ABSTRACT
The roles of lncRNA TSLNC8 and its synergetic effects with osimertinib remain unknown in lung cancer. qRT-PCR or western blotting was performed to determine the expression levels of TSLNC8, EGFR and STAT3. Colony formation and MTT assays were used to evaluate cell proliferation. Transwell and wound healing assays were performed to assess migration and invasion abilities. Flow cytometry with Annexin V/PI staining was used to detect changes in cell apoptosis. Nude mice subcutaneous tumor model was constructed and used for validating the effects of TSLNC8 and osimertinib in vivo. Expression of TSLNC8 was down-regulated in clinical lung cancer tissues and cell lines. TSLNC8 overexpression or osimertinib administration led to promotion of apoptosis and inhibition of cell proliferation, migration and invasion, as well as deactivation of the EGFR-STAT3 pathway, whereas TSLNC8 knockdown had opposite effects. Moreover, the above effects of osimertinib were remarkably enhanced by TSLNC8 overexpression and inhibited by TSLNC8 knockdown, respectively. Meanwhile, the effects of TSLNC8 overexpression were reversed by STAT3 activation or EGFR overexpression. In the animal model, combination of TSLNC8 overexpression and osimertinib administration resulted in efficient suppression of tumor growth. In this study, we revealed a TSLNC8-EGFR-STAT3 signaling axis in lung cancer, and TSLNC8 overexpression significantly enhanced the anti-tumor effects of osimertinib via inhibiting EGFR-STAT3 signaling.
Introduction
Lung cancer represents the most prevalent cause of malignant tumor-related death worldwide [Citation1,Citation2]. Small-cell lung cancer (SCLC) and non-small cell lung cancer (NSCLC) are the most common types of lung cancer, with the latter accounting for 80–85% of all lung cancer cases [Citation3]. Regardless of the recent advances in diagnosis, chemotherapy, radiotherapy and targeted molecular therapy, the prognosis of lung cancer remains disappointing, and the 5-year survival rate is less than 15% [Citation4]. The lung cancer-related death mainly resulted from tumor migration, invasion and recurrence [Citation5]. To date, the specific underlying molecular mechanisms of these processes remain poorly understood. Dysregulation of long non-coding RNAs (lncRNAs) is frequently implicated in diverse cancer types, and shown to play critical regulatory roles in growth and metastasis of tumors [Citation6,Citation7]. In particular, multiple studies have unveiled the critical functions of lncRNAs in tumorigenesis and progression of lung cancer [Citation1,Citation8–10]. Nevertheless, the specific roles of many lncRNAs in lung cancer remain elusive, and further exploration is needed.
LncRNAs, which are non-coding transcripts longer than 200 nucleotides [Citation11,Citation12], have been demonstrated to regulate various stages of gene expression, including transcriptional, post-transcriptional and epigenetic levels [Citation13,Citation14]. LncRNAs have been implicated in various cellular processes, including differentiation, inflammation and cellular development [Citation15–19]. TSLNC8, a lncRNA on chromosome 8p12, has been identified as a tumor suppressor gene significantly down-regulated in tumor cells and tissues, including hepatocellular carcinoma and glioma [Citation20,Citation21]. Moreover, TSLNC8 has been shown to inactivate the IL-6/STAT3 signaling pathway via suppression of phosphorylation and transcriptional activity of STAT3 in hepatocellular carcinoma [Citation20]. TSLNC8 has also been reported to inhibit tumor metastasis and promote apoptosis by regulating IL-6/STAT3/HIF-1α signaling pathway in non-small cell lung cancer [Citation22]. However, the expression level of TSLNC8 and its function in lung cancer need further exploration. Meanwhile, STAT3 has been established as an important downstream effector of the epidermal growth factor receptor (EGFR), and is shown to be phosphorylated upon activation by EGFR, followed by translocation into the nucleus to achieve transcriptional regulation [Citation23]. To date, dysregulated EGFR-STAT3 signaling has been implicated in various cancers, including lung cancer [Citation23–25]. However, the interaction between TSLNC8 and EGFR-STAT3 signaling has never been reported in lung cancer.
Osimertinib, also known as AZD9291, is a third-generation irreversible epidermal growth factor receptor tyrosine kinase inhibitor (EGFR-TKI) developed by AstraZeneca [Citation26,Citation27]. Osimertinib exerts its inhibitory effect by forming a covalent bond with the cysteine 797 residue in the ATP binding site of EGFR kinase [Citation28,Citation29]. It was approved for the treatment of NSCLC patients carrying a specific mutation in EGFR (T790M) and who responded poorly to previous generation of EGFR-TKIs, such as afatinib and gefitinib [Citation26,Citation27]. As evidenced by numerous clinical studies, osimertinib exhibited excellent efficacy for NSCLC by exclusively targeting T790M mutant EGFR while sparing wild-type EGFR [Citation30,Citation31]. In addition, most NSCLC patients with the T790M mutant EGFR eventually developed resistance to osimertinib approximately 10 months after treatment, posing substantial risk of relapse [Citation32]. Therefore, the current chemotherapy scheme with osimertinib needs to be further improved to prevent resistance-induced relapse as well as to minimize the application dosage in lung cancer.
In this work, we performed studies both in vitro and in vivo to assess the inhibitory effects of TSLNC8 overexpression on the EGFR-STAT3 signaling pathway, as well as on cell proliferation, migration, invasion and apoptosis properties in lung cancer. We observed that TSLNC8 overexpression markedly enhanced the anti-tumor effects of osimertinib via suppressing the EGFR-STAT3 signaling pathway. Our results provided helpful information on the potential combination therapy using TSLNC8 and osimertinib in chemo-resistant lung cancer. Our findings also provided the possibility of decreasing the application dosage of osimertinib, thereby minimizing its side effects in lung cancer patients.
Materials and methods
Clinical sample collection
Thirty-one pairs of clinical lung cancer tissues and adjacent normal lung tissues were collected lung cancer patients from the First Affiliated Hospital of Henan University of Traditional Chinese Medicine (Zhengzhou, Henan, China). The information of patients was shown in . The use of these tissues was approved by the Ethics Committee of the First Affiliated Hospital of Henan University of Traditional Chinese Medicine, and each patient submitted a written informed consent. The collected tissues were rinsed with sterile phosphate-buffered saline (PBS), frozen with liquid nitrogen, followed by storage at −80°C until use.
Table 1. The correlation between TSLNC8 expression and clinicopathological characteristics in lung cancer patients
Cell culture
Human lung cancer cell lines (H358, H460, H1975, H1299, H1395, H1650 and A549) and normal human embryonic lung fibroblast (MRC-5) were purchased from the Chinese Academy of Sciences (Shanghai, China). The cells were cultured in Roswell Park Memorial Institute (RPMI) 1640 medium (Gibco) at 37°C in a humidified incubator with 5% CO2. 100 μg/mL streptomycin, 100 U/mL penicillin (Beyotime) and 10% fetal bovine serum (Gibco) were added to the medium.
Cell transfection
H1975 and H358 cells seed into 24 well plates (1 × 105/well) were transfected with pIRES2 vector containing TSLNC8 or shRNA targeting TSLNC8 (shTSLNC8) (each plasmid: 1 μg/well) to modulate the expression of TSLNC8 with Lipofectamine 2000 (Invitrogen, Carlsbad, USA) according to the manufacturer’s instructions. shRNA targeting EGFR (shEGFR), EGFR overexpression vector or their scrambled negative controls (each plasmid: 1 μg/well) were also transfected with Lipofectamine 2000. Additionally, the cells were stably transfected with lentiviral vector for overexpression of TSLNC8 (pWPXL-TSLNC8) to perform the tumor xenograft experiments. The overexpression vectors, shRNAs and lentiviral vectors used in this work were designed and purchased from GenePharma (Shanghai, China). The cells were used for the indicated experiments 48 h after transfection.
Cell viability assay
Cell Proliferation Kit I (MTT, Roche Biotechnology) was used according to the manufacturer’s instructions. H1975 cells transfected with shRNA or overexpression vector were seeded in each well of a 96-well plate with 200 μL culture medium, followed by incubation for 24 h, 48 h or 72 h. To determine the cytotoxicity of osimertinib, the initial medium of H1975 or H358 cells was discarded and replaced with 100 μL medium containing a concentration gradient of osimertinib (1, 2, 4, 6, 8 μM) followed by incubation for 24, 48 or 72 h. Next, 10 µL MTT labeling reagent was added to each well, followed by incubation for 4 h. Then, 100 µL solubilization reagent was added followed by overnight incubation at 37°C. Finally, absorbance at a wavelength of 490 nm was measured with a microplate reader (Bio-Rad, USA).
Colony formation assay
H1975 and H358 cells were trypsinized at 80–90% confluence, pelleted, resuspended and counted. Next, the cells with indicated transfection were seeded at a density of 500 cells/well in 6-well plates followed by incubation for two weeks. Then, the cells were rinsed with PBS, fixed with 100% methanol, stained with 1% crystal violet (Sigma Aldrich) and counted.
In vitro wound healing assay
H1975 and H358 cells transfected with shRNA or overexpression vector were cultured in 6-well plates at a concentration of 1 × 106 cells/well for 24 h in a humidified chamber. After formation of a confluent monolayer, the monolayer was wounded by scratching with a 200 µL pipette tip, and washed with PBS to remove cell debris. An inverted light microscope (Nikon Eclipse, TS100) with a digital camera was used to record images at 0 h and 24 h.
Transwell assay
Transwell invasion assay was conducted in Boyden chambers (Corning, NY) carrying membranes (8 μm pore) coated with Matrigel. H1975 and H358 cells transfected with shRNA or overexpression vector were seeded into the upper chamber with serum-free medium, while the lower chamber was filled with 1640 medium supplemented by 10% fetal bovine serum. After incubation for 24 h, the cells remaining on the upper surface of the filter were erased with cotton swabs. Cells that invaded through the filter to attach on the lower surface were fixed with methanol and stained with 1% crystal violet for 10 min. Next, the filters were washed with PBS and observed under a light microscope (Nikon Eclipse, TS100).
Cell apoptosis detection by flow cytometry
H1975 and H358 cells transfected with shRNA or overexpression vector were stained with Annexin V-FITC apoptosis detection kit (Sigma Aldrich) following the manufacturer’s instruction. The cells were rinsed twice with PBS buffer and then resuspended in binding buffer (100 μL) containing 5 μL Annexin V-FITC. Next, the samples were stained with 5 μL propidium iodide for 10 min in the dark, and then 400 μL binding buffer was added. The resulting mixture was analyzed with FACSCalibur (BD Biosciences, Germany).
Luciferase reporter assay
Luciferase reporter vector for EGFR was constructed with pGL3-basic vector (Promega, WI, USA). H1975 cells were co-transfected with the luciferase reporter vector and pIRES2-TSLNC8, shTSLNC8 or negative controls. The cells were lysed 48 h after transfection. Luciferase activities were measured with the Dual-Luciferase Reporter Assay System (Promega, WI, USA) following the manufacturer’s instructions.
Animal model construction
Six-week-old male BALB/c nude (nu/nu) mice were purchased from Shanghai SLAC Laboratory Animal Center (Shanghai, China). Mice were bred and acclimated under specific pathogen-free condition. All of the animal studies were conducted in accordance with the Institutional Animal Care and Use Committee of the First Affiliated Hospital of Henan University of Traditional Chinese Medicine. H1975 cells were stably transfected with pWPXL-TSLNC8 or pWPXL-vector, followed by subcutaneous injection into nude mice. The tumors were allowed to grow to a volume of 50 mm3. Next, osimertinib (5 mg/kg/day) was administered intragastrically over 2 weeks. Tumor volume was measured with caliper every 5 days until 30 days to plot the tumor growth curve. The tumors were collected for further examinations.
Immunohistochemistry assay
Immunohistochemistry assay was used on the paraffin-embedded tumor tissues fixed by 10% formaldehyde (ZSGB-BIO, Beijing, China). The primary antibodies used were EGFR (#4267) and p-STAT3 (Tyr705, #9145) antibodies (Cell signaling technology, Denver, MA, USA) at a dilution of 1:200. After incubation with the secondary antibody (anti-mouse biotinylated, Dako) at a dilution of 1:1000, the avidin-biotin peroxidase method was used to determine the location and expression level of the target proteins. At last, the visualization signals were developed with 3,3′-diaminobenzidine tetrahydrochloride, and the tissues were counterstained in hematoxylin.
Protein extraction and western blotting assay
Lung cancer tissues and cells were lysed using cold lysis buffer (RIPA buffer, Thermo Fisher Scientific), and quantification of protein concentration was performed with a BCA protein assay kit (Beyotime). Then, equal amounts of proteins from each group were purified with 10% SDS-PAGE, and then transferred to polyvinylidene difluoride (PVDF) membrane. 5% skim milk was used for blocking of nonspecific binding by incubation for 1 h. Subsequently, the membrane was incubated with primary antibodies overnight at 4°C. GAPDH was used as an internal control. The membrane was incubated with horseradish peroxidase-conjugated secondary antibody (#7074) at a dilution of 1:2000 for 1 h. The bands were detected with the ECL reagent (Millipore Corp.). The proteins were quantified with Quantity One software (Bio-Rad Laboratories, Inc., Hercules, CA, USA). Antibodies against p-STAT3 (Tyr705, #9145, 1:1000), STAT3 (#30,835, 1:1500), EGFR (#4267, 1:2000), p-EGFR (Tyr1068, #3777, 1:1000) and GAPDH (#5174, 1:2000) were purchased from Cell Signaling Technology (Boston, USA).
RNA extraction and qRT-PCR assay
Total RNA was extracted from the tissues and cells with the Trizol reagent (Invitrogen) following the manufacturer’s instructions. cDNA was synthesized with Prime-Script RT-PCR master mix (Takara). TSLNC8 expression level was detected by qRT-PCR with SYBR Green Premix Ex Taq (Takara) following the manufacturer’s instructions. Primers for TSLNC8 were purchased from Sangon Biotech Co., Ltd (Shanghai, China). GAPDH was used as internal reference, and relative expression level was calculated using the 2−ΔΔCt method.
Statistical analysis
All experiments were repeated at least three times, and one representative result was presented. Statistical analysis was carried out using SPSS 16.0 (SPSS Inc., Chicago, USA). Student’s t-test was used to evaluate differences between two groups. One-way analysis of variance (ANOVA) and Tukey’s post hoc test were performed to evaluate differences among multiple groups. The correlation between TSLNC8 expression and clinicopathological characteristics of lung cancer patients was assessed by the Chi-squared test. P values smaller than 0.05 were considered statistically significant.
Results
Expression levels of lncRNA TSLNC8, EGFR and STAT3 in lung cancer tissues
Firstly, we determined the expression levels of lncRNA TSLNC8, EGFR and STAT3 in lung cancer tissues. We collected 31 pairs of clinical lung cancer tissues and the adjacent normal lung tissues, and assessed the expression of lncRNA TSLNC8 in these samples using qRT-PCR ()). The results revealed significantly lower expression level of TSLNC8 in lung cancer tissues in comparison with normal lung tissues. Also, as shown in , low TSLNC8 expression levels were associated with gender (p < 0.05), lymph node metastasis (p < 0.05) and TNM stage (p < 0.05). We further assessed the expression levels of EGFR and STAT3 in lung cancer tissues by western blotting, and discovered higher expression level of total EGFR and the increased phosphorylation level of STAT3 (Tyr705) (-c)). Therefore, downregulation of TSLNC8 appeared to be linked to activation of the EGFR-STAT3 pathway in clinical lung cancer tissues, suggesting that negative correlation existed in the expression pattern of lncRNA TSLNC8, EGFR and STAT3 in lung cancer tissues.
Figure 1. Expression levels of lncRNA TSLNC8, EGFR and STAT3 in lung cancer tissues. (a). qRT-PCR was used to detect expression of TSLNC8 in clinical lung cancer tissues (n = 31) and normal lung tissues (n = 31). (b). Western blotting was performed to detect expression of EGFR and phosphorylation of STAT3 (Tyr705) in clinical lung cancer tissues (n = 31) and in normal lung tissues (n = 31). GAPDH was used as a loading control. (c). Quantitative analysis of protein levels in B. Data were shown as mean±SD, the result was a representative of three independent experiments. ** p< 0.01 and * p< 0.05
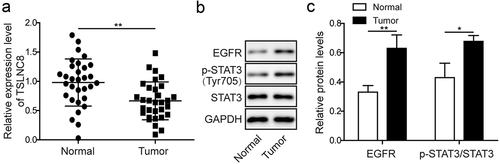
Expression levels of lncRNA TSLNC8, EGFR and STAT3 in lung cancer cell lines
Having determined the expression levels of TSLNC8, EGFR and STAT3 in clinical tumor tissues, we next validated the results at the cellular level using normal human embryonic lung fibroblast (MRC-5) and a collection of human lung cancer cell lines (H358, H460, H1975, H1299, H1395, H1650 and A549). As demonstrated by qRT-PCR, TSLNC8 expression was significantly down-regulated in all the lung cancer cell lines in comparison with MRC-5 ()). Based on western blotting results, the lung cancer cell lines exhibited significantly higher expression level of EGFR and higher phosphorylation level of STAT3 (Tyr705) in comparison with MRC-5 (-c)). These results were consistent with the findings in clinical tumor tissues ().
Figure 2. Expression levels of lncRNA TSLNC8, EGFR and STAT3 in lung cancer cell lines. (a). qRT-PCR was used to detect expression of TSLNC8 in human lung cancer cell lines (H358, H460, H1975, H1299, H1395, H1650 and A549) and normal human embryonic lung fibroblast cell line (MRC-5). (b). Western blotting was performed to assess expression of EGFR and phosphorylation of STAT3 (Tyr705) in human lung cancer cell lines and a normal human embryonic lung fibroblast cell line. GAPDH was used as a loading control. (c). Quantitative analysis of protein levels in B. Data were shown as mean±SD, the result was a representative of three independent experiments. *** p< 0.001, ** p< 0.01 and * p< 0.05
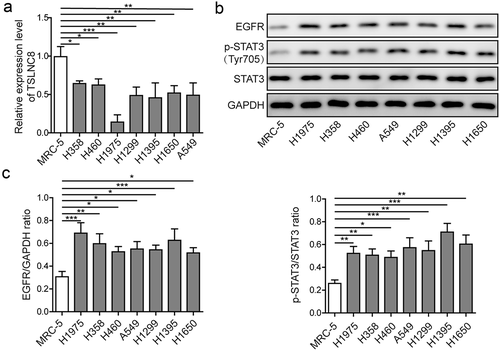
TSLNC8 enhances the effects of osimertinib on proliferation, apoptosis, migration and invasion of lung cancer cells via inhibiting the EGFR-STAT3 pathway
H1975 cells exhibited the lowest expression level of TSLNC8 ()), and was thus selected as the representative cell line for further investigations. MTT assay revealed that osimertinib inhibited the proliferation of H1975 cells in a time and concentration-dependent manner ()). To evaluate the effects of TSLNC8 on lung cancer cell proliferation and tumorigenesis, cells for overexpressing TSLNC8 were established by transfecting H1975 cells with pIRES2-TSLNC8 vector. Overexpression of TSLNC8 in the H1975 cells was confirmed by qRT-PCR ()). Next, colony formation assay () and flow cytometry with Annexin V/PI staining () demonstrated that both TSLNC8 overexpression and osimertinib administration (2 μM) led to enhancement of apoptosis and inhibition of cell proliferation. Meanwhile, transwell assay () and wound healing assay () demonstrated that both TSLNC8 overexpression and osimertinib administration inhibited migration and invasion abilities. Importantly, the above effects of osimertinib were remarkably enhanced by TSLNC8 overexpression (). Moreover, both TSLNC8 overexpression and osimertinib administration were found to decrease the levels of EGFR, p-EGFR (Tyr1068) and p-STAT3 (Tyr705), indicating deactivation of the EFGR-STAT3 signaling pathway (). Moreover, the inhibitory effects of osimertinib on expression levels of EGFR, p-EGFR (Tyr1068) and p-STAT3 (Tyr705) were enhanced by TSLNC8 overexpression (). These results indicated that TSLNC8 might enhance the effects of osimertinib on cell proliferation, migration, invasion and apoptosis via inhibiting the EGFR-STAT3 pathway.
Figure 3. TSLNC8 enhances the effects of osimertinib on cell proliferation, apoptosis, migration and invasion via inhibiting the EGFR-STAT3 pathway. (a). MTT assay was used to evaluate the inhibitory effects of osimertinib on proliferation of H1975 cells. (b). Overexpression of TSLNC8 in the resulting cells was confirmed by qRT-PCR. (c-d). Colony formation assay was performed to evaluate the effects of TSLNC8 overexpression and/or osimertinib administration on proliferation of lung cancer cell line H1975. (e-f). Flow cytometry with Annexin V/PI staining was used to assess the effects of TSLNC8 overexpression and/or osimertinib administration on apoptosis of lung cancer cell line H1975. (g-h). Transwell assay was used to determine the effects of TSLNC8 overexpression and/or osimertinib administration on invasion ability of lung cancer cell line H1975. I-J. In vitro wound healing assay was performed to determine the effects of TSLNC8 overexpression and/or osimertinib administration on migration ability of lung cancer cell line H1975. (k). Western blotting was performed to assess expression of EGFR and phosphorylation of EGFR (Tyr1068) and STAT3 (Tyr705) in lung cancer cell line H1975 in response to TSLNC8 overexpression and/or osimertinib administration. Data were shown as mean±SD, the result was a representative of three independent experiments. *** p< 0.001, ** p< 0.01 and * p< 0.05
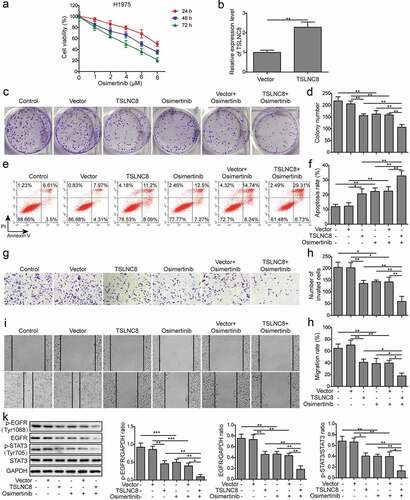
TSLNC8 knockdown suppresses the effects of osimertinib on proliferation, apoptosis, migration and invasion of lung cancer cells
H358 cells exhibited the highest expression level of TSLNC8 ()), H358 cells were thus transfected with shTSLNC8 to investigate the roles of TSLNC8 knockdown along with osimertinib on proliferation, apoptosis, migration and invasion abilities of lung cancer cells. Osimertinib also inhibited the proliferation of H358 cells in a time and concentration-dependent manner, and H358 cells were more sensitive to osimertinib treatment compared with H1975 cells ()). As shown in ), qRT-PCR data indicated that TSLNC8 expression was silenced in H358 cells. Next, colony formation assay showed that TSLNC8 knockdown alone enhanced proliferation of H358 cells, and TSLNC8 knockdown along with osimertinib led to enhance cell proliferation compared with only osimertinib treatment (). Flow cytometry demonstrated that TSLNC8 knockdown suppressed apoptosis of H358 cells, and TSLNC8 knockdown along with osimertinib resulted in decreased cell apoptosis compared with only osimertinib treatment (). Consistently, transwell assay () and wound healing assay () showed that TSLNC8 knockdown alone promoted migration and invasion abilities of H358 cells, and TSLNC8 knockdown reversed the effects of osimertinib on cell migration and invasion abilities in comparison to only osimertinib treatment. Collectively, these results suggested that TSLNC8 knockdown suppressed the effects of osimertinib on proliferation, apoptosis, migration and invasion of lung cancer cells.
Figure 4. TSLNC8 knockdown suppresses the effects of osimertinib on proliferation, apoptosis, migration and invasion of lung cancer cells. (a). MTT assay was used to evaluate the inhibitory effects of osimertinib on proliferation of H358 cells. (b). Knockdown of TSLNC8 in the resulting cells was confirmed by qRT-PCR. (c-d). Colony formation assay was performed to evaluate the effects of TSLNC8 knockdown and/or osimertinib administration on proliferation of lung cancer cell line H358. (e-f). Flow cytometry with Annexin V/PI staining was used to assess the effects of TSLNC8 knockdown and/or osimertinib administration on apoptosis of lung cancer cell line H358. (g-h). Transwell assay was used to determine the effects of TSLNC8 knockdown and/or osimertinib administration on invasion ability of lung cancer cell line H358. I-J. In vitro wound healing assay was performed to determine the effects of TSLNC8 knockdown and/or osimertinib administration on migration ability of lung cancer cell line H358. Data were shown as mean±SD, the result was a representative of three independent experiments. *** p< 0.001, ** p< 0.01 and * p< 0.05
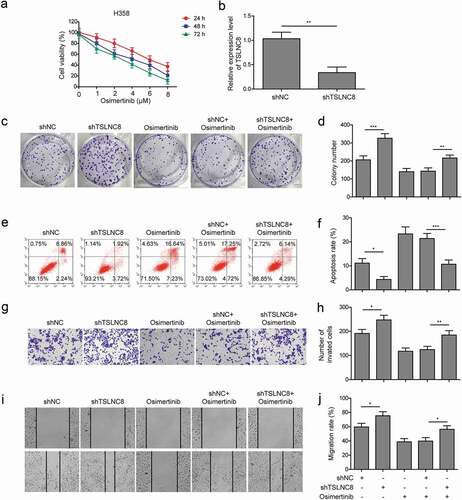
Interaction between TSLNC8 and EGFR and EGFR knockdown inhibits proliferation, migration, invasion and promotes apoptosis of lung cancer cells
Next, we performed further experiments to reveal the molecular mechanisms through which TSLNC8 exerted its inhibitory effects on lung cancer cell proliferation and tumorigenesis. Luciferase reporter assay showed that overexpression of TSLNC8 resulted in the decreased luciferase activity for EGFR, while TSLNC8 knockdown led to the enhanced luciferase activity for EGFR, indicating that TSLNC8 interacted with EGFR ()). Western blotting results validated knockdown of EGFR expression with shEGFR and the consequent reduced phosphorylation of STAT3 (Tyr705) ()). Subsequently, we performed MTT assay (), flow cytometry of cell apoptosis assay (), transwell assay () and wound healing assay () to demonstrate that EGFR knockdown effectively inhibited cell proliferation, migration and invasion, while enhanced cell apoptosis. These findings suggested that TSLNC8 inhibited cancer cell proliferation, migration and invasion by down-regulating the expression of EGFR.
Figure 5. Interaction between TSLNC8 and EGFR and EGFR knockdown inhibits cell proliferation, migration, invasion and promotes apoptosis. (a). Luciferase reporter assay was performed to validate interaction between TSLNC8 and EGFR. (b). Western blotting was performed to assess expression of EGFR and phosphorylation of STAT3 (Tyr705) in lung cancer cell line H1975 in response to EGFR knockdown. GAPDH was used as a loading control. (c). MTT assay was performed to evaluate the effect of EGFR knockdown on proliferation of lung cancer cell line H1975. (d-e). Flow cytometry with Annexin V/PI staining was used to assess the effect of EGFR knockdown on apoptosis of lung cancer cell line H1975. (f-g). Transwell assay was used to determine the effect of EGFR knockdown on invasion ability of lung cancer cell line H1975. (h-i). In vitro wound healing assay was performed to evaluate the effect of EGFR knockdown on migration ability of lung cancer cell line H1975. Data were shown as mean±SD, the result was a representative of three independent experiments. ** p< 0.01 and * p< 0.05
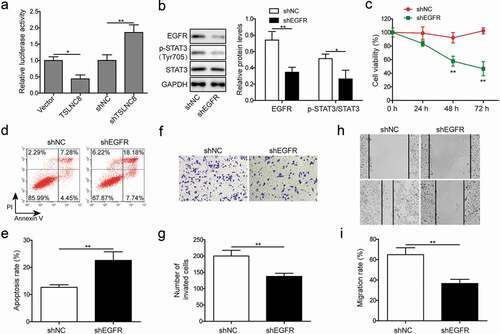
STAT3 inhibition suppresses proliferation, apoptosis, migration and invasion of lung cancer cells
Next, we evaluated the effects of selective STAT3 inhibitor cryptotanshinone (4 μM) to verify the roles of STAT3 signaling in proliferation and tumorigenesis of lung cancer cells. Cryptotanshinone is a natural quinoid diterpene that inhibits the phosphorylation of STAT3 at Y705 [Citation33,Citation34]. We used western blotting to verify the effect of cryptotanshinone on STAT3 phosphorylation. In comparison to the DMSO-treated group, cryptotanshinone-treated H1975 cells exhibited down-regulation of p-STAT3 (Tyr705), whereas the expression level of EGFR remained essentially unaffected ()). Furthermore, results of MTT assay ()), flow cytometry of cell apoptosis assay (), transwell assay () and wound healing assay () consistently indicated that cryptotanshinone administration inhibited proliferation, migration and invasion, as well as stimulated apoptosis of human lung cancer cell line. These findings indicated that TSLNC8 likely inhibited cancer cell proliferation, migration and invasion via inhibition of STAT3 pathway.
Figure 6. STAT3 inhibition suppresses cell proliferation, apoptosis, migration and invasion. (a). Western blotting was performed to assess expression of EGFR and phosphorylation of STAT3 (Tyr705) in lung cancer cell line H1975 in response to cryptotanshinone treatment. GAPDH was used as a loading control. (b). MTT assay was performed to evaluate the effect of cryptotanshinone on proliferation of lung cancer cell line H1975. (c-d). Flow cytometry with Annexin V/PI staining was used to assess the effect of cryptotanshinone on apoptosis of lung cancer cell line H1975. (e-f). Transwell assay was used to determine the effect of cryptotanshinone on invasion ability of lung cancer cell line H1975. G-H. Wound healing assay was performed to evaluate the effect of cryptotanshinone on migration ability of lung cancer cell line H1975. Data were shown as mean±SD, the result was a representative of three independent experiments. ** p< 0.01 and * p< 0.05
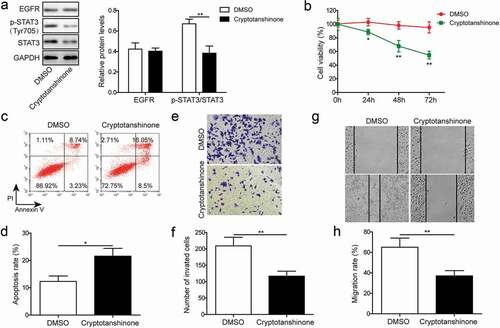
EGFR overexpression and STAT3 signaling activation reverse the effects of TSLNC8 on cell proliferation, apoptosis, migration and invasion
IL-6 is a cytokine that activates the transcription factor STAT3 in inflammation and cancers [Citation35]. To further validate that TSLNC8 modulates the EGFR-STAT3 signaling pathway, we overexpressed EGFR and induced activation of STAT3 via IL-6 addition (10 ng/mL) in H1975 cells (). As illustrated in ), the levels of EGFR and p-STAT3 (Tyr705) were up-regulated following overexpression of EGFR. After addition of IL-6, the expression level of p-STAT3 (Tyr705) was up-regulated, while the level of EGFR remained essentially unchanged ()). Meanwhile, after overexpression of EGFR, the effects of TSLNC8 overexpression on cell proliferation, apoptosis, invasion and migration were reversed (). Similarly, IL-6 stimulation was also found to reverse the effects of TSLNC8 overexpression on cell proliferation, apoptosis, invasion and migration (). Taken together, EGFR overexpression and STAT3 signaling activation counteracted the inhibitory effects of TSLNC8 on cancer cells.
Figure 7. EGFR overexpression and STAT3 signaling activation reverse the effects of TSLNC8 on proliferation, apoptosis, migration and invasion. (a). Western blotting was performed to determine the expression level of EGFR and phosphorylation level of STAT3 (Tyr705) in TSLNC8-overexpressing H1975 cells in response to EGFR overexpression or IL-6 stimulation. GAPDH was used as a loading control. (b). MTT assay was performed to evaluate the effect of EGFR overexpression or IL-6 stimulation on proliferation of H1975 cells that overexpressing TSLNC8. (c-d). Flow cytometry with Annexin V/PI staining was used to assess the influence of EGFR overexpression or IL-6 stimulation on apoptosis of H1975 cells that overexpressing TSLNC8. (e-f). Transwell assay was used to determine the effect of EGFR overexpression or IL-6 stimulation on invasion properties of H1975 cells that overexpressing TSLNC8. (g-h). Wound healing assay was performed to evaluate the effect of EGFR overexpression or IL-6 stimulation on migration ability of H1975 cells that overexpressing TSLNC8. Data were shown as mean±SD, the result was a representative of three independent experiments. ** p< 0.01 and * p< 0.05
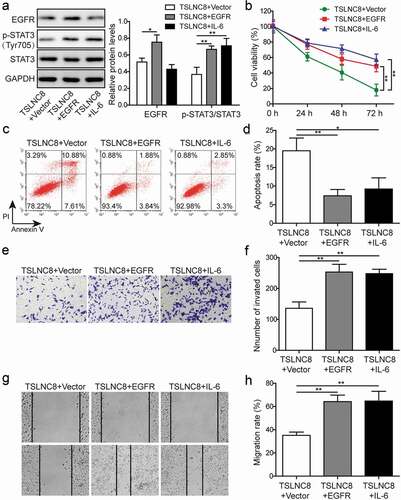
TSLNC8 enhances the inhibitory effects of osimertinib on tumor growth in vivo
The functions of TSLNC8 overexpression and osimertinib were further validated in nude mice lung cancer model. Tumor photographs ()), tumor growth curves ()) and tumor weights ()) indicated that tumor growth was inhibited by TSLNC8 overexpression or osimertinib administration, whereas the combination of TSLNC8 overexpression and osimertinib administration resulted in most efficient suppression of tumor growth. Consistent with the results on the cellular level ), TSLNC8 overexpression or osimertinib administration down-regulated the levels of EGFR, p-EGFR (Tyr1068) and p-STAT3 (Tyr705), while the combination of TSLNC8 overexpression and osimertinib administration exhibited most pronounced inhibition of EGFR, p-EGFR (Tyr1068) and p-STAT3 (Tyr705) levels (, e and g). In addition, the expression level of TSLNC8 in tumors was up-regulated in TSLNC8 overexpression groups, while osimertinib administration showed no effects on TSLNC8 expression ()). Thus, we have demonstrated that combination of osimertinib and TSLNC8 overexpression effectively suppressed tumor growth in vivo.
Figure 8. TSLNC8 enhances the inhibitory effects of osimertinib on tumor growth in vivo. (a). Photographs of tumors with TSLNC8 overexpression and/or osimertinib administration. (b). Tumor growth curves of mice model with TSLNC8 overexpression and/or osimertinib administration. Tumor volume (mm3) was measured every 5 days over 4 weeks. (c). Comparison of tumor weights (g) with TSLNC8 overexpression and/or osimertinib administration. (d-e). Western blotting was performed to detect expression of EGFR and phosphorylation of EGFR (Tyr1068) and STAT3 (Tyr705) in tumor tissues in response to TSLNC8 overexpression and/or osimertinib administration. GAPDH was used as a loading control. (f). Overexpression of TSLNC8 by transfection with pWPXL-TSLNC8 was confirmed by qRT-PCR. (g). Immunohistochemistry was performed to detect expression of EGFR and phosphorylation of STAT3 (Tyr705) in tumor tissues in response to TSLNC8 overexpression and/or osimertinib administration. Data were shown as mean±SD, the result was a representative of three independent experiments. ** p< 0.01 and * p< 0.05
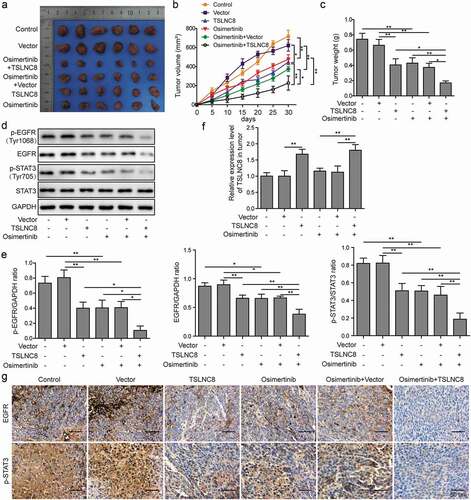
Discussion
Globally, lung cancer has been a major contributor of tumor-related death [Citation2,Citation36]. Despite the recent developments in diagnosis and therapeutic technologies, the prognosis of lung cancer remains disappointing [Citation4], exerting substantial financial and psychological burdens on patients and the society. It is therefore of great significance to uncover the molecular mechanisms behind the pathogenesis of lung cancer. In this work, we demonstrated that the expression of lncRNA TSLNC8 was down-regulated in clinical lung cancer tissues and human lung cancer cell lines and the therapeutic effects of osimertinib were greatly enhanced by TSLNC8 overexpression via inhibition of the EGFR-STAT3 pathway. Thus, the synergistic effects of TSLNC8 and osimertinib offered exciting opportunities for novel combination therapies that featured weaker chemoresistance, lower dosage and fewer side effects of osimertinib.
Numerous studies have shown that lncRNAs are intricately involved in the proliferation, metastasis and invasion of lung cancer [Citation1,Citation8–10]. For instance, lncRNA LINC00673 has been shown to bind LSD1 and promote NSCLC tumor growth [Citation9]. Overexpression of lncRNA LINC00473 has been revealed to be closely associated with the poor prognosis of LKB1-inactivated NSCLC [Citation36]. Additionally, lncRNA NEAT1 has been identified as a key regulator of NSCLC progression by serving as a competing endogenous RNA (ceRNA) of miR-377-3p [Citation10]. However, the specific roles of other lncRNAs in lung cancer remain poorly understood. LncRNA TSLNC8 has been shown to be significantly down-regulated in hepatocellular carcinoma and glioma [Citation20,Citation21]. Previously, Fan et.al reported that TSLNC8 was down-regulated in lung cancer cell lines, and significantly suppressed the proliferation and migration and promoted apoptosis of lung cancer cells via targeting IL-6/STAT3/HIF-1α signaling pathway [Citation22]. In this work, we attempted to test if aberrant expression of TSLNC8 was also implicated in clinical lung cancer tissues. Using qRT-PCR assay, we showed the expression of TSLNC8 was markedly down-regulated in both clinical lung cancer tissues () and a panel of human lung cancer cell lines (). In this work, we disclosed the first evaluation of TSLNC8 expression in lung cancer tissues, however, there were only 31 pairs of lung cancer samples. The number of samples is limited, and further studies will require more clinical samples. Intriguingly, TSLNC8 was remarkably increased in breast cancer tissues and cell lines, which inhibited the proliferation of breast cancer cells mediated by miR-214-3p/FOXP2 axis [Citation37].
In hepatocellular carcinoma, TSLNC8 has been shown to repress the phosphorylation and transcriptional activity of STAT3 [Citation20]. Accumulating evidence demonstrated that EGFR/STAT3 signaling pathway mediated tumor progression. For example, it was reported that IGFBP2 regulated PD-L1 expression by promoting EGFR nuclear accumulation and EGFR/STAT3 activation in melanoma cells [Citation38]. Yue et al. found that 2′-Hydroxyflavanone had anti-tumor efficacy and sensitized human pancreatic cancer cells to EGFR inhibitors via the inhibition of STAT3 [Citation39]. Li et al. reported that inhibition of DYRK1A sensitized EGFR wild-type NSCLC cells to AZD9291 by suppressing STAT3/EGFR/Met signaling pathway [Citation40]. Since dysregulated EGFR-STAT3 signaling pathway has been implicated in diverse cancer types, including lung cancer [Citation23–25], we suspected that a TSLNC8-EGFR-STAT3 regulatory axis might be relevant in lung cancer. Indeed, we discovered that the expression level of EGFR and phosphorylation level of STAT3 (Tyr705) were markedly increased in both clinical lung cancer tissues () and human lung cancer cell lines (), indicating that downregulation of TSLNC8 expression was linked to activation of the EGFR-STAT3 pathway.
To investigate in detail the effects of TSLNC8 on the proliferation and tumorigenesis of lung cancer cells, we induced overexpression or knockdown of TSLNC8 in cell line H1975 or H358. Using clone formation assay, flow cytometry of apoptosis assay, transwell assay and wound healing assay, we consistently found that TSLNC8 overexpression promoted cell apoptosis and suppressed cell proliferation, migration and invasion (), whereas TSLNC8 knockdown had opposite effects (). Osimertinib is a third-generation EGFR-TKI developed by AstraZeneca, and specifically inhibits EGFR with T790M mutation [Citation26,Citation27]. Accordingly, in this work, osimertinib administration was found to enhance apoptosis and inhibit cell proliferation, migration and invasion. The results in combination of osimertinib and TSLNC8 overexpression indicated that anti-tumor effects of osimertinib were substantially enhanced by TSLNC8. Moreover, the inhibitory effects of osimertinib on the expression of EGFR and phosphorylation of EGFR (Tyr1068) and STAT3 (Tyr705) were enhanced by TSLNC8 overexpression (), while these anti-tumor effects of osimertinib were inhibited by TSLNC8 knockdown (). This report represented the first assessment of synergistic application of TSLNC8 and osimertinib for lung cancer. There have been no reports of osimertinib being used in combination with other non-coding RNAs to increase its sensitivity up to now. The combination therapy using TSLNC8 and osimertinib has potential benefits of reducing dosage and side effects of osimertinib, which has important implications for the optimal clinical use of osimertinib. As for the effect of the combination therapy using TSLNC8 and osimertinib on other cancers, it is also worthy of further study in the future.
Although we have demonstrated the expression level of TSLNC8 was negatively correlated with the expression level of EGFR and phosphorylation of EGFR and STAT3 (–), the direct interaction between TSLNC8 and EGFR remains to be verified. We used the luciferase reporter assay to validate that TSLNC8 overexpression resulted in the decreased luciferase activity of EGFR, while TSLNC8 knockdown induced the increased luciferase activity of EGFR (). Therefore, TSLNC8 regulated the expression of EGFR, providing first support for the proposed TSLNC8-EGFR-STAT3 signaling axis in lung cancer. Next, we tested the effects of EGFR knockdown and a selective STAT3 inhibitor cryptotanshinone [Citation33,Citation34], on proliferation and tumorigenesis of lung cancer cells. EGFR knockdown and STAT3 pathway inhibition suppressed cell proliferation, migration and invasion, while stimulated apoptosis (–). In addition, cryptotanshinone administration reduced the phosphorylation level of STAT3 (Tyr705), while the expression of EGFR remained unchanged. It was reported that cryptotanshinone played its anti-tumor roles by targeting STAT3/SIRT3/HIF-1α signaling [Citation41]. The cytokine IL-6 is known to activate STAT3 in inflammation and cancers [Citation35]. In the current work, we showed that the addition of IL-6 or overexpression of EGFR reversed the effects of TSLNC8 overexpression on cell proliferation, apoptosis, invasion and migration, further verifying that TSLNC8 was an upstream regulator of the EGFR-STAT3 signaling pathway (). Finally, we validated the roles of TSLNC8 overexpression and osimertinib in nude mice model of lung cancer (). As a result, combination therapy of TSLNC8 overexpression and osimertinib administration efficiently suppressed tumor growth in vivo.
Taken together, the current study defined a regulatory axis consisting of TSLNC8, EGFR and STAT3 in lung cancer. Cell and animal model studies revealed that TSLNC8 overexpression remarkably enhanced the anti-tumor effects of osimertinib via inhibition of the EGFR-STAT3 signaling pathway. This work sets the stage for further in-depth mechanistic studies, and provides guidelines for the development of new combination therapy for lung cancer based on TSLNC8 and osimertinib.
List of abbreviations
LncRNA Long non-coding ribonucleic acid
NSCLC Non-small cell lung cancer
SCLC Small-cell lung cancer
shRNA Small hairpin ribonucleic acid
qRT-PCRQuantitative real-time polymerase chain reaction
PBS Phosphate-buffered saline
RPMI Roswell Park Memorial Institute
MTT 3-(4,5-dimethyl-2-thiazolyl)-2,5-diphenyl-2-H-tetrazolium bromide
EGFR Epidermal growth factor receptor
EGFR-TKI EGFR tyrosin kinase inhibitor
STAT3 Signal transducer and activator of transcription 3
Ethics approval
The use of these tissues was approved by the Ethics Committee of the First Affiliated Hospital of Henan University of CM, and each patient submitted a written informed consent.
Authors’ contribution
guarantor of integrity of the entire study: Su-Zhen Zhou;
study concepts: Su-Zhen Zhou;
study design: Ming-Hang Wang, Su-Zhen Zhou;
definition of intellectual content: Ming-Hang Wang;
literature research: Su-Zhen Zhou;
clinical studies: Su-Zhen Zhou, Han Li, Ming-Hang Wang, Zhi-Wan Wang, Ning Li, Yan-Fang Wang
experimental studies: Han Li, Su-Zhen Zhou;
data acquisition: Su-Zhen Zhou: Han Li, Ming-Hang Wang, Zhi-Wan Wang, Ning Li, Yan-Fang Wang;
data analysis: Su-Zhen Zhou, Han Li, Ming-Hang Wang;
statistical analysis: Han Li, Ming-Hang Wang;
manuscript preparation: Su-Zhen Zhou, Han Li;
manuscript editing: Su-Zhen Zhou, Han Li;
manuscript review: Su-Zhen Zhou, Han Li.
Acknowledgments
Not applicable.
Disclosure statement
The authors declare that no competing financial interest exists.
Data availability statement
All data generated or analyzed during this study are included in this published article [and its supplementary information files].
References
- Chen Z, Li JL, Lin S, et al. cAMP/CREB-regulated LINC00473 marks LKB1-inactivated lung cancer and mediates tumor growth. J Clin Invest. 2016;126(6):2267–2279.
- Siegel RL, Miller KD, Jemal A. Cancer Statistics. CA Cancer J Clin. 2017;67(1):7–30.
- Herbst RS, Heymach JV, Lippman SM. Lung cancer. N Engl J Med. 2008;359(13):1367–1380.
- De Angelis R, Sant M, Coleman MP, et al. Cancer survival in Europe 1999-2007 by country and age: results of EUROCARE–5-a population-based study. Lancet Oncol. 2014;15:23–34.
- Wood SL, Pernemalm M, Crosbie PA, et al. Molecular histology of lung cancer: from targets to treatments. Cancer Treat Rev. 2015;41(4):361–375.
- Spizzo R, Almeida MI, Colombatti A, et al. Long non-coding RNAs and cancer: a new frontier of translational research? Oncogene. 2012;31:4577–4587.
- Bartonicek N, Maag JL, Dinger ME. Long noncoding RNAs in cancer: mechanisms of action and technological advancements. Mol Cancer. 2016;15:43.
- Dong Y, Huo X, Sun R, et al. lncRNA Gm15290 promotes cell proliferation and invasion in lung cancer through directly interacting with and suppressing the tumor suppressor miR-615-5p. Biosci Rep. 2018;38(5):BSR20181150.
- Shi X, Ma C, Zhu Q, et al. Upregulation of long intergenic noncoding RNA 00673 promotes tumor proliferation via LSD1 interaction and repression of NCALD in non-small-cell lung cancer. Oncotarget. 2016;7(18):25558–25575.
- Sun C, Li S, Zhang F, et al. Long non-coding RNA NEAT1 promotes non-small cell lung cancer progression through regulation of miR-377-3p-E2F3 pathway. Oncotarget. 2016;7(32):51784–51814.
- Mercer TR, Dinger ME, Mattick JS. Long non-coding RNAs: insights into functions. Nat Rev Genet. 2009;10(3):155–159.
- Shi X, Sun M, Liu H, et al. Long non-coding RNAs: a new frontier in the study of human diseases. Cancer Lett. 2013;339(2):159–166.
- Kornienko AE, Guenzl PM, Barlow DP, et al. Gene regulation by the act of long non-coding RNA transcription. BMC Biol. 2013;11(1):59.
- Dykes IM, Emanueli C. Transcriptional and post-transcriptional gene regulation by long non-coding RNA. Genomics, Proteomics & Bioinformatics. 2017;15(3):177–186.
- Ginger MR, Shore AN, Contreras A, et al. A noncoding RNA is a potential marker of cell fate during mammary gland development. Proc Natl Acad Sci U S A. 2006;103:5781–5786.
- Cesana M, Cacchiarelli D, Legnini I, et al. A long noncoding RNA controls muscle differentiation by functioning as a competing endogenous RNA. Cell. 2011;147(2):358–369.
- Kretz M, Siprashvili Z, Chu C, et al. Control of somatic tissue differentiation by the long non-coding RNA TINCR. Nature. 2013;493(7431):231–235.
- Batista PJ, Chang HY. Long noncoding RNAs: cellular address codes in development and disease. Cell. 2013;152(6):1298–1307.
- Wang P, Xue Y, Han Y, et al. The STAT3-binding long noncoding RNA lnc-DC controls human dendritic cell differentiation. Science. 2014;344(6181):310–313.
- Zhang J, Li Z, Liu L, et al. Long noncoding RNA TSLNC8 is a tumor suppressor that inactivates the interleukin-6/STAT3 signaling pathway. Hepatology. 2018;67(1):171–187.
- Chen D, Yu X. Long noncoding RNA TSLNC8 suppresses cell proliferation and metastasis and promotes cell apoptosis in human glioma. Mol Med Rep. 2018;18:5536–5544.
- Fan H, Li J, Wang J, et al. Long non-coding RNAs (lncRNAs) tumor-suppressive role of lncRNA on chromosome 8p12 (TSLNC8) inhibits tumor metastasis and promotes apoptosis by regulating interleukin 6 (IL-6)/signal transducer and activator of transcription 3 (STAT3)/hypoxia-inducible factor 1-alpha (HIF-1alpha) signaling pathway in non-small cell lung cancer. Med Sci Monit. 2019;25:7624–7633.
- Fang B. Genetic interactions of STAT3 and anticancer drug development. Cancers (Basel). 2014;6(1):494–525.
- Gao SP, Mark KG, Leslie K, et al. Mutations in the EGFR kinase domain mediate STAT3 activation via IL-6 production in human lung adenocarcinomas. J Clin Invest. 2007;117(12):3846–3856.
- Zhao X, Sun X, Li XL. Expression and clinical significance of STAT3, P-STAT3, and VEGF-C in small cell lung cancer. Asian Pac J Cancer Prev. 2012;13(6):2873–2877.
- Cross DA, Ashton SE, Ghiorghiu S, et al. AZD9291, an irreversible EGFR TKI, overcomes T790M-mediated resistance to EGFR inhibitors in lung cancer. Cancer Discov. 2014;4(9):1046–1061.
- Janne PA, Yang JC, Kim DW, et al. AZD9291 in EGFR inhibitor-resistant non-small-cell lung cancer. N Engl J Med. 2015;372:1689–1699.
- Ward RA, Anderton MJ, Ashton S, et al. Structure- and reactivity-based development of covalent inhibitors of the activating and gatekeeper mutant forms of the epidermal growth factor receptor (EGFR). J Med Chem. 2013;56:7025–7048.
- Wang S, Cang S, Liu D. Third-generation inhibitors targeting EGFR T790M mutation in advanced non-small cell lung cancer. J Hematol Oncol. 2016;9:34.
- Sullivan I, Planchard D. Osimertinib in the treatment of patients with epidermal growth factor receptor T790M mutation-positive metastatic non-small cell lung cancer: clinical trial evidence and experience. Ther Adv Respir Dis. 2016;10(6):549–565.
- Remon J, Menis J, Hasan B, et al. The APPLE trial: feasibility and activity of AZD9291 (Osimertinib) treatment on positive plasma T790M in EGFR-mutant NSCLC patients. EORTC 1613. Clin Lung Cancer. 2017;18:583–588.
- Tang ZH, Lu JJ. Osimertinib resistance in non-small cell lung cancer: mechanisms and therapeutic strategies. Cancer Lett. 2018;420:242–246.
- Zhang Y, Jiang P, Ye M, et al. Tanshinones: sources, pharmacokinetics and anti-cancer activities. Int J Mol Sci. 2012;13:13621–13666.
- Chen W, Lu Y, Chen G, et al. Molecular evidence of cryptotanshinone for treatment and prevention of human cancer. Anticancer Agents Med Chem. 2013;13(7):979–987.
- Bromberg J, Wang TC. Inflammation and cancer: IL-6 and STAT3 complete the link. Cancer Cell. 2009;15(2):79–80.
- Chen W, Zheng R, Baade PD, et al. Cancer statistics in China, 2015. CA Cancer J Clin. 2016;66(2):115–132.
- Qin CX, Yang XQ, Jin GC, et al. LncRNA TSLNC8 inhibits proliferation of breast cancer cell through the miR-214-3p/FOXP2 axis. Eur Rev Med Pharmacol Sci. 2019;23:8440–8448.
- Li T, Zhang C, Zhao G, et al. IGFBP2 regulates PD-L1 expression by activating the EGFR-STAT3 signaling pathway in malignant melanoma. Cancer Lett. 2020;477:19–30.
- Yue Y, Qian W, Li J, et al. 2′-Hydroxyflavanone inhibits the progression of pancreatic cancer cells and sensitizes the chemosensitivity of EGFR inhibitors via repressing STAT3 signaling. Cancer Lett. 2020;471:135–146.
- Li YL, Ding K, Hu X, et al. DYRK1A inhibition suppresses STAT3/EGFR/Met signalling and sensitizes EGFR wild-type NSCLC cells to AZD9291. J Cell Mol Med. 2019;23:7427–7437.
- Yang Y, Cao Y, Chen L, et al. Cryptotanshinone suppresses cell proliferation and glucose metabolism via STAT3/SIRT3 signaling pathway in ovarian cancer cells. Cancer Med. 2018;7:4610–4618.