ABSTRACT
Bone marrow-derived mesenchymal stem cells (BMSCs) are widely used for the treatment of inflammatory and immune diseases, and microRNA-126 (miR-126) is a critical regulator in inflammation as well as immunity. However, the mediating role of miR-126 in BMSCs is still not clear. Thus, this study aimed to preliminarily investigate the effect of miR-126 on proliferation, apoptosis, migration, invasion, differentiation, and its potential regulating pathways in BMSCs. Human BMSCs were obtained and infected with miR-126 overexpression lentivirus, control overexpression lentivirus, miR-126 knock-down lentivirus and control knock-down lentivirus, then cell functions, the PI3 K/AKT pathway and MEK1/ERK1 pathway were evaluated. Subsequently, PI3 K overexpression plasmid and MEK1 overexpression plasmid were transfected into BMSCs with miR-126 knockdown, then the cell functions were assessed as well. BMSCs with miR-126 overexpression displayed elevated proliferation, migration and invasion while decreased apoptosis; however, BMSCs with miR-126 knockdown presented with decreased proliferation, migration, invasion but increased apoptosis. As for differentiation, BMSCs with miR-126 overexpression showed higher levels of CD31, eNOS and VE-cadherin but lower expressions of ALP, OPN and RUNX2, while BMSCs with miR-126 knockdown disclosed the opposite results. Additionally, BMSCs with miR-126 overexpression showed elevated PI3 K, pAKT, MEK1 and pERK1 expressions, while BMSCs with miR-126 knockdown displayed opposite results. Furthermore, PI3 K overexpression and MEK1 overexpression both reversed the effects of miR-126 on cell functions in BMSCs. In conclusion, miR-126 is a genetic regulator in BMSCs via modulating multiple cell functions through the PI3 K/AKT and MEK1/ERK1 signaling pathways.
Introduction
Mesenchymal stem cells (MSCs), a class of stem cells that are available in various tissues with multi-potent to differentiate into multiple cell lineages, are becoming increasingly critical in the investigation of regenerative medicine [Citation1,Citation2]. Bone marrow-derived MSCs (BMSCs) are a crucial type of MSCs, with the potential to differentiate into osteoblasts, chondrocytes and endothelial cells, which establishes the therapeutic role of BMSCs in multiple diseases, for instance, the cartilage damage [Citation3–7]. Furthermore, there have been reports illuminating that BMSCs present with good efficacy in enhancing the recovery of inflammation and immunity-related diseases, which consist of liver fibrosis, brain damage following ischemic stroke, immune-mediated keratitis and so on [Citation8–10].
Rheumatoid arthritis (RA), one of the most common chronic inflammatory joint diseases worldwide, has a prevalence of 0.5% to 1% in general population, bringing about a large disease burden to patients and society worldwide [Citation11]. RA is featured by the involvement of numerous joints with an inflammatory infiltration in synovial tissue, leading to the destroy of cartilage and the near joint bones, which present as pain and swollen joints mostly in hands and feet in patients suffered from RA [Citation12]. Nowadays, the mainstay of RA treatment is the use of disease-modifying antirheumatic drugs (DMARDs) (both biological DMARDs and conventional DMARDs), with the assistance of glucocorticoids, nonsteroidal anti-inflammatory drugs and analgesics as adjunctive medicine, which display acceptable efficacy in ameliorating symptoms, improving physical functions and preventing progressions [Citation13,Citation14]. However, there are still roughly 30% RA patients who cannot achieve a low disease activity after treatment, indicating a need for more novel and efficient therapies for patients [Citation15]. As a potential substitutive therapy for RA, BMSCs are able to regulate immunity and inflammation, and allogenic BMSC transplantation has been demonstrated to be tolerant and efficient in RA patients by a previous study [Citation16].
MicroRNAs (miRNAs) are a category of non-coding RNAs with lengths of about 20 nt, functioning by binding to the 3′ untranslated region of mRNAs to regulate mRNA degradation and translation [Citation17]. MiRNAs have been demonstrated to participate in RA pathogenesis through regulating multiple pathological processes, such as inflammation and cartilage damage, which indicates that miRNAs are a promising class of genetic factors that could serve as assistant biomarkers or treatment targets in RA patients [Citation18–21]. MiR-126 is a miRNA recently reported to correlate with immune- and inflammation-related diseases, such as diabetic mellitus, chronic obstructive pulmonary disease, RA, etc. [Citation22–24]. In our previous studies, we observed that miR-126 dysregulated cell death and growth by modulating PI3 K/AKT signaling pathway in RA fibroblasts like synoviocytes [Citation25]; meanwhile, miR-126 regulated IL-23 R, TNF-α, IFN-γ and exhibited treatment potential in collagen-induced RA rat model [Citation26], furthermore, our preliminary experiments observed that miR-126 regulates cartilage/bone erosion in collagen-induced RA rat model. Based on our previous findings of miR-126 in RA pathogenesis and the therapeutic role of BMSCs in RA, we hypothesized that miR-126 might participate in RA etiology by regulating BMSC cell functions apart from its direct effect on synovium, which might provide more information of miR-126 being treatment target in RA. While the regulatory role of miR-126 in BMSCs needs to be firstly investigated for this purpose.
Thus, this study aimed to investigate the effect of miR-126 on proliferation, apoptosis, migration, invasion and differentiation as well as its potential regulating pathways in BMSCs.
Methods
Cell culture
Human BMSCs were purchased from ScienCell Research Laboratories, Inc. (CA, USA) and cultured in Mesenchymal Stem Cell Medium (ScienCell, USA) with 1% penicillin/streptomycin (Gibco, USA). The 293 T cell was purchased from American Type Culture Collection (ATCC) (VA, USA). All cells were contained in a 37°C, 5% CO2, humidified incubator.
Lentivirus envelope and BMSCs infection
MiR-126 overexpression lentivirus and control overexpression lentivirus were constructed using pLV-CMV-IRES-Puro vector (Hanbio, China), and miR-126 knock-down lentivirus and control knock-down lentivirus were constructed using pLV-U6-RFP-T2A-Puro vector (Hanbio, China). For the generation of overexpression lentivirus, the pLV-CMV-IRES-Puro vector cloned with pre-miR-126 DNA fragment or nonsense DNA fragment was co-transfected into 293 T cells using HilyMax (Dojindo, Japan) with envelope helper vectors. For the generation of knock-down lentivirus, the pLV-U6-RFP-T2A-Puro vector cloned with miR-126 knock-down DNA fragment or nonsense DNA fragment was co-transfected into 293 T cells using HilyMax (Dojindo, Japan) with envelope helper vectors. And the structures of vectors used in our study were presented in Supplementary Figure 1A-C (www.addgene.org; https://www.hanbio.net/cn; http://www.biovector.net/). Then, the culture supernatants containing lentivirus were harvested and ultra-centrifuged. For infection, BMSCs were incubated with lentivirus for 24 hours (hr). Then 2 μg/mL puromycin (Sigma, USA) was added to the culture medium for 7 days for the selection of stably infected BMSCs. The BMSCs infected with miR-126 overexpression lentivirus and the BMSCs infected with control overexpression lentivirus were termed as miR-126(+) BMSCs and NC(+) BMSCs, respectively. And the BMSCs infected with miR-126 knock-down lentivirus and the BMSCs infected with control knock-down lentivirus were termed as miR-126(-) BMSCs and NC(-) BMSCs, accordingly. In addition, each experiment was performed in triplicate.
Cell activities of infected BMSCs
After stably infected BMSCs were constructed, the expression of miR-126 was detected by Reverse Transcription Quantitative-Polymerase Chain Reaction (RT-qPCR). Cell viability was detected by Cell Counting Kit-8 (CCK-8) Assay. Cell apoptosis was detected by Annexin V/Propidium Iodide (AV/PI) Assay. Migration ability and invasive ability were detected by Wound Healing Assay and Transwell Assay.
Endothelial cell (EC) differentiation of infected BMSCs
Stably infected BMSCs were plated in 24-well plates and cultured with Endothelial Cell Growth Medium (Lonza, USA) supplemented with growth supplements which containing 10 ng/ml human fibroblast growth factor (hFGF) (Sigma, USA), 0.2 μg/ml hydrocortisone (Sigma, USA), 1 μg/ml ascorbic acid (Sigma, USA), 20 ng/ml R3-IGF-1 (Sigma, USA), 2% FBS (Gibco, USA) for 7 days. The mRNA and protein expressions of vascular endothelial cell markers including endothelial cell adhesion molecule 1 (CD31), endothelial nitric oxide synthase 3 (eNOS) and vascular endothelial-cadherin (VE-cadherin) were detected by RT-qPCR and Western Blot.
Osteogenic differentiation of infected BMSCs
Stably infected BMSCs were plated in 24-well plates and cultured in osteogenic differentiation culture medium containing 90% DMEM (Gibco, USA), 10% FBS (Gibco, USA), 0.1 nmol/L dexamethasone (Sigma, USA), 0.01 mmol/L b-glycerophosphate (Sigma, USA), 0.05 mmol/L ascorbic acid-2-phosphate (Sigma, USA) for 7 days. The mRNA and protein expressions of osteogenic markers including alkaline phosphatase (ALP), osteopontin (OPN) and runt-related transcription factor 2 (RUNX2) were detected by RT-qPCR and Western Blot.
Pathway detection
It is reported that miR-126 regulates phosphatidylinositol 3-kinase/protein kinase B (PI3 K/AKT) pathway [Citation25] as well as mitogen-activated protein kinase 1/extracellular signal-regulated kinase 1 (MEK1/ERK1) related pathway [Citation27]. Meanwhile, the PI3 K/AKT pathway and MEK1/ERK1 pathway are deeply involved in the BMSC differentiation [Citation25,Citation27]. To investigate the role of PI3 K/AKT pathway and MEK1/ERK1 pathway in BMSC differentiation regulated by miR-126, we detected the relative mRNA and protein expressions of PI3 K and MEK1 using RT-qPCR and Western Blot, and further assessed the protein expressions of AKT, phospho-AKT (pAKT), ERK1, phospho-ERK1 (pERK1) using Western Blot.
Construction and transfection of overexpression plasmid
PI3 K overexpression plasmid, MEK1 overexpression plasmid and control overexpression plasmid were constructed using pEX-2 vector and were transfected into miR-126(-) BMSCs with HilyMax (Dojindo, Japan). The miR-126(-) BMSCs transfected with PI3 K overexpression plasmid and the miR-126(-) transfected with MEK1 overexpression plasmid were named as miR-126(-)&PI3 K and miR-126(-)&MEK1, respectively. The miR-126(-) BMSCs transfected with control overexpression plasmid were named as miR-126(-)&NC(+). The NC(-) BMSCs transfected with control overexpression plasmid were named as NC(+)/(-). The expression of miR-126 was detected by RT-qPCR at 24 hr. The mRNA and protein expressions of PI3 K and MEK1 were detected by RT-qPCR and Western Blot at 24 hr. The protein expressions of AKT, pAKT, ERK1, pERK1 were detected by Western Blot at 24 hr. Cell viability was detected by CCK-8 Assay. Cell apoptosis was detected by AV/PI Assay at 24 hr. Migration ability and invasive ability were detected by Wound Healing Assay and Transwell Assay at 24 hr. After transfection, EC differentiation was induced as described in “EC differentiation of infected BMSCs” subsection above; then, the mRNA and protein expressions of CD31, eNOS and VE-cadherin were detected by RT-qPCR and Western Blot. Meanwhile, osteogenic differentiation was also induced as described in “Osteogenic differentiation of infected BMSCs” subsection above; then, the mRNA and protein expressions of ALP, OPN and RUNX2 were detected by RT-qPCR and Western Blot.
RT-qPCR
After cells were collected, RNA isolation was performed with TRizol Reagent (Invitrogen, USA); then, the total RNA was reversely transcribed into complementary DNA using the QuantiTect Rev. Transcription Kit (Qiagen, German) strictly followed the instructions of the manufacturer. Subsequently, the QuantiNova SYBR Green PCR Kit (Qiagen, German) was used for PCR, and the expression of miR-126 was measured with U6 as internal reference, the mRNA expressions of CD31, eNOS, VE-cadherin, ALP, OPN, RUNX2, PI3 K and MEK1 were calculated using glyceraldehyde-phosphate dehydrogenase (GAPDH) as internal reference. Finally, the results were calculated by the formula 2−ΔΔCt. In addition, primers applied are listed in .
Table 1. Primers applied in RT-qPCR
Western blot
Total protein was extracted with RIPA Buffer (Sigma, USA) and its concentration was evaluated by Pierce™ BCA Protein Assay Kit (Thermo, USA). Afterward, the total protein was transferred to the protein gel NuPAGE™ 4–12% Bis-Tris Protein Gels (Thermo, USA), and subsequently to the polyvinylidene fluoride (PVDF) membrane (Millipore, USA); then, the PVDF membrane was blocked and incubated. Then, the primary antibody was added and incubated at 4°C overnight, after which, the second antibody was added and incubated at 37°C for 1 hr. Finally, the bands were developed by an ECL kit and exposed under an X-ray (Kodak, USA); then, the pictures were taken using E-Gel™ Imager System (Thermo, USA). Besides, the antibodies applied in the Western Blot are listed in .
Table 2. Antibodies applied in Western Blot
CCK-8 assay
At the beginning, 10 μL CCK-8 reagent (Sigma, USA) mixed with 100 μL mesenchymal stem cell medium was added into each plate, which was incubated in 5% CO2 at 37°C for 2 hr. Then the cell proliferation was assessed by reading the optical density (OD) value by the Synergy H1 microplate reader (BioTek, USA).
AV/PI assay
The BMSCs were collected and washed by 1 mL precooled phosphate buffer (PBS) for twice. Then the cells were washed with loading buffer and re-suspended in loading buffer. Afterward, 5 μL AV (R&D, USA) and 5 μL PI (R&D, USA) was added, and cells were incubated in dark for 15 minutes (mins). Then the cell apoptosis rate was calculated by a FACSCanto II flow cytometer (BD, USA), and the data were analyzed by Flowjo 7.0 (Becton, Dickinson and Company, USA).
Wound healing assay
First, BMSCs were seeded in a 24-well plate one day before the experiment. Second, a 10 μL tip was used to scratch 3 lines in each well. Subsequently, a picture of every line in each well was taken with BX41 microscope (Olympus, Japan) at 0 hr and 24 hr.
Transwell assay
After collection, cells were plated in matrigel membrane matrix (BD, USA) coated chamber (Costar, USA). Then the 50 μL serum-free medium was added into each well and incubated at 37°C for 30 mins. Subsequently, 200 μL single-cell suspension containing 1% FBS was added into the upper side of transwell chamber. Afterward, 500 μL culture medium with 10% FBS was added into the lower side of transwell chamber, which was put into an incubator for 24 hr. After which, the transwell chamber was fixed with 4% formaldehyde (Sigma, USA) for 30 mins and washed with PBS for three times. After being dyed with 0.1% crystal violet (Sigma, USA) at room temperature for 20 mins, pictures were taken using a BX41 microscope (Olympus, Japan), and the invasive cells were count.
Tube formation experiment
Matrigel Basement Membrane Matrix (Corning, USA) was used for the assessment of the tube formation potential of BMSCs. Detailed procedures were as follows: first, the melted Matrigel with concentration of 50 μL/cm2 was added to the 96-well plate, which was incubated for 30 min at 37°C. Second, post-induction for 7 days, the 3ⅹ104 trypsinized cells were plated onto Matrigel for 8 h for tube formation. Third, the cells were put under a light microscope with 100 ⅹ magnification, then 4 pictures were taken.
Flow cytometry
To analyze the expression of von Willebrand factor (vWF), flow cytometry was carried out. After being digested with Tyrisin (Thermo, USA) and washed with PBS, the cells were incubated with Rabbit Anti-vWF (Alexa Fluor® 488) (Abcam, USA) in dark with a dilution of 1:500 for 1 h at room temperature. Then the cells were washed and detected with a FACSCanto II flow cytometer (BD, USA). Afterward, the data were analyzed by Flowjo 7.0 (Becton, Dickinson and Company, USA).
Statistical analysis
GraphPad Prism 7.02 software (GraphPad Software Inc., USA) was applied for data analysis and graph construction. Data were expressed as mean and standard deviation (SD). Comparison between two groups was determined by unpaired t-test. Multiple comparisons were determined by analysis of variance (ANOVA) test followed by the Tuke’s multiple comparisons test. P value <0.05 was considered statistically significant.
Results
Effect of miR-126 on BMSCs proliferation, apoptosis, migration and invasion
After transfections, miR-126 was upregulated in miR-126(+) group compared with NC(+) (P < 0.001) group while was downregulated in miR-126(-) group than that in NC(-) group (P < 0.001), indicating that the transfections were successful ()). The cell proliferation of BMSCs was increased in miR-126(+) group than that in NC(+) group (P < 0.05) while was inhibited in miR-126(-) group compared with NC(-) group (P < 0.05) at 72 hr post-transfections ()). As for cell apoptosis rate, it was repressed in miR-126(+) group compared with NC(+) group (P < 0.05) while was elevated in miR-126(-) group than that in NC(-) group (P < 0.05) at 24 hr post-transfections (). In terms of cell migration, the cell migration rate was elevated in miR-126(+) group compared with NC(+) group (P < 0.05) but was reduced in miR-126(-) group compared with NC(-) group (P < 0.05) (). And the invasive cell number was elevated in miR-126(+) group compared with NC(+) group (P < 0.01) while was decreased in miR-126(-) group than that in NC(-) group (P < 0.01) (). These data indicated that miR-126 promoted cell proliferation, migration and invasion while inhibited cell apoptosis of BMSCs.
Figure 1. Effect of miR-126 on BMSCs proliferation and apoptosis. After transfections, the miR-126 expression (a), cell proliferation (b) and cell apoptosis (c, d) in each group of BMSCs. Each experiment was conducted in triplicate. Comparison between two groups was determined by unpaired t-test. P value <0.05 was considered statistically significant. *P < 0.05, ***P < 0.001. MiR-126, microRNA-126; BMSCs, bone marrow-derived mesenchymal stem cells
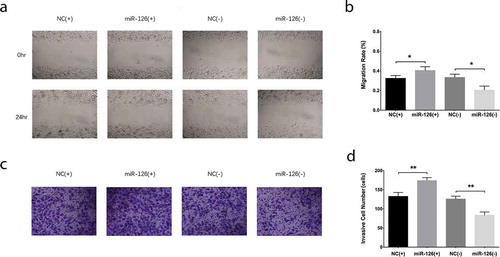
Figure 2. Effect of miR-126 on BMSCs migration and invasion. After transfections, the migration rate (a, b) and invasive cell number (c, d) in each group of BMSCs. Comparison between two groups was determined by unpaired t-test. Each experiment was conducted in triplicate. P value <0.05 was considered statistically significant. *P < 0.05, **P < 0.01. MiR-126, microRNA-126; BMSCs, bone marrow-derived mesenchymal stem cells
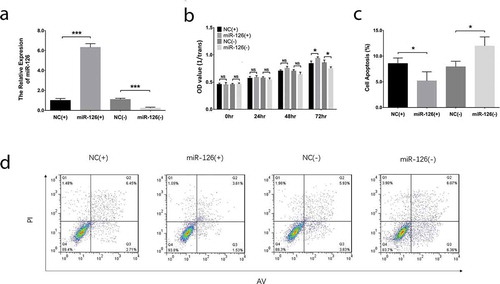
Effect of miR-126 on endothelial cell differentiation and osteogenic differentiation in BMSCs
With regard to the endothelial differentiation makers, the mRNA expressions of CD31 ()), eNOS ()) and VE-cadherin ()) were increased in miR-126(+) group compared with NC(+) group (all P < 0.05) while were downregulated in miR-126(-) group than those in NC(-) group (all P < 0.05). In addition, the protein expressions of CD31, eNOS and VE-cadherin exhibited similar trends as their mRNA expressions did (all P < 0.05) (). As to the markers for osteogenic differentiation, the mRNA expressions of ALP ()), OPN ()) and RUNX2 ()) were decreased in miR-126(+) group than those in NC(+) group (all P < 0.05), while were increased in miR-126(-) group compared with NC(-) group (all P < 0.05). And the protein expressions of ALP, OPN and RUNX2 displayed similar trends as their mRNAs did (all P < 0.05) (). Additionally, tube formation assay revealed that the tube formation ability of BMSCs was better in miR-126(+) group compared to NC(+) group, while it was worse in miR-126(-) group compared with NC(-) group (Supplementary Figure 2A). And the vWF positive cells were increased in miR-126(+) group compared with NC(+) group, while they were decreased in miR-126(-) group than that in NC(-) group (all P < 0.01) (Supplementary Figure 2B, C). These results suggested that miR-126 promoted endothelial cell differentiation while inhibited osteogenic differentiation in BMSCs.
Figure 3. Effect of miR-126 on BMSCs endothelial differentiation. After transfections, the mRNA expressions of CD31 (a), eNOS (b) and VE-cadherin (c), their protein expressions (d), and gray-scale quantification of their protein expressions (e) in each group of BMSCs. Each experiment was conducted in triplicate. Comparison between two groups was determined by unpaired t-test. P value <0.05 was considered statistically significant. *P < 0.05, **P < 0.01. MiR-126, microRNA-126; BMSCs, bone marrow-derived mesenchymal stem cells; CD31, endothelial cell adhesion molecule 1; eNOS, endothelial nitric oxide synthase 3; VE-cadherin, vascular endothelial-cadherin
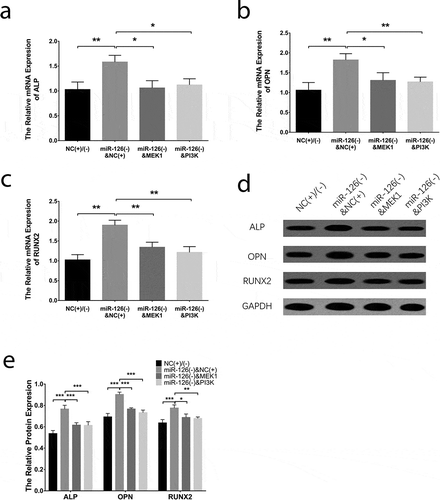
Figure 4. Effect of miR-126 on BMSCs osteogenic differentiation. After transfections, the mRNA expressions of ALP (a), OPN (b) and RUNX2 (c), their protein expressions (d), and the gray-scale quantification of their protein expression (e) in each group of BMSCs. Each experiment was conducted in triplicate. Comparison between two groups was determined by unpaired t-test. P value <0.05 was considered statistically significant. *P < 0.05, **P < 0.01. MiR-126, microRNA-126; BMSCs, bone marrow-derived mesenchymal stem cells; ALP, alkaline phosphatase; OPN, osteopontin; RUNX2, runt-related transcription factor 2
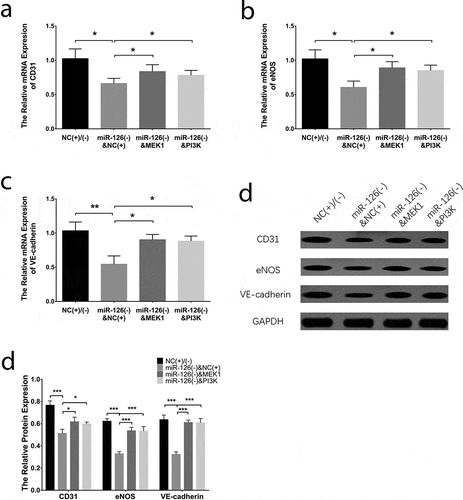
Regulatory effect of miR-126 on PI3K/AKT and MEK1/ERK1 signaling pathways in BMSCs
After transfections, the PI3K mRNA expression was elevated in miR-126(+) group compared with NC(+) group (P < 0.01) while was declined in miR-126(-) group compared with NC(-) group (P < 0.05) ()), and the protein expressions of PI3 K and pAKT were increased in miR-126(+) group compared with NC(+) group while were reduced in miR-126(-) group compared with NC(-) group (all P < 0.05) (). Furthermore, the MEK1 mRNA expression was increased in miR-126(+) group compared with NC(+) group (P < 0.01); however, it was downregulated in miR-126(-) group than that in NC(-) group (P < 0.01) ()). In addition, the MEK1 and pERK1 protein expressions were higher in miR-126(+) group compared with NC(+) group but were downregulated in miR-126(-) compared with NC(-) group (all P < 0.05) (). These data suggested that miR-126 could upregulate PI3 K/AKT and MEK1/ERK1 signaling pathways in BMSCs.
Figure 5. Effect of miR-126 on PI3 K/AKT and MEK1/ERK1 expressions in BMSCs. After transfections, the PI3 K mRNA expression (a), MEK1 mRNA expression (b), the protein expressions of MEK1, ERK1, pERK1, PI3 K, AKT as well as pAKT (c) and the gray-scale quantification of protein expressions (d) in each group of BMSCs. Each experiment was conducted in triplicate. Comparison between two groups was determined by unpaired t-test. P value <0.05 was considered statistically significant. *P < 0.05, **P < 0.01. MiR-126, microRNA-126; PI3 K, phosphatidylinositol 3-kinase; AKT, protein kinase B; MEK1, mitogen-activated protein kinase kinase 1; ERK1, extracellular signal-regulated kinase 1; p-ERK1, phospho-ERK1; p-AKT, phospho-AKT; BMSCs, bone marrow-derived mesenchymal stem cells
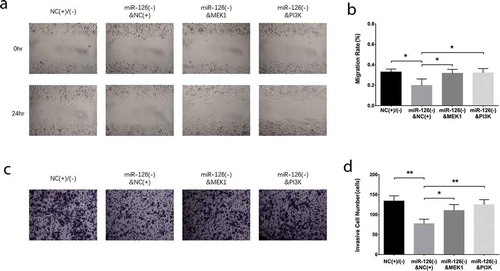
Effect of MEK1 and PI3K on proliferation and apoptosis in miR-126 knockdown BMSCs
After miR-126 knockdown, the MEK1 and PI3 K overexpression plasmids were transfected in BMSCs, and miR-126 was downregulated in miR-126(-)&NC(+) group compared with NC(+)/(-) group (P < 0.01), while it was of no difference between miR-126(-)&NC(+) group and miR-126(-)&MEK1 group (P > 0.05), nor between miR-126(-)&NC(+) group and miR-126(-)&PI3 K group (P > 0.05) ()). MEK1 mRNA expression was inhibited in miR-126(-)&NC(+) group compared with NC(+)/(-) group (P < 0.01), while was increased in miR-126(-)&MEK1 group than that in miR-126(-)&NC(+) group (P < 0.001) ()), as for PI3 K, its mRNA expression was decreased in miR-126(-)&NC(+) group than that in NC(+)/(-) group (P < 0.01), but was increased in miR-126(-)&PI3 K group compared with miR-126(-)&NC(+) group (P < 0.001) ()). As for the protein expressions, MEK1 and PI3 K protein expressions displayed similar trends as their mRNAs did; in addition, pERK1 was inhibited in miR-126(-)&NC(+) group compared with NC(+)/(-) group, and it was increased in miR-126(-)&MEK1 group than that in miR-126(-)&NC(+) group; pAKT protein expression was reduced in miR-126(-)&NC(+) group compared with NC(+)/(-) group, while it was elevated in miR-126(-)&PI3 K group compared with miR-126(-)&NC(+) group (all P < 0.05) (). The cell proliferation rate was reduced in miR-126(-)&NC(+) group than that in NC(+)/(-) group (P < 0.05), while was increased in both miR-126(-)&MEK1 group (P < 0.05) and miR-126(-)&PI3 K group (P < 0.05) compared with miR-126(-)&NC(+) group at 72 hr after transfections ()). As to cell apoptosis, it was increased in miR-126(-)&NC(+) group compared with NC(+)/(-) group (P < 0.05), but was declined in both miR-126(-)&MEK1 group (P < 0.05) and miR-126(-)&MEK1 group (P < 0.05) than that in miR-126(-)&NC(+) group ().
Figure 6. PI3 K and MEK1 reversed the effect of miR-126 on proliferation and apoptosis in BMSCs. The miR-126 expression (a), MEK1 mRNA expression (b), PI3 K mRNA expression (c), protein expressions of MEK1, ERK1, pERK1, PI3 K, AKT and pAKT (d), gray-scale quantification of the protein expression (e), cell proliferation (f) and cell apoptosis (g, h) in BMSCs. Each experiment was conducted in triplicate. Multiple comparison was determined by ANOVA test followed by Tukey’s multiple comparisons test. P value <0.05 was considered statistically significant. *P < 0.05, **P < 0.01. PI3 K, phosphatidylinositol 3-kinase; MEK1, mitogen-activated protein kinase kinase 1; miR-126, microRNA-126; BMSCs, bone marrow-derived mesenchymal stem cells; ERK1, extracellular signal-regulated kinase 1; AKT, protein kinase B; p-ERK1, phospho-ERK1; p-AKT, phospho-AKT; ANOVA, analysis of variance
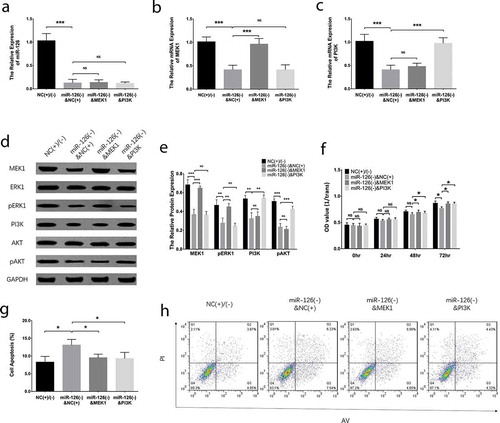
Effect of MEK1 and PI3K on invasion and migration in miR-126 knockdown BMSCs
Post transfections, the cell migration rate of BMSCs was decreased in miR-126(-)&NC(+) group than that in NC(+)/(-) group (P < 0.05), while was elevated in miR-126(-)&MEK1 group (P < 0.05) and miR-126(-)&PI3 K group (P < 0.05) compared with miR-126(-)&NC(+) group (). In terms of cell invasion, the invasive cell number was reduced in miR-126(-)&NC(+) group than that in NC(+)/(-) group (P < 0.01), but was higher in miR-126(-)&MEK1 group (P < 0.05) and miR-126(-)&PI3 K group (P < 0.01) compared with miR-126(-)&NC(+) group ().
Figure 7. PI3 K and MEK1 reversed the effect of miR-126 on migration and invasion in BMSCs. The cell migration rate (a, b) and invasive cell number (c, d) in BMSCs. Each experiment was conducted in triplicate. Multiple comparison was determined by ANOVA test followed by Tukey’s multiple comparisons test. P value <0.05 was considered statistically significant. *P < 0.05, **P < 0.01. PI3 K, phosphatidylinositol 3-kinase; MEK1, mitogen-activated protein kinase kinase 1; miR-126, microRNA-126; BMSCs, bone marrow-derived mesenchymal stem cells; ANOVA, analysis of variance
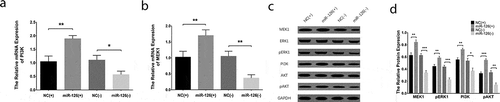
Effect of MEK1 and PI3K on differentiation of miR-126 knockdown BMSCs
With regard to endothelial differentiation markers, the mRNA expressions of CD31 ()), eNOS ()) and VE-cadherin ()) were decreased in miR-126(-)&NC(+) group compared with NC(+)/(-) group (all P < 0.05) while were increased in miR-126(-)&MEK1 group (all P < 0.05) and miR-126(-)&PI3 K group (all P < 0.01) compared to miR-126(-)&NC(+) group. In addition, the protein expressions of CD31, eNOS and VE-cadherin displayed similar trends as the mRNA expressions did (all P < 0.05) (). As to osteogenic differentiation markers, the mRNA expressions of ALP ()), OPN ()) and RUNX2 ()) were increased in miR-126(-)&NC(+) group compared with NC(+)/(-) group (all P < 0.05), while were declined in miR-126(-)&MEK1 group (all P < 0.05) and miR-126(-)&PI3 K group (all P < 0.01) than those in miR-126(-)&NC(+) group. Besides, the ALP, OPN and RUNX2 protein expressions disclosed similar trends as their mRNA expressions did (all P < 0.05) (). In addition, the tube formation ability of BMSCs was worse in miR-126(-)&NC(+) group compared with NC(+)/(-) group, while was increased in miR-126(-)&MEK1 group compared to miR-126(-)&NC(+) group, and in miR-126(-)&PI3 K group compared with miR-126(-)&NC(+) group (Supplementary Figure 3A). As for vWF expression, it was declined in miR-126(-)&NC(-) group than that in NC(+)/(-), while was increased in miR-126(-)&MEK1 group compared to miR-126(-)&NC(+) group, and in miR-126(-)&PI3 K group compared with miR-126(-)&NC(+) group (all P < 0.05) (Supplementary Figure 3B, C). The results in our study all together indicated that miR-126 promoted cell proliferation, migration, invasion and endothelial differentiation while inhibited cell apoptosis and osteogenic differentiation of BMSCs via positively regulating MEK1/ERK1 and PI3 K/AKT signaling pathways.
Figure 8. PI3 K and MEK1 reversed the effect of miR-126 on BMSC endothelial differentiation. The mRNA expressions of CD31 (a), eNOS (b) and VE-cadherin (c) their protein expressions (d), and the gray-scale quantification of their protein expressions (e) in BMSCs. Each experiment was conducted in triplicate. Multiple comparison was determined by ANOVA test followed by Tukey’s multiple comparisons test. P value <0.05 was considered statistically significant. *P < 0.05, **P < 0.01. PI3 K, phosphatidylinositol 3-kinase; MEK1, mitogen-activated protein kinase kinase 1; miR-126, microRNA-126; BMSCs, bone marrow-derived mesenchymal stem cells; CD31, endothelial cell adhesion molecule 1; eNOS, endothelial nitric oxide synthase 3; VE-cadherin, vascular endothelial-cadherin; ANOVA, analysis of variance
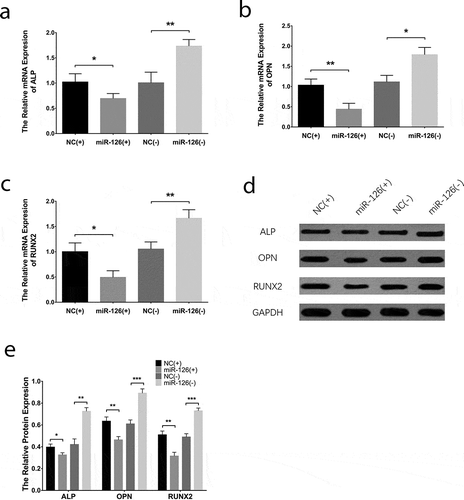
Figure 9. PI3 K and MEK1 reversed the effect of miR-126 on BMSC osteogenic differentiation. The mRNA expressions of ALP (a), OPN (b) and RUNX2 (c), their protein expressions (d) and the gray-scale quantification of their protein expressions (e) in BMSCs. Each experiment was conducted in triplicate. Multiple comparison was determined by ANOVA test followed by Tukey’s multiple comparisons test. P value <0.05 was considered statistically significant. *P < 0.05, **P < 0.01. PI3 K, phosphatidylinositol 3-kinase; MEK1, mitogen-activated protein kinase kinase 1; miR-126, microRNA-126; BMSCs, bone marrow-derived mesenchymal stem cells; ALP, alkaline phosphatase; OPN, osteopontin; RUNX2, runt-related transcription factor 2; ANOVA, analysis of variance
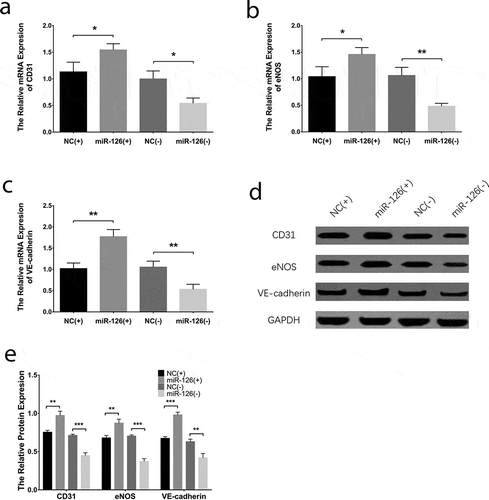
Effect of miR-126 on PIK3R2 in BMSCs
After transfection, the PIK3R2 mRNA expression in BMSCs was of no difference between miR-126(+) group and NC(+) group (P > 0.05), while was upregulated in miR-126(-) group compared to NC(-) group (P < 0.05) (Supplementary Figure 4A). No difference of PIK3R2 protein expression was found between miR-126(+) group compared with NC(+) group (P > 0.05), while it was increased in miR-126(-) group compared to NC(-) group (P < 0.05) (Supplementary Figure 4B, C). Furthermore, rescue experiment was conducted, which disclosed that there was no difference in PIK3R2 mRNA expression between miR-126(-)&MEK1 group and miR-126(-)&NC(-) group, nor between miR-126(-)&PI3 K group and miR-126(-)&NC(-) group (all P > 0.05) (Supplementary Figure 5A). As for the protein expression, the PIK3R2 protein expression displayed similar trends as its mRNA did among groups (all P > 0.05) (Supplementary Figure 5B, C).
Discussion
In the present study, we evaluated the effect of miR-126 on cell functions and its potential regulating signaling pathways in BMSCs, and discovered that: (a) miR-126 advocated cell proliferation, migration and invasion, while inhibited cell apoptosis in BMSCs; (b) miR-126 promoted cell differentiation to endothelial cells but repressed cell differentiation to osteoblasts in BMSCs; (c) miR-126 promoted BMSCs proliferation, migration, invasion and differentiation to endothelial cells while inhibited BMSC apoptosis and differentiation to osteoblasts by positively mediating PI3 K/AKT and MEK1/ERK1 signaling pathways.
As a potential therapeutic approach for RA, BMSCs present with a capability of differentiating into multiple cell lineages and mediating immunity as well as angiogenesis, thus, the studies aiming at investigating the role of BMSCs in inflammation and autoimmunity-related diseases are increasing [Citation28]. For instance, a previous study reveals that BMSC-derived exosomes could protect the ischemia/reperfusion injury by decreasing kidney cell apoptosis through delivering miR-199a-3p [Citation29]. And a recent study illuminates that BMSCs promote the bone marrow regeneration in mice models of dental extraction sockets [Citation30]. Another recent study discovers a novel immunomodulatory mechanism in which the human BMSCs secreted acetylcholine could regulate the lymphocyte proliferation and pro-inflammatory cytokine excretion [Citation31]. And thanks to that the BMSCs are convenient to obtain, cause almost no immunological reactions nor disease transmission, the BMSC transplantation is widely applied in the treatment of several diseases, including several orthopedic diseases. A multi-center prospective randomized control clinical trial demonstrates that in patients with articular cartilage lesions of the knee, autologous BMSC transplantation using microfracture (MFX) achieves increased effect of postoperative cartilage healing and subchondral bone compared with using MFX alone [Citation32]. Furthermore, factors involved in the regulation of BMSC functions are of great concern as well. For instance, a study conducted in rat models reports that the transmembrane protein 18 represses osteogenic differentiation of BMSCs through inactivating β-catenin [Citation33]. Another study illuminates that 5ʹ-hydroxy auraptene provokes the osteoblast differentiation in mouse BMSCs via the mechanism dependent on bone morphogenetic protein [Citation34]. Moreover, recently, accumulating studies have revealed the crucial roles of BMSCs in RA, for instance, a previous study reveals that BMSCs display a check-control effect of regulating inflammation in peripheral blood mononuclear cells obtained from RA patients [Citation35]. And another study reports that the functions of immunomodulation and differentiation in BMSCs are inhibited in an environment of RA lymphocyte activation [Citation36]. In addition, BMSCs also display treatment potential in RA, for example, a former study elucidates that BMSC injection ameliorates RA in rat models regarding the thickness of dis and condylar cartilage, bone trabeculae and regenerated collagen [Citation37]. And a further clinical trial illustrates that using BMSC transplantation in patients with refractory RA decreases Th17 proportion, IL-17A level, DAS28-ESR and VAS score; however, the sample size in this trial is small, indicating that the results should be validated in a larger sample size [Citation38]. Thus, BMSC is a promising alternative therapy for RA patients. Moreover, one of our prior studies found that miR-126 regulated TNF-α and IFN-γ production by fibroblasts-like synoviocytes in collagen-induced RA rat models, and another our study revealed that miR-126 mediated the synovial fibroblast proliferation and resistance to cell apoptosis via regulating PIK3R2 [Citation25,Citation26]. In addition, our preliminary experiment also disclosed that miR-126 could mediate cartilage/bone erosion in collagen-induced RA rat model. Based on these findings, we speculated that miR-126 might also be able to regulate BMSC functions when participating in RA etiology; thus, we conducted this study to firstly investigate the effect of miR-126 on BMSC cell functions. And these investigations might provide a preliminary information for other future studies that are also interested in the role of miR-126 in BMSCs and RA.
In the most recent decade, among all factors involved in the regulation of BMSC functions, miRNAs have become increasingly popular with multiple reports illuminating that miRNAs can modulate various cell functions of BMSCs, such as proliferation, migration and differentiation [Citation39–41]. For instance, miR-30e inhibits osteogenic program in BMSCs via targeting insulin growth factor 2 and it promotes the differentiation to adipogenic and smooth muscle cells [Citation39]. And miR-10b advocates cell migration while represses E-cadherin expression in BMSCs obtained from mice [Citation41]. As for miR-126, to our best knowledge, its effect on BMSC cell functions has not been reported yet, however, there are several studies illuminating that miR-126 is a regulator of cell functions of other stem cells. A previous experiment displays that miR-126 confines cell cycle progression, reduces cell differentiation and elevates self-renewal in primary leukemia stem cells in vivo [Citation42]. Another experiment reveals that obesity diminishes pro-angiogenic function of adipose tissue stem cell-secreted extracellular vesicles via damaging the content of miR-126 in adipose tissue stem cells obtained from obese patients [Citation43]. And a prior experiment elucidates that miR-126 overexpression enhances cell proliferation and colony numbers while represses cell apoptosis in neural stem cells [Citation44]. Besides, a previous study elucidates that MSC secreted exosome deminishes injury induced by hypoxia/reoxygenation in endothelial cells by transferring miR-126 [Citation45]. And a study reports that MSC-derived exosomes attenuate the retinal inflammation in a rat model of diabetic mellitus through modulating miR-126 [Citation46]. These findings all indicate that miR-126 plays a role in regulating stem cell functions. In this study, we investigated the effect of miR-126 on BMSC cell functions and found that miR-126 promoted proliferation, migration and invasion while inhibited apoptosis in BMSCs. And here is a possible explanation for this result: miR-126 might regulate the BMSC proliferation, apoptosis, migration and invasion by interacting with other factors, such as PI3 K/AKT/MTOR signaling pathway, SPRED1 and PLK2, which is similar as its role in regulating cell functions of other stem cells; and in our further experiments, miR-126 was able to positively regulate the PI3 K/AKT and MEK1/ERK1 signaling pathways to promote proliferation, migration, invasion and inhibit apoptosis in BMSCs, which could also provide explanation to this result [Citation42–47]. And these findings in our study indicated that the ways through which miR-126 participates in RA etiology may include the regulation of BMSC cell functions. For instance, miR-126 could enhance the recovery of RA via promoting BMSC proliferation, migration and invasion while repressing BMSC apoptosis, which may enhance the recovery of bone erosion. In addition, we also discovered that miR-126 advocated the BMSC differentiation to endothelial cells while repressed BMSC differentiation to osteoblasts, which could be derived from that miR-126 is found to be a regulator of stem cell differentiation as previously reported in other studies, which elucidate that miR-126 can regulate stem cell differentiation via mediating multiple pathways, for instance, the PI3 K/AKT/MTOR signaling pathway, or by mediating PI3 K/AKT and MEK1/ERK1 signaling pathways as shown in our further experiments [Citation42]. More importantly, the findings in our study that miR-126 regulated cell functions in BMSCs provided preliminary but promising information for future studies, which allows them to explore more detailed mechanisms of the regulatory role of miR-126 on BMSCs in RA in vivo and in vitro. And in view that RA is still a disease with complex pathogenesis, and there is still no cure for this disease, these findings in our study might also provide encouraging information for the exploration of novel and promising therapeutic method for RA patients.
Furthermore, we also discovered that miR-126 might regulate the cell functions of BMSCs through positively regulating the PI3 K/AKT and MEK1/ERK1 signaling pathways in this study. These signaling pathways are related to the regulation of stem cell function, including the BMSCs. Such as, a previous experiment reveals that bone morphogenetic protein 2 (BMP2) regulates beta-Catenin signaling through mediating the PI3 K pathway to regulate the BMP2-induced BMSCs osteogenic differentiation [Citation48]. And another experiment shows that inhibiting PI3 K/AKT signaling pathway contributes to reduced adhesion and migration in BMSCs obtained from non-obese diabetic mice [Citation49]. An experiment also reports that PI3 K/AKT pathway inhibition reverses the hypoxia-induced proliferation and endothelial differentiation in BMSCs from rats [Citation50]. Moreover, blocking MEK/ERK signaling cascade using a selective MEK1/2 inhibitor results in multiple anti-myeloma activities in BMSCs [Citation51]. And an experiment elucidates that mechano-growth factor (MGF) E peptide pretreatment enhances the proliferation and differentiation of BMSCs by mediating MEK-ERK1/2 and PI3 K/AKT signaling pathways under the condition of severe hypoxia [Citation52]. And miR-126 is reported to be able to regulate PI3 K/AKT and MEK1/ERK1 pathways in other animal models or cells as well. For instance, miR-126 could enhance endothelial cell apoptosis via modulating PI3 K/AKT in lower limb arteriosclerosis obliterans rat models [Citation53]. And another experiment also elucidates that miR-126 promotes the cell viability, colony formation and migration of keratinocytes through mediating the PI3 K/AKT signaling pathway [Citation54]. An experiment illuminates that miR-126 induces the VEGF-A/VEGFR-2/ERK signaling pathway activation to mediate the antitumor effects of Tubeimoside-1 in non-small cell lung cancer cells [Citation55]. In addition, another experiment reveals that miR-126 enhances proliferation and invasion by directly targeting the plexin-B2 (PLXNB2) gene in ovarian cancer cell, which subsequently regulates the AKT and ERK1/2 expressions [Citation56]. And a study illuminates that miR-126 treated mice model of hindlimb ischemia markedly elevates the pERK/ERK levels modulated by sprouty-related EVH1 domain-containing protein 1 (SPRED1) [Citation57]. Another study reveals that miR-126 regulates cell functions of glioma cells via stimulating ERK signaling by targeting Kirsten rat sarcoma viral oncogene (KRAS) [Citation58]. These previous findings are partially in line with our study. Furthermore, these results in our study revealed a novel insight in the regulatory role of miR-126 on BMSCs, and due to that BMSCs also play a role in RA pathogenesis, these results may also provide more information on exploring novel and intriguing therapeutic target in RA. In addition, there was a limitation in this study. We did not add an external control of cells with no infection, which should be conducted in the future experiments.
In conclusion, miR-126 is a genetic regulator in BMSCs via modulating multiple cell functions through the PI3 K/AKT and MEK1/ERK1 signaling pathways.
Supplemental Material
Download Zip (4.4 MB)Disclosure statement
No potential conflict of interest was reported by the authors.
Data availability statement
All data generated or analyzed during this study are included in this published article.
Supplementary material
Supplemental data for this article can be accessed here.
References
- Jia Z, Wang S, Liang Y, et al. Combination of kartogenin and transforming growth factor-beta3 supports synovial fluid-derived mesenchymal stem cell-based cartilage regeneration. Am J Transl Res. 2019;11:2056–2069.
- Lubis AMT, Panjaitan T, Hoo C. Autologous mesenchymal stem cell application for cartilage defect in recurrent patellar dislocation: a case report. Int J Surg Case Rep. 2019;55:183–186.
- Gopal K, Amirhamed HA, Kamarul T. Advances of human bone marrow-derived mesenchymal stem cells in the treatment of cartilage defects: a systematic review. Exp Biol Med (Maywood). 2014;239:663–669.
- Zhao R, Li Y, Lin Z, et al. miR-199b-5p modulates BMSC osteogenesis via suppressing GSK-3beta/beta-catenin signaling pathway. Biochem Biophys Res Commun. 2016;477:749–754.
- Mahboudi H, Kazemi B, Soleimani M, et al. Enhanced chondrogenesis of human bone marrow mesenchymal stem cell (BMSC) on nanofiber-based polyethersulfone (PES) scaffold. Gene. 2018;643:98–106.
- Zhao J, Yi C, Zheng Y, et al. Enhancement of fat graft survival by bone marrow-derived mesenchymal stem cell therapy. Plast Reconstr Surg. 2013;132:1149–1157.
- Sun X, Luo LH, Feng L, et al. Down-regulation of lncRNA MEG3 promotes endothelial differentiation of bone marrow derived mesenchymal stem cells in repairing erectile dysfunction. Life Sci. 2018;208:246–252.
- Idriss NK, Sayyed HG, Osama A, et al. Treatment efficiency of different routes of bone marrow-derived mesenchymal stem cell injection in rat liver fibrosis model. Cell Physiol Biochem. 2018;48:2161–2171.
- Jahromi GP, Shabanzadeh AP, Hashtjini MM, et al. Bone marrow-derived mesenchymal stem cell and simvastatin treatment leads to improved functional recovery and modified c-Fos expression levels in the brain following ischemic stroke. Iran J Basic Med Sci. 2018;21:1004–1012.
- Davis AB, Schnabel LV, Gilger BC. Subconjunctival bone marrow-derived mesenchymal stem cell therapy as a novel treatment alternative for equine immune-mediated keratitis: a case series. Vet Ophthalmol. 2019;22:674–682.
- van der Woude D, van der Helm-van Mil AHM. Update on the epidemiology, risk factors, and disease outcomes of rheumatoid arthritis. Best Pract Res Clin Rheumatol. 2018;32:174–187.
- Smolen JS, Aletaha D, McInnes IB. Rheumatoid arthritis. Lancet. 2016;388:2023–2038.
- Ishchenko A, Lories RJ. Safety and efficacy of biological disease-modifying antirheumatic drugs in older rheumatoid arthritis patients: staying the distance. Drugs Aging. 2016;33:387–398.
- Hazlewood GS, Barnabe C, Tomlinson G, et al. Methotrexate monotherapy and methotrexate combination therapy with traditional and biologic disease modifying antirheumatic drugs for rheumatoid arthritis: abridged cochrane systematic review and network meta-analysis. BMJ. 2016;353:i1777.
- Putrik P, Ramiro S, Kvien TK, et al. Inequities in access to biologic and synthetic DMARDs across 46 European countries. Ann Rheum Dis. 2014;73:198–206.
- Liang J, Li X, Zhang H, et al. Allogeneic mesenchymal stem cells transplantation in patients with refractory RA. Clin Rheumatol. 2012;31:157–161.
- Lin S, Gregory RI. MicroRNA biogenesis pathways in cancer. Nat Rev Cancer. 2015;15:321–333.
- Jiang L, Cao S. Role of microRNA-26a in cartilage injury and chondrocyte proliferation and apoptosis in rheumatoid arthritis rats by regulating expression of CTGF. J Cell Physiol. 2020;235:979–992.
- Qiang J, Lv T, Wu Z, et al. Down-regulation of microRNA-142-3p inhibits the aggressive phenotypes of rheumatoid arthritis fibroblast-like synoviocytes through inhibiting nuclear factor-kappaB signaling. Biosci Rep. 2019;39. DOI:https://doi.org/10.1042/BSR20190700.
- Huang Z, Xing S, Liu M, et al. MiR-26a-5p enhances cells proliferation, invasion, and apoptosis resistance of fibroblast-like synoviocytes in rheumatoid arthritis by regulating PTEN/PI3K/AKT pathway. Biosci Rep. 2019;39. DOI:https://doi.org/10.1042/BSR20182192.
- Hernandez-Preciado MR, Moran-Moguel MC, Davalos-Rodriguez IP, et al. miRNA-24 gene sequence, DHFR −829C-T genotypes, and methotrexate response in mexican patients with rheumatoid arthritis. Genet Test Mol Biomarkers. 2019;23:223–227.
- Qu Y, Wu J, Deng JX, et al. MicroRNA-126 affects rheumatoid arthritis synovial fibroblast proliferation and apoptosis by targeting PIK3R2 and regulating PI3K-AKT signal pathway. Oncotarget. 2016;7:74217–74226.
- Paschalaki KE, Zampetaki A, Baker JR, et al. Downregulation of MicroRNA-126 augments DNA damage response in cigarette smokers and patients with chronic obstructive pulmonary disease. Am J Respir Crit Care Med. 2018;197:665–668.
- Zampetaki A, Kiechl S, Drozdov I, et al. Plasma microRNA profiling reveals loss of endothelial miR-126 and other microRNAs in type 2 diabetes. Circ Res. 2010;107:810–817.
- Gao J, Zhou XL, Kong RN, et al. microRNA-126 targeting PIK3R2 promotes rheumatoid arthritis synovial fibro-blasts proliferation and resistance to apoptosis by regulating PI3K/AKT pathway. Exp Mol Pathol. 2016;100:192–198.
- Gao J, Kong R, Zhou X, et al. MiRNA-126 expression inhibits IL-23R mediated TNF-alpha or IFN-gamma production in fibroblast-like synoviocytes in a mice model of collagen-induced rheumatoid arthritis. Apoptosis. 2018;23:607–615.
- Chen JJ, Zhou SH. Mesenchymal stem cells overexpressing MiR-126 enhance ischemic angiogenesis via the AKT/ERK-related pathway. Cardiol J. 2011;18:675–681.
- Lee KW, Kim DH, Lee JH, et al. The effect of pulsatile flow on bMSC-derived endothelial-like cells in a small-sized artificial vessel made by 3-dimensional bioprinting. Stem Cells Int. 2018;2018:7823830.
- Zhu G, Pei L, Lin F, et al. Exosomes from human-bone-marrow-derived mesenchymal stem cells protect against renal ischemia/reperfusion injury via transferring miR-199a-3p. J Cell Physiol. 2019;234:23736–23749.
- Mashimo T, Sato Y, Akita D, et al. Bone marrow-derived mesenchymal stem cells enhance bone marrow regeneration in dental extraction sockets. J Oral Sci. 2019;61:284–293.
- Yi TG, Cho YK, Lee HJ, et al. A novel Immunomodulatory mechanism dependent on acetylcholine secreted by human bone marrow-derived mesenchymal stem cells. Int J Stem Cells. 2019;12:315–330.
- Hashimoto Y, Nishida Y, Takahashi S, et al. Transplantation of autologous bone marrow-derived mesenchymal stem cells under arthroscopic surgery with microfracture versus microfracture alone for articular cartilage lesions in the knee: a multicenter prospective randomized control clinical trial. Regen Ther. 2019;11:106–113.
- Zhang Y, Wang H, Yin T, et al. TMEM18 inhibits osteogenic differentiation of rat bone marrow-derived mesenchymal stem cells by inactivating beta-catenin. Exp Cell Res. 2019:111491. DOI:https://doi.org/10.1016/j.yexcr.2019.07.004.
- Abdallah BM, Ali EM. 5ʹ-hydroxy auraptene stimulates osteoblast differentiation of bone marrow-derived mesenchymal stem cells via a BMP-dependent mechanism. J Biomed Sci. 2019;26:51.
- Lamas JR, Mucientes A, Lajas C, et al. Check-control of inflammation displayed by bone marrow mesenchymal stem cells in rheumatoid arthritis patients. Immunotherapy. 2019;11:1107–1116.
- Lamas JR, Fernandez-Gutierrez B, Mucientes A, et al. RNA sequencing of mesenchymal stem cells reveals a blocking of differentiation and immunomodulatory activities under inflammatory conditions in rheumatoid arthritis patients. Arthritis Res Ther. 2019;21:112.
- El Qashty RMN, Mohamed NN, Radwan LRS, et al. Effect of bone marrow mesenchymal stem cells on healing of temporomandibular joints in rats with induced rheumatoid arthritis. Eur J Oral Sci. 2018;126:272–281.
- Ghoryani M, Shariati-Sarabi Z, Tavakkol-Afshari J, et al. Amelioration of clinical symptoms of patients with refractory rheumatoid arthritis following treatment with autologous bone marrow-derived mesenchymal stem cells: a successful clinical trial in Iran. Biomed Pharmacother. 2019;109:1834–1840.
- Ding W, Li J, Singh J, et al. miR-30e targets IGF2-regulated osteogenesis in bone marrow-derived mesenchymal stem cells, aortic smooth muscle cells, and ApoE-/- mice. Cardiovasc Res. 2015;106:131–142.
- Minayi N, Alizadeh S, Dargahi H, et al. The effect of miR-210 up-regulation on proliferation and survival of mouse bone marrow derived mesenchymal stem cell. Int J Hematol Oncol Stem Cell Res. 2014;8:15–23.
- Zhang F, Jing S, Ren T, et al. MicroRNA-10b promotes the migration of mouse bone marrow-derived mesenchymal stem cells and downregulates the expression of E-cadherin. Mol Med Rep. 2013;8:1084–1088.
- Lechman ER, Gentner B, Ng SW, et al. miR-126 regulates distinct self-renewal outcomes in normal and malignant hematopoietic stem cells. Cancer Cell. 2016;29:214–228.
- Togliatto G, Dentelli P, Gili M, et al. Obesity reduces the pro-angiogenic potential of adipose tissue stem cell-derived extracellular vesicles (EVs) by impairing miR-126 content: impact on clinical applications. Int J Obes (Lond). 2016;40:102–111.
- Zhang Q, Zeng S, Quan C, et al. Induction function of miR-126 in survival and proliferation in neural stem cells. Med Sci Monit. 2015;21:3023–3027.
- Pan Q, Wang Y, Lan Q, et al. Exosomes derived from mesenchymal stem cells ameliorate hypoxia/reoxygenation-injured ECs via transferring MicroRNA-126. Stem Cells Int. 2019;2019:2831756.
- Zhang W, Wang Y, Kong Y. Exosomes derived from mesenchymal stem cells modulate miR-126 to ameliorate hyperglycemia-induced retinal inflammation via targeting HMGB1. Invest Ophthalmol Vis Sci. 2019;60:294–303.
- Pitzler L, Auler M, Probst K, et al. miR-126-3p promotes matrix-dependent perivascular cell attachment, migration and intercellular interaction. Stem Cells. 2016;34:1297–1309.
- Lee JH, Kim BG, Ahn JM, et al. Role of PI3K on the regulation of BMP2-induced beta-catenin activation in human bone marrow stem cells. Bone. 2010;46:1522–1532.
- Li L, Xia Y, Wang Z, et al. Suppression of the PI3K-Akt pathway is involved in the decreased adhesion and migration of bone marrow-derived mesenchymal stem cells from non-obese diabetic mice. Cell Biol Int. 2011;35:961–966.
- Sheng L, Mao X, Yu Q, et al. Effect of the PI3K/AKT signaling pathway on hypoxia-induced proliferation and differentiation of bone marrow-derived mesenchymal stem cells. Exp Ther Med. 2017;13:55–62.
- Kim K, Kong SY, Fulciniti M, et al. Blockade of the MEK/ERK signalling cascade by AS703026, a novel selective MEK1/2 inhibitor, induces pleiotropic anti-myeloma activity in vitro and in vivo. Br J Haematol. 2010;149:537–549.
- Sha Y, Lv Y, Xu Z, et al. MGF E peptide pretreatment improves the proliferation and osteogenic differentiation of BMSCs via MEK-ERK1/2 and PI3K-Akt pathway under severe hypoxia. Life Sci. 2017;189:52–62.
- Li WM, Yue JN, Guo DQ, et al. MiR-126 promotes endothelial cell apoptosis by targeting PI3K/Akt in rats with lower limb arteriosclerosis obliterans. Eur Rev Med Pharmacol Sci. 2019;23:327–333.
- Chang L, Liang J, Xia X, et al. miRNA-126 enhances viability, colony formation, and migration of keratinocytes HaCaT cells by regulating PI3 K/AKT signaling pathway. Cell Biol Int. 2019;43:182–191.
- Shi H, Bi H, Sun X, et al. Antitumor effects of Tubeimoside-1 in NCI-H1299 cells are mediated by microRNA-126-5p-induced inactivation of VEGF-A/VEGFR-2/ERK signaling pathway. Mol Med Rep. 2018;17:4327–4336.
- Xiang G, Cheng Y. MiR-126-3p inhibits ovarian cancer proliferation and invasion via targeting PLXNB2. Reprod Biol. 2018;18:218–224.
- Tsumaru S, Masumoto H, Minakata K, et al. Therapeutic angiogenesis by local sustained release of microRNA-126 using poly lactic-co-glycolic acid nanoparticles in murine hindlimb ischemia. J Vasc Surg. 2018;68:1209–1215.
- Li Y, Li Y, Ge P, et al. MiR-126 regulates the ERK pathway via targeting KRAS to inhibit the glioma cell proliferation and invasion. Mol Neurobiol. 2017;54:137–145.