ABSTRACT
LncRNAs play important roles in the regulation of podocyte apoptosis in diabetic nephropathy (DN). However, the role of lncRNA SNHG17 in controlling mitophagy-induced apoptosis of podocytes in DN is unknown. This study aims to elucidate the underlying mechanism of lncRNA SNHG17 in the regulation of mitophagy-induced apoptosis of podocytes in DN. LncRNA SNHG17 and Mammalian Sterile 20-like kinase 1 (Mst1) expression were upregulated in glomeruli and podocytes of DM mice and high glucose-treated podocytes, whereas Parkin expression was downregulated. LncRNA SNHG17 overexpression suppressed mitophagy and induced apoptosis of podocytes while silencing lncRNA SNHG17 promoted mitophagy and reduced the apoptosis of podocytes. In addition, lncRNA SNHG17 interacted with Mst1 and regulated the degradation of Mst1. We further found lncRNA SNHG17 regulated Parkin expression through Mst1. Mechanistically, lncRNA SNHG17 regulated Parkin-dependent mitophagy and apoptosis of podocytes through regulating Mst1. Finally, silencing lncRNA SNHG17 promoted mitophagy and relieved DNin vivo. In conclusion, lncRNA SNHG17 knockdown promotes Parkin-dependent mitophagy and reduces apoptosis of podocytes through regulating the degradation of Mst1.
Introduction
Diabetic nephropathy (DN) is one of the common microvascular complications of diabetes mellitus, which is characterized by increased urine protein excretion, elevated blood pressure, and declined glomerular filtration [Citation1]. Although therapeutic interventions have shown to postpone the progression of DN, the morbidity and mortality of DN remain high and new treatment measures are needed [Citation2–Citation4]. Podocytes are highly specialized and terminally differentiated cells, which play important roles in maintaining glomerular filtration barrier and the derangements of podocytes in pathological states will lead to albuminuria [Citation5]. Many studies have shown that excessive apoptosis of podocytes contributes to the progression of DN [Citation6,Citation7]. Recent studies also show that mitophagy dysregulation is closely related to the apoptosis of podocytes [Citation8,Citation9]. However, the molecular mechanisms that control mitophagy-induced apoptosis of podocytes in DN are still unclear.
More and more studies have shown that long non-coding RNAs (lncRNAs) play important roles in the regulation of podocyte apoptosis in DN [Citation7,Citation10]. LncRNA Small Nucleolar RNA Host Gene 17 (SNHG17), located on human chromosome 20, was firstly found to be highly expressed in colorectal cancer tissues [Citation11]. LncRNA SNHG17 knockdown promotes the apoptosis of colorectal cancer cells through binding to the enhancer of zeste homolog 2 [Citation11]. In addition, lncRNA SNHG17 can bind to the enhancer of zeste homolog 2 in gastric cancer, thus affecting the proliferation, cell cycle, invasion/migration, and apoptosis of gastric cancer cells [Citation12]. These evidences indicate that lncRNA SNHG17 has the potential ability of binding to protein to modulate the level of protein. However, whether the aberrant expression of lncRNA SNHG17 occurs during the apoptosis of podocytes in DN is still unclear.
In the present study, we investigate the role of lncRNA SNHG17 in the mitophagy and apoptosis of podocytes and determine whether lncRNA SNHG17 regulates mitophagy-induced apoptosis of podocytes through regulating protein stability in vivo and in vitro.
Materials and methods
Diabetic mice model
Male C57BL/6 mice (3-month-old) were purchased from the Experimental Animal Center of Henan Province and maintained in a 12 h light/dark cycle environment. After fasting for 12 h, 130 mg/kg streptozocin (STZ; Sigma-Aldrich, Missouri, USA) dissolved in 0.05 M citrate buffer (pH 4.5) was intraperitoneally injected into the mice to induce diabetes (n = 7). Mice injected with citrate buffer alone were used as normal control mice (n = 7). Seventy-two days after injection of STZ or citrate buffer, mice with post fasting blood glucose (200–300 mg/dl) were considered as diabetic mice. Twelve weeks after injection, 24-h urine from each mouse was collected for the detection of 24-h urine protein level. Then, all mice were anesthetized and perfused with 8 × 107 dynabeads (Invitrogen, CA, USA) diluted in 40 ml PBS through the heart. Later, the mice were sacrificed by an overdose of pentobarbital sodium, and kidney tissues were collected for the isolation of glomeruli and podocytes.
Adeno-associated virus serotype 9 (AAV9) system carrying siRNA-SNHG17 (rAAV9-siRNA-SNHG17) was purchased from GENECHEM (Shanghai, China). An empty AAV9 vector (rAAV9-NC) was used as a negative control. For the observation of silencing SNHG17 in diabetic mice, 100 μl rAAV9-siRNA-SNHG17 (n = 7) or rAAV9-NC (n = 7) was injected to the caudal vein of diabetic mice. The viral titer of AAV9 vectors was 2 × 1013 genomes/ml, resulting in approximately 2 × 1012 genomes per injection. All the experiments were approved by the Ethics Committee of the First Affiliated Hospital of Zhengzhou University and adhered to the Declaration of Helsinki guidelines.
Isolation of glomeruli and podocytes
Glomeruli were isolated as described [Citation13]. Kidney tissues were minced into small pieces (1 mm3) and digested with 1 mg/ml collagenase A (Sigma-Aldrich) and 100 U/ml deoxyribonuclease I (Sigma-Aldrich) for 30 min. The digested tissues were gently passed through a 100 μm cell strainer with pressing with a flattened pestle, and 5 ml Hank’s Balanced Salt Solution (HBSS; Gibco, NY, USA) was used to wash the cell strainer. Then, the cells were gently passed through a new cell strainer without pressing and 5 ml HBSS was used to wash the cell strainer again. The cells were centrifuged for 5 min at 200 × g, the precipitates were re-suspended in 2 ml HBSS. Glomeruli were collected using a magnetic stand and washed with HBSS three times. Glomeruli were further incubated in RPMI 1640 medium (Gibco) for seven days. Outgrowing epithelial cells were trypsinized and filtered through a 33 μm sieve, then incubated in RPMI 1640 medium supplemented with 10% fetal bovine serum (FBS; Gibco) at 37°C. The primary podocytes were confirmed by the substantial expression of podocyte-specific markers (Wilms’ tumor-1 protein and nephrin). Then, the mitochondria fractions were isolated from podocytes utilizing a Mitochondria Isolation Kit (Sigma-Aldrich).
Western blot
Proteins were extracted from glomeruli and podocytes using RIPA Lysis Buffer (Beyotime Biotechnology, Nantong, China). The concentration of protein was measured by Bradford Protein Assay Kit (Beyotime Biotechnology). The equal amount of proteins were separated by 12% SDS-PAGE, and transferred onto a PVDF membrane (Sigma-Aldrich). The membranes were blocked with 5% nonfat milk and subjected to primary antibodies. Antibodies against Drp-1 (ab184247; 1:1000 dilution; Abcam, UK), Mfn1 (ab129154; 1:10000 dilution; Abcam), p62 (ab155686; 1:3000 dilution; Abcam), LC3 (ab51520; 1:3000 dilution; Abcam), Mammalian Sterile 20-like kinase 1 (Mst1; PA5-53295; 1:1000 dilution; Invitrogen), Parkin (702785; 1:500 dilution; Invitrogen), β-actin (ab179467; 1:5000 dilution; Abcam) were obtained commercially. Then the membranes were incubated with a goat anti-rabbit IgG-HRP antibody (ab6721; 1:5000 dilution; Abcam) for 1.5 h. The membranes were immersed with BeyoECL Moon enhanced chemiluminescence kit (Beyotime Biotechnology), and visualized on Gel Doc™ EZ System (Bio-Rad, CA, USA).
qRT-PCR
Total RNAs were extracted from glomeruli and podocytes using Trizol reagent (Beyotime Biotechnology) according to the manufacturer’s instructions. RNAs were transcribed into cDNA using High Capacity cDNA Reverse Transcription Kit (Applied Biosystems, CA, USA). PCR mixtures (25 μl) containing 2.5 μM of primer pairs, cDNA template, and SYBR Green Real-Time PCR Master Mixes (Applied Biosystems) were subjected to PCR amplification using QuantStudio 5 Real-Time PCR System (Applied Biosystems). The relative SNHG17 and Mst1 expressions were calculated using the 2−ΔΔCT method. GAPDH was used as an internal reference.
Cell culture and transfection
Conditionally immortalized mouse podocytes (MPC5) were cultured in RPMI 1640 medium (Gibco) supplemented with 10% FBS (Gibco), 100 U/mL penicillin and 100 μg/mL streptomycin (Sinopharm Chemical Reagent Co., Ltd, Shanghai, China) in a 5% CO2 incubator at 37°C for 24 h. Then, podocytes (MPC5) were treated with high glucose (HG, 25 mM) or normal glucose (NG, 5.6 mM) for 24 h.
pcDNA-SNHG17 and pcDNA-Mst1 were constructed by inserting SNHG17 cDNA or Mst1 cDNA into plasmid pcDNA (Invitrogen). pcDNA was used as a negative control. For SNHG17 and Mst1 knockdown, small interference RNA targeting SNHG17 (siRNA-SNHG17) or siRNA-Mst1 were synthesized by RiboBio Co., Ltd. (Guangzhou, China). siRNA was used as a negative control. Transfection experiments were conducted using Lipofectamine 2000 reagent (Invitrogen).
Assessment of ATP level
ATP level was detected using the ATP Bioluminescence Assay Kit (Beyotime Biotechnology). MPC5 cells were lysed using RIPA Lysis Buffer (Beyotime Biotechnology), and centrifuged at 4°C for 5 min at 12,000 × g. The supernatant was collected, and the ATP detection reagent was added into the supernatant. The ATP level was measured using a luminescence luminometer (Promega, Wisconsin, USA).
Flow cytometry
MPC5 cells at different groups were collected after 48 h of treatment. Cells (5 × 104) were centrifuged for 5 min at 1000 × g, and the supernatant was discarded. Annexin V-EGFP binding buffer (195 μl) was added to re-suspend the cells. Then, 5 μl Annexin V-EGFP was added and mixed gently. After adding 10 μl propidium iodide staining solution, cells were incubated at 25°C for 20 min. Data analysis was performed using Cell Quest software using flow cytometer (FACScan; BD, USA)
RNA pull-down
Pierce Magnetic RNA-Protein Pull-Down Kit (Thermo Scientific) was used to determine the interaction between SNHG17 and Mst1. MPC5 cells were transfected with biotinylated SNHG17 and negative control (NC). The lysate was incubated with streptavidin magnetic beads. Then, the SNHG17-Mst1 complex bound to the beads were eluted and detected by western blot analysis.
RNA immunoprecipitation (RIP)
RIP assay was performed to confirm the interaction between SNHG17 and Mst1. Briefly, MPC5 cells were lysed with RIP lysis buffer. The lysate was incubated with an anti-Mst1 rabbit polyclonal antibody at 4°C overnight with Magnetic Beads protein A/G. The beads were washed with cold RIP wash buffer for six times. Then, beads were re-suspended in 150 μl Proteinase K buffer and incubated at 55°C for 30 min. RNA was extracted and purified. qRT-PCR was then conducted with the purified RNA. Total RNAs (input controls) and isotype controls (normal mouse IgG) were simultaneously determined.
Ubiquitination assay
To examine whether SNHG17 affects Mst1 ubiquitination, HA-Ub, FLAG-Mst1, siRNA-control, siRNA-SNHG17 were transfected into MPC5 cells using transfection reagent for 12 h. After transfecting, MPC5 cells were treated with MG132 (10 μM) for 4 h and then collected and lysed. Western blot analysis of cell lysates was used to confirm Mst1 protein expression. Then, cell lysates were immunoprecipitated with an anti-FLAG antibody at 4°C followed by immunoblotting with an anti-HA antibody.
Mondansylcadaverine (MDC) staining
To evaluate the formation of autophagosomes, MDC staining was performed on MPC5 cells. Cells were treated with MDC (0.05 mM) at 37°C. Ten minutes later, phosphate-buffer saline (PBS) was used to wash cells. Then, the autophagic vacuoles were observed under a microscope.
Statistical analysis
Data were presented as mean±standard deviation (SD), and statistical analysis of the data was performed using Student’s t-test or one-way ANOVA followed by Bonferroni posthoc test. P-value < 0.05 was considered statistical significance.
Results
LncRNA SNHG17 was elevated in glomeruli and podocytes of DM mice and high glucose-treated MPC5 cells
We established DM mice to investigate whether lncRNA SNHG17 was abnormally expressed in glomeruli and podocytes. As shown in ,b), the blood glucose (BG) level, urine protein (Upro) level, kidney weight (the percent of bilateral kidneys out of total body weight), and renal injury were significantly increased in DM mice than that of normal mice, indicating that DM mice were successfully established. As shown in ,d), the LC3II/LC3I ratio was downregulated whereas the p62 protein level was upregulated in glomeruli, podocytes, and mitochondria of podocytes in DM mice, indicating that mitophagy was suppressed in DM mice. Besides, the fusion-related protein Mfn1 was down-regulated while fission-related protein Drp-1 was up-regulated in glomeruliand podocytes (,d)), indicating that mitochondrial dynamics may be related to the podocyte apoptosis during DN. In addition, lncRNA SNHG17 expression and Mst1 protein level were significantly elevated in glomeruli and podocyte of DM mice, and the Parkin protein level was down-regulated in glomeruli, podocytes, and mitochondria of podocytes in DM mice (,d)). We further established in vitro high glucose-induced injury in MPC5 cells and found lncRNA SNHG17 expression and Mst1 expression were significantly elevated in high glucose-treated MPC5 cells, whereas Parkin protein level was reduced ()).
Figure 1. The expression levels of lncRNA SNHG17 in glomeruli and podocytes of DM mice. C57BL/6 mice were divided into the normal group (n = 7) and DM group (n = 7). a. Blood glucose (BG) level, urine protein (Upro) level, and kidney weight (%) were detected in the normal group and DM group. Upro and kidney weight were measured at 12 weeks after diabetic induction. The kidney weight (%) was determined as the percent of bilateral kidneys out of total body weight. b. HE staining of kidney tissue. Scar bar = 25 µm. c. Left: LncRNA SNHG17 expression in glomeruli was detected using qRT-PCR. Right: Mitofusin 1 (Mfn1), p62, light chain 3 (LC3), dynamin-related protein 1 (Drp-1), Mammalian Sterile 20-like kinase 1 (Mst1), and Parkin protein levels in glomeruli were detected in normal group and DM group by western blot. The most representative bands were shown. d. Left: LncRNA SNHG17 expression in podocyte was detected using qRT-PCR. Right: Mfn1, p62, LC3, Drp-1, Mst1, and Parkin protein levels in podocytes were detected using western blot. Below: Parkin, p62, and LC3 protein levels in mitochondria which were isolated from podocytes were detected using western blot. The most representative bands were shown. Podocytes (MPC5) were treated with high glucose (HG, 25 mM) or normal glucose (NG, 5.6 mM) for 24 h. e. LncRNA SNHG17 was detected in the NG group and HG group. Mst1 and Parkin protein levels were measured by western blot. *p < 0.05 vs Normal or NG.
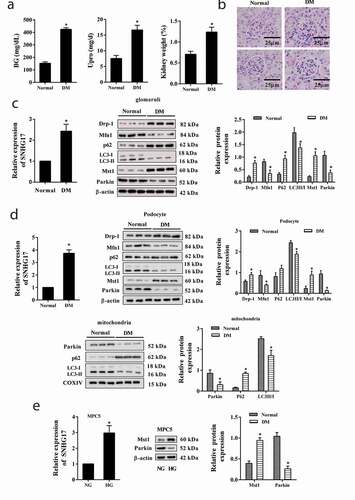
SNHG17 overexpression suppressed mitophagy and induced apoptosis of podocytes
To determine the role of lncRNA SNHG17 on mitophagy and the apoptosis of podocytes, MPC5 cells were transfected with pcDNA-SNHG17. As shown in ), pcDNA-SNHG17 reduced LC3II/LC3I ratio and Parkin protein level, and increased p62 protein levels in MPC5 cells and mitochondria of MPC5 cells, indicating SNHG17 overexpression suppressed mitophagy. In addition, pcDNA-SNHG17 significantly inhibited the ATP level ()) and promoted the apoptosis of podocytes ()).
Figure 2. SNHG17 overexpression suppressed mitophagy and induced apoptosis of podocytes. MPC5 cells were divided into pcDNA and pcDNA-SNHG17 groups. a. Left: The transfection efficiency of pcDNA-SNHG17 was measured by qRT-PCR. Right: Protein levels of Mfn1, p62, LC3, Parkin, and Drp-1 in the pcDNA group and the pcDNA-SNHG17 group were detected using western blot. Below: Protein levels of p62, LC3, and Parkin in the mitochondria of MPC5 cells were detected. b. ATP level was detected using the ATP Bioluminescence Assay Kit. c. The apoptosis of podocytes was detected using the flow cytometry method. Three replicates were used. *p < 0.05 vs pcDNA.
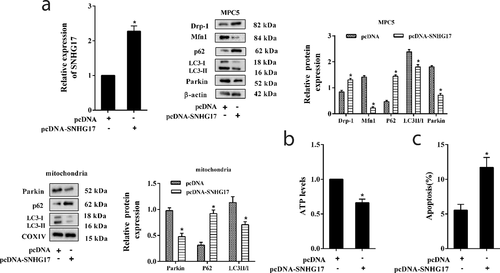
Silencing SNHG17 promoted mitophagy and reduced apoptosis of podocytes
We further investigated the effects of silencing SNHG17 on mitophagy and apoptosis of podocytes through transfecting siRNA-SNHG17 into MPC5 cells ()). As shown in ), high glucose suppressed LC3II/LC3I ratio, decreased Parkin protein level, and increased p62 protein level in MPC5 cells and mitochondria of MPC5 cells. Whereas siRNA-SNHG17 abolished the inhibition effect of high glucose on mitophagy in MPC5 cells. In addition, high glucose decreased ATP level, and siRNA-SNHG17 abolished the inhibition effect of high glucose on ATP production ()). High glucose also induced the apoptosis of podocytes, and siRNA-SNHG17 abolished the promotion effect of high glucose on podocyte apoptosis ()).
Figure 3. Silencing SNHG17 promoted mitophagy and reduced apoptosis of podocytes. MPC5 cells were transfected with si-control or siRNA-SNHG17, then treated with HG (25 mM) for 24 h. a. The transfection efficiency of siRNA-SNHG17 was measured by qRT-PCR. b: The protein levels of Parkin, p62, and LC3 in the mitochondria of MPC5 cells were detected using western blot. The protein levels of Mfn1, p62, LC3, Parkin, and Drp-1 in MPC5 cells were detected using western blot. c. ATP level was detected using the ATP Bioluminescence Assay Kit. d. The apoptosis of podocytes was detected using the flow cytometry method. Three replicates were used. *p < 0.05 vs NG; #p < 0.05 vs HG+si-control.
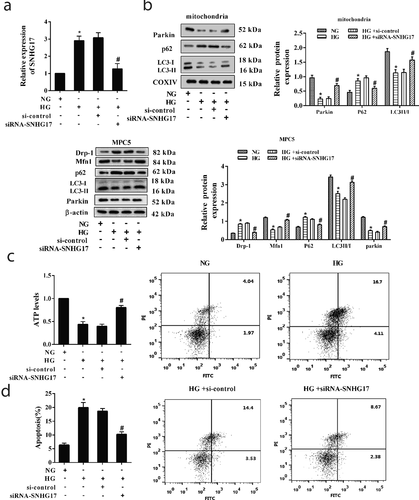
LncRNA SNHG17 regulated the degradation of Mst1
We conducted RNA pull-down and RIP assays to confirm the interaction between SNHG17 and Mst1. The result of RNA pull-down assay showed that Mst1 protein was detected in the SNHG17 pull-down complex ()), and the result of the RIP assay showed that SNHG17 was accumulated in Mst1 precipitate ()), indicating SNHG17 interacted with Mst1. Besides, siRNA-SNHG17 decreased the Mst1 protein level, and pcDNA-SNHG17 increased the Mst1 protein level ()), indicating Mst1 protein level was positively regulated by SNHG17. Whereas the mRNA level of Mst1 in MPC5 cells did not significantly change after the transfection of siRNA-SNHG17 ()), which indicated that SNHG17 may affect the ubiquitination of Mst1. Later, MPC5 cells were treated with CHX, and we found CHX promoted the degradation of Mst1 protein in MPC5 cells transfected with siRNA-SNHG17 ()). Moreover, siRNA-SNHG17 promoted the ubiquitination of Mst1 ()).
Figure 4. The interaction between SNHG17 and Mst1. a. RNA pull-down analysis of Mst1 expression in the SNHG17 pull-down complex. NC, negative control of the SNHG17 group. b. RIP analysis of SNHG17 expression in Mst1 precipitate. IgG was used as a negative control. *p < 0.05 vs IgG. c. Mst1 protein level in MPC5 cells transfected with siRNA-control, siRNA-SNHG17, pcDNA, or pcDNA-SNHG17. d. Relative mRNA levels of Mst1 in MPC5 cells which were transfected with siRNA-control or siRNA-SNHG17. e. MPC5 cells were transfected with siRNA-control or siRNA-SNHG17, then treated with CHX for 0 h, 3 h, 6 h, and 9 h. Mst1 protein level in MPC5 cells at different time points was detected using western blot. f. MPC5 cells were transfected with HA-Ub, FLAG-Mst1, siRNA-control, or siRNA-SNHG17 for 12 h, follwed by 10 μM MG132 treatment for 4 hours. Lysates of MPC5 cells were confirmed for Mst1 expression (bottom) and subjected to immunoprecipitation using an anti-FLAG antibody, followed by immunoblotting with an anti-HA antibody (top). Input, lysates of MPC5 cells.
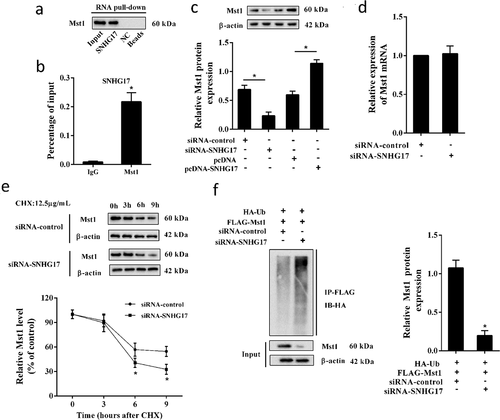
LncRNA SNHG17 regulated Parkin expression through Mst1
As shown in ), pcDNA-SNHG17 significantly decreased the protein level of Parkin in MPC5 cells, and siRNA-Mst1 abolished the inhibition effect of pcDNA-SNHG17 on Parkin expression without affecting SNHG17 expression ()). In high glucose-treated MPC5 cells, siRNA-SNHG17 significantly increased the protein level of Parkin, and pcDNA-Mst1 abolished the promotion effect of siRNA-SNHG17 on Parkin expression ()) without affecting SNHG17 expression ()). These findings suggested that lncRNA SNHG17 inhibited Parkin expression through regulating Mst1.
Figure 5. SNHG17 regulated Parkin expression through Mst1. MPC5 cells were divided into pcDNA, pcDNA-SNHG17, pcDNA-SNHG17+ si-control, pcDNA-SNHG17+ siRNA-Mst1 groups. a. The expression level of SNHG17 in MPC5 cells was measured by qRT-PCR. b. The protein level of Parkin in MPC5 cells was detected using western blot. MPC5 cells were divided into HG, HG+si-control, HG+siRNA-SNHG17, HG+siRNA-SNHG17+ pcDNA, HG+siRNA-SNHG17+ pcDNA-Mst1 groups. c. The expression level of SNHG17 in MPC5 cells was measured by qRT-PCR. d. The protein level of Parkin in MPC5 cells was detected using western blot. *p < 0.05 vs pcDNA or HG+si-control; #p < 0.05 vs pcDNA-SNHG17+ si-control or HG+siRNA-SNHG17+ pcDNA.
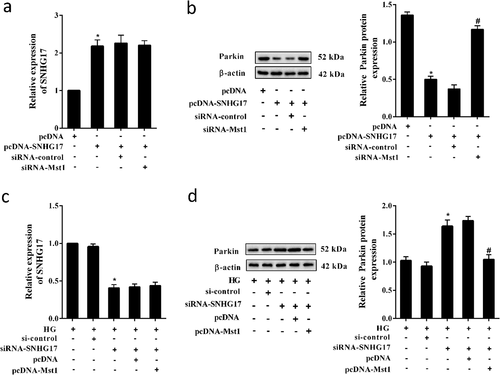
LncRNA SNHG17 regulated mitophagy and apoptosis of podocytes through Mst1
To investigate whether lncRNA SNHG17 regulated mitophagy and apoptosis of podocytes through Mst1, MPC5 cells were transfected with siRNA-SNHG17 and pcDNA-Mst1. We found that siRNA-SNHG17 promotes the formation of autophagic vacuoles, increased LC3II/LC3I ratio ()), and decreased p62 protein level in MPC5 cells and mitochondria of MPC5 cells ()). Also, in response to SNHG17 silencing, the Parkin expression was up-regulated in mitochondria of MPC5 cells ()). Whereas pcDNA-Mst1 abolished the promotion effect of siRNA-SNHG17 on mitophagy (,b)). In addition, siRNA-SNHG17 increased the ATP level, whereas pcDNA-Mst1 abolished the promotion effect of siRNA-SNHG17 on ATP production ()). Moreover, siRNA-SNHG17 reduced the apoptosis of MPC5 cells, whereas pcDNA-Mst1 abolished the inhibition effect of siRNA-SNHG17 on cell apoptosis ()).
Figure 6. SNHG17 regulated mitophagy and apoptosis of podocytes through Mst1. MPC5 cells were divided into HG, HG+si-control, HG+siRNA-SNHG17, HG+siRNA-SNHG17+ pcDNA, HG+siRNA-SNHG17+ pcDNA-Mst1 groups. a. Monodansylcadaverine (MDC) staining was used to evaluate the formation of autophagosomes in MPC5 cells. b. Left: The protein levels of Mfn1, p62, LC3, and Drp-1 were detected in MPC5 cells. Right: The protein levels of Parkin, p62, and LC3 were detected in the mitochondria of MPC5 cells. c. ATP level was detected in MPC5 cells using the ATP Bioluminescence Assay Kit. d. The apoptosis of podocytes was detected using the flow cytometry method. Three replicates were used. *p < 0.05 vs HG+si-control; #p < 0.05 vs HG+siRNA-SNHG17+ pcDNA.
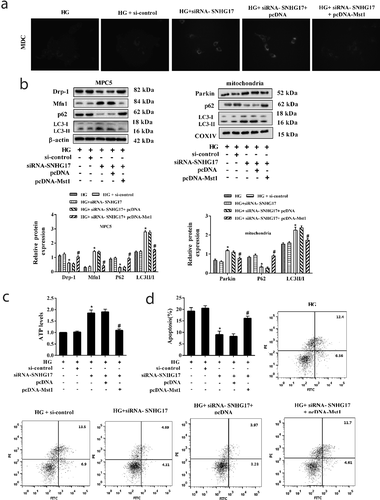
Silencing SNHG17 promoted mitophagy and relieved DN
Finally, we observed the role of silencing SNHG17 in relieving DN via injecting rAAV9-siRNA-SNHG17 into the caudal vein of mice. rAAV9-siRNA-SNHG17 significantly decreased diabetic urine protein and kidney weight (%) ()). LncRNA SNHG17 expression and Mst1 protein level were significantly decreased in the glomeruli of DM mice which were treated with rAAV9-siRNA-SNHG17 (,)). Parkin protein level was up-regulated in glomeruli of DM mice which were treated with rAAV9-siRNA-SNHG17 ()). The levels of autophagy-related proteins (p62 and LC3) in glomeruli of DM mice which were treated with rAAV9-siRNA-SNHG17 indicated rAAV9-siRNA-SNHG17 promoted mitophagy ()). Thus, silencing SNHG17 may promote mitophagy and ameliorate DN. Considering that the BG level wasn’t changed after SNHG17 silencing ()), we considered that the protective effect of SNHG17 silencing on DN is kidney-specific and independent of the benefits of reduced blood glucose.
Figure 7. Silencing SNHG17 promoted mitophagy and relieved diabetic nephropathy (DN). Mice were divided into DM+rAAV9-NC (n = 7) and DM+rAAV9-siRNA-SNHG17 groups (n = 7). 100 μl rAAV9-siRNA-SNHG17 or rAAV9-NC was injected into the caudal vein of mice. Upro and kidney weight were measured at 12 weeks after the injection of rAAV9. After anesthetizing the mice, the renal cortex was isolated. Then, glomeruli were collected. a. BG level, Upro level, and kidney weight (%) were detected in the three groups. b. LncRNA SNHG17 expression in the glomeruli of mice was detected. c. Mst1 and Parkin protein levels in glomeruli were detected. d. The protein levels of Mfn1, p62, LC3, and Drp-1 in glomeruli were detected and the most representative bands were shown. *p < 0.05 vs DM+rAAV9-NC.
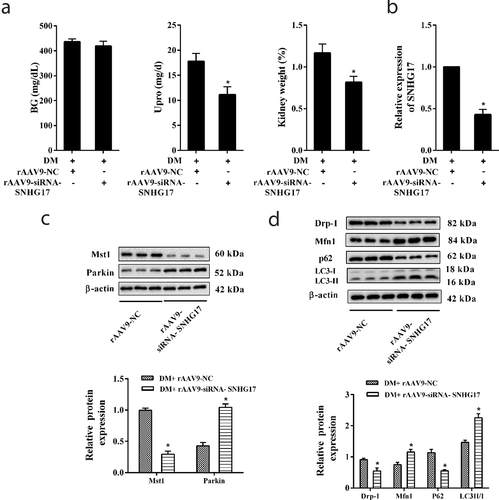
Discussion
Recent studies provided evidence that mitochondrial dysfunction is one of the causes of DN, and accumulations of damaged mitochondria are found in kidney tissues [Citation14,Citation15]. Mitophagy plays a protective role in DN for it can select removal of damaged or dysfunctional mitochondria [Citation16]. Studies indicated that phosphatase and tensin homolog-induced kinase 1 (PINK1), a mitochondrially targeted serine/threonine protein kinase, and Parkin, an E3 ubiquitin protein ligase, are essential mediators in promoting mitophagy [Citation17,Citation18]. PINK1 is upstream of Parkin, and the PINK1-Parkin pathway induces mitophagy in toxin-treated cultured cells in vivo especially when Parkin is highly expressed [Citation19]. Importantly, FOXO1 overexpression promotes mitophagy in podocytes which were treated with high-glucose through inducing the activation of the PINK1-Parkin pathway [Citation20], indicating the PINK1-Parkin pathway plays an important role in promoting mitophagy of podocytes under high-glucose condition. Moreover, it has been reported that mitophagy induced by the PINK1-Parkin pathway blocks the induction of apoptosis [Citation21]. FOXO1 overexpression activated the PINK1-Parkin pathway and prevented the mitophagy-induced apoptosis of podocytes which were treated with high glucose [Citation8]. However, the molecular mechanisms that regulate PINK1-Parkin pathway activation in mitophagy-induced apoptosis of podocytes are not fully discovered.
Mst1, a member of the Mst family, is widely expressed in the mammalian system and can modulate autophagy and apoptosis in diabetes-related diseases [Citation22]. Mst1 knockdown reduces the level of Upro and suppresses podocyte apoptosis-related signaling pathway FAS/FASL, thereby attenuating the injury of DN [Citation23]. In addition, Mst1 knockdown remarkably increases the expression of Parkin, triggers Parkin to the mitochondria, and enhances the mitophagy of cardiomyocytes treated with high glucose [Citation24,Citation25]. These studies provide evidences that Mst1 knockdown may promote the mitophagy of podocytes in DN. In this study, the Mst1 protein level was elevated in glomeruli/podocytes of DM mice and high glucose-treated podocytes. Silencing Mst1 increased protein level of Parkin, whereas Mst1 overexpression obtained the opposite results. In addition, Mst1 overexpression suppressed mitophagy, reduced ATP level and promoted the apoptosis of high glucose-treated podocytes, which were consistent with previous reports [Citation24,Citation25].
Mounting evidences have shown that lncRNAs can regulate the stability of proteins through binding to these proteins [Citation26,Citation27]. Previous reports have shown that lncRNA SNHG17 can directly bind to proteins [Citation11,Citation12]. In addition, Mst1 protein can be stabilized by protein kinase c-Abl through blocking ubiquitin-mediated proteasomal degradation [Citation28]. The ubiquitination and degradation of Mst1 can be promoted by TRAF6 [Citation29]. And Mst1 protein level can be regulated by lncRNA [Citation30]. However, whether the ubiquitination and degradation of Mst1 can be regulated by lncRNA is still not known. In this study, we found lncRNA SNHG17 could bind to Mst1, and positively regulate the Mst1 protein level. Importantly, we found the ubiquitination and degradation of Mst1 were promoted by siRNA-SNHG17, indicating lncRNA SNHG17 reduced the ubiquitination and degradation of Mst1. This is the first study focused on the regulation of lncRNA on the ubiquitination and degradation of Mst1, which will provide directions for the modulation of lncRNAs in Mst1 degradation.
Mitochondria are dynamic organelles that undergo a constant cycle of fission and fusion to maintain their function. Under normal conditions, fission segregates mitochondria for autophagy by the lysosome to remove the damaged organelles (“mitophagy”) [Citation31]. However, under a high glucose condition, overexpressed Mst1 suppressed Parkin-dependent mitophagy [Citation24]. Then, the cumulation of impaired mitochondria activated the mitochondrial apoptotic pathway and eventually leading to cell apoptosis [Citation31]. In the present study, the fusion-related protein Mfn1 was down-regulated while fission-related protein Drp-1 was up-regulated in mitochondria of podocytes in DM mice and HG-treated MPC5 cells, indicating the increased mitochondrial fission during DN. In line with our study, Zhan et al. [Citation32] also found that in the high glucose-treated renal proximal tubular epithelial cell line, mitochondrial fission was increased while mitophagy was suppressed, resulted in the generation of reactive oxygen species and apoptosis. In future work, we will further explore the relationship between mitochondrial dynamics and podocyte apoptosis in DN.
In summary, this study demonstrates that lncRNA SNHG17 knockdown promotes Parkin-dependent mitophagy and reduces apoptosis of podocytes in DN mice and high glucose -treated podocytes through regulating the degradation of Mst1 (), which may provide potential targets for relieving DN.
Disclosure statement
No potential conflict of interest was reported by the authors.
Additional information
Funding
References
- Flyvbjerg A. The role of the complement system in diabetic nephropathy. Nat Rev Nephrol. 2017;13:311.
- Elsherbiny NM, Al-Gayyar MM. The role of IL-18 in type 1 diabetic nephropathy: the problem and future treatment. Cytokine. 2016;81:15–22.
- Modafferi S, Ries M, Calabrese V, et al. Clinical trials on diabetic nephropathy: a cross-sectional analysis. Diabetes Ther. 2019;10(1):229–243.
- Chen C-Y, Wu V-C, Lin C-J, et al. Improvement in mortality and end-stage renal disease in patients with type 2 diabetes after acute kidney injury who are prescribed dipeptidyl peptidase-4 inhibitors. Mayo Clin Proc. 2018;93(12):1760–1774.
- Bose M, Almas S, Prabhakar S. Wnt signaling and podocyte dysfunction in diabetic nephropathy. J Invest Med. 2017;65(8):1093.
- Ming L, Ning J, Ge Y, et al. Excessive apoptosis of podocytes caused by dysregulation of microRNA-182-5p and CD2AP confers to an increased risk of diabetic nephropathy. J Cell Biochem. 2019;120(10):16516–16523.
- Shen H, Ming Y, Xu C, et al. Deregulation of long noncoding RNA (TUG1) contributes to excessive podocytes apoptosis by activating endoplasmic reticulum stress in the development of diabetic nephropathy. J Cell Physiol. 2019;234(9):15123–15133.
- Li W, Wang Q, Du M, et al. Effects of overexpressing FoxO1 on apoptosis in glomeruli of diabetic mice and in podocytes cultured in high glucose medium. Biochem Biophys Res Commun. 2016;478(2):612–617.
- Lin TA, Wu VC, Wang CY. Autophagy in chronic kidney diseases. Cells. 2019;8(1):61.
- Bai X, Geng J, Li X, et al. Long noncoding RNA LINC01619 regulates MicroRNA-27a/forkhead box protein O1 and endoplasmic reticulum stress-mediated podocyte injury in diabetic nephropathy. Antioxid Redox Signal. 2018;29(4):355–376.
- Ma Z, Gu S, Song M, et al. Long non-coding RNA SNHG17 is an unfavourable prognostic factor and promotes cell proliferation by epigenetically silencing P57 in colorectal cancer. Mol Biosyst. 2017;13(11):2350–2361.
- Zhang G, Xu Y, Wang S, et al. LncRNA SNHG17 promotes gastric cancer progression by epigenetically silencing of p15 and p57. J Cell Physiol. 2019;234(4):5163–5174.
- Takemoto M, Asker N, Gerhardt H, et al. A new method for large scale isolation of kidney glomeruli from mice. Am J Pathol. 2002;161(3):799–805.
- Higgins GC, Coughlan MT. Mitochondrial dysfunction and mitophagy: the beginning and end to diabetic nephropathy? Br J Pharmacol. 2014;171(8):1917–1942.
- Livingston MJ, Wang J, Zhou J, et al. Clearance of damaged mitochondria via mitophagy is important to the protective effect of ischemic preconditioning in kidneys. Autophagy. 2019;15(12):2142–2162.
- Sun J, Zhu H, Wang X, et al. CoQ10 ameliorates mitochondrial dysfunction in diabetic nephropathy through mitophagy. J Endocrinol. 2019:445–465. DOI:https://doi.org/10.1530/JOE-18-0578.
- Vincow ES, Merrihew G, Thomas RE, et al. The PINK1-Parkin pathway promotes both mitophagy and selective respiratory chain turnover in vivo. Proc Natl Acad Sci U S A. 2013;110(16):6400–6405.
- Wang X, Winter D, Ashrafi G, et al. PINK1 and Parkin target Miro for phosphorylation and degradation to arrest mitochondrial motility. Cell. 2011;147(4):893–906.
- Jin SM, Youle RJ. PINK1- and Parkin-mediated mitophagy at a glance. J Cell Sci. 2012;125(Pt 4):795–799.
- Li W, Du M, Wang Q, et al. FoxO1 promotes mitophagy in the podocytes of diabetic male mice via the PINK1/Parkin pathway. Endocrinology. 2017;158(7):2155–2167.
- Mariño G, Niso-Santano M, Baehrecke EH, et al. Self-consumption: the interplay of autophagy and apoptosis. Nature Reviews Molecular Cell Biology. 2014;15(2):81–94.
- Zhang M, Zhang L, Hu J, et al. MST1 coordinately regulates autophagy and apoptosis in diabetic cardiomyopathy in mice. Diabetologia. 2016;59(11):2435–2447.
- Wu W, Zhang M, Ou S, et al. Early protective role of MST1 knockdown in response to experimental diabetic nephropathy. Am J Transl Res. 2016;8(3):1397–1411.
- Wang S, Zhao Z, Fan Y, et al. Mst1 inhibits Sirt3 expression and contributes to diabetic cardiomyopathy through inhibiting Parkin-dependent mitophagy. Biochim Biophys Acta Mol Basis Dis. 2019;1865(7):1905–1914.
- Wang S, Zhao Z, Feng X, et al. Melatonin activates Parkin translocation and rescues the impaired mitophagy activity of diabetic cardiomyopathy through Mst1 inhibition. J Cell Mol Med. 2018;22(10):5132–5144.
- Jiang Y, Wu W, Jiao G, et al. LncRNA SNHG1 modulates p38 MAPK pathway through Nedd4 and thus inhibits osteogenic differentiation of bone marrow mesenchymal stem cells. Life Sci. 2019;228:208–214.
- Wang X, Yang J, Guo G, et al. Novel lncRNA-IUR suppresses Bcr-Abl-induced tumorigenesis through regulation of STAT5-CD71 pathway. Mol Cancer. 2019;18(1):84.
- Xiao L, Chen D, Hu P, et al. The c-Abl-MST1 signaling pathway mediates oxidative stress-induced neuronal cell death. J Neurosci. 2011;31(26):9611.
- Li J-A, Kuang T, Pu N, et al. TRAF6 regulates YAP signaling by promoting the ubiquitination and degradation of MST1 in pancreatic cancer. Clin Exp Med. 2019;19(2):211–218.
- Lv B, Zhang L, Miao R, et al. Comprehensive analysis and experimental verification of LINC01314 as a tumor suppressor in hepatoblastoma. Biomed Pharmacother. 2018;98:783–792.
- Rovira-Llopis S, Bañuls C, Diaz-Morales N, et al. Mitochondrial dynamics in type 2 diabetes: pathophysiological implications. Redox Biol. 2017;11:637–645.
- Zhan M, Usman IM, Sun L, et al. Disruption of renal tubular mitochondrial quality control by Myo-Inositol oxygenase in diabetic kidney disease. J Am Soc Nephrol. 2015;26(6):1304–1321.