, despite Chk2 levels do not fluctuate throughout the cell cycle [Citation37]](/cms/asset/7cfda865-a5a9-445d-ac12-e55c7a324667/kccy_a_1728025_f0002_oc.jpg)
The above characteristics revealed striking phenotypic differences between the two cell lines DAOY and D324 on the one hand, and the pronounced stem cell-like features of the D283 cells on the other. To see whether these phenotypic traits may also correlate with differential cellular responses to genotoxic insults, we first examined signaling responses to ionizing radiation, a modality that is part of the standard-of-care treatment schemes for medulloblastomas. A multi-panel example of immunoblotting analysis of cell extracts prepared from exponentially growing MB cell lines exposed to a clinically relevant dose (3Gy) of ionizing radiation is presented in ). As can be seen from this analysis, all three cell lines showed the expected increase of the Chk2 and Chk1 kinase activating phosphorylations, as well as increased overall levels of γH2AX, the latter marker being already very high in the unirradiated D283 cells. A parameter that was clearly distinct in the D283 cells was a very modest phosphorylation of the replication protein A at serine 33 (RPA pS33), a marker that was much more robustly evident in the DAOY and D324 cell lines already before irradiation.
We conclude that, in contrast to the other two MB cell lines, the D283 cells show phenotypic traits reminiscent of stemness (spontaneous sphere formation, high CD133), suggesting that D283 might offer a convenient model system to study MBSCs. Whereas all 3 MB cell models show broadly similar signaling responses to ionizing radiation, the D283 cells feature higher degree of “spontaneous”/endogenous DNA damage (γH2AX) and an unorthodox phosphorylation pattern of the replication protein A, results that are further explored in the ultimate section of this manuscript, devoted to replication stress.
3.3. The MBSC-like D283 cells show higher infectivity by, and capacity to express, HCMV
As HCMV proteins have been detected in human clinical samples of MBs in several studies including our own [Citation9,Citation10], we next examined the extent to which the MB cell line models can be infected by a commonly used clinical strain of the HCMV (VR1814) and express the key HCMV immediate early transcription factors IE72 and IE86, in time-course experiments. To this end, considering the biological differences among the three MB cell lines revealed in the previous section of our present study, we chose the MBSC-like D283 cells, and the DAOY line as a representative of the two “non-stem” MB lines for such comparative infection experiments, side by side with a normal human fibroblast strain, BJ. The reason to choose the latter cell type was that like other diploid human fibroblasts, the BJ cells are permissive for infection by HCMV and show a robust IE72/IE86 expression with well-defined kinetics [Citation9]. The three cell types were either infected with the VR1814 HCMV strain at MOI 3 for 1 h or mock-infected (as parallel non-infected controls, NI), after which the cells were washed twice to remove any remaining virus particles from the cell cultures, and then grown for different periods of time post-infection. At 24 and 96 h post-infection (hpi), the cell populations were analyzed by either flow cytometry or immunofluorescence microscopy, to obtain information about cell cycle profiles and expression of the HCMV IE72/IE86 protein. As can be seen from the flow cytometry results presented in ), the D283 cells showed substantially more IE72/IE86 protein expression compared to the other MB model of DAOY cells. It is also apparent that while the proportion of S-phase cells was reduced at the 96 h post-infection in both cell lines, the double-parameter flow cytometry profiles show that the abundant expression of the HCMV IE72/IE86 proteins in D283 cells was detectable in all cell cycle phases ()). The flow cytometry data were consistent with parallel immunofluorescence examination of fractions of IE72/IE86-positive cells in all three infected cell types ()). Whereas only rare IE72/IE86-expressing cells were seen in the DAOY population (around 2%) at either 24 or 96 h post-infection ()), the fraction of IE72/86-positive cells in the stem-cell-like D283 cell model ranged between 40% and 60% ()), not much below the IE72/IE86 expression seen in the HCMV-permissive BJ cells ()). Furthermore, unlike the permissive BJ cells that are soon arrested and eventually produce HCMV particles and die, the infected D283 cells were able to survive and grow/proliferate despite the infection (Supplementary figure S1A, B). Notably, consistent with their viability while infected by HCMV, and despite showing a high propensity to become infected and express IE72/IE86, the stem-like D283 cells did not produce infectious particles, based on testing their culture supernatant on HCMV-sensitive BJ cells (Supplementary figure S1C, D).
Figure 3. Flow cytometry profiles and HCMV IE72 expression in MB cell lines and BJ cells. (a) Cell cycle profiles of DAOY (left) and D283 (right) medulloblastoma cell lines. DAOY and D283 cells were infected with VR1814 HCMV (MOI 3) and their cell cycle profiles were analyzed by flow cytometry after propidium iodide staining at 24 and 96 hpi. Additionally, the expression of IE72 was assessed in both cell lines by two-parameter flow cytometry. NI – non-infected. (b–d) Medulloblastoma cell lines DAOY (b) and D283 (c) and normal BJ fibroblasts (d) were infected with VR1814 HCMV (MOI 3) and analyzed for expression of IE72 by fluorescence microscopy at 24 and 96 hpi. Graphs show the mean percentage of IE72-positive cells ± s.d.; NI – non-infected
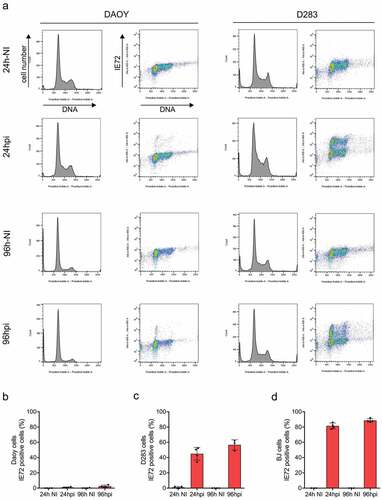
Overall, we conclude that in contrast to very low infectivity of the medulloblastoma DAOY cells, the MBSC-like D283 cells are highly susceptible to HCMV infection and abundantly express IE proteins and tolerate HCMV infection. While the D283 cells seem to be non-permissive for the production of infective HCMV, themselves being highly susceptible to infection may potentially have multifaceted phenotypic consequences due to the ability of the IE transcription factors to impact host-cell gene expression and thereby function of several important cell-regulatory pathways.
3.4. High chronic endogenous replication stress and aberrant signaling in the MBSC-like cells
Endogenous replication stress (RS) is an emerging hallmark of cancer [Citation11,Citation23,Citation41], a concept that is also consistent with our recent report on frequent and widespread occurrence of RS markers in the clinical cohort of medulloblastomas [Citation9], the same cohort that we have examined here for the stem-cell markers (see the immunohistochemistry data above). To complement our previous report and shed more light on the extent of, response to, and molecular basis for RS in the cellular MB models characterized here, we first assessed responses of the three MB cell lines to the RS-inducing chemotherapy drug hydroxyurea, side by side with response to ionizing radiation ()). Immunoblot analysis of the untreated control cell lysates showed very low or absent phosphorylation of Kap1 (serine 824) and RPA2 (serine residues S4 and S8). As expected, Kap1 was strongly phosphorylated upon irradiation, a response that was more pronounced in the DAOY and D324 cells compared to a more modest response of the stem-cell-like D283 cells. More relevant to responses to RS, treatment with hydroxyurea evoked a very strong RPAS4/8 phosphorylation response selectively in the D283 cell line ()). This phosphorylation of RPA-S4/8 was independently verified by immunostaining of formalin-fixed, paraffin sections of cell pellets ()), where the variable positivity may reflect the fraction of cells in S phase during this short-term experiment.
Figure 4. MBSC-like D283 cells show aberrant response to drug-induced replication stress. (a) Immunoblot analysis of the indicated DNA damage markers in the indicated three MB cell lines, under non-treated (NT), irradiated (one hour post 3Gy) and Hydroxyurea treated (HU) cells; Note the more robust Kap1 phosphorylation after irradiation in DAOY and D324 cells compared to the stem-like D283 cells, and particularly the hyperactive RPA Ser4/8 phosphorylation response to hydroxyurea (2 h, 2 mM) treatment in the D283 cells; NT – not treated. (b) Examples of immunoperoxidase staining patterns of RPA Ser4/8 phosphorylation in untreated and hydroxyurea treated D283 cells; Scale bar: 50 ◽m
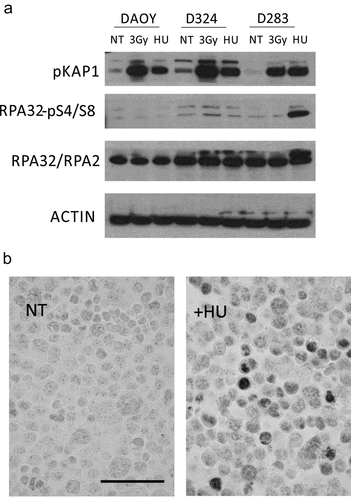
These results suggested a rather unique/hyperactive response mode of the D283 cells to RS, and prompted us to perform a detailed comparative analysis of replication fork function in the three MB cell line models, using the ultimate approach to RS analysis, namely DNA fiber assays under both unperturbed growth conditions and in cells challenged by treatment with hydroxyurea. The DNA fiber analysis showed that the MBSC-like D283 cells indeed experience an exceptionally high level of chronic endogenous replication stress, as indicated by slower replication fork speed progression and increased proportion of asymmetric forks, in comparison with either the D324 or DAOY cells under unperturbed growth conditions ()). Moreover, a short exposure to hydroxyurea (HU) induced robust fork arrest in the D283 cell line and the stalled forks could not recover efficiently from the treatment ()). The other two medulloblastoma cell lines, D324 and DAOY, were affected to a much lower extent ().
Figure 5. DNA fiber analysis in MB cell lines reveals high replication stress in D283. (a) Representative images of double-labeled DNA fibers. Medulloblastoma cell lines D324 (top), D283 (middle) and DAOY (bottom) were pulse-labeled with CldU (red) for 20 min, washed and pulse-labeled with IdU (green) for subsequent 20 min. Nucleosides were detected with antibodies and fiber length measured. (b) The length of CldU-IdU pulses from (a) was converted into kb/min. The graph shows total fork speed. Mean fork speed D324 = 1.1 kb/min, scored forks n = 479; D283 = 0.8 kb/min, n = 650; DAOY = 1.38 kb/min, n = 262. (c) The ratio between CldU/IdU was analyzed and plotted as relative frequencies. Values >1.5 and <0.5 indicate highly asymmetric forks. (D) The diagram of experimental settings for (e, f) is shown. (e) Induction of fork arrest by hydroxyurea (HU) treatment (2 mM, 30 min) in medulloblastoma cell lines (CldU mean fork speed D324 = 0.14 kb/min, scored forks n = 523; D283 = 0.09 kb/min, n = 258; Daoy = 0.31 kb/min, n = 363). (f) Fork recovery after HU treatment in medulloblastoma cell lines. CldU and HU were washed, and cells were incubated for 20 min in the fresh medium containing IdU (IdU mean fork speed D324 = 0.28 kb/min; D283 = 0.16 kb/min; DAOY = 1.0 kb/min). Whiskers in box plots indicate the 5–95th percentiles and the center values depict the median. Scale bars, 10 μm
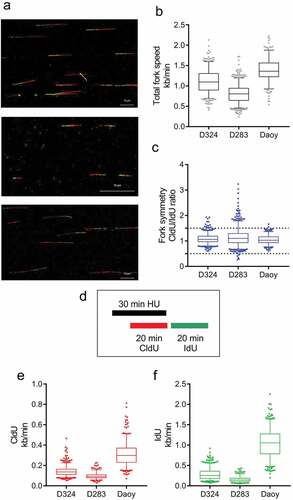
Collectively, these results further emphasize the distinct pattern of elevated endogenous replication stress and aberrant responses to RS-inducing insults in the MBSC-like D283 cell model.
4. Discussion
The results obtained in this study broaden the current knowledge in the field of cell stemness in general, and specifically for medulloblastoma stem-like cells, particularly in the context of endogenous replication stress and HCMV infection. While these three aspects of tumor pathobiology have been reported to exist in human medulloblastomas and other types of malignancies originating from the central nervous system [Citation9,Citation30], there are no studies to date attempting to functionally connect all three phenomena in a coherent manner. Our present dataset offers several advances toward addressing such potential interplay of stemness, replication stress and HCMV infection, by combining clinical material with cellular models amenable to experimentation.
First, in terms of the stem-like-cell markers in our clinical material, we found small subsets, ranging mostly between 0.5% and 5% of tumor cells expressing the CD133 (22 of 25 cases) and also the plasma membrane-localized VEGFR2 (21 of 25 cases) markers, while the CD15 marker was expressed more widely, with 17 of 25 cases showing 7–40% positive tumor cells (). These differences, seen on parallel consecutive tissue sections, suggest that CD15 may identify subsets of MB cells broader than the bona fide stem-like cells, possibly including also some more differentiated progenitor cells. What we can conclude from our results is that at least from the 3 cases that showed subsets of CD133-positive cells above 5% (cases 3, 5 and 11) two also showed more than 10% of CD15-positive MB cells, while the membrane VEGFR2 was rather low in these cases. On the other hand, 3 of the 4 cases showing higher membrane VEGFR2 (cases 5, 13, 14, 15) expression also showed more than 10% CD15-positive MB cells.
CSC markers did not show any strict correlation with either proliferation rate (Ki67), the extent of endogenous DNA damage (γH2AX), molecular class, or HCMV protein expression, the latter uniformly encompassing subsets of 10% or fewer HCMV-positive tumor cells per lesion in all cases. For example, the only case of secondary (recurrent) MB in our series, no. 16 () showed the highest proliferation rate among the entire cohort, very high degree of endogenous DNA damage and one of the highest HCMV protein expression patterns, yet only 1% of the cells were positive for either CD133 or membrane VEGFR2. What we suggest with confidence is that the overall pan-cellular VEGFR2 is very unlikely to identify the stem-like cells in medulloblastomas, simply because these subsets of cells are far too abundant (). The issue of VEGFR2 as a candidate MBSC marker is further complicated by the fact that the protein is likely dynamically recycled between the plasma membrane and the intracellular compartment. Overall, it is apparent from our results obtained with the clinical specimens, that addressing the conceptual issue of diverse subclasses among the MBSCs, their potential plasticity, and phenotypic impact on a given tumor will require larger cohorts and studies including very sensitive double-fluorescence staining methods for in-situ analysis, as well as side-by-side sorting of cell subsets from fresh surgical specimens using these markers, followed by marker cross-examination and analysis of biological properties of such isolated cell fractions. Furthermore, while the overall percentage range of the stem-cell marker CD133-positive and HCMV protein-positive cancer cells were similar at the cohort level, making a direct comparison at the level of individual cancer cells would require very sensitive immunofluorescence double-staining protocols (for simultaneous detection of, e.g., CD133 and IE72/86 proteins) applicable on archival tissue sections, an approach that is still missing in the field. It also remains to be seen whether the markers used here might more tightly correlate with the emerging more sophisticated molecular sub-classification of medulloblastomas, which postulates 4 subtypes (alpha to delta) within the Shh class, and 3 subtypes (alpha to gamma) within each of the currently recognized main classes 3, 4 and Wnt, respectively [Citation42].
The other approach to the main goal of this study was to search for a potential cellular model representing MB stem cells, and applying a range of cell and molecular biology techniques to assess links to replication stress and HCMV infection, comparing such MBSC model with other, “non-stem” human MB-derived cell lines. To this end, we report here that the D283 cell line, featuring a sphere-forming growth pattern in standard media, and highly abundant CD133 membrane expression, appears to be a good candidate for an MBSC-like model. On the other hand, the MB cell lines DAOY and D324, which showed a highly adherent flat morphology and no CD133 expression, provided models of “non-stem” MB cells under standard cell culture conditions used here, although in special stemness-supporting culture environments also the DAOY cell line shows a small subset of sphere-forming CD133-expressing cells [Citation43]. Using the MB cell line models, one of the major novel results of our present study was the finding that the stem-like D283 cells are highly susceptible to infection by HCMV, demonstrating expression of the viral transcription factors IE72/86 in 40–60% of the cells, and in all phases of the cell cycle. This was unexpected, given that the clear majority of human cancer cell lines are difficult to infect with HCMV, the DAOY cells being one such example, with only very rare cells expressing IE proteins despite infected with the same viral strain, the same MOI and under the same culture conditions. The fate of virus infection is apparently different in these different MB cell models, resulting in a rather abundant yet non-permissive infection in stem cell-like MB cells allowing for viral IE72/86 protein expression that may have genome destabilizing and other tumor-promoting effects on these cells, while not able to establish a lytic infection resulting in G1 cell cycle blockade or cell death. This preferential infection of CD133-positive MB cells by HCMV is even more intriguing considering that in human primary gliomas, experimental HCMV infection triggered a stem-cell phenotype, and that the extent of concomitant expression of the HCMV IE72/86 protein and CD133 in clinical glioblastoma specimens predicted poor patient survival [Citation44].
Even more relevant to tumorigenesis, clonal evolution and response to treatment are our present results demonstrating that the D283 cells show an altered, and rather unique pattern of molecular responses to genotoxic insults, including ionizing radiation at a clinically relevant moderate dose, as well as to drug-induced replication stress. Whereas the radiation response by Chk2 and Chk1 kinase phosphorylation was similar in the three MB cell lines and followed the expected pattern, the irradiated D283 cells showed dramatically decreased phosphorylation of RPA Ser33, and moderately lower Kap1 phosphorylation compared to the CD133-negative cell lines DAOY and D324. Furthermore, the D283 cells showed high chronic endogenous DNA damage signaling documented by the constitutively elevated γH2AX signal and, when treated with hydroxyurea, responded in a robustly “hyperactive mode” in terms of RPA Ser4/8 phosphorylation, collectively indicative of potentially high degree of endogenous replication stress. Indeed, the subsequent single-molecule DNA fiber assays proved this prediction, as the D283 cells featured aberrantly slow speed of replication for progression and pronounced fork asymmetry under unperturbed growth conditions. Furthermore, when transient replication fork stalling was experimentally induced by a pulse-exposure to hydroxyurea, the stalled forks in the D283 cells could not restart and timely resume progression, unlike those in the DAOY or D234 cells which showed the expected “normal” pattern for fork recovery. These results are significant, as we previously observed signs of enhanced endogenous replication stress in the clinical samples from primary MBs, surgically removed before the patients became treated by the combined radio-chemotherapy [Citation9]. Given the present results with the MB models, the overall scenario that is emerging is that the MB cells in general, and possibly even more so the medulloblastoma stem-like cells, experience enhanced replication stress, likely due to impaired DNA replication, deregulated by oncogenes such as Myc or the aberrant Shh pathway. The persistent RS, in turn, may evoke adaptive changes in the cellular signaling pathways that normally respond to abnormal replication structures and DNA lesions, possibly leading to unorthodox signaling responses along the line we found in the D283 cells. Such adaptations might, at least to some extent reflect an untimely re-appearance of an intrinsic program associated with stemness, since embryonic stem cells and early embryonic development show a high degree of replication stress [Citation45]. But unlike during physiological embryogenesis, the enhanced replication stress in cancer stem cells is associated with abnormal oncogenic cues and/or loss of important tumor suppressor functions. While the adaptive rewiring of cellular DNA damage signaling pathways may allow the chronically stressed, oncogene-driven MB cells (and MBSCs in particular) to survive and proliferate, the ongoing elevated RS must inevitably undermine the genomic integrity of such cells, analogous to some models of oncogene-triggered RS in other cell types [Citation11]. It remains to be established whether ectopic expression of HCMV IE72/86 alone can induce replication stress, and whether medulloblastoma patients may benefit from add-on antiviral therapy with drugs such as ganciclovir. Notably, the enhanced genomic instability caused by replication stress commonly promotes tumor cell heterogeneity and fuels both tumor resistance to standard-of-care genotoxic treatment modalities and tumor recurrence [Citation2,Citation22], two major hurdles in contemporary oncology. These two major challenges are also faced by neurosurgeons and pediatric oncologists treating medulloblastomas, in an ongoing effort to which we hope our present results may provide some inspiring clues to be pursued in future studies.
Supplemental Material
Download PDF (1.3 MB)Disclosure statement
No potential conflict of interest was reported by the authors.
Supplementary material
Supplementary material for this article can be accessed here.
Additional information
Funding
References
- Yi Y, Hsieh IY, Huang X, et al. Glioblastoma stem-like cells: characteristics, microenvironment, and therapy. Front Pharmacol. 2016;7:477.
- Burrell RA, McClelland SE, Endesfelder D, et al. Replication stress links structural and numerical cancer chromosomal instability. Nature. 2013;494:492–496.
- Harkins L, Volk AL, Samanta M, et al. Specific localisation of human cytomegalovirus nucleic acids and proteins in human colorectal cancer. Lancet. 2002;360:1557–1563.
- Samanta M, Harkins L, Klemm K, et al. High prevalence of human cytomegalovirus in prostatic intraepithelial neoplasia and prostatic carcinoma. J Urol. 2003;170:998–1002.
- Harkins LE, Matlaf LA, Soroceanu L, et al. Detection of human cytomegalovirus in normal and neoplastic breast epithelium. Herpesviridae. 2010;1:8.
- Taher C, Frisk G, Fuentes S, et al. High prevalence of human cytomegalovirus in brain metastases of patients with primary breast and colorectal cancers. Transl Oncol. 2014;7:732–740.
- Cobbs CS, Harkins L, Samanta M, et al. Human cytomegalovirus infection and expression in human malignant glioma. Cancer Res. 2002;62:3347–3350.
- Rahbar A, Orrego A, Peredo I, et al. Human cytomegalovirus infection levels in glioblastoma multiforme are of prognostic value for survival. J Clin Virol. 2013;57:36–42.
- Bartek J Jr., Fornara O, Merchut-Maya JM, et al. Replication stress, DNA damage signalling, and cytomegalovirus infection in human medulloblastomas. Mol Oncol. 2017;11:945–964.
- Baryawno N, Rahbar A, Wolmer-Solberg N, et al. Detection of human cytomegalovirus in medulloblastomas reveals a potential therapeutic target. J Clin Invest. 2011;121:4043–4055.
- Halazonetis TD, Gorgoulis VG, Bartek J. An oncogene-induced DNA damage model for cancer development. Science. 2008;319:1352–1355.
- Kanu N, Cerone MA, Goh G, et al. DNA replication stress mediates APOBEC3 family mutagenesis in breast cancer. Genome Biol. 2016;17:185.
- Lugli N, Sotiriou SK, Halazonetis TD. The role of SMARCAL1 in replication fork stability and telomere maintenance. DNA Repair (Amst). 2017;56:129–134.
- Galanos P, Vougas K, Walter D, et al. Chronic p53-independent p21 expression causes genomic instability by deregulating replication licensing. Nat Cell Biol. 2016;18:777–789.
- Galanos P, Pappas G, Polyzos A, et al. Mutational signatures reveal the role of RAD52 in p53-independent p21-driven genomic instability. Genome Biol. 2018;19:37.
- Bartkova J, Horejsi Z, Koed K, et al. DNA damage response as a candidate anti-cancer barrier in early human tumorigenesis. Nature. 2005;434:864–870.
- Gorgoulis VG, Vassiliou LV, Karakaidos P, et al. Activation of the DNA damage checkpoint and genomic instability in human precancerous lesions. Nature. 2005;434:907–913.
- Bartkova J, Bakkenist CJ, Rajpert-De Meyts E, et al. ATM activation in normal human tissues and testicular cancer. Cell Cycle. 2005;4:838–845.
- Bartkova J, Rezaei N, Liontos M, et al. Oncogene-induced senescence is part of the tumorigenesis barrier imposed by DNA damage checkpoints. Nature. 2006;444:633–637.
- Bartkova J, Hamerlik P, Stockhausen MT, et al. Replication stress and oxidative damage contribute to aberrant constitutive activation of DNA damage signalling in human gliomas. Oncogene. 2010;29:5095–5102.
- Bartek J, Bartkova J, Lukas J. DNA damage signalling guards against activated oncogenes and tumour progression. Oncogene. 2007;26:7773–7779.
- Burrell RA, McGranahan N, Bartek J, et al. The causes and consequences of genetic heterogeneity in cancer evolution. Nature. 2013;501:338–345.
- Bartek J, Mistrik M, Bartkova J. Thresholds of replication stress signaling in cancer development and treatment. Nat Struct Mol Biol. 2012;19:5–7.
- Kool M, Korshunov A, Remke M, et al. Molecular subgroups of medulloblastoma: an international meta-analysis of transcriptome, genetic aberrations, and clinical data of WNT, SHH, Group 3, and Group 4 medulloblastomas. Acta Neuropathol. 2012;123:473–484.
- Holgado BL, Guerreiro Stucklin A, Garzia L, et al. Tailoring medulloblastoma treatment through genomics: making a change, one subgroup at a time. Annu Rev Genomics Hum Genet. 2017;18:143–166.
- Thompson EM, Hielscher T, Bouffet E, et al. Prognostic value of medulloblastoma extent of resection after accounting for molecular subgroup: a retrospective integrated clinical and molecular analysis. Lancet Oncol. 2016;17:484–495.
- Wang X, Ramaswamy V, Remke M, et al. Intertumoral and intratumoral heterogeneity as a barrier for effective treatment of medulloblastoma. Neurosurgery. 2013;60(Suppl 1):57–63.
- Northcott PA, Korshunov A, Pfister SM, et al. The clinical implications of medulloblastoma subgroups. Nat Rev Neurol. 2012;8:340–351.
- Polkinghorn WR, Tarbell NJ. Medulloblastoma: tumorigenesis, current clinical paradigm, and efforts to improve risk stratification. Nat Clin Pract Oncol. 2007;4:295–304.
- Soderberg-Naucler C, Johnsen JI. Cytomegalovirus infection in brain tumors: A potential new target for therapy? Oncoimmunology. 2012;1:739–740.
- Soderberg-Naucler C, Johnsen JI. Cytomegalovirus in human brain tumors: role in pathogenesis and potential treatment options. World J Exp Med. 2015;5:1–10.
- Baumgarten P, Michaelis M, Rothweiler F, et al. Human cytomegalovirus infection in tumor cells of the nervous system is not detectable with standardized pathologico-virological diagnostics. Neuro Oncol. 2014;16:1469–1477.
- Sardi I, Lucchesi M, Becciani S, et al. Absence of human cytomegalovirus infection in childhood brain tumors. Am J Cancer Res. 2015;5:2476–2483.
- Yamashita Y, Ito Y, Isomura H, et al. Lack of presence of the human cytomegalovirus in human glioblastoma. Mod Pathol. 2014;27:922–929.
- Cojoc M, Mabert K, Muders MH, et al. A role for cancer stem cells in therapy resistance: cellular and molecular mechanisms. Semin Cancer Biol. 2015;31:16–27.
- Turi Z, Senkyrikova M, Mistrik M, et al. Perturbation of RNA Polymerase I transcription machinery by ablation of HEATR1 triggers the RPL5/RPL11-MDM2-p53 ribosome biogenesis stress checkpoint pathway in human cells. Cell Cycle. 2018;17:92–101.
- Lukas C, Bartkova J, Latella L, et al. DNA damage-activated kinase Chk2 is independent of proliferation or differentiation yet correlates with tissue biology. Cancer Res. 2001;61:4990–4993.
- Bartkova J, Bartek J, Vojtesek B, et al. Immunochemical analysis of the p53 oncoprotein in matched primary and metastatic human tumours. Eur J Cancer. 1993;29A:881–886.
- Maya-Mendoza A, Moudry P, Merchut-Maya JM, et al. High speed of fork progression induces DNA replication stress and genomic instability. Nature. 2018;559:279–284.
- Hamerlik P, Lathia JD, Rasmussen R, et al. Autocrine VEGF-VEGFR2-Neuropilin-1 signaling promotes glioma stem-like cell viability and tumor growth. J Exp Med. 2012;209:507–520.
- Jackson SP, Bartek J. The DNA-damage response in human biology and disease. Nature. 2009;461:1071–1078.
- Cavalli FMG, Remke M, Rampasek L, et al. Intertumoral Heterogeneity within Medulloblastoma Subgroups. Cancer Cell. 2017;31:737–54 e6.
- Gong C, Valduga J, Chateau A, et al. Stimulation of medulloblastoma stem cells differentiation by a peptidomimetic targeting neuropilin-1. Oncotarget. 2018;9:15312–15325.
- Fornara O, Bartek J Jr., Rahbar A, et al. Cytomegalovirus infection induces a stem cell phenotype in human primary glioblastoma cells: prognostic significance and biological impact. Cell Death Differ. 2016;23:261–269.
- Alvarez S, Diaz M, Flach J, et al. Replication stress caused by low MCM expression limits fetal erythropoiesis and hematopoietic stem cell functionality. Nat Commun. 2015;6:8548.