ABSTRACT
Bone marrow-derived mesenchymal stem cells (BM-MSCs) implantation shows a repair effect on erectile function in diabetes mellitus-induced erectile dysfunction (DMED) due to its differentiative capacity into endothelial cells (ECs) that contributes to endothelial repair. This study was designed to explore the functional role and mechanism of long noncoding RNA (lncRNA)-metastasis-associated lung adenocarcinoma transcript 1 (MALAT1) in BM-MSCs-mediated DMED repairing. The DMED rat model was established and the erectile function was evaluated by calculating the intracavernous pressure (ICP)/mean arterial pressure (MAP) ratio in the DMED models with or without BM-MSCs implantation. The differentiation of BM-MSCs toward ECs was assessed by measuring the expression of EC-specific genes. RNA pull-down and luciferase reporter assay were performed to explore the interaction between miR-206 and MALAT1 or VEGFA. BM-MSCs implantation improved the erectile function of DMED rats and increased MALAT1 expression. MALAT1 was time-dependently upregulated during the VEGF-induced BM-MSCs differentiation into ECs. Mechanistically, MALAT1 acted as a sponge of miR-206 to upregulate VEGFA expression, thereby promoting the differentiation of BM-MSCs into ECs. Moreover, MALAT1 silencing in vivo impaired the repairing effect of BM-MSCs on erectile dysfunction. Collectively, MALAT1 facilitates BM-MSCs differentiation into ECs via regulating miR-206/VEGFA axis.
Introduction
Erectile dysfunction (ED) is a common comorbidity in males with diabetes mellitus (DM) [Citation1]. The prevalence of ED is higher in DM patients than in the general population [Citation2,Citation3]. The pathophysiology of DM-induced ED (DMED) is very complex and associated with several factors, chiefly, endothelial dysfunction [Citation4]. Data show the response of DMED patients to first-line oral phosphodiesterase type 5 inhibitors is relatively poor compared to non-DM ED patients [Citation5]. Thus, developing new therapeutic approaches for DMED is of important clinical significance.
Recently, mounting evidence has strongly suggested that stem-cell therapy represents as a novel and promising therapeutic option for the treatment of DMED [Citation6,Citation7]. Among them, bone marrow-derived mesenchymal stem cells (BM-MSCs) have been used as an ideal source of cell transplantation for recovering DMED [Citation8,Citation9]. BM-MSCs have differentiative capacity into endothelial cells (ECs) that contribute to endothelial repair and thereby have therapeutic potential in ED [Citation10,Citation11]. However, the mechanisms by which BM-MSCs improve erectile function remain not fully elucidated.
Long noncoding RNAs (lncRNAs) are a class of non-coding RNAs greater than 200 nucleotides in length and are implicated in diverse pathological processes, such as cell proliferation, cell apoptosis, and cell differentiation [Citation12,Citation13]. Recent evidence has shown that lncRNAs are also involved in regulating erectile function by modulating differentiation of BM-MSCs into ECs [Citation10,Citation11]. Metastasis-associated lung adenocarcinoma transcript 1 (MALAT1), a tumor-related lncRNA [Citation14,Citation15], is also associated with DM. A previous study demonstrated that MALAT1 knockdown significantly alleviated diabetes-induced microvascular dysfunction in vivo and inhibited endothelial cell migration and tube formation in vitro [Citation16]. Nonetheless, whether MALAT1 controls BM-MSCs differentiating toward ECs has yet to be explored.
LncRNAs can act as competitive endogenous RNAs (ceRNAs) to segregate microRNAs (miRNAs) away from target mRNAs [Citation17–19]. Our bioinformatic analysis indicated that MALAT1 harbors binding sites of miR-206 that can target 3ʹ-UTR of vascular endothelial growth factor A (VEGFA, generally referred to as VEGF). VEGF has an inducing effect on the endothelial differentiation of BM-MSCs [Citation11,Citation20]. Based on this, we speculated that MALAT1 might act as a ceRNA of miR-206 to upregulate VEGFA expression, and thus promote the differentiation of BM-MSCs toward ECs. Hence, the present study was designed to explore whether MALAT1 controls BM-MSCs differentiating toward ECs and to validate whether the miR-206/VEGFA axis is involved in this process.
Materials and methods
Ethics statement
The animal experimental processes were approved by the Ethics Committee of the First Affiliated Hospital of Nanchang University and performed in strict accordance with the Guidelines for the Care and Use of Laboratory Animals of the National Institutes of Health.
Establishment of a DMED rat model
Sprague-Dawley (SD) male rats weighing 250–300 g (10 wk old) were maintained under specific pathogen-free conditions. After 1 wk of acclimatization, SD rats were intraperitoneally injected with 1% streptozotocin (60 mg/kg; Sigma-Aldrich, St. Louis, MO, USA) to induce a rat model of DM. After 72 h, the blood sugar content was measured, and the rats with the blood sugar content above 16.7 mmol/L were considered as the successful DM models. These DM model rats were subcutaneously injected with apomorphine (100 μg/kg, Sigma-Aldrich) on the necks to induce ED. The rat model of DMED was considered to be successfully established if animals exhibited the pathological features of DMED (glans penis hyperemia, increase of penis volume, and erection of the terminal phallosome).
Animal grouping and treatment
The animals were randomly assigned into six groups (n = 7/group): Control, DMED, DMED+PBS, DMED+BM-MSCs, DMED+BM-MSCs-scramble, DMED+BM-MSCs-MALAT1 shRNA. The rats in the control group received a regular diet without any additional treatment. The DMED model rats were injected with PBS (100 μL), untreated BM-MSCs (5 × 106 cells in 100 μL of PBS), or BM-MSCs infected with recombinant lentiviruses (5 × 106 cells in 100 μL of PBS) expressing MALAT1 shRNA or scrambled control shRNA into the corpora cavernosa.
Determination of ICP/MAP
The intra-cavernous pressure (ICP) and mean arterial pressure (MAP) were recorded and the ICP/MAP ratio was calculated to evaluate the erectile function in all rats as previously described [Citation9,Citation10]. Briefly, the rats were intraperitoneally anesthetized with ketamine (100 mg/kg) and midazolam (5 mg/kg), fixed on a sterile surgical table, and then underwent tracheal cannula to maintain airway patency. The right carotid artery was cannulated with a PE-50 tube and connected to a pressure transducer (Viggo Spectramed, Oxnard, CA, USA) for continuously monitoring MAP. A ventral midline incision was made to expose the main pelvic ganglion and cavernous nerve under an operative microscope. The cavernous nerve was surrounded with the electrode. A 23-gauge needle filled with 250 U/ml heparin and connected to PE-50 tube was inserted into the bottom of corpora cavernosa to measure the ICP. The electrical stimulation was at 5.0 mV and a frequency of 20 Hz with a pulse width of 5 ms for 1 min. Another electrical stimulation was repeated after 5 min.
Isolation and culture of BM-MSCs
BM-MSCs were isolated from male SD rats according to a previous study from our laboratory [Citation9]. The isolated BM-MSCs were cultured in Dulbecco’s Modified Eagle Medium (DMEM; Gibco, Carlsbad, CA, USA) supplemented with 10% fetal bovine serum (FBS, Gibco) in an incubator with 5% CO2 at 37°C. The BM-MSCs in the third generation were used for further experiments.
Cell transfection
The full-length sequences of MALAT1 were subcloned into pcDNA3.1 vector (Invitrogen, Thermo Fisher Scientific), named as pcDNA3.1-MALAT1. An empty pcDNA3.1 vector was used as a negative control (NC). MALAT1 siRNA (si-MALAT1), si-VEGFA, scramble siRNA controls, miR-206 mimic, and mimic NC were purchased from GenePharma (Shanghai, China). For cell transfection, BM-MSCs (1.5 × 105 cells/well) were cultured in 6-well plates overnight. When cell confluence reached 80%, cells were transfected with these plasmids, siRNAs, or miRNAs using Lipofectamine-2000 (Invitrogen).
Quantitative real-time PCR (qRT-PCR) analysis
Total RNA was extracted from rat corpora cavernosa or BM-MSCs using RNeasy mini kit (Qiagen, Hilden, Germany) and then reverse-transcribed to cDNA using iScript cDNA synthesis kit (Bio-Rad, Hercules, CA, USA). qRT-PCR was performed with cDNA products and gene-specific primers using SYBR Premix EX Taq (TaKaRa, Dalian, China). The levels of candidate genes were examined using the 2−ΔΔCt method. 18S was used as the reference gene for miR-206 and MALAT1. GAPDH was used for VEGFA, von Willebrand factor (vWF), vascular endothelial cadherin (VE-cadherin), and endothelial NO synthase (eNOS).
RNA pull-down assay
The 3ʹ-end biotin-labeled lncRNA MALAT1 or random pull-down probe sequence as a negative control (NC) were transcribed with reaction buffer containing Biotin RNA Labeling Mix and T7 RNA polymerase (Roche, Basel, Switzerland). Total RNA bound to MALAT1 from cell lysate was isolated using RNA pull-down assay. Briefly, BM-MSCs were washed twice with ice pre-cooled PBS solution, and then lysed in 1 mL 0.1% NP40 lysate containing protease inhibitor and centrifuged at 12000 × g for 5 min to extract supernatant. Biotinylated RNA was then incubated with streptavidin agarose-treated magnetic beads (Life Technologies, Thermo Fisher Scientific) and the cell lysates at room temperature for 1 h. The precipitated miR-206 level was detected by qRT-PCR.
Luciferase reporter assay
A dual-luciferase reporter assay was performed to verify the direct interaction between miR‐206 and MALAT1 or between miR-206 and 3ʹ‐UTR of VEGFA. The fragments of MALAT1 and VEGFA 3ʹUTR were amplified by PCR and then cloned into pGL3 vector. HEK293T cells were seeded at a density of 2 × 104 cells per well in 24-well plates. After 24 h, cells were co-transfected with wild type (WT) or mutant (Mut) MALAT1 luciferase reporter plasmids, and miR-206 mimic or mimic NC (GenePharma) by Lipofectamine 2000 (Invitrogen). Also, the WT or Mut VEGFA 3ʹ-UTR luciferase reporter plasmids were transfected in a similar way. The luciferase activity was analyzed after 48 h of transfection using a Dual-Luciferase Reporter Assay Kit (Promega, Madison, WI, USA).
Western blot
Total protein was retrieved from rat corpora cavernosa or BM-MSCs using radioimmunoprecipitation assay lysis buffer (Beyotime, Shanghai, China) and quantitatively analyzed using BCA Protein Assay Kit (Beyotime). Then, the protein samples were separated using 10% SDS-PAGE gel electrophoresis and then transferred to PVDF membranes. Subsequently, the membranes were blocked with 5% skim milk at room temperature for 2 h and then incubated with the primary antibodies against VEGFA and β-actin at a dilution of 1:1000 (Abcam, Cambridge, MA, USA) overnight at 4°C, followed by 2 h of incubation with the horseradish peroxidase-conjugate secondary antibody (1:2000, Santa Cruz Biotechnology, Dallas, TX, USA) at room temperature. The protein was detected with an enhanced chemiluminescence kit (Pierce Biotechnology, Rockford, IL, USA).
Statistical analysis
All statistical analyses were performed using SPSS version 20.0 (IBM, Chicago, IL, USA). Data are presented as the mean ± standard deviation from three independent experiments. The unpaired Student’s t-test was used to analyze differences between the two groups. One-way ANOVA was used to analyze differences among three or more groups. P < 0.05 was considered statistically significant.
Results
BM-MSCs implantation increased MALAT1 expression in DMED rats
Flow cytometry analysis showed that BM-MSCs were positive for CD29, CD105, CD44, and CD90 (MASC markers), while negative for CD34 and CD45 (). The ICP/MAP ratio was calculated to evaluate the erectile function in rats. Compared with the control rats, the ICP/MAP ratio was significantly reduced in DMED rats, indicating obvious ED in diabetic rats. As expected, BM-MSCs injection into the corpora cavernosa of DMED rats led to a notable increase in the ICP/MAP ratio ()). Meanwhile, MALAT1 expression was significantly lower in corpus cavernosum tissues from DMED rats than that in the control rats. Intriguingly, BM-MSCs injection obviously increased MALAT1 expression in corpus cavernosum tissues from DMED rats ()).
Figure 1. Identification of BM-MSCs by flow cytometry
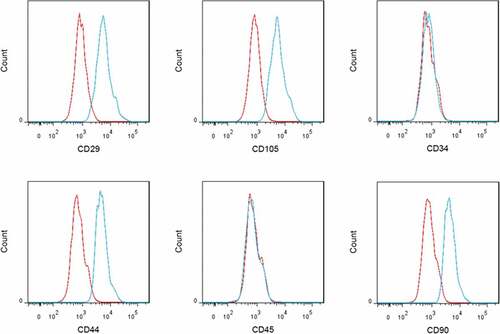
Figure 2. ICP/MAP ratio and MALAT1 expression in DMED rats with or without BM-MSCs implantation
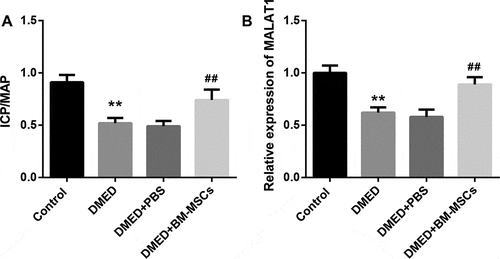
MALAT1 overexpression promoted, whereas MALAT1 silencing inhibited the differentiation of BM-MSCs into ECs
To determine the role of MALAT1 in the BM-MSCs-mediated DMED repairing, we treated BM-MSCs with VEGF to induce the differentiation of BM-MSCs toward ECs in vitro. The mRNA levels of ECs markers, including vWF, VE-cadherin, and eNOS, were markedly elevated in a time-dependent manner during the differentiation of BM-MSCs into ECs ()). Furthermore, expression of MALAT1 was also significantly and time-dependently upregulated throughout the VEGF-induced differentiation of BM-MSCs into ECs ()). We then explored the functional role of MALAT1 in regulating the differentiation of BM-MSCs into ECs. To this end, we overexpressed and silenced MALAT1 in BM-MSCs. The overexpression ()) and knockdown efficiencies ()) were confirmed by qRT-PCR. Data revealed that the mRNA levels of vWF, VE-cadherin, and eNOS were greatly increased following MALAT1 overexpression ()). In contrast, MALAT1 knockdown significantly decreased the mRNA levels of vWF, VE-cadherin, and eNOS ()).
Figure 3. MALAT1 overexpression promoted, whereas MALAT1 silencing inhibited the differentiation of BM-MSCs into ECs
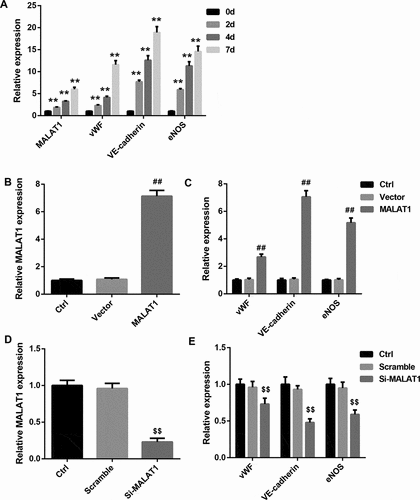
MALAT1 promoted the differentiation of BM-MSCs into ECs by regulating the miR-206/VEGFA axis
To unravel the underlying mechanisms of MALAT1 in the endothelial differentiation of BM-MSCs, we tested the interaction between MALAT1 and miR-206 as well as miR-206 and VEGFA 3ʹ-UTR. RNA pull-down assay showed a significantly higher abundance of miR-206 in Bio-MALAT1 group when compared with the Bio-NC group ()). Furthermore, miR-206 mimic resulted in adistinct reduction of luciferase activity in the MALAT1 WT group, further indicating that MALAT1 could directly bind to miR-206 ()). In addition, miR-206 mimic notably decreased the luciferase activity in the VEGFA WT group, whereas had no obvious effect on the luciferase activity in the VEGFA Mut group, suggesting that VEGFA was adirect target of miR-206 ()). Moreover, MALAT1 overexpression significantly decreased miR-206 expression, whereas prominently increased mRNA and protein levels of VEGFA in BM-MSCs ( and )). On the contrary, MALAT1 knockdown exerted the opposite effects on the expression of miR-206 and VEGF ( and )).
Figure 4. Effects of MALAT1 overexpression and silencing on expression of miR-206 and VEGFA
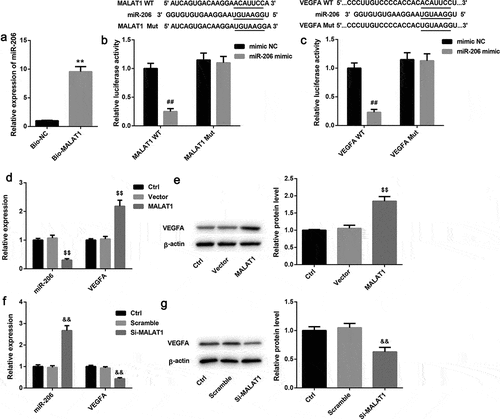
To determine whether MALAT1 acts as asponge of miR-206 to elevate VEGFA expression, the MALAT1-overexpressing BM-MSCs were transfected with miR-326 mimic. Data demonstrated that miR-206 mimic notably inhibited mRNA and protein levels of VEGFA and efficiently rescued the MALAT1 overexpression-mediated upregulation of VEGFA expression in BM-MSCs ( and )). Importantly, miR-206 mimic led to a prominent downregulation of vWF, VE-cadherin, and eNOS and reduced the MALAT1 overexpression-mediated upregulation of these EC-specific genes ()). Moreover, the MALAT1 overexpression-mediated upregulation of VEGFA, vWF, VE-cadherin, and eNOS was also effectively counteracted by VEGFA silencing (–)). Collectively, these results convincingly suggested that MALAT1 acted as a ceRNA of miR-206 to elevate VEGFA expression, thereby promoting BM-MSCs differentiation into ECs.
Figure 5. miR-206 mimic and VEGFA silencing abrogated the MALAT1 overexpression-induced VEGFA, vWF, VE-cadherin, and eNOS
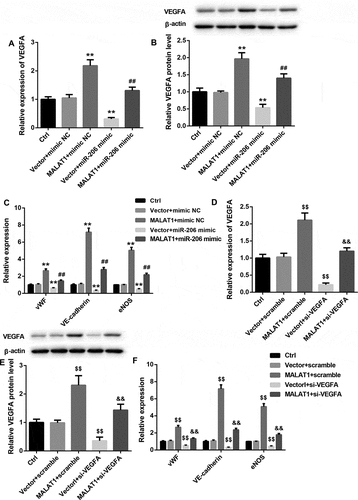
MALAT1 was involved in the repair effect of BM-MSCs implantation on erectile function in DMED rats
Finally, we evaluated the involvement of MALAT1 in the erectile function-repairing effect of BM-MSCs in DMED rats. To address this issue, BM-MSCs infected with MALAT1 shRNA-lentiviruses or scramble control shRNA-lentiviruses were injected into the corpora cavernosa of DMED rats. The ICP/MAP ratio in the DMED rats inoculated with MALAT1 shRNA-infected BM-MSCs was significantly reduced compared with the scramble shRNA-treated DMED rats ()). Meanwhile, qRT-PCR analysis confirmed that MALAT1 expression was notably lower in the DMED+BM-MSCs-MALAT1 shRNA group than that in the DMED+BM-MSCs-scramble group ()). In contrast to MALAT1 expression, miR-206 expression in the corpora cavernosa was higher in DMED rats than that in the controls but was reduced after intracavernous injection with BM-MSCs ()). However, VEGF mRNA and protein levels were decreased in DMED rats compared with the controls but were decreased after BM-MSCs implantation ( and )). Meanwhile, in the MALAT1 shRNA-treated group, miR-206 expression was significantly higher whereas VEGFA mRNA and protein levels were lower than that in the scramble shRNA-treated group ( and )). Furthermore, the expression pattern of EC-specific vWF, VE-cadherin, and eNOS was similar to that of MALAT1 and VEGFA ()). These data indicated that MALAT1 silencing reduced BM-MSCs repairing effect on erectile function in DMED rats, which might involve the MALAT1/miR-206/VEGFA axis-regulated BM-MSCs differentiation into ECs.
Figure 6. Effect of MALAT1 silencing on ED in vivo.
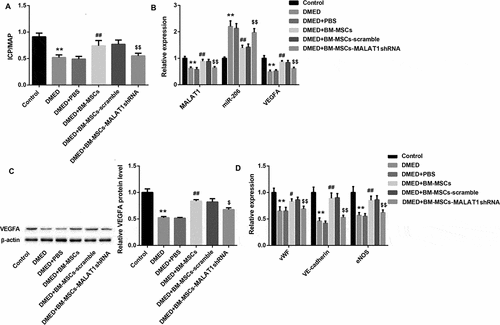
Discussion
Studies have affirmed the efficiency of intracavernous and periprostatic implantations of BM-MSCs for recovering and alleviating DMED [Citation9–11]. BM-MSCs accelerate repair of erectile function, which can be attributed to its potential to differentiate toward ECs in rat cavernosa [Citation21]. Recent studies have emphasized the important role of lncRNAs in improving erectile function by regulating the differentiation of BM-MSCs into ECs [Citation10,Citation11]. For example, in a previous report from our laboratory, intracavernous implantation of BM-MSCs effectively improved the erectile function of DMED rats, accompanied by a notable downregulation of lncRNA maternally expressed gene 3 (MEG3); and the downregulation of MEG3 was further confirmed to facilitate the differentiation of BM-MSCs toward ECs [Citation10]. Also, Wang et al. [Citation11] found that BM-MSCs restored erectile function in ED rats by upregulating the expression of lncRNA myocardial infarction-associated transcript (MIAT), while lncRNA MIAT promoted the differentiation of BM-MSCs into ECs by targeting miR-200a. MALAT1 is often regarded as a tumor-related lncRNA, but its function in erectile function and mesenchymal stem-cell differentiation remains unclear. In the present study, we provided the first evidence that BM-MSCs attenuated erectile dysfunction in DMED rats, at least partially, by upregulating MALAT1. These results provide new insights into the mechanisms underlying the BM-MSCs-mediated DMED repairing.
In the current study, we found that intracavernous implantation of BM-MSCs effectively restored the DMED-mediated reduction of ICP/MAP ratio and MALAT1 expression in DMED model rats, suggesting that the BM-MSCs therapy-mediated improvement of erectile function might be closely related to MALAT1 upregulation. We then found that MALAT1 expression was time-dependently upregulated during the differentiation of BM-MSCs toward ECs. More importantly, gain- and loss-of-function assays indicated that MALAT1 promoted the differentiation of BM-MSCs into ECs. Endothelial dysfunction has been recognized as a mainstay in the pathophysiology of DMED [Citation4]. Thus, our in vitro studies suggest that MALAT1 is involved in the BM-MSCs-mediated improvement of erectile function. Our further in vivo data showed that MALAT1 knockdown reduced the repair effects of BM-MSCs implantation on erectile function in DMED rats. These findings here elucidated the functional role of MALAT1 in BM-MSCs differentiation process and suggested that MALAT1 might be a novel regulator for the BM-MSCs-mediated protective effects for DMED.
LncRNAs can exert roles by acting as ceRNAs to segregate miRNAs away from target mRNAs, leading to suppression of miRNA-mediated functional roles [Citation17–19]. MALAT1 is an abundant, ubiquitously expressed lncRNA and has been reported to play a key role in the progression of various diseases, especially cancer [Citation22–24]. One important mechanism responsible for the functions of MALAT1 is its capacity to sponge certain miRNAs by acting as a ceRNA [Citation25–27]. For example, Gao et al. [Citation28] suggested that MALAT1 promoted osteogenic differentiation of human BM-MSCs by sponging miR-143 to upregulate osterix expression. In this investigation, results of RNA pull-down and luciferase reporter assay confirmed the interaction between miR-206 and MALAT1 as well as the interaction between miR-206 and 3ʹ-UTR of VEGFA mRNA. Further rescue experiments further suggested that miR-206 mimic transfection suppressed the MALAT1 overexpression-mediated upregulation of VEGFA mRNA and protein levels. These findings suggested that MALAT1 acted as a sponge of miR-206 to upregulate VEGFA expression.
VEGFA is a common variant of VEGF, generally referred to as VEGF. Several reports have demonstrated that VEGF promotes the endothelial differentiation of BM-MSCs [Citation11,Citation20]. Moreover, a previous study has shown that VEGF gene therapy using a nonviral gene delivery system improved erectile function in a diabetic rat model [Citation29]. As expected, our results in this study showed that VEGFA silencing attenuated the MALAT1 overexpression-induced upregulation of EC-specific vWF, VE-cadherin, and eNOS. Importantly, miR-206 mimic exerted an endothelial differentiation-inhibitory effect similar to that of VEGFA silencing. To our knowledge, this study is the first to demonstrate that miR-206 inhibits the endothelial differentiation of BM-MSCs. Furthermore, the MALAT1 overexpression-induced upregulation of EC-specific vWF, VE-cadherin, and eNOS could be abrogated by both miR-206 mimic and VEGFA silencing, indicating that MALAT1 promoted BM-MSCs differentiation into ECs via regulating the miR-206/VEGFA axis.
Conclusion
In summary, the current study demonstrated that MALAT1 was upregulated by BM-MSCs implantation and facilitated BM-MSCs differentiation into ECs by regulating the miR-206/VEGFA axis. These findings provide new mechanistic insights into the BM-MSCs-mediated DMED repairing.
Disclosure statement
The authors declare no conflicts of interest.
Additional information
Funding
References
- Burnett AL, Nehra A, Breau RH, et al. Erectile dysfunction: AUA guideline. J Urol. 2018;200:633–641.
- Malavige LS, Levy JC. Erectile dysfunction in diabetes mellitus. J Sex Med. 2009;6:1232–1247.
- Xu Y, Zhang Y, Yang Y, et al. Prevalence and correlates of erectile dysfunction in type 2 diabetic men: a population-based cross-sectional study in Chinese men. Int J Impot Res. 2019;31:9–14.
- Castela A, Costa C. Molecular mechanisms associated with diabetic endothelial-erectile dysfunction. Nat Rev Urol. 2016;13:266–274.
- Balhara YP, Sarkar S, Gupta R. Phosphodiesterase-5 inhibitors for erectile dysfunction in patients with diabetes mellitus: A systematic review and meta-analysis of randomized controlled trials. Indian J Endocrinol Metab. 2015;19:451–461.
- Zhang HB, Chen FZ, He SH, et al. In vivo tracking on longer retention of transplanted myocardin gene-modified adipose-derived stem cells to improve erectile dysfunction in diabetic rats. Stem Cell Res Ther. 2019;10:208.
- Yiou R. Stem-cell therapy for erectile dysfunction. Biomed Mater Eng. 2017;28:S81–s5.
- Matsuda Y, Sasaki M, Kataoka-Sasaki Y, et al. Intravenous Infusion of Bone Marrow-Derived Mesenchymal Stem Cells Reduces Erectile Dysfunction Following Cavernous Nerve Injury in Rats. Sex Med. 2018;6:49–57.
- Sun X, Luo LH, Feng L, et al. B cell lymphoma-2-modified bone marrow-derived mesenchymal stem cells transplantation for the treatment of diabetes mellitus-induced erectile dysfunction in a rat model. Urol Int. 2017;98:358–366.
- Sun X, Luo LH, Feng L, et al. Down-regulation of lncRNA MEG3 promotes endothelial differentiation of bone marrow derived mesenchymal stem cells in repairing erectile dysfunction. Life Sci. 2018;208:246–252.
- Yan L, Guo N, Cao Y, et al. miRNA145 inhibits myocardial infarction-induced apoptosis through autophagy via Akt3/mTOR signaling pathway in vitro and in vivo. Int J Mol Med. 2018;42:1537–1547.
- Cao J. The functional role of long non-coding RNAs and epigenetics. Biol Proced Online. 2014;16:11.
- Chen X, Sun Y, Cai R, et al. Long noncoding RNA: multiple players in gene expression. BMB Rep. 2018;51:280–289.
- Shen F, Zheng H, Zhou L, et al. Overexpression of MALAT1 contributes to cervical cancer progression by acting as a sponge of miR-429. J Cell Physiol. 2019;234:11219–11226.
- Liu W, Zhang Q, Zhang J, et al. Long non-coding RNA MALAT1 contributes to cell apoptosis by sponging miR-124 in Parkinson disease. Cell Biosci. 2017;7:19.
- Liu JY, Yao J, Li XM, et al. Pathogenic role of lncRNA-MALAT1 in endothelial cell dysfunction in diabetes mellitus. Cell Death Dis. 2014;5:e1506.
- Wang P, Chen D, Ma H, et al. LncRNA MEG3 enhances cisplatin sensitivity in non-small cell lung cancer by regulating miR-21-5p/SOX7 axis. Onco Targets Ther. 2017;10:5137.
- Xiong D, Li Z, Liang L, et al. The LncRNA NEAT1 Accelerates Lung Adenocarcinoma Deterioration and Binds to Mir-193a-3p as a Competitive Endogenous RNA. Cell Physiol Biochem. 2018;48:905–918.
- Gu J, Wang Y, Wang X, et al. Effect of the LncRNA GAS5-MiR-23a-ATG3 axis in regulating autophagy in patients with breast cancer. Cell Physiol Biochem. 2018;48:194–207.
- Wang N, Zhang R, Wang SJ, et al. Vascular endothelial growth factor stimulates endothelial differentiation from mesenchymal stem cells via Rho/myocardin-related transcription factor–a signaling pathway. Int J Biochem Cell Biol. 2013;45:1447–1456.
- Song YS, Lee HJ, Park IH, et al. Potential differentiation of human mesenchymal stem cell transplanted in rat corpus cavernosum toward endothelial or smooth muscle cells. Int J Impot Res. 2007;19:378–385.
- Gutschner T, Hammerle M, Diederichs S. MALAT1 – a paradigm for long noncoding RNA function in cancer. J Mol Med (Berl). 2013;91:791–801.
- Chen Y, Huang W, Sun W, et al. LncRNA MALAT1 Promotes cancer metastasis in osteosarcoma via activation of the PI3K-Akt signaling pathway. Cell Physiol Biochem. 2018;51:1313–1326.
- Xu Y, Zhang X, Hu X, et al. The effects of lncRNA MALAT1 on proliferation, invasion and migration in colorectal cancer through regulating SOX9. Mol Med (Cambridge, MA). 2018;24:52.
- Feng C, Zhao Y, Li Y, et al. LncRNA MALAT1 promotes lung cancer proliferation and gefitinib resistance by acting as a miR-200a sponge. Arch Bronconeumol. 2019;55:627–633.
- Liu S, Qiu J, He G, et al. LncRNA MALAT1 acts as a miR-125a-3p sponge to regulate FOXM1 expression and promote hepatocellular carcinoma progression. J Cancer. 2019;10:6649–6659.
- Yuan X, Guo Y, Chen D, et al. Long non-coding RNA MALAT1 functions as miR-1 sponge to regulate Connexin 43-mediated ossification of the posterior longitudinal ligament. Bone. 2019;127:305–314.
- Gao Y, Xiao F, Wang C, et al. Long noncoding RNA MALAT1 promotes osterix expression to regulate osteogenic differentiation by targeting miRNA-143 in human bone marrow-derived mesenchymal stem cells. J Cell Biochem. 2018;119:6986–6996.
- Dall’Era JE, Meacham RB, Mills JN, et al. Vascular endothelial growth factor (VEGF) gene therapy using a nonviral gene delivery system improves erectile function in a diabetic rat model. Int J Impot Res. 2008;20:307–314.